DOI:
10.1039/C7RA13391J
(Paper)
RSC Adv., 2018,
8, 3899-3902
Synthesis of C14–C21 acid fragments of cytochalasin Z8 via anti-selective aldol condensation and B-alkyl Suzuki–Miyaura cross-coupling†
Received
17th December 2017
, Accepted 15th January 2018
First published on 22nd January 2018
Abstract
An efficient synthesis of the C14–C21 acid fragment of cytochalasin Z8 was accomplished in 10 steps with 14% overall yield. Boron-mediated anti-selective aldol condensation and Pd(OAc)2–Aphos-Y-catalysed B-alkyl Suzuki–Miyaura cross-coupling were employed to construct the requisite C17 and C18 stereogenic centres and alkene subunit.
Cytochalasins are secondary fungal metabolites with a wide range of biological activities that target cytoskeletal processes.1 Cytochalasins Z7–Z9 (1–3, Chart 1) were isolated from the marine-derived fungus Spicaria elegans, and their structures and absolute configurations were established by Zhu et al.2 Cytochalasin Z8 (2, Chart 1) is structurally related to cytochalasins Z7 and Z9 and features highly substituted hydroisoindol-1-one fused with a 12-membered macrolactone ring at the C-8 and C-9 positions. Cytochalasin Z8 has been reported to exert cytotoxicity against P388 and A-549 cell lines with IC50 values of 56 and 21 μM, respectively, and therefore has significant potential in cell biology and medicine. A number of laboratories have worked towards total synthesis of the cytochalasin family and developed linear3 or convergent4 strategies for their total synthesis. Total synthesis of cytochalasin congeners was accomplished by the laboratories of Stork,3a,4a Thomas,3b,3c,3e,3f Trost,4d Vedejs (zygosporin E),4b,4c,4e Myers,5 Liu and Tang (periconiasins A–E)6 and Nay (periconiasin G).7 To the best of our knowledge, total synthesis of cytochalasin with a 12-membered macrocyclic ring has not been reported. The intriguing molecular architecture and potent biological activity of cytochalasin Z8 prompted us to pursue its total synthesis and render it to be readily available for biological investigations.
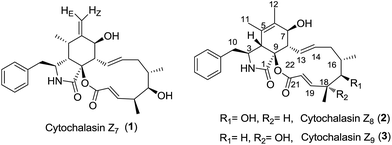 |
| Chart 1 Structures of cytochalasin Z7–Z9. | |
The retrosynthetic strategy is depicted in Scheme 1. Intramolecular ring-closing metathesis (RCM) strategy8 which is a promising tool for constructing macrolactone is often used for synthesising macrolides.9 We envisioned an RCM reaction at C13 and C14 positions and an esterification for assembling a 12-membered macrolactone. Thus, acid fragment 4 was required for the total synthesis of 2. Our strategy was flexible and it allowed rapid access to structural analogues. In this study, we report the synthesis of C14–C21 acid fragment 4 via a highly anti-selective aldol condensation10 of aldehyde 6 with Abiko's chiral norephedrine-derived propionate (1R,2S)-7 (ref. 11) and B-alkyl Suzuki–Miyaura cross-coupling12 of chiral alkyl iodide 5 with (Z)-1-bromoprop-1-ene.
