DOI:
10.1039/C7RA13583A
(Paper)
RSC Adv., 2018,
8, 11375-11387
A study on the impact of water quality on the murrel fish Channa striata and Channa punctata from three major Southern Tamilnadu rivers, India†
Received
22nd December 2017
, Accepted 6th March 2018
First published on 22nd March 2018
Abstract
Rivers are one of the natural fresh water resources that satisfy the domestic, agricultural and industrial needs of people. The Cauvery, Vaigai and Thamirabarani are the three major rivers flowing through the Southern Tamil Nadu region of India. In this study, the Water Quality Index (WQI) and heavy metal concentrations of river water during the pre- and post-monsoon periods in 2015 were recorded and the impact of heavy metal accumulation in two important murrel fish species, Channa striata and Channa punctata, was analysed using micronuclei and histology assays. The results revealed that the WQI was greater than 50 in most sites, indicating poor water quality for the sustainability of living organisms. The Heavy metal Pollution Index (HPI) was critical with values >100 in a few sites along the Cauvery and the Vaigai. The bioaccumulation of heavy metals was higher in the C. punctata than in the C. striata in most instances. Cd, Cu and Pb showed higher bioaccumulation in the pre-monsoon samples whereas As, Cr and Zn exhibited higher bioaccumulation during the post monsoon period. The Ni bioconcentration was consistent in both of the periods. The accumulation of heavy metals in the fish organs was as follows: gills > liver > kidney > muscle. The muscles exhibited Hazard Quotient (HQ) values 0.05–3.3 × 10−6 times lower than the Reference Dose (RfD) level, indicating no significant health risk from the intake of these metals through the consumption of their muscle tissues. The MN% was 0.19–0.22% in the C. striata, 0.15–0.25% in the C. punctata from heavily polluted sites and less polluted sites showed 0.05% and 0.07% MN in the C. striata and C. punctata, respectively. The MN% in the pre-monsoon samples was higher than that of the post-monsoon samples and the C. punctata had a higher MN% than the C. striata. The gills of the fish with high bioaccumulation showed severe lamellar fusion, hyperplasia, hypertrophy and epithelial lifting, their liver hepatocytes showed necrosis of the parenchymal cells and vacuolation, their Kidney tubules were dilated and vacuolated glomeruli with no Bowman's space were observed. Their muscles had normal myotomes with equally spaced muscle bundles. The C. punctata showed more severe histopathological changes than the C. striata. Thus, the present study defines a warning alarm for the proper remediation steps to be taken to safeguard the natural water resources as well as the aquatic ecosystem.
1. Introduction
Water is the elixir of life that cannot be substituted by any other resource. Rivers are one of the major water sources that satisfy the essential needs of living organisms. The Cauvery, the Vaigai and the Thamirabarani are three major rivers flowing through the Southern Districts of Tamil Nadu, India. The Cauvery flows across two states of India, namely Karnataka and Tamil Nadu. The Vaigai streams through the Theni, Madurai, Sivagangai and Ramanathapuram districts of Tamil Nadu. The Tirunelveli and Tuticorin districts of Tamil Nadu intensively depend on the River Thamirabarani for their water.1,2 The rate of utilization of these rivers by varied populations in rural, urban and semi-urban areas for agriculture, fisheries, and domestic and industrial purposes is enormous.3
In aquatic environments, heavy metals are found naturally due to withering of rocks and geographical location but nowadays due to urbanization, and anthropogenic activities from agricultural and urban land, the release of partially or untreated sewage/industrial waste into water bodies has led to an increased concentration of heavy metals in aquatic environments.4–6 This leads to undesirable changes in the physico-chemical parameters of water, thus decreasing the Water Quality Index (WQI), making it unsafe for living organisms and favoring bioaccumulation and bioconcentration7,8 by affecting the aquatic ecosystem.
In ecotoxicological studies, fish (aquatic vertebrates) serve as excellent subjects to study the pollution in water samples as they metabolize, concentrate and bioaccumulate water pollutants.9 There is concern for fish, which have been widely preferred for consumption among the public in recent days due to their protein, omega-3 fatty acids and vitamin richness, as they can be contaminated with toxic metals from polluted aquatic environments.10–12 The accumulation of heavy metals in nature leads to bioconcentration and biomagnification across the food chain in ecosystems, which ultimately adversely affects human health.
Channa striata and Channa punctata, which belong to the Channidae family, are carnivorous, bottom feeding and edible fresh water fish. They are available throughout the year.13 Trace elements occur in water and sediment of low concentration. In higher concentrations, they may have harmful effects on murrel fish.14 Therefore, in the present study based on the WQI and Heavy metal Pollution Index (HPI) reports from the post-monsoon season of 2014, sites of the Cauvery, Vaigai and Thamirabarani rivers with low, moderate and high levels of pollution were chosen and fish were analysed for bioaccumulation and bioconcentration in various organs. The effect of pollution was analyzed in these fish using micronuclei (red blood cells) and histology assays (gill, liver, kidney and muscle tissue) to evaluate the impact of heavy metal accumulation and bioconcentration in these fish.
2. Materials and methods
2.1 Study area
Eight different sites from each of the three major rivers in the Southern Tamil Nadu region [Cauvery, Vaigai and Thamirabarani] where chosen (Fig. 1, Table 1) for analysis in this study.
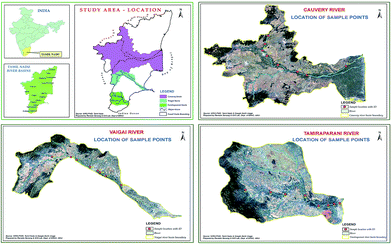 |
| Fig. 1 Sampling sites in three river systems. | |
Table 1 Geographical locations of the sampling sites
Site ID |
Location |
Latitude |
Longitude |
Cauvery river |
C1 |
Mettur dam |
11° 47′ 52.58′′ N |
77° 48′ 25.11′′ E |
C2 |
Singampettai |
11° 35′ 57.46′′ N |
77° 44′ 3.29′′ E |
C3 |
Bhavani |
11° 25′ 51.38′′ N |
77° 40′ 56.36′′ E |
C4 |
Kokkarayanpettai |
11° 19′ 55.36′′ N |
77° 46′ 38.48′′ E |
C5 |
Semmadapalayam |
11° 05′ 41.25′′ N |
78° 0′ 21.20′′ E |
C6 |
Musiri |
10° 56′ 26.52′′ N |
78° 26′ 43.54′′ E |
C7 |
Allur |
10° 52′ 4.03′′ N |
78° 38′ 46.00′′ E |
C8 |
Anaikarai |
11° 8′ 31.76′′ N |
79° 26′ 51.09′′ E |
![[thin space (1/6-em)]](https://www.rsc.org/images/entities/char_2009.gif) |
Thamirabarani river |
T1 |
Papanasam |
8° 42′ 46.01′′ N |
77° 22′ 7.93′′ E |
T2 |
Seranmahadevi |
8° 42′ 4.26′′ N |
77° 33′ 55.38′′ E |
T3 |
Melapalayam |
8° 42′ 58.78′′ N |
77° 41′ 58.95′′ E |
T4 |
Vallanadu |
8° 42′ 41.21′′ N |
77° 50′ 12.99′′ E |
T5 |
Alwarthirunagari |
8° 36′ 30.07′′ N |
77° 56′ 37.12′′ E |
T6 |
Eral |
8° 37′ 8.41′′ N |
78° 1′ 12.26′′ E |
T7 |
Authoor |
8° 37′ 33.99′′ N |
78° 4′ 13.06′′ E |
T8 |
Punnaikayal |
8° 38′ 0.97′′ N |
78° 6′ 47.63′′ E |
![[thin space (1/6-em)]](https://www.rsc.org/images/entities/char_2009.gif) |
Vaigai river |
V1 |
Vaigai Dam |
10° 2′ 59.82′′ N |
77° 35′ 34.15′′ E |
V2 |
Annaipatti |
10° 5′ 20.45′′ N |
77° 51′ 10.16′′ E |
V3 |
Sholavanthan |
10° 1′ 31.05′′ N |
77° 57′ 23.49′′ E |
V4 |
Arapalayam |
9° 56′ 15.56′′ N |
78° 6′ 23.53′′ E |
V5 |
Viraganoor |
9° 54′ 4.98′′ N |
78° 10′ 3.57′′ E |
V6 |
Silaiman |
9° 52′ 28.71′′ N |
78° 11′ 25.88′′ E |
V7 |
Tiruppuvanam |
9° 49′ 42.89′′ N |
78° 15′ 28.90′′ E |
V8 |
Manamadurai |
9° 41′ 47.88′′ N |
78° 27′ 5.46′′ E |
2.2 Collection of water samples
Water samples were collected in sterile polypropylene bottles during the pre- (March and April) and post-monsoon (September and October) seasons of 2015. In the pre-monsoon season of 2015, the Vaigai river had no incessant water flow in most of the selected sites and hence for the Vaigai river and so the water quality of the 2016 pre-monsoon period was monitored and used for the pre-monsoon analysis.
2.3 Analysis of physico-chemical parameters
Physical parameters such as the pH, Electrical Conductivity (EC), Total Dissolved Solids (TDS) and salinity were determined using a Eutech PCTestr 35. The chemical parameters were analyzed via Winkler's method for Dissolved Oxygen (DO), the Ethylene Diamine Tetra Acetic acid (EDTA) titrimetric method for the Total Hardness (calcium (Ca2+) and magnesium (Mg2+), and other parameters such as chloride (Cl−), sulfate (SO42−), nitrate (NO3−), and phosphate (PO43−) were analyzed by argentometric, gravimetric, ultraviolet spectrophotometric screening and ascorbic acid methods, respectively.15
The samples for heavy metal analysis were pre-treated with 1% of concentrated nitric acid for acidification. Further, the samples were stored in polypropylene containers at 4 °C until the analysis was performed. Heavy metals such as arsenic (As), cadmium (Cd), chromium (Cr), lead (Pb), copper (Cu), nickel (Ni) and zinc (Zn) were analyzed by Inductively Coupled Plasma-Mass Spectrometry (ICP-MS) (Nex ION 300X-Perkin Elmer).
2.4 Water index
2.4.1 Water quality index. The water quality index was calculated based on the weighted arithmetic method using the 10 physico-chemical parameters analyzed.16,17
The quality rating scale (Qi) for each parameter was calculated using the following expression:
where,
Vi = the estimated concentration of the
ith parameter in the analyzed water.
Vid = the ideal value of this parameter in pure water, these are = 0 (except pH = 7.0 and DO = 14.6 mg L
−1). Si = the recommended standard value of the
ith parameter.
The unit weight (Wi) for each water quality parameter was calculated using the following formula:
where,
K = the proportionality constant, which can be calculated using the following equation:
The heavy metal pollution index is a method of rating that shows the composite influence of individual heavy metals on the overall quality of water.18 In this indexing, weights (Wi) between 0 and 1 were assigned for each metal. The rating is based on the relative importance of individual quality considerations and is defined as being inversely proportional to the recommended standard (Si) for each parameter. Water quality and its suitability can be examined by determining its quality index.19–21
2.4.2 Heavy metal pollution index. The HPI is calculated using the following equation:.19
where, Wi is the unit weight of the ith parameter, Qi is the sub index of the ith parameter, and n is the number of parameters considered.The weighted arithmetic index method was used for calculation of the HPI. The unit weight (Wi) was found using the formula:
where,
K is the proportionality constant and
Si is the standard permissible value of the
ith parameter.
The sub index (Qi) of the parameter is calculated by:
where,
Mi is the monitored value of the heavy metal of the
ith parameter,
Ii is the ideal value of the
ith parameter which is taken from the BIS Standard;
32 and Si is the standard value of the
ith parameter, in ppb (μg L
−1). Agglomerative Hierarchical Clustering (AHC) was performed based on Ward's method and the Euclidean distance.
2.5 Fish collection
Live C. striata and C. punctata (n = 5 of each) were collected from three study sites in each river based on the level of water pollution (low, medium and high) during the post-monsoon period of 2014. The sites were C2, C5 and C8; V1, V3 and V5; and T4, T2 and T8 from the Cauvery, Vaigai and Thamirabarani rivers, respectively. The ranges of the standard lengths and weights of the adult fish were 20–35 cm, 180–220 g; and 15–25 cm, 80–150 g for C. striata and C. punctata, respectively.
2.6 Estimation of heavy metals in fish tissues
The fish were manually dissected and their organs were separated. Accumulated heavy metals such as As, Cr, Cd, Cu, Pb, Ni and Zn in the gill, liver, kidney and muscle tissues were analysed. The tissues were oven dried at 60 °C. The dried samples were ground into a fine powder using a pestle and mortar, and sieved (0.5–1.0 mm). The tissue samples, (1 g) were digested in 10 mL of HNO3
:
HClO4
:
H2SO4 (5
:
2
:
1 v/v) as described in earlier literature.22 Finally, the solution was filtered using Whatman filter paper (no. 42) and transferred into a 25 mL standard measuring cylinder. This was diluted with double distilled water and read on an Atomic Absorption Spectrophotometer (Perkin Elmer, Analyst A 800).
2.7 Bioconcentration factor (BCF)
The BCF is the ratio of the concentration of a specific metal in the tissue of an organism to the concentration of the same metal in the water.23
2.8 Health risk assessment for fish consumption
The average concentrations of heavy metals in all of the fish muscle samples and the daily fish consumption rates were used to calculate the Estimated Daily Intake of metals (EDI). The risks for fish consumption were assessed based on Hazard Quotients (HQ). The human health risk was evaluated based on the Acceptable Daily Intake (ADI) and the Reference Dose (RfD), which were previously found by the USEPA and JECFA.24,25 The EDI (μg kg−1 d−1) was computed using the following formula:26
where, CUCL is the exposure muscle tissue concentration upper confidence limit (μg g−1), and DCfish is the daily fish consumption (1.04 g per capita per day, FAO)27 of the target population. The target population Body Weight (BW) is taken as the average body weight of an Asian individual, which is 57.7 kg.28 The hazard quotient evaluates the health risk from fish consumption. A value of HQ ≤ 1 indicates no obvious risk.
2.9 Micronuclei assay
About 50 μL of peripheral blood from the caudal vein of C. striata and C. punctata was collected using 1 mL heparinized disposable syringes and transferred into sterile 1.5 mL Eppendorf tubes containing 450 μL of chilled Ca++ and Mg++ free phosphate buffered saline. The tubes were placed on ice till the micronuclei (MN) test was performed as per Nagpure et al., 2015.29 The peripheral blood was applied as thin smears on pre-cleaned slides, individually for each blood sample. Methanol fixation for 20 min was carried out, followed by air drying of the slides. 6% Giemsa (Merck) was used to stain the slides for 25 min. 2000 erythrocytes from each slide were examined at 100× magnification under a light microscope (Nikon Eclipse Ci2) equipped with a digital camera (Fig. 4). Small, non-refractive, circular or ovoid chromatin bodies displaying the same staining and focusing pattern as the main nucleus were scored as micronuclei.30 The MN frequency (%) was calculated as:
2.10 Histopathology
The fish tissues (gills, kidney, liver and muscles) were fixed in Bouin's fluid for 24 h, then histological processes were performed according to Bancroft and Cook.31 A microtome was used to make 4 μm thick sections and these were stained with hematoxylin and eosin (HE) for examination under the light microscope (Nikon Eclipse Ci2) at 10× and 40× and examined for histopathological modifications.
2.11 Statistical analysis
All of the analyses were done in triplicate and the values were expressed as mean ± standard deviation using SPSS 21.0. The raw values were subjected to the non-parametric Mann–Whitney test to evaluate the significance.
3. Results and discussion
3.1 Water quality index
The physico-chemical parameters analysed from the rivers during the pre-monsoon and post-monsoon periods of 2015 were compared with those obtained from the Bureau of Indian Standards (BIS) and World Health Organization Standards (WHO).32,33 The standard WQI values used for comparison are mentioned in Table 2. The WQIs calculated for the Cauvery, the Vaigai and the Thamirabarani are explained in Fig. 2. The Cauvery sampling sites C1 and C2 had a WQI of around 52, indicating a nearer to good quality of water, whereas the C5 and C8 sites are very poor for human utilization with a WQI greater than 75. In the Vaigai, sampling site V1 recorded a WQI of 45 during the post-monsoon season in 2015 whereas site V5 showed a maximum WQI of 88. A similar scenario was observed in the Thamirabarani at the T1 and T8 sites with WQIs of 50 and 80, respectively. Agglomerative Hierarchical Clustering (AHC) of the WQIs in the Cauvery classified them into 4 different clusters. In sites C1, C2 and C3 the water quality was better than that of the other sampling sites, and sites C5 and C8 were extremely polluted and formed separate classes (Fig. 2).
Table 2 Status of the water quality index based on earlier reports19
Water quality index range |
Status |
0–25 |
Excellent |
26–50 |
Good |
51–75 |
Poor |
76–100 |
Very poor |
Above 100 |
Unsuitable |
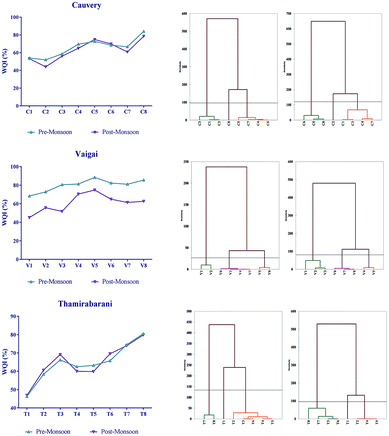 |
| Fig. 2 The water quality index and agglomerative hierarchical clustering based on Ward’s method and Euclidean distance. | |
In the Vaigai, V1 and V2 formed a class with a WQI of 40–50 and in the Thamirabarani, the T1 site was a separate class from the other sites with good water quality. In all of the cases, sites in the urban area had a poorer WQI than those in the rural sites. It is worth noting that the pH of the C5, C8 and T8 sites with low WQI was around 8.50, which is also one of the major factors contributing to the reduction of water quality. Based on earlier reports by Solairaj et al. (2010)34 about monsoon variations in the physico-chemical parameters of the Cauvery water during July–December 2007, the total dissolved solids (TDS) and phosphate levels were also beyond the standard levels. Similar results were found in this study which may be due to the agricultural, urban land runoffs and industrial effluents released into the river water. The initial sampling points in all of the cases were quite far away from anthropogenic activities, while the final sampling sites were completely located in the semi-urban to urban area with various land use patterns for agricultural, residential, commercial and industrial uses and hence, the possible sources of contamination were from agricultural runoff, sand mining, solid waste dumping, sewage and industrial runoff, ritual discards by pilgrims, etc.34–39
3.2. Heavy metal pollution
Among the 7 heavy metals analysed, the levels of arsenic, copper, cadmium and zinc were found to be within the standard limits. The heavy metal pollution index was high during the post-monsoon period compared to that during the pre-monsoon period. Fig. 3 describes the HPI and clustering of sites based on their pollution level. An HPI index above 100 is considered to be critical according to Prasad and Bose, 2001.40 The Cauvery C6 and C8 sites had HPIs of 102.07 and 106.90, respectively, the V5 and V6 sites of the Vaigai recorded maximum HPIs of 119.24 and 106.59, respectively. The Thamirabarani stream had no sites with an HPI > 100, the maximum HPI of 81.05 was observed at the T2 site.
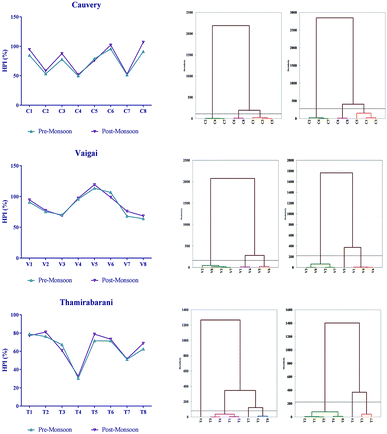 |
| Fig. 3 The heavy metal pollution index and agglomerative hierarchical clustering based on Ward’s method and Euclidean distance. | |
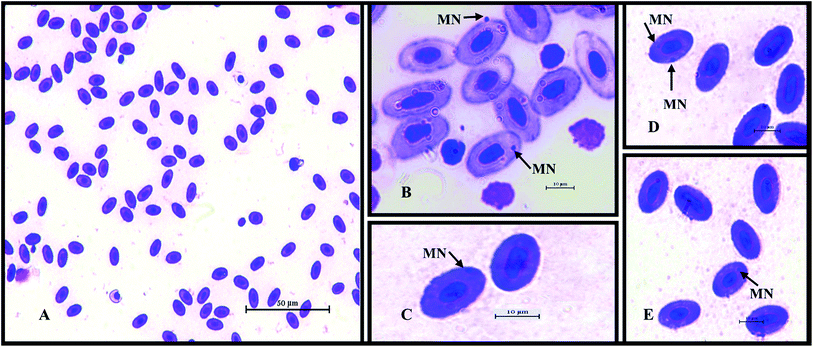 |
| Fig. 4 Representative microscopy images of Giemsa stained fish erythrocytes with micronuclei. (A) 40× and (B–E) 100× magnification under a light microscope [Nikon Eclipse Ci2]. | |
Based on heavy metal analysis in each river and its study sites for each metal, the sites exhibiting low, medium and high levels during the study period are shown below. The river Cauvery: As (C2, C5 and C8), Cd (C4, C2 and C8), Cr (C5, C7 and C1), Cu (C4, C6 and C5), Ni (C2, C7 and C8) Pb (C7, C2 and C3) and Zn (C2, C7 and C8); the river Vaigai: As (V1, V2 and V3), Cd (V1, V6 and V8), Cr (V3, V1 and V5), Cu (V8, V5 and V1), Ni (V8, V3 and V4) Pb (V4, V6 and V5) and Zn (V8, V6 and V1); and the river Thamirabarani: As (T8, T7 and T1), Cd (T1, T2 and T7), Cr (T8, T2 and T7), Cu (T4, T1 and T8), Ni (T8, T3 and T1) Pb (T7, T1 and T8) and Zn (T4, T6 and T1). Cadmium was found to be slightly higher (0.02 μg L−1) in the T5 and T7 sites of the river Thamirabarani. The heavy metal concentration of Cr, Ni and Pb were found to be 239 μg L−1, 139 μg L−1 and 20 μg L−1 in the Cauvery and 213 μg L−1, 89 μg L−1 and 22 μg L−1 in the Thamirabarani. This was observed in most of the sampling points in the urban limits because of the textile mill, pulp and paper industry effluents loaded with synthetic dyes, copper, zinc, lead, phenolics, and commercial and household waste accumulation.41–43 In contrast, the level of Cr was observed to be high in the C1 site of the Cauvery which might be due to withering of rocks. Earlier reports suggested that the heavy metal concentration in stream water may vary due to their low solubility and monsoonal variations. Heavy metal toxicity may lead to several neurogenetic, nephrotoxic disorders and ill health.44 The report of Dhanakumar et al. (2015)6 elucidated the bioaccumulation of heavy metals in fish liver, gill and kidney tissues. The accumulation of heavy metals in fish takes place when there are extremely high concentrations of metals in the environment primarily via the gills during ion exchange and then by ingestion of food or sedimentary particles through diet.
