DOI:
10.1039/C8RA01972J
(Paper)
RSC Adv., 2018,
8, 19539-19550
4-Hydroxybenzyl alcohol derivatives and their sedative–hypnotic activities
Received
6th March 2018
, Accepted 4th May 2018
First published on 29th May 2018
Abstract
4-Hydroxybenzyl alcohol (HBA), one of the characteristic active components of Gastrodia elata, exhibits obvious effects on the human central nervous system. In order to acquire compounds with superior bioactivity, 10 derivatives of HBA were synthesized from HBA and carboxylic acids. The sedative effects of the 10 HBA derivatives were evaluated using a spontaneