DOI:
10.1039/C8RA00101D
(Paper)
RSC Adv., 2018,
8, 9017-9022
Synthesis and characterisation of Ba(Zn1−xCox)2Si2O7 (0 ≤ x ≤ 0.50) for blue-violet inorganic pigments
Received
5th January 2018
, Accepted 23rd February 2018
First published on 27th February 2018
Abstract
Ba(Zn1−xCox)2Si2O7 (0 ≤ x ≤ 0.50) solid solutions were synthesized as novel blue-violet inorganic pigments by a conventional solid-state reaction method. The crystal structure, optical properties, and colour of the pigments were characterized. All the pigments were obtained in a single-phase form. The pigments strongly absorbed visible light at wavelengths from 550 to 650 nm, corresponding to the range of green to orange light. This optical absorption was caused by the d–d transition of the tetrahedrally coordinated Co2+ (4A2(F) → 4T1(P)), which was the origin of the blue-violet colour of the pigments. The most intense colour was obtained for Ba(Zn0.85Co0.15)2Si2O7, where a* = +52.2 and b* = −65.5 in the CIE (Commission Internationale de l'Éclairage) L*a*b* system. These absolute values were significantly larger than those of commercial violet pigments such as Co3(PO4)2 (a* = +33 and b* = −32) and NH4MnP2O7 (a* = +39 and b* = −21). Therefore, the Ba(Zn0.85Co0.15)2Si2O7 pigment could be a novel blue-violet inorganic pigment.
Introduction
Cobalt (Co) and its compounds have been applied in alloys, paints, catalysts, cements and ceramic pigments.1–5 In particular, the Co2+ ion is often employed as a colouring source for blue and violet pigments. There are a number of reports on blue pigments using Co2+ ions, such as Co2SiO4 olivine,6 (Co, Zn)2SiO4 willemite,7 CoAl2O4 spinel8 and Co2SnO4.9 The colouring performance of these pigments mainly depends on the coordination number around the Co2+ ion, which is very important for the appearance of the bluish colour. However, the use of a large amount of Co increases the cost of the material, which becomes expensive because of its rarity.
Accordingly, selection of an appropriate host lattice, minimization of the Co content and strong colouring performance are necessary, when Co is applied to colour pigments. Although many studies on the reduction of the amount of Co in the pigments have been reported,10–14 almost of them are on the blue pigments and there are only a few reports on the violet pigments.13,15,16
Generally, the colour of inorganic pigments is mainly affected by the crystal field, generated by the ions surrounding the chromophore.17 Cobalt violet (Co3(PO4)2) and manganese violet (NH4MnP2O7) has been well known as current commercial violet pigments. The structure of Co3(PO4)2 is formed by distorted trigonal bipyramids CoO5, fairly regular CoO6 octahedra and almost regular PO4 tetrahedra.13 Generally, cobalt ion can take various coordination numbers and represent various colours in phosphates, In Co3(PO4)2·8H2O, Co2+ has octahedral 6 coordination to show reddish violet colour, while in KCoPO4 it has tetrahedral 4 coordination to evince blue-violet colour. On the other hand, NH4MnP2O7 presents violet colour due to the d–d transition of the octahedral coordinated Mn3+.18 However, the vividness of the Co3(PO4)2 and the NH4MnP2O7 pigments is insufficient, that is, their absolute values of a* and b* in the CIE L*a*b* system are not so large. In this system, the parameter L* indicate the brightness or darkness of a colour on relation to a neutral grey scale, while the parameters a* (the red-green axis) and b* (the yellow-blue axis) express the colour qualitatively. In addition, the thermal resistance of NH4MnP2O7 is not enough, because it is decomposed around 340 °C.18 Some violet pigments without Co have been proposed recently,19–21 but their colours are not much different from those of the existing commercial violet pigments. Organic pigments are inferior to inorganic pigments in heat resistance and weather resistance. Thus, it is significant to synthesize a novel violet inorganic pigment in which the colour property is improved.
Because of this situation, we focused on barium zinc silicate BaZn2Si2O7 as a host lattice of the novel violet pigment. This compound has a layered structure composed of [ZnO4]2− tetrahedra connected at each corner to [SiO4] tetrahedra. Each [SiO4] tetrahedron is connected over three corners to one [SiO4] and two [ZnO4]2− tetrahedra, and the forth corner is a non-bridging oxygen atom. The Ba2+ ions are located in between the zinc silicate layers.22,23 As a related compound, Ba(M, Ni)2Si2O7 (M = Zn or Mg) pigments have been ever reported, but they exhibit red and purplish red colours due to the d–d transition of the tetrahedral coordinated Ni2+.24 In this study, Ba(Zn1−xCox)2Si2O7 (0 ≤ x ≤ 0.50) pigments were synthesized by a conventional solid-state reaction method, and the colour properties of the pigments were investigated as novel blue-violet inorganic pigments.
Experimental
Materials and methods
The Ba(Zn1−xCox)2Si2O7 (0 ≤ x ≤ 0.50) pigments were synthesized by a conventional solid-state reaction method. BaCO3 (Kishida Chemical, Japan), ZnO (Kishida Chemical, Japan), SiO2 (Wako Pure Chemical, Japan) and Co3O4 (Wako Pure Chemical, Japan) were used as starting materials. The raw materials were mixed in a stoichiometric amount in an agate mortar. The mixture was calcined in an alumina boat at 1250 °C for 6 h. The samples were ground in an agate mortar before characterisation.
Characterisation
The composition of the samples was confirmed by X-ray fluorescence spectroscopy (XRF; Rigaku, ZSX Primus). The crystal structures of the samples were identified by X-ray powder diffraction (XRD; Rigaku, Ultima IV) with Cu-Kα radiation (40 kV, 40 mA). The sampling width and the scan speed were 0.02° and 6.0 min−1, respectively. The lattice parameters and volumes were calculated from the XRD peak angles, which were refined using α-Al2O3 as a standard and using the CellCalc Ver. 2.20 software. The size and morphology of the Ba(Zn0.85Co0.15)2Si2O7 particles were observed by using scanning electron microscopy (SEM; JEOL, JSM-6701F). Gold was sputtered before observation to avoid the charge-up of the samples. The purity of the samples was analysed by energy dispersive X-ray analysis (EDX; Oxford Instruments, INCA Energy). An X-ray photoelectron spectrum (XPS; ULVAC-PHI, PHI5000 VersaProve II) of the Ba(Zn0.85Co0.15)2Si2O7 pigment was measured using Mg-Kα radiation to investigate the oxidation state of the Co ion.
The optical reflectance of the Ba(Zn1−xCox)2Si2O7 (0 ≤ x ≤ 0.50) samples were measured using a UV-Vis spectrometer (Shimadzu, UV-2550) with barium sulphate as a reference. The colour properties of the samples were estimated in terms of the CIE L*a*b*Ch° system using a calorimeter (Konica-Minolta, CR-300). This colour measurement was made for powder samples. In the case of the blue-violet pigment, positive a* and negative b* values are desirable. Chroma parameter (C) represents the colour saturation of the pigments and is calculated according to the following formula: C = [(a*)2 + (b*)2]1/2. The parameter h° ranges from 0 to 360° (300 ≤ h° ≤ 330 means blue-violet), and is calculated with the formula, h° = tan−1(b*/a*).
Results and discussion
X-ray fluorescence analysis (XRF)
The XRF analysis data of the samples were listed in Table 1. All compositions were almost in good agreement with those of the nominal stoichiometric ones.
Table 1 The compositions of the Ba(Zn1−xCox)2Si2O7 (0 ≤ x ≤ 0.50) pigments
Stoichiometric composition |
Analysed composition |
BaZn2Si2O7 |
Ba0.99Zn1.99Si2.02O7.02 |
Ba(Zn0.95Co0.05)2Si2O7 |
Ba0.95(Zn0.92Co0.04)2Si2.14O7.15 |
Ba(Zn0.90Co0.10)2Si2O7 |
Ba1.04(Zn0.87Co0.11)2Si2.00O7.00 |
Ba(Zn0.85Co0.15)2Si2O7 |
Ba1.05(Zn0.84Co0.15)2Si1.96O6.96 |
Ba(Zn0.80Co0.20)2Si2O7 |
Ba0.94(Zn0.81Co0.22)2Si2.00O7.00 |
Ba(Zn0.75Co0.25)2Si2O7 |
Ba1.00(Zn0.76Co0.24)2Si2.00O7.00 |
Ba(Zn0.70Co0.30)2Si2O7 |
Ba1.05(Zn0.71Co0.29)2Si2.14O7.14 |
Ba(Zn0.65Co0.35)2Si2O7 |
Ba0.97(Zn0.63Co0.34)2Si2.10O7.10 |
Ba(Zn0.60Co0.40)2Si2O7 |
Ba0.95(Zn0.58Co0.37)2Si2.15O7.15 |
Ba(Zn0.55Co0.45)2Si2O7 |
Ba1.03(Zn0.54Co0.42)2Si2.05O7.05 |
Ba(Zn0.50Co0.50)2Si2O7 |
Ba1.00(Zn0.51Co0.47)2Si2.05O7.05 |
X-ray powder diffraction (XRD)
Fig. 1 shows the XRD patterns of the Ba(Zn1−xCox)2Si2O7 (0 ≤ x ≤ 0.50) pigments. All samples were obtained in a single-phase form and the diffraction patterns were well indexed to that of the monoclinic BaZn2Si2O7 structure whose space group was C2/c.22,23,25 This structure is different from that of orthorhombic BaCu2Si2O7 (ref. 26) having a similar composition. The diffraction peaks about 54° shifted to higher angles with increasing the Co2+ content. The lattice volumes of the Ba(Zn1−xCox)2Si2O7 (0 ≤ x ≤ 0.50) samples calculated from the diffraction peaks are summarized in Table 2. The volume decreased with increasing the Co2+ concentration, indicating that the Zn2+ (ionic radius: 0.060 nm)27 ions were partially substituted with the smaller Co2+ (ionic radius: 0.058 nm)27 ions and the solid solutions based on monoclinic BaZn2Si2O7 were successfully synthesized in a single-phase form.
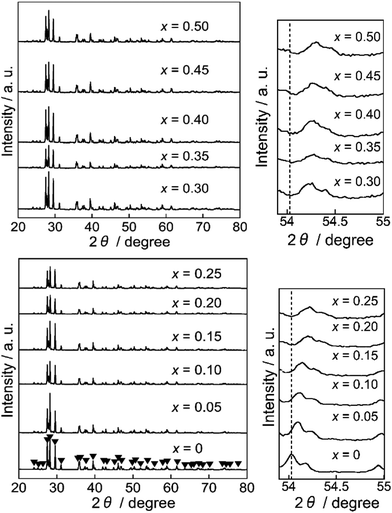 |
| Fig. 1 XRD patterns of the Ba(Zn1−xCox)2Si2O7 (0 ≤ x ≤ 0.50) pigments. | |
Table 2 Lattice volumes of the Ba(Zn1−xCox)2Si2O7 (0 ≤ x ≤ 0.50) pigments
x |
Lattice volume/nm3 |
0 |
1.27603 |
0.05 |
1.27570 |
0.10 |
1.27559 |
0.15 |
1.27555 |
0.20 |
1.27497 |
0.25 |
1.27463 |
0.30 |
1.27449 |
0.35 |
1.27383 |
0.40 |
1.27370 |
0.45 |
1.27356 |
0.50 |
1.27330 |
Scanning electron microscopic (SEM) image and energy dispersive X-ray (EDX) analysis
Fig. 2 depicts the SEM image and particle distribution of the Ba(Zn0.85Co0.15)2Si2O7 pigment. The average particle size calculated from 400 particles was about 13 μm. The EDX analysis result for the Ba(Zn0.85Co0.15)2Si2O7 sample is shown in Fig. 3. It was confirmed that Ba, Zn, Si, Co and O were present and non-impurities were observed without Au (charge-up preventer). The X-ray dot mapping analysis results is depicted in Fig. 4, indicating that the component elements were uniformly distributed in the particle.
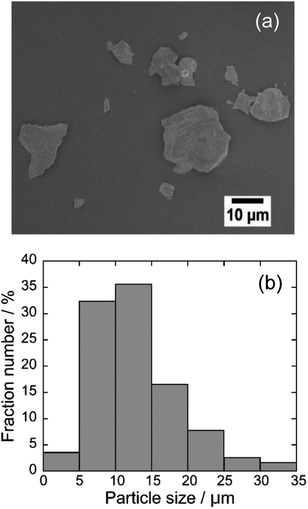 |
| Fig. 2 SEM image (a) and particle distribution (b) of the Ba(Zn0.85Co0.15)2Si2O7 pigment. | |
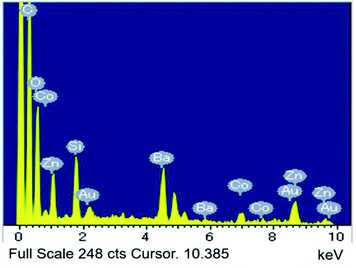 |
| Fig. 3 The EDS analysis for Ba(Zn0.85Co0.15)2Si2O7. | |
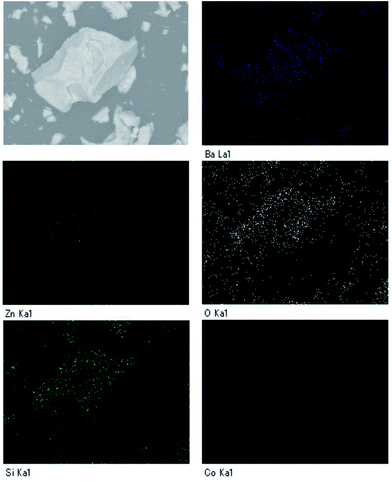 |
| Fig. 4 The X-ray dot mapping analysis of the Ba(Zn0.85Co0.15)2Si2O7. | |
X-ray photoelectron spectrum (XPS)
The XPS of the Ba(Zn0.85Co0.15)2Si2O7 pigment is shown in Fig. 5. This spectrum was deconvoluted into three components, considering the spin–orbit doublets. The intense peaks at 795.3 eV and 780.2 eV were attributed to the Ba2+ 3d3/2 and 3d5/2 configurations, respectively.28,29 Although the small peaks observed at 793.7 eV and 778.6 eV were assigned to the Co3+ 2p3/2 and 2p1/2 lines, more intense peaks were also detected at 796.3 eV and 781.0 eV, corresponding to those of Co2+.29–31 These results indicate that the dominant oxidation state of cobalt ions was divalent on the surface of the Ba(Zn0.85Co0.15)2Si2O7 pigment. Furthermore, the d–d transition of the tetrahedral coordinated Co3+ ions was appeared around 9000 cm−1 (1111 nm).32 Therefore, the Co3+ ions do not affect the colour of the present Ba(Zn1−xCox)2Si2O7 (0.05 ≤ x ≤ 0.50) pigments.
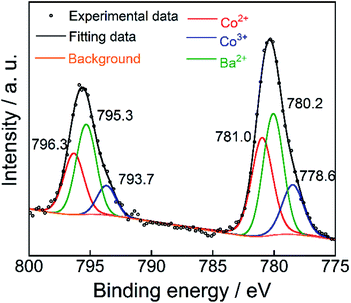 |
| Fig. 5 XPS of Co 2p and Ba 3d on the surface of the Ba(Zn0.85Co0.15)2Si2O7 pigment. | |
Reflectance spectra
Fig. 6 depicts the UV-Vis diffuse reflectance spectra for the Ba(Zn1−xCox)2Si2O7 (0 ≤ x ≤ 0.50) pigments. High reflectance was observed in the visible light region for the Co2+-free BaZn2Si2O7 (x = 0) sample. On the other hand, strong absorption bands originated by the d–d transition of tetrahedral coordinated Co2+ (ref. 8, 33 and 34) were observed in the Ba(Zn1−xCox)2Si2O7 (0.05 ≤ x ≤ 0.50) pigments from 550 to 650 nm corresponding to the green-orange lights. The Co2+ ion has the d7 electron configuration and the energy level structure of the Co2+ ion in a tetrahedral site is similar to that of d3 ion in an octahedral site.35 According to the Tanabe–Sugano diagram, the bands from 550 to 650 nm are assigned to the 4A2(F) → 4T1(P) transition of the tetrahedral coordinated Co2+.33–36