3.3 Bioaccumulation and bioconcentration factors of heavy metals in fish tissues
The levels of accumulation of heavy metals (As, Cd, Cr, Cu, Ni, Pb and Zn) in the gill, kidney, liver and muscle tissues were investigated. Fish mostly migrate in aquatic environments rather than settling in one place, and so metal accumulation in their organs provides evidence of their exposure to environmental pollutants in water.45 Fish, the primary constituent of aquatic food chains, can accumulate heavy metals from their food and environment.46,47 According to earlier reports, metal bioaccumulation by fish is a complex process that depends upon various exogenous and endogenous factors, subsequent distribution in organs is inter specific and influenced by species, sex, age, size, reproductive cycle, swimming patterns, feeding behaviour and living environment (geographical location).47,48
The bioconcentration factors, revealing the levels of water pollutant accumulation in the various fish organs, are listed in Table 3. Table 4 evidently proves the significant variation of bioconcentration in both of the study species in the pre- and post-monsoon periods with P < 0.5 for the non-parametric Mann–Whitney test.
Table 3 Maximum bioconcentration factors observed during the study period
Organs |
Metal |
Pre-monsoon |
Post-monsoon |
Channa striata |
Channa punctata |
Channa striata |
Channa punctata |
Cauvery |
Thamirabarani |
Vaigai |
Cauvery |
Thamirabarani |
Vaigai |
Cauvery |
Thamirabarani |
Vaigai |
Cauvery |
Thamirabarani |
Vaigai |
Gills |
As |
666.67 |
973.68 |
256.76 |
6125.00 |
1000.00 |
1095.00 |
3596.77 |
1315.79 |
351.35 |
5375.00 |
1593.75 |
893.94 |
Cd |
2500.00 |
1500.00 |
4166.67 |
4500.00 |
4000.00 |
3500.00 |
4000.00 |
3500.00 |
5000.00 |
5500.00 |
5000.00 |
7000.00 |
Cr |
9.05 |
48.55 |
16.87 |
8.00 |
23.27 |
26.07 |
7.71 |
49.11 |
17.93 |
7.76 |
16.61 |
28.46 |
Cu |
76.47 |
12.50 |
36.97 |
1149.28 |
115.38 |
45.40 |
58.82 |
12.50 |
35.21 |
654.68 |
163.46 |
52.84 |
Pb |
319.12 |
455.06 |
170.67 |
115.38 |
52.42 |
431.90 |
152.45 |
404.49 |
174.28 |
143.10 |
80.84 |
422.94 |
Ni |
36.19 |
45.21 |
9.48 |
37.44 |
74.10 |
25.42 |
35.29 |
59.79 |
9.74 |
39.42 |
49.44 |
28.61 |
Zn |
61.93 |
63.15 |
24.35 |
885.29 |
1120.16 |
302.57 |
54.79 |
59.05 |
29.58 |
1102.13 |
769.69 |
305.85 |
Kidney |
As |
666.67 |
675.44 |
696.81 |
11 875.00 |
875.00 |
770.00 |
2822.58 |
1092.11 |
283.78 |
3750.00 |
1359.38 |
772.73 |
Cd |
3500.00 |
0.00 |
0.00 |
100.00 |
6000.00 |
4500.00 |
4333.33 |
2500.00 |
4166.67 |
344.44 |
5000.00 |
6500.00 |
Cr |
8.12 |
18.69 |
14.10 |
6.45 |
18.67 |
22.60 |
6.38 |
0.70 |
16.63 |
4.51 |
15.69 |
30.62 |
Cu |
73.53 |
82.56 |
19.37 |
492.81 |
75.00 |
31.11 |
85.29 |
73.67 |
22.89 |
388.49 |
69.23 |
2409.90 |
Pb |
207.74 |
233.15 |
122.60 |
96.07 |
58.13 |
403.23 |
110.58 |
328.65 |
122.60 |
129.52 |
40.66 |
311.83 |
Ni |
17.99 |
33.57 |
8.93 |
35.76 |
46.59 |
10.43 |
18.19 |
46.92 |
6.95 |
23.41 |
34.82 |
22.32 |
Zn |
29.72 |
35.63 |
21.57 |
415.57 |
320.09 |
18.85 |
25.41 |
55.54 |
22.69 |
799.15 |
839.45 |
348.09 |
Liver |
As |
1000.00 |
697.37 |
162.16 |
21 750.00 |
1218.75 |
1045.00 |
1870.97 |
1350.88 |
85.11 |
3125.00 |
1750.00 |
575.76 |
Cd |
2000.00 |
0.00 |
0.00 |
1500.00 |
6000.00 |
3000.00 |
1000.00 |
1500.00 |
0.00 |
1500.00 |
3000.00 |
1500.00 |
Cr |
6.83 |
13.78 |
11.90 |
4.95 |
16.53 |
16.58 |
8.76 |
12.59 |
13.04 |
25.44 |
13.32 |
26.07 |
Cu |
94.12 |
6.76 |
76.92 |
413.67 |
1.98 |
9.45 |
17.65 |
5.69 |
88.24 |
190.65 |
53.85 |
2069.52 |
Pb |
167.46 |
168.54 |
84.13 |
137.44 |
62.87 |
152.33 |
169.04 |
179.78 |
122.60 |
204.19 |
29.94 |
275.99 |
Ni |
19.79 |
28.18 |
7.58 |
21.63 |
29.19 |
14.88 |
16.99 |
48.03 |
8.68 |
20.35 |
49.57 |
14.47 |
Zn |
42.20 |
53.23 |
17.68 |
737.95 |
273.36 |
14.24 |
24.16 |
35.18 |
19.91 |
2028.57 |
1085.45 |
264.08 |
Muscle |
As |
64.52 |
276.32 |
42.55 |
178.57 |
234.38 |
136.36 |
1000.00 |
460.53 |
40.54 |
130.95 |
812.50 |
255.00 |
Cd |
3333.33 |
0.00 |
0.00 |
0.00 |
0.00 |
0.00 |
3000.00 |
3000.00 |
0.00 |
0.00 |
0.00 |
0.00 |
Cr |
3.14 |
16.00 |
1.96 |
3.86 |
7.81 |
12.65 |
7.17 |
4.53 |
2.53 |
2.89 |
6.35 |
10.72 |
Cu |
17.65 |
1.07 |
1.76 |
401.08 |
13.03 |
7.79 |
8.82 |
1.07 |
2.93 |
100.72 |
4.25 |
5.56 |
Pb |
7.11 |
28.09 |
19.23 |
40.16 |
2.20 |
127.24 |
30.81 |
8.43 |
19.23 |
45.25 |
3.08 |
136.20 |
Ni |
3.20 |
16.54 |
1.78 |
6.32 |
29.12 |
8.36 |
3.00 |
14.70 |
2.32 |
7.68 |
12.72 |
7.56 |
Zn |
13.38 |
9.47 |
1.60 |
702.35 |
65.09 |
40.68 |
13.16 |
5.98 |
4.00 |
696.16 |
459.28 |
159.11 |
Table 4 An intergroup comparison of the bioconcentration factor based on species and monsoonal variation using the non-parametric Mann–Whitney test
Monsoon |
Pre |
Post |
P value |
Significance level |
Species |
C. striata |
C. striata |
|
|
Site |
Cauvery |
Cauvery |
0.91 |
Nil |
Site |
Vaigai |
Vaigai |
0.49 |
P < 0.5 |
Site |
Thamirabarani |
Thamirabarani |
0.23 |
P < 0.5 |
Monsoon |
Pre |
Post |
|
|
Species |
C. punctata |
C. punctata |
|
|
Site |
Cauvery |
Cauvery |
0.99 |
Nil |
Site |
Vaigai |
Vaigai |
0.23 |
P < 0.5 |
Site |
Thamirabarani |
Thamirabarani |
0.86 |
Nil |
Monsoon |
Pre |
Pre |
|
|
Species |
C. striata |
C. punctata |
|
|
Site |
Cauvery |
Cauvery |
0.04 |
P < 0.5 |
Site |
Vaigai |
Vaigai |
0.14 |
P < 0.5 |
Site |
Thamirabarani |
Thamirabarani |
0.26 |
P < 0.5 |
Monsoon |
Post |
Post |
|
|
Species |
C. striata |
C. punctata |
|
|
Site |
Cauvery |
Cauvery |
0.005 |
P < 0.05 |
Site |
Vaigai |
Vaigai |
0.6 |
Nil |
Site |
Thamirabarani |
Thamirabarani |
0.3 |
P < 0.5 |