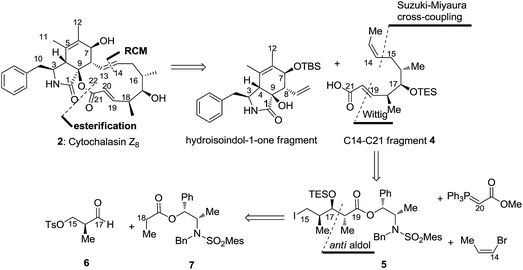 |
| Scheme 1 Retrosynthetic bond disconnections of cytochalasin Z8 (2) yielding C14–C21 acid fragment 4 and hydroisoindol-1-one fragment. | |
Our first task was to construct C16–C18 syn–anti stereotriad.13 The aldehyde functionality in 6 was expected to undergo an anti-selective aldol reaction with the (E)-boron enolate generated from Abiko's chiral propionate 7 for installing C17–C18 anti stereochemistry according to our synthetic strategy in Scheme 1. We initially prepared crude aldehyde 6 from commercially available (S)-methyl 3-hydroxy-2-methyl propionate (Roche ester)14 by tosylation and partial ester reduction15 (Scheme 2). The unstable crude aldehyde 6, without column chromatographic purification, was immediately used with the (E)-boron enolate derived from 7 for anti-selective aldol reaction to secure the syn/anti stereotriad in 8. The key intermediate 8 was prepared in high diastereoselectivity of 98
:
2 (determined by proton nuclear magnetic resonance spectroscopy) and in the desired absolute configuration as predicted by the chiral auxiliary in 7. The influence of the stereogenic centre of aldehyde 6 on the stereochemical course of the aldol reaction was not observed. The hydroxyl group in 8 was then protected as TES ether 9 (TESOTf, 2, 6-lutidine, 98% yield). Iodide replacement of the tosylate group in 9 with LiI–THF furnished alkyl iodide 5 in 95% yield (Scheme 2).
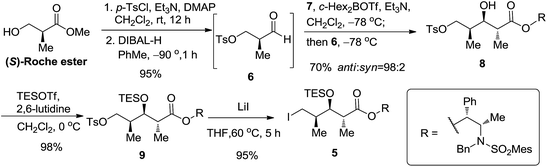 |
| Scheme 2 Synthesis of alkyl iodide 6. | |
The cross-coupling reaction of chiral alkyl iodide 5 with (Z)-1-bromoprop-1-ene was performed under the established conditions16 for the ‘9-MeO-9-BBN variant’ of the B-alkyl Suzuki–Miyaura cross-coupling reaction.12f,17 Alkyl iodide 5 was treated with t-BuLi in the presence of 9-MeO-9-BBN in Et2O–THF to form the corresponding borinate species which was subjected to Pd(OAc)2–Aphos-Y-catalysed16,18 cross-coupling reaction with (Z)-1-bromoprop-1-ene in the presence of K3PO4·3H2O as the base in THF–H2O at room temperature to furnish 11 in 15% yield along with cyclopentanol 10 and deiodinated byproduct 12 (entry 1, Table 1). We speculated that cyclopentanol byproduct 10 would be formed in the following pathway. Treatment of 5 with t-BuLi formed alkyllithium which underwent an intramolecular cycloaddition to form cyclopentanone; cyclopentanol 10 was formed by the addition of t-BuLi (Scheme 3). These results suggested that the formation of 10 could be suppressed by controlling reaction temperature. The first step reaction was maintained under low temperatures for a long time before warming up. After adding t-BuLi and THF, the reaction temperature was sequentially kept at −78 °C for 30 min, at −40 °C for 30 min, at −20 °C for 30 min and at room temperature for 2 h. The newly formed borinate species was subjected to coupling reaction with (Z)-1-bromoprop-1-ene. The yield was improved to 40% (entry 3, Table 1), and deiodinated byproduct 12 was inhibited to a large extent but could not be eliminated (in 10% yield). This condition might be associated with the steric hindrance imposed by the bulky TES and Abiko's chiral ester moieties of 5.