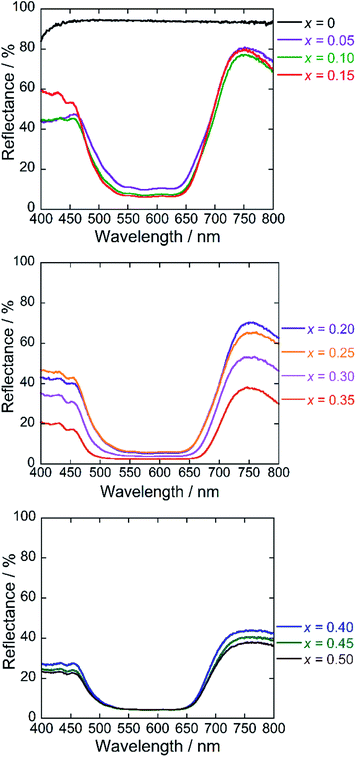 |
| Fig. 6 UV-Vis reflectance spectra of the Ba(Zn1−xCox)2Si2O7 (0 ≤ x ≤ 0.50) pigments. | |
Chromatic properties
The L*a*b*Ch° colour coordinate data for the Ba(Zn1−xCox)2Si2O7 (0 ≤ x ≤ 0.50) pigments are summarized in Table 3. They were compared using powder samples. It is obvious that the a* and b* values became significantly positive and negative, respectively, by the introduction of Co2+ in the host BaZn2Si2O7 lattice. As mentioned in the previous section, the Ba(Zn1−xCox)2Si2O7 pigments absorbed the green-orange lights but reflected the complementary blue and red lights. This is the reason for that positive a* and negative b* values were obtained in these pigments. The photographs of the Ba(Zn1−xCox)2Si2O7 (0 ≤ x ≤ 0.50) samples are shown in Fig. 7. The colour of the Ba(Zn1−xCox)2Si2O7 (0 ≤ x ≤ 0.50) pigments gradually changed from white to dark blue-violet as the Co2+ concentration increased. Among the samples synthesized in this study, the largest absolute values in the colour coordinate data were obtained for Ba(Zn0.85Co0.15)2Si2O7 (a* = +52.2 and b* = −65.5).
Table 3 The L*a*b*Ch° colour coordinates of the Ba(Zn1−xCox)2Si2O7 (0 ≤ x ≤ 0.50) pigments
x |
L* |
a* |
b* |
C |
h° |
0 |
93.7 |
−0.01 |
+0.20 |
0.20 |
92.9 |
0.05 |
43.8 |
+27.9 |
−46.6 |
54.3 |
300.9 |
0.10 |
30.1 |
+39.8 |
−54.8 |
67.7 |
306.0 |
0.15 |
28.6 |
+52.2 |
−65.5 |
83.8 |
308.6 |
0.20 |
25.9 |
+44.9 |
−57.8 |
76.4 |
307.8 |
0.25 |
21.8 |
+49.9 |
−59.8 |
77.9 |
309.8 |
0.30 |
25.0 |
+33.3 |
−45.4 |
56.3 |
306.3 |
0.35 |
22.8 |
+27.4 |
−38.0 |
46.8 |
305.8 |
0.40 |
21.0 |
+35.9 |
−45.9 |
58.3 |
308.0 |
0.45 |
20.2 |
+33.4 |
−43.3 |
54.6 |
307.6 |
0.50 |
20.8 |
+30.4 |
−40.5 |
50.6 |
306.9 |
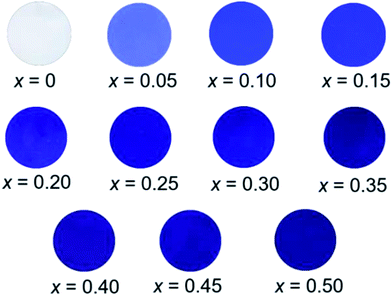 |
| Fig. 7 Photographs of the Ba(Zn1−xCox)2Si2O7 (0 ≤ x ≤ 0.50) pigments. | |
They were compared with those of the commercially available Co3(PO4)2 and NH4MnP2O7 pigments in Table 4. It is notable that the absolute values of a* and b* for the Ba(Zn0.85Co0.15)2Si2O7 were significantly larger than those for the commercial violet pigments.