Gills, the respiratory organs of fish, facilitate metal ion exchange from water45 along with toxic metals.49 Hence, water pollutants are concentrated in the gills. Pb, Cr, Zn, As, Cu and Ni flaunted the highest concentrations in the gills. Similarly, C. striata and C. punctata showed inter-specific variation of metal accumulation based on their age, geographical distribution and sex in this study, as reported in earlier literature.50 Similar results for high Pb concentrations in the gills were observed earlier in other species, indicating a negative correlation between the metal residues and feeding habitats in aquatic organisms.45,51–53
Table 5 shows the organ based bioconcentration between the species, with C. punctata exhibiting significantly greater bioconcentration than C. striata. Several earlier reports have also observed a higher level of bioaccumulation and genotoxicity in C. punctata.58 The bioaccumulation pattern observed in this study mainly followed the order given below:
Table 5 A comparison of the bioconcentration factor in both species based on monsoonal variation using the non-parametric Mann–Whitney test
Monsoon |
Pre |
Post |
P value |
Significance level |
Species |
C. striata, C. punctata |
C. striata, C. punctata |
|
|
Site |
Cauvery |
Cauvery |
0.26 |
P < 0.5 |
Site |
Vaigai |
Vaigai |
0.49 |
P < 0.5 |
Site |
Thamirabarani |
Thamirabarani |
0.23 |
P < 0.5 |
As gills > liver > kidney > muscle
Cd kidney > gills > liver > muscle
Cu gills > kidney > liver > muscle
Ni gills > liver > kidney > muscle
Pb gills > liver > kidney > muscle
Zn gills > kidney > liver > muscle
The accumulation of essential metals in the liver is due to their essential role in metabolism.47 The elevated Zn and Cu levels in the hepatic tissues were due to natural binding proteins such as metallothioneins (MT).54 On the other hand, the liver also showed high levels of non-essential metals such as Cd, As and Pb due to displacement of normal MT-associated essential metals in the hepatic tissues.55 Similar results of high Zn, Cu and Cd in the liver were observed in earlier literature.47,56,57 The kidney tissues also accumulated the heavy metals Cd, Cu, Cr, Zn and Ni next to the gills and liver. The lowest concentration of metals was observed in muscle tissues as the bioaccumulation of metals is a complex process.
The heavy metal levels varied among the fish organs and muscles. In most instances, Cd, Cu and Pb showed higher bioaccumulation in the pre-monsoon samples whereas As, Cr and Zn exhibited higher bioaccumulation in the post-monsoon samples than in the pre-monsoon samples. The Ni bioconcentration was consistent in both of the periods. Table 6 shows the significant variation in the bioconcentration of metals between the fish species studied based on the monsoonal and species level variation with P < 0.5 for non-parametric Mann–Whitney test.
Table 6 Comparison of the bioconcentration factor in both species based on organs accumulated using the non-parametric Mann–Whitney test
S. no. |
Metal |
Gill |
Liver |
Kidney |
Muscle |
Bold italics – significance level P < 0.5. Significance level P < 0.05. Maximum bioconcentration among the two-species studied; non-italicized – no significance. |
1 |
As |
C. punctata,c C. striata |
C. punctata,c C. striata |
C. punctata,c C. striata |
C. striata,c C. punctata |
2 |
Cd |
C. punctata,c C. striata |
C. punctata,c C. striatab |
C. punctata,c C. striata |
C. punctata,c C. striata |
3 |
Cr |
C. striata,c C. punctata |
C. punctata,c C. striata |
C. punctata,c C. striata |
C. striata,c C. punctata |
4 |
Cu |
C. punctata,c C. striatab |
C. punctata,c C. striata |
C. punctata,c C. striata |
C. punctata,c C. striata |
5 |
Pb |
C. punctata,c C. striata |
C. punctata,c C. striata |
C. punctata,c C. striata |
C. punctata,c C. striata |
6 |
Ni |
C. punctata,c C. striata |
C. punctata,c C. striata |
C. punctata,c C. striata |
C. punctata,c C. striata |
7 |
Zn |
C. punctata,c C. striatab |
C. punctata,c C. striata |
C. punctata,c C. striata |
C. punctata,c C. striatab |
To substantiate, the study revealed a higher bioaccumulation of heavy metals in the gills, liver, and kidney than in the muscles. The bioconcentration was higher in certain sites like C5 and V6 as there were sediments rich in heavy metals based on earlier reports.6,59 Thus, bioconcentration leads to biomagnification based on age, feeding habits (piscivorous or carnivorous) and residing habitats.
3.4 Hazard index in fish muscle tissues
The concentrations of metals in the present fish muscles were below the hazard quotient limits and are safe for human consumption as muscles are not an active site for metal biotransformation and accumulation.60 In general, benthic fish are known to have a higher bioaccumulation of heavy metals than those of upper water column fish.61–63 The behavioural and feeding pattern variation in fish also tends to variation in the bioaccumulation levels.49,56 The metal concentration differences for several heavy metals are based on the trophic levels of the fish.64–67 The Estimated Daily Intakes (EDIs) of heavy metals as per the EPA are shown in Table 7. The maximum permissible concentration of trace metals in fish is shown in Table 8. The HQs calculated showed those of As, Cd, Cu, Ni and Zn to be less than 1 (Table 9). The HQ was not calculated for Pb and Cr due to the non-availability of their RfD values. The EDI values obtained in this study were 0.05–3.3 × 10−6 times lower than the RfD values, indicating no significant health risks from the intake of heavy metals through the consumption of these fish muscles.
Table 7 Reference dosage values based on the environmental protection agency (EPA) – risk assessment information system (RAIS).24
Metal [CASNUM] |
Arsenic, inorganic [7440-38-2] |
Cadmium (Diet) [7440-43-9] |
Copper [7440-50-8] |
Nickel soluble salts [7440-02-0] |
Zinc and compounds [7440-66-6] |
Reference dose (RfD) (mg kg−1 d−1) |
0.03 |
1 |
40 |
20 |
300 |
Table 8 The maximum permissible limits (MPLs) of heavy metals in fish muscles (μg g−1) according to standards
S. no. |
Cd |
Cu |
Pb |
Ni |
Zn |
Reference |
1 |
0.5 |
30 |
0.5 |
8.97 |
30 |
FAO/WHO25 |
Table 9 Hazard quotient (HQ) values of fish muscle tissuesa
S. no. |
Metal |
Monsoon |
Cauvery |
Vaigai |
Thamirabarani |
Channa striata |
Channa punctata |
Channa striata |
Channa punctata |
Channa striata |
Channa punctata |
Pre |
Post |
Pre |
Post |
Pre |
Post |
Pre |
Post |
Pre |
Post |
Pre |
Post |
HQ < 1, safe for human consumption. |
1 |
As |
0.00120 |
0.00721 |
0.00481 |
0.00661 |
0.00240 |
0.00661 |
0.00240 |
0.01532 |
0.00631 |
0.00451 |
0.01051 |
0.01562 |
2 |
Cd |
0.00090 |
0.00000 |
0.00081 |
0.00000 |
0.00000 |
0.00000 |
0.00000 |
0.00000 |
0.00000 |
0.00000 |
0.00054 |
0.00000 |
3 |
Cu |
0.00001 |
0.00050 |
0.00001 |
0.00013 |
0.00001 |
0.00003 |
0.00001 |
0.00002 |
0.00001 |
0.00010 |
0.00001 |
0.00003 |
4 |
Ni |
0.000397 |
0.000798 |
0.000356 |
0.000969 |
0.000216 |
0.000937 |
0.000302 |
0.000982 |
0.000608 |
0.001059 |
0.000541 |
0.000991 |
5 |
Zn |
3.54 × 10−5 |
0.00099 |
3.48 × 10−5 |
0.000981 |
5.11 × 10−6 |
7.81 × 10−5 |
1.08 × 10−5 |
0.000306 |
1.26 × 10−5 |
5.86 × 10−5 |
6.31 × 10−6 |
0.000413 |
3.5 Micronuclei analysis
The MN% analysis revealed the genotoxic effect of the water quality affecting the aquatic life by use of fish vein blood samples and therefore the fish were also maintained alive.30,68,69 Increases in the MN% of 0.19–0.22% in the C. striata, and 0.15–0.25% in the C. punctata from heavily polluted sites were observed. The less polluted sites showed increases of 0.05% and 0.07% in the C. striata and C. punctata, respectively (Table 10). The high MN% in polluted sites were observed earlier.70 The MN% in the pre-monsoon samples was higher than that in the post-monsoon samples and C. punctata had a higher MN% than that of C. striata.
Table 10 Micronuclei % in C. striata and C. punctata
S. no. |
Monsoon |
Fish/rivers |
Cauvery |
Thamirabarani |
Vaigai |
Less |
Moderate |
High |
Less |
Moderate |
High |
Less |
Moderate |
High |
1 |
Pre-monsoon |
Channa striata |
0.06 ± 0.01 |
0.09 ± 0.01 |
0.12 ± 0.01 |
0.06 ± 0.01 |
0.07 ± 0.01 |
0.09 ± 0.01 |
0.06 ± 0.01 |
0.10 ± 0.01 |
0.22 ± 0.01 |
2 |
Channa punctata |
0.09 ± 0.01 |
0.13 ± 0.01 |
0.19 ± 0.01 |
0.08 ± 0.01 |
0.12 ± 0.01 |
0.19 ± 0.01 |
0.07 ± 0.01 |
0.15 ± 0.01 |
0.25 ± 0.01 |
3 |
Post-monsoon |
Channa striata |
0.05 ± 0.01 |
0.08 ± 0.01 |
0.11 ± 0.01 |
0.04 ± 0.01 |
0.06 ± 0.01 |
0.09 ± 0.01 |
0.04 ± 0.01 |
0.08 ± 0.01 |
0.17 ± 0.01 |
4 |
Channa punctata |
0.07 ± 0.01 |
0.08 ± 0.01 |
0.15 ± 0.01 |
0.05 ± 0.01 |
0.09 ± 0.01 |
0.12 ± 0.01 |
0.05 ± 0.01 |
0.07 ± 0.01 |
0.20 ± 0.01 |
3.6 Histopathology
Histopathological examination (Fig. 5) of polluted and non-polluted fish gills has been extensively used to study environmental stress as respiration and osmoregulation are the major functions of gills.71 In this study, the effect of bioaccumulated heavy metals on the gills, kidney, liver and muscle tissues of the fish from low, moderate and highly polluted sites from both the pre- and post-monsoon samples in C. striata and C. punctata were examined using histology. In accordance with earlier literature, the gills of the fish with high bioaccumulation suffered severe lamellar fusion, hyperplasia, hypertrophy and epithelial lifting, swelling and deformed lamella, in some parts sloughing off and curving of lamellae.72–74 Their liver hepatocytes and bile ducts showed necrosis of parenchyma, vacuolation, congestion of blood vessels, pyknosis and infiltration of leucocytes as observed earlier by Zheng et al., 2011 and Fatima and Usmani, 2013.75,76 Dilated kidney tubules and vacuolated glomeruli with no Bowman's space, degeneration of glomeruli and necrosis of hematopoietic tissue were observed as in previous reports made by Perera et al., 2016; Kaur and Dua 2014.77,78 Their muscles had normal myotomes with equally spaced muscle bundles and were similar to those observed by Madhusudan et al., 2003, and Senthilmurugan et al., 2008.79,80 The C. punctata showed more severe histopathological damage than the C. striata. Though, MN induction and histopathological damages could be caused by a variety of pollutants such as heavy metals, pesticides and a few other factors in the environment, few in vivo experimental studies have revealed the induction of MN and histopathological damage due to heavy metal exposure alone.58,76 Thus, the bioconcentration of metals in fish tissues is greatly influenced by most of the physical and chemical water quality parameters in the environment.7
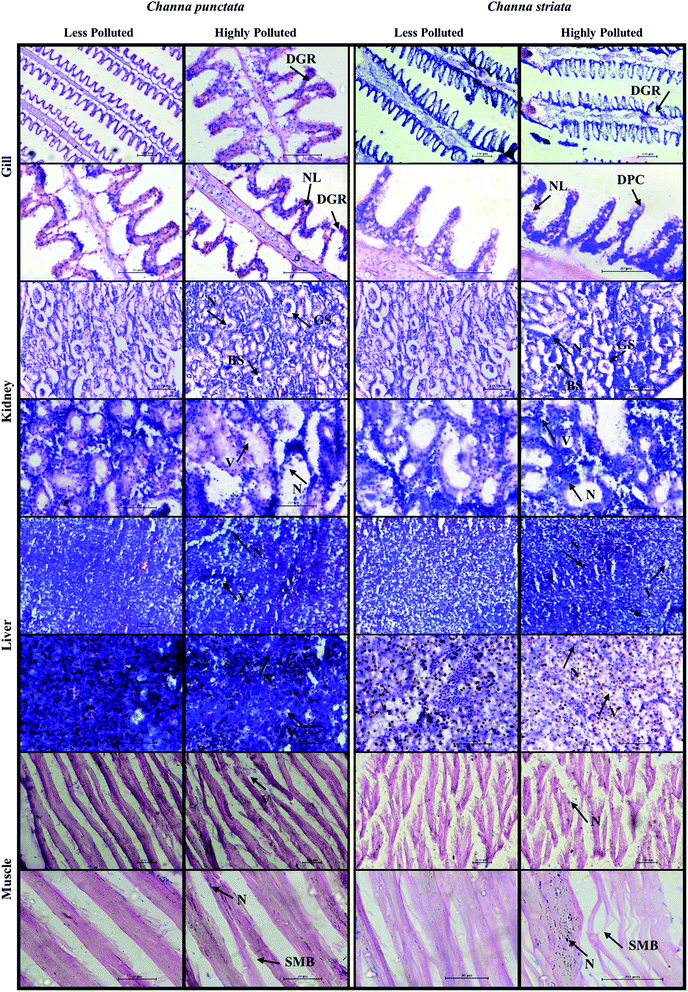 |
| Fig. 5 Representative histopathological images of various tissues of C. striata and C. punctata. [damaged pillar cells (DPC); damaged gill rackers (DGR); necrosis of lamellae (NL); dilation and vacuolation (V); glomerular shrinkage (GS); necrosis (N); glomeruli with Bowman's Space (BS); separation of muscle bundles (SMB)]. | |
The study thus revealed that the fish samples collected from heavily polluted sites with poor WQIs (C8, V5, and T8) showed a higher bioconcentration than the fish collected from less polluted sites (C2, V1 and T4). The medium polluted sites C5, V3 and T2 are to be taken into concern as they may very soon turn to highly polluted sites in the near future if care is not taken. To conclude, C. punctata was more bioconcentrated with heavy metals than C. striata; the pre-monsoon samples showed an increased level of heavy metal concentration compared to that of the post-monsoon samples indicating that the difference in water quality levels in the study sites is attributed to the effect of rainfall levels. The Cauvery was most polluted at sites C6 and C8, then the Vaigai (at V5 and V6) with HPI > 100, and the Thamirabarani was the least polluted among the three river streams studied, with no sites having an HPI > 100.