Table 1 Results of cross coupling of chiral alkyl iodide 5 with (Z)-1-bromoprop-1-ene
Entry |
Conditions |
Conditions |
Yielda (%) |
Step 1 |
Step 2 |
Isolated yield of product 11. Data in the parentheses are the isolated yields of cyclopentanol 10 and deiodinated byproduct 12, respectively. |
1 |
2.8 eq. t-BuLi, 3.0 eq. 9-MeO-9-BBN, Et2O/THF, −78 °C then r.t. for 2 h |
5.0 mol% Pd(OAc)2, 7.5 mol% Aphos-Y, 3.0 eq. K3PO4·3H2O, 18.0 eq. H2O, THF, r.t. (14 h) |
15; (21; 15) |
2 |
3.8 eq. t-BuLi, 5.0 eq. 9-MeO-9-BBN, Et2O/THF, −78 °C (30 min), −40 °C (30 min), −20 °C (90 min), r.t. (2 h) |
5.0 mol% Pd(OAc)2, 7.5 mol% Aphos-Y, 3.0 eq. K3PO4·3H2O, 18.0 eq. H2O, THF, r.t. (14 h) |
32; (19; 32) |
3 |
4.0 eq. t-BuLi, 4.5 eq. 9-MeO-9-BBN, Et2O/THF, −78 °C (30 min), −40 °C (30 min), −20 °C (90 min), r.t. (2 h) |
10 mol% Pd(OAc)2, 15 mol% Aphos-Y, 3.0 eq. K3PO4·3H2O, 18.0 eq. H2O, THF, r.t. (12 h) |
40; (17; 10) |
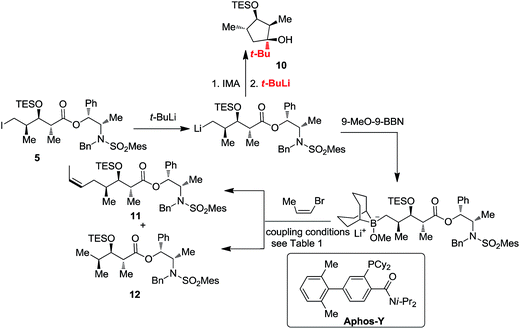 |
| Scheme 3 Cross-coupling of chiral alkyl iodide 5 with (Z)-1-bromoprop-1-ene. | |
The completion of the total synthesis of acid fragment 4 is illustrated in Scheme 4. Reduction of 11 with DIBAL-H provided the resultant primary alcohol 13 in 75% isolated yield. Dess–Martin periodinane oxidation19 in the presence of NaHCO3 converted 13 into the corresponding aldehyde 14. Aldehyde 14 was subjected to Wittig olefination with the stabilised ylide, Ph3P
CHCO2Me, in toluene at 60 °C to produce α,β-unsaturated ester 15 with exclusive E configuration for the newly formed disubstituted double bond. The hydrolysis of methyl ester 15 with LiOH in THF/H2O at room temperature furnished the target C14–C21 acid fragment 4 in 72% overall yield for the three steps.
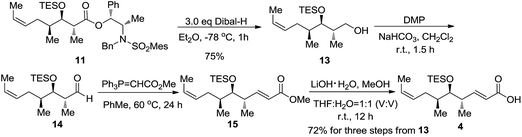 |
| Scheme 4 Synthesis of C14–C21 acid fragment 4. | |
Conclusions
We developed a concise synthesis of the C14–C21 acid fragment 4 of cytochalasin Z8. The anti-selective aldol reaction of 6 with the (E)-boron enolate derived from Abiko's chiral propionate 7 achieved the desired C16–C18 syn/anti stereotriad in high diastereoselectivity presumably attained via a reagent control process. (Z)-Alkene functionality was introduced by the Pd(OAc)2–Aphos-Y-catalysed C(sp2)–C(sp3) bond formation reaction of chiral alkyl iodide 5 with (Z)-1-bromoprop-1-ene. The target acid fragment 4 could be prepared from chiral (S)-Roche ester by a 10-step sequence in an overall yield of 14%. The strategy is concise and flexible to produce additional analogues of cytochalasin Z8 in enantiomerically pure form. Efforts to achieve this goal are ongoing in our laboratory.
Conflicts of interest
There are no conflicts to declare.
Acknowledgements
We are grateful for financial support from the National Natural Science Foundation of China (Project no. 21172191).