Table 4 The L*a*b*Ch° colour coordinates of the Ba(Zn0.85Co0.15)2Si2O7 pigment and commercial violet pigments
Samples |
L* |
a* |
b* |
C |
h° |
Cited from ref. 20. |
Ba(Zn0.85Co0.15)2Si2O7 |
28.6 |
+52.2 |
−65.5 |
83.8 |
308.6 |
Co3(PO4)2a |
46 |
+33 |
−32 |
44.3 |
315.9 |
NH4MnP2O7a |
31 |
+39 |
−21 |
46.0 |
331.7 |
Chemical stability tests
The chemical stability of the Ba(Zn0.85Co0.15)2Si2O7 pigment was also evaluated using a powder sample. The pigment was soaked into 4% acetic acid and 4% ammonium bicarbonate. After leaving them at room temperature for 2 h, the pigments were washed with deionized water and ethanol, and then dried at room temperature. The colour of the pigment after the leaching test was evaluated using the calorimeter. As seen in Table 5, the colour of the present Ba(Zn0.85Co0.15)2Si2O7 pigment was almost unchanged.
Table 5 The L*a*b*Ch° colour coordinates of the Ba(Zn0.85Co0.15)2Si2O7 pigment before and after the acid and base resistance tests
Pigment |
L* |
a* |
b* |
C |
h° |
Non-treatment |
28.6 |
+52.2 |
−65.5 |
83.8 |
308.6 |
4% CH3COOH |
28.0 |
+57.0 |
−69.4 |
89.8 |
309.4 |
4% NH4HCO3 |
25.7 |
+56.2 |
−67.8 |
88.1 |
309.7 |
Conclusions
Ba(Zn1−xCox)2Si2O7 (0 ≤ x ≤ 0.50) solid solutions were successfully synthesized as novel blue-violet inorganic pigments. The samples strongly absorbed the visible light from 550 to 650 nm (green to orange), which was originated by the d–d transition of tetrahedrally coordinated Co2+. Ba(Zn0.85Co0.15)2Si2O7 showed the most intense colour among the samples, and the L*a*b*Ch° parameters were L* = 28.6, a* = +52.2, b* = −65.5, C = 66.3, and h° = 308.6. The absolute values of a* and b* of Ba(Zn0.85Co0.15)2Si2O7 were significantly larger than those of the commercial Co3(PO4)2 (a* = +33 and b* = −32) and NH4MnP2O7 (a* = +39 and b* = −21) pigments. Furthermore, the Ba(Zn0.85Co0.15)2Si2O7 pigment has excellent chemical resistance and thermal stability. These results indicate that Ba(Zn0.85Co0.15)2Si2O7 could serve as an effective alternative to the conventional blue-violet inorganic pigments.
Conflicts of interest
There are no conflicts to declare.
Acknowledgements
This work was partially supported by JSPS KAKENHI Grant Number 15K05643. The authors thank Dr Hirokazu Izumi (Hyogo Prefectural Institute of Technology) for his assistance with the X-ray photoelectron spectroscopy measurement.
References
- N. V. Russell, F. Wigley and J. Williamson, J. Mater. Sci., 2000, 35, 2131 CrossRef CAS.
- F. Miccichè, E. Oostveen, J. Van Haveren and R. Van Der Linde, Prog. Org. Coat., 2005, 53, 99 CrossRef.
- D. Uner, M. K. Demirkol and B. Dernaika, Appl. Catal., B, 2005, 61, 334 CrossRef CAS.
- M. Frías and M. I. S. de Rojas, Cem. Concr. Res., 2002, 32, 435 CrossRef.
- W. S. Cho and M. Kakihana, J. Alloys Compd., 1999, 287, 87 CrossRef CAS.
- K. Ullrich, O. Ott, K. Langer and K. D. Becker, Phys. Chem. Miner., 2004, 31, 247 CrossRef CAS.
- L. C. K. De Souza, J. R. Zamian, R. G. N. da Filho, L. E. B. Soledade, I. M. G. Dos Santos, A. G. Souza, T. Scheller, R. S. Angélica and C. E. F. Da Costa, Dyes Pigm., 2009, 81, 187 CrossRef CAS.
- J. Merikhi, H. O. Jungk and C. Feldmann, J. Mater. Chem., 2000, 10, 1311 RSC.
- A. Shamirian, M. Edrisi and M. Naderi, J. Mater. Eng. Perform., 2013, 22, 306 CrossRef CAS.
- S. Erič, L. Kostic-Gvozdenvić, M. Mirandinović and L. Pavlovič, Ceram.-Silik., 1990, 34, 61 Search PubMed.
- M. Llusar, A. Forés, J. A. Badenes, J. Calbo, M. A. Tena and G. Monrós, J. Eur. Ceram. Soc., 2001, 21, 1121 CrossRef CAS.