4. Conclusion
The bioaccumulation of heavy metals either in the water or in aquatic organisms elicits toxicity to humans by direct or indirect means. C. punctata had a greater bioaccumulation of heavy metals than C. striata. The present study focussed on the water quality and heavy metal bioaccumulation in murrel fish of the three major rivers in Southern Tamil Nadu, India based on water quality, heavy metal indices, bioaccumulation, bioconcentration, micronuclei and histological analysis. The water quality indices in most of the sampling sites were poor and very poor which necessitates immediate steps to be taken for improving the water quality. The cadmium level was high particularly in the Thamirabarani, which may be due to rocks withering and industrial pollution but must be paid immediate attention to in order to maintain the water quality. The increased heavy metal concentrations of chromium, lead and nickel in these rivers elicits prompt bioremediation and diminution of anthropogenic activities to protect the natural resources for future generations. This highlights the importance of following proper water management practises to reduce the seemingly deteriorating water quality. Therefore, the present study defines a warning alarm for the proper remediation steps to be taken to safeguard the natural water resources to sustain the ecosystem.
Conflicts of interest
There are no conflicts to declare.
Acknowledgements
The financial support of DST SERB YSP File No: SB/YS/LS-62/2013 and the Instrumentation Facility of SBS, CEGS, NRCBS – MKU, CAS is highly acknowledged. Dr R. S. Suja Rose, Department of Environmental Remote Sensing and Cartography (ERAC), School of Earth and Atmospheric Sciences is thanked for her kind help in cartography. The authors sincerely thank the valuable suggestions provided by Dr S. Chandrasekaran, Department of Plant Sciences, SBS, MKU.
References
- C. W. Commission, Integrated hydrological data book, New Delhi, 2012, pp. 1–680 Search PubMed.
- P. Kumarasamy, R. Arthur James, H.-U. Dahms, C.-W. Byeon and R. Ramesh, Environ. Earth Sci., 2014, 71, 2441–2451 CrossRef CAS.
- K. Raghavan and U. Ramesh, J. Res. Ecol., 2015, 2, 115–120 Search PubMed.
- M. Falkenmark and C. Widstrand, Popul. Bull., 1992, 47, 1–36 CAS.
- M. Vosylienė and A. Jankaitė, Ekologija, 2006, 12–17 Search PubMed.
- S. Dhanakumar, G. Solaraj and R. Mohanraj, Ecotoxicol. Environ. Saf., 2015, 113, 145–151 CrossRef CAS PubMed.
- B. Jezierska and M. Witeska, Soil and Water Pollution Monitoring, Protection and Remediation, NATO Science Series, 2006, pp. 107–114 Search PubMed.
- R. Udhayakumar, P. Manivannan, K. Raghu and S. Vaideki, Ecotoxicol. Environ. Saf., 2016, 134, 474–477 CrossRef CAS PubMed.
- R. Mdegela, J. Myburgh, D. Correia, M. Braathen, F. Ejobi, C. Botha, M. Sandvik and J. U. Skaare, Ecotoxicology, 2006, 15, 51–59 CrossRef CAS PubMed.
- A. K. Sinha, V. Kumar, H. P. S. Makkar, G. De Boeck and K. Becker, Food Chem., 2011, 127, 1409–1426 CrossRef CAS.
- R. J. Medeiros, L. M. G. dos Santos, A. S. Freire, R. E. Santelli, A. Braga, T. M. Krauss and S. D. Jacob, Food Contr., 2012, 23, 535–541 CrossRef CAS.
- J. Liu, X. Xu, S. Yu, H. Cheng, Y. Hong and X. Feng, Environ. Res., 2014, 131, 160–164 CrossRef CAS PubMed.
- P. S. Harikumar, U. P. Nasir and M. P. M. Rahman, Int. J. Environ. Sci. Technol., 2009, 6, 225–232 CrossRef CAS.
- U. Förstner and G. T. W. Wittmann, Folia Geobot. Phytotaxonomica, 1983, 18, 194 CrossRef.
- L. S. Clesceri, A. E. Greenberg and A. D. Eaton, Standard Methods for the Examination of water and wastewater, 20th edn, 1999 Search PubMed.
- R. K. Horton, J. Water Pollut. Control Fed., 1965, 37, 300–306 Search PubMed.
- R. M. Brown, N. I. McClelland, R. A. Deininger and M. F. O'Connor, Indic. Environ. Qual., 1972, 1, 173–178 Search PubMed.
- V. Sheykhi and F. Moore, Environ. Monit. Assess., 2013, 185, 3219–3232 CrossRef CAS PubMed.
- S. V. Mohan, P. Nithila and S. J. Reddy, J. Environ. Sci. Health, Part A: Environ. Sci. Eng. Toxic Hazard. Subst. Control, 1996, 31, 283–289 CrossRef.
- B. Prasad and K. Sangita, Mine Water Environ., 2008, 27, 265–267 CrossRef CAS.
- B. Prasad and K. K. Mondal, Mine Water Environ., 2008, 27, 40–45 CrossRef CAS.
- R. Chester and M. J. Hughes, Chem. Geol., 1967, 2, 249–262 CrossRef CAS.
- S. Lau, M. Mohamed, A. Tan Chi Yen and S. Su'ut, Sci. Total Environ., 1998, 214, 113–121 CrossRef CAS PubMed.
- USEPA, Risk-based concentration table. Region 3, US Environmental Protection Agency, Washington DC, 2011 Search PubMed.
- WHO. World Health Organization, Evaluations of the Joint FAO/WHO Expert Committee on Food Additives (JECFA), http://apps.who.int/food-additives-contaminants-jecfa-database/search.aspx?fcc=2.
- Y. G. Gu, Q. Lin, X.-H. Wang, F.-Y. Du, Z.-L. Yu and H.-H. Huang, Mar. Pollut. Bull., 2015, 96, 508–512 CrossRef CAS PubMed.
- FAO, Faostat: Food Balance Sheets, http://faostat.fao.org/beta/en/#data/FBS.
- S. C. Walpole, D. Prieto-Merino, P. Edwards, J. Cleland, G. Stevens and I. Roberts, BMC Publ. Health, 2012, 12, 439 CrossRef PubMed.
- N. S. Nagpure, R. Srivastava, R. Kumar, A. Dabas, B. Kushwaha and P. Kumar, Indian J. Exp. Biol., 2015, 53, 476–483 CAS.
- K. Al-Sabti and C. D. Metcalfe, Mutat. Res. Genet. Toxicol., 1995, 343, 121–135 CrossRef CAS.
- J. D. Bancroft and H. C. Cook, Manual of histological techniques and their diagnostic application, Churchill Livingstone, 1994, pp. 105–215 Search PubMed.
- BIS, Indian Standards Drinking Water Specifications IS 10500:2012, 2012.