Notes and references
-
(a) M. Binder and C. Tamm, Angew. Chem., Int. Ed., 1973, 12, 370 CrossRef CAS PubMed
;
(b) F. Wang, H. Wei, T. Zhu, D. Li, Z. Lin and Q. Gu, Chem. Biodiversity, 2011, 8, 887 CrossRef CAS PubMed
;
(c) J. Wang, Z. Wang, Z. Ju, J. Wan, S. Liao, X. Lin, T. Zhang, X. Zhou, H. Chen and Z. Tu, Planta Med., 2015, 81, 160 CrossRef CAS PubMed
;
(d) J. Xu, RSC Adv., 2015, 5, 841 RSC
. - R. Liu, Q. Gu, W. Zhu, C. Cui, G. Fan, Y. Fang, T. Zhu and H. Liu, J. Nat. Prod., 2006, 69, 871 CrossRef CAS PubMed
. -
(a) G. Stork and E. Nakamura, J. Am. Chem. Soc., 1983, 105, 5510 CrossRef CAS
;
(b) H. Dyke, R. Sauter, P. Steel and E. J. Thomas, J. Chem. Soc., Chem. Commun., 1986, 1447 RSC
;
(c) E. J. Thomas and J. W. F. Whitehead, J. Chem. Soc., Chem. Commun., 1986, 727 RSC
;
(d) E. J. Thomas and J. W. F. Whitehead, J. Chem. Soc., Perkin Trans. 1, 1989, 499 RSC
;
(e) E. Merifield and E. J. Thomas, J. Chem. Soc., Chem. Commun., 1990, 464 RSC
;
(f) E. Merifield and E. J. Thomas, J. Chem. Soc., Perkin Trans. 1, 1999, 3269 RSC
. -
(a) G. Stork, Y. Nakahara, Y. Nakahara and W. J. Greenlee, J. Am. Chem. Soc., 1978, 100, 7775 CrossRef CAS
;
(b) E. Vedejs and J. G. Reid, J. Am. Chem. Soc., 1984, 106, 4617 CrossRef CAS
;
(c) E. Vedejs, J. D. Rodgers and S. J. Wittenberger, J. Am. Chem. Soc., 1988, 110, 4822 CrossRef CAS
;
(d) B. M. Trost, M. Ohmori, S. A. Boyd, H. Okawara and S. J. Brickner, J. Am. Chem. Soc., 1989, 111, 8281 CrossRef CAS
;
(e) E. Vedejs, J. G. Reid, J. D. Rodgers and S. J. Wittenberger, J. Am. Chem. Soc., 1990, 112, 4351 CrossRef CAS
. - A. M. Haidle and A. G. Myers, Proc. Natl. Acad. Sci. U. S. A., 2004, 101, 12048 CrossRef CAS PubMed
. - C. Tian, X. Lei, Y. Wang, Z. Dong, G. Liu and Y. Tang, Angew. Chem., Int. Ed., 2016, 55, 6992 CrossRef CAS PubMed
. - M. Zaghouani, C. Kunz, L. Guédon, F. Blanchard and B. Nay, Chem.–Eur. J., 2016, 22, 15257 CrossRef CAS PubMed
. -
(a) R. H. Grubbs and S. Chang, Tetrahedron, 1998, 54, 4413 CrossRef CAS
;
(b) A. Fürstner, Angew. Chem., Int. Ed., 2000, 39, 3012 CrossRef
;
(c) T. M. Trnka and R. H. Grubbs, Acc. Chem. Res., 2001, 34, 18 CrossRef CAS PubMed
;
(d) R. R. Schrock and A. H. Hoveyda, Angew. Chem., Int. Ed., 2003, 42, 4592 CrossRef CAS PubMed
;
(e) S. P. Nolan and H. Clavier, Chem. Soc. Rev., 2010, 39, 3305 RSC
;
(f) J. W. Herndon, Coord. Chem. Rev., 2010, 254, 103 CrossRef CAS
;
(g) G. C. Vougioukalakis and R. H. Grubbs, Chem. Rev., 2010, 110, 1746 CrossRef CAS PubMed
;
(h) A. H. Hoveyda, S. J. Malcolmson, S. J. Meek and A. R. Zhugralin, Angew. Chem., Int. Ed., 2010, 49, 34 CrossRef CAS PubMed
;
(i) A. Fürstner, Chem. Commun., 2011, 47, 6505 RSC
;
(j) S. Kotha and M. K. Dipak, Tetrahedron, 2012, 68, 397 CrossRef CAS
;
(k) A. Fürstner, Science, 2013, 341, 1229713 CrossRef PubMed
. - B. Thirupathi and D. K. Mohapatra, RSC Adv., 2014, 4, 8027 RSC
. -
(a) B. Schetter and R. Mahrwald, Angew. Chem., Int. Ed., 2006, 45, 7506 CrossRef CAS PubMed
;
(b) L. M. Geary and P. G. Hultin, Tetrahedron: Asymmetry, 2009, 20, 131 CrossRef CAS
. -
(a) A. Abiko, J.-F. Liu and S. Masamune, J. Am. Chem. Soc., 1997, 119, 2586 CrossRef CAS
;
(b) T. Inoue, J.-F. Liu, D. C. Buske and A. Abiko, J. Org. Chem., 2002, 67, 5250 CrossRef CAS PubMed
;
(c) A. Abiko, Acc. Chem. Res., 2004, 37, 387 CrossRef CAS PubMed
. -
(a) S. R. Chemler, D. Trauner and S. J. Danishefsky, Angew. Chem., Int. Ed., 2001, 40, 4544 CrossRef CAS
;
(b) K. C. Nicolaou, P. G. Bulger and D. Sarlah, Angew. Chem., Int. Ed., 2005, 44, 4442 CrossRef CAS PubMed
;
(c) S. Kotha and K. Mandal, Chem.–Asian J., 2009, 4, 354 CrossRef CAS PubMed
;
(d) A. Suzuki, Angew. Chem., Int. Ed., 2011, 50, 6722 CrossRef CAS PubMed
;
(e) R. Jana, T. P. Pathak and M. S. Sigman, Chem. Rev., 2011, 111, 1417 CrossRef CAS PubMed
;
(f) G. Seidel and A. Furstner, Chem. Commun., 2012, 48, 2055 RSC
;
(g) M. M. Heravi and E. Hashemi, Tetrahedron, 2012, 68, 9145 CrossRef CAS
. - R. W. Hoffmann, Angew. Chem., Int. Ed. Engl., 1987, 26, 489 CrossRef
. -
(a) C. Aïssa, R. Riveiros, J. Ragot and A. Fürstner, J. Am. Chem. Soc., 2003, 125, 15512 CrossRef PubMed
;
(b) D. A. Kummer, J. B. Brenneman and S. F. Martin, Org. Lett., 2005, 7, 4621 CrossRef CAS PubMed
. - H. Li, J. Wu, J. Luo and W.-M. Dai, Chem.–Eur. J., 2010, 16, 11530 CrossRef CAS PubMed
. -
(a) N. Ye and W. M. Dai, Eur. J. Org. Chem., 2013, 2013, 831 CrossRef CAS
;
(b) Y. Wu, Y. Lai and W.-M. Dai, ChemistrySelect, 2016, 1, 1022 CrossRef CAS
. -
(a) A. Fürstner and G. Seidel, Tetrahedron, 1995, 51, 11165 CrossRef
;
(b) J. A. Soderquist, K. Matos, A. Rane and J. Ramos, Tetrahedron Lett., 1995, 36, 2401 CrossRef CAS
. - J. Jin, Y. Chen, Y. Li, J. Wu and W.-M. Dai, Org. Lett., 2007, 9, 2585 CrossRef CAS PubMed
. -
(a) D. B. Dess and J. C. Martin, J. Org. Chem., 1983, 48, 4155 CrossRef CAS
;
(b) S. D. Meyer and S. L. Schreiber, J. Org. Chem., 1994, 59, 7549 CrossRef CAS
.
Footnote |
† Electronic supplementary information (ESI) available: Experimental details and scanned copies of 1H and 13C NMR spectra for new compounds. See DOI: 10.1039/c7ra13391j |
|
This journal is © The Royal Society of Chemistry 2018 |
Click here to see how this site uses Cookies. View our privacy policy here.