- M. Llusar, A. Zielinska, M. A. Tena, J. A. Badenes and G. Monrós, J. Eur. Ceram. Soc., 2010, 30, 1887 CrossRef CAS.
- S. Meseguer, M. A. Tena, C. Gargor, J. A. Badenes, M. Llusar and G. Monrós, Ceram. Interfaces, 2007, 33, 843 CrossRef CAS.
- I. S. Ahmed, S. A. Shama, M. M. Moustafa, H. A. Dessouki and A. A. Ali, Spectrochim. Acta, Part A, 2009, 74, 665 CrossRef CAS PubMed.
- L. Robertson, M. Duttine, M. Gaudon and A. Demourgues, Chem. Mater., 2011, 23, 2419 CrossRef CAS.
- N. Gorodylova, V. Kosinová, Ž. Dohnalová, P. Bělina and P. Šulcová, Dyes Pigm., 2013, 98, 393 CrossRef CAS.
- D. Gryffroy, B. E. Vandenberghe and D. Poelman, Solid State Commun., 1992, 82, 497 CrossRef CAS.
- Y. Begum and A. J. Wright, J. Mater. Chem., 2010, 22, 21110 RSC.
- A. E. Smith, H. Mizoguchi, K. Delaney, N. A. Spaldin, A. W. Sleight and M. A. Subramanian, J. Am. Ceram. Soc., 2009, 131, 17084 CAS.
- S. W. Kim, Y. Saito, T. Hasegawa, K. Toda, K. Uematsu and M. Sato, Dyes Pigm., 2017, 136, 243 CrossRef CAS.
- Y. Chen, Y. Zhang and S. Feng, Dyes Pigm., 2014, 105, 167 CrossRef CAS.
- M. Kerstan, M. Müller and C. Rüssel, J. Solid State Chem., 2012, 188, 84 CrossRef CAS.
- J. H. Lin, G. X. Lu, J. Du, M. Z. Su, C. K. Loong and J. W. Richardson Jr, J. Phys. Chem. Solids, 1999, 60, 975 CrossRef CAS.
- C. Thieme and C. Rüssel, Dyes Pigm., 2014, 111, 75 CrossRef CAS.
- M. Krestan, C. Thieme, M. Grosch, M. Müller and C. Rüssel, J. Solid State Chem., 2013, 207, 55 CrossRef.
- J. Janczak and R. Kubiak, Acta Crystallogr., Sect. C: Struct. Chem., 1990, 46, 1383 CrossRef.
- R. D. Shannon, Acta Crystallogr., Sect. A: Cryst. Phys., Diffr., Theor. Gen. Crystallogr., 1976, 32, 751 CrossRef.
- L. Wang, J. Zhang, Q. Zhang, N. Xu and J. Song, J. Magn. Magn. Mater., 2015, 377, 362 CrossRef CAS.
- B. Liu, Y. Zhang and L. Tang, Int. J. Hydrogen Energy, 2009, 34, 435 CrossRef CAS.
- J. Šponer, J. Čejka, J. Dědeček and B. Wichterlová, Microporous Mesoporous Mater., 2000, 37, 117 CrossRef.
- J. F. Marco, J. R. Gancedo, M. Gracia, J. L. Gautier, E. I. Ríos, H. M. Palmer, C. Greaves and F. J. Berry, J. Mater. Chem., 2001, 11, 3087 RSC.
- D. L. Wood and J. P. Remeika, J. Chem. Phys., 1967, 46, 3595 CrossRef CAS.
- S. Meseguer, M. A. Tena, C. Gargori, R. Galindo, J. A. Badenes, M. Llusar and G. Monrós, Ceram. Interfaces, 2008, 34, 1431 CrossRef CAS.
- N. V. Kuleshov, V. P. Mikhailov, V. G. Scherbitsky, P. V. Prokoshin and K. V. Yumashev, J. Lumin., 1993, 55, 265 CrossRef CAS.
- A. K. Zvezdin and V. A. Kotov, Modern Magnetooptics and Magnetooptical Materials, CRC Press, 1993 Search PubMed.
- M. Dondi, C. Zanelli, M. Ardit and G. Cruciani, J. Am. Ceram. Soc., 2011, 94, 1025 CrossRef CAS.
|
This journal is © The Royal Society of Chemistry 2018 |
Click here to see how this site uses Cookies. View our privacy policy here.