- WHO, Guidelines for drinking-water quality: Fourth edition incorporating the first addendum, 2017 Search PubMed.
- G. Solairaj, S. Dhanakumar, K. Rutharvel Murthy and R. Mohanraj, Environ. Monit. Assess., 2010, 166, 435–444 CrossRef PubMed.
- A. Jameel and A. Z. Hussain, Indian J. Environ. Prot., 2005, 25, 941 Search PubMed.
- A. Jameel and A. Z. Hussain, Indian J. Environ. Prot., 2007, 27, 713 Search PubMed.
- A. Jameel and A. Z. Hussain, Environ. Monit. Assess., 2011, 183, 103–111 CrossRef PubMed.
- K. P. Singh, A. Malik and S. Sinha, Anal. Chim. Acta, 2005, 538, 355–374 CrossRef CAS.
- S. Tyagi, B. Sharma, P. Singh and R. Dobhal, Am. J. Water Resour., 2013, 1, 34–38 Search PubMed.
- B. Prasad and J. M. Bose, Environ. Geol., 2001, 41, 183–188 CrossRef CAS.
- S. Kalavathy, T. R. Sharma and P. Sureshkumar, Archives of Environmental Science, 2011, 55–61 Search PubMed.
- K. Mophin and A. G. Murugesan, Int. J. Environ. Protect., 2011, 1(10), 24–33 Search PubMed.
- S. Hema and T. Subramani, Asian J. Chem., 2013, 25, 3199–3203 CAS.
- J. García-Lestón, J. Méndez, E. Pásaro and B. Laffon, Environ. Int., 2010, 36, 623–636 CrossRef PubMed.
- A. Qadir and R. N. Malik, Biol. Trace Elem. Res., 2011, 143, 1524–1540 CrossRef CAS PubMed.
- F. Yılmaz, N. Özdemir, A. Demirak and A. L. Tuna, Food Chem., 2007, 100, 830–835 CrossRef.
- S. Zhao, C. Feng, W. Quan, X. Chen, J. Niu and Z. Shen, Mar. Pollut. Bull., 2012, 64, 1163–1171 CrossRef CAS PubMed.
- M. Canli and G. Atli, Environ. Pollut., 2003, 121, 129–136 CrossRef CAS PubMed.
- K. V. Dhaneesh, M. Gopi, R. Ganeshamurthy, T. T. A. Kumar and T. Balasubramanian, Food Chem., 2012, 131, 985–991 CrossRef CAS.
- J. W. Moore and S. Ramamoorthy, Appl. Monit. Impact Assess., 1984, 7779 Search PubMed.
- F. Kargin, Bull. Environ. Contam. Toxicol., 1998, 60, 822–828 CrossRef CAS PubMed.
- H. M. Avenant-Oldewage, Water SA, 2000, 26, 569–582 Search PubMed.
- A. H. A. Hilala and N. S. Ismailb, Jordan J. Biol. Sci., 2008, 1, 13–18 Search PubMed.
- F. Korkmaz Gorur, R. Keser, N. Akcay and S. Dizman, Chemosphere, 2012, 87, 356–361 CrossRef CAS PubMed.
- J. C. Amiard, C. Amiard-Triquet, S. Barka, J. Pellerin and P. S. Rainbow, Aquat. Toxicol., 2006, 76, 160–202 CrossRef CAS PubMed.
- M. Dural, M. Z. L. Göksu and A. A. Özak, Food Chem., 2007, 102, 415–421 CrossRef CAS.
- R. Eisler, Compendium of Trace Metals and Marine Biota, 2010, vol. 2, pp. 39–220 Search PubMed.
- M. Javed and N. Usmani, Int. J. Environ. Sci., 2011, 2, 659 CAS.
- B. R. Kiran, T. R. Shashi Shekhar, E. T. Puttaiah and Y. Shivaraj, J. Environ. Sci. Eng., 2006, 48, 15–20 CAS.
- K. J. Elnabris, S. K. Muzyed and N. M. El-Ashgar, J. Assoc. Arab Univ. Basic Appl. Sci., 2013, 13, 44–51 Search PubMed.
- H. Y. Çoǧun, T. A. Yüzereroǧlu, Ö. Firat, G. Gök and F. Kargin, Environ. Monit. Assess., 2006, 121, 429–436 CrossRef PubMed.
- K. M. El-Moselhy, A. I. Othman, H. Abd El-Azem and M. E. A. El-Metwally, Egyptian journal of Basic and Applied Sciences, 2014, 1, 97–105 CrossRef.
- Y. H. Wei, J. Y. Zhang, D. W. Zhang, T. H. Tu and L. G. Luo, Ecotoxicol. Environ. Saf., 2014, 104, 182–188 CrossRef CAS PubMed.
- D. Lemly, Environ. Monit. Assess., 1993, 28, 83–100 CrossRef PubMed.
- D. Lemly, Aquat. Toxicol., 1993, 27, 133–158 CrossRef.
- W. J. Sydeman and W. M. Jarman, Mar. Pollut. Bull., 1998, 36, 828–832 CrossRef CAS.
- J. Burger, K. F. Gaines, C. S. Boring, W. L. Stephens, J. Snodgrass, C. Dixon, M. McMahon, S. Shukla, T. Shukla and M. Gochfeld, Environ. Res., 2002, 89, 85–97 CrossRef CAS PubMed.
- V. V. Arkhipchuk and N. N. Garanko, Ecotoxicol. Environ. Saf., 2005, 62, 42–52 CrossRef CAS PubMed.
- L. M. Zapata, B. C. Bock, L. Y. Orozco and J. A. Palacio, Ecotoxicol. Environ. Saf., 2016, 127, 108–116 CrossRef CAS PubMed.
- J. d. S. Azevedo, E. d. S. Braga and C. A. O. Ribeiro, Braz. J. Oceanogr., 2012, 60, 323–330 CrossRef.
- J. Mersch, M. N. Beauvais and P. Nagel, Mutat. Res. Genet. Toxicol., 1996, 371, 47–55 CrossRef CAS.
- E. I. Akpakpan, E. O. Akpanyung, N. O. Anazodo and U. M. Ekanemesang, World Appl. Sci. J., 2014, 32, 1209–1218 Search PubMed.
- M. Javed, N. Usmani, I. Ahmad and M. Ahmad, Environ. Monit. Assess., 2015, 187, 1–11 CrossRef CAS PubMed.
- M. Javed, I. Ahmad, N. Usmani and M. Ahmad, Chemosphere, 2016, 151, 210–219 CrossRef CAS PubMed.
- J. L. Zheng, Z. Luo, Q. L. Chen, X. Liu, C. X. Liu, Y. H. Zhao and Y. Gong, Ecotoxicol. Environ. Saf., 2011, 74, 1864–1873 CrossRef CAS PubMed.
- M. Fatima and N. Usmani, Pakistan J. Biol. Sci., 2013, 16, 412–420 CrossRef CAS.
- P. C. T. Perera, T. V. Sundarabarathy, T. Sivananthawerl, S. P. Kodithuwakku and U. Edirisinghe, Achievements in the Life Sciences, 2016, 10, 144–160 CrossRef.
- R. Kaur and A. Dua, J. Environ. Biol., 2014, 35, 265–272 Search PubMed.
- S. Madhusudan, F. LiyaQuat and C. Nadim, Indian J. Fish., 2003, 50, 53–65 Search PubMed.
- S. Senthil Murugan, R. Karuppasamy, K. Poongodi and S. Puvaneswari, Turk. J. Fish. Aquat. Sci., 2008, 55–59 Search PubMed.
Footnote |
† Electronic supplementary information (ESI) available. See DOI: 10.1039/c7ra13583a |
|
This journal is © The Royal Society of Chemistry 2018 |
Click here to see how this site uses Cookies. View our privacy policy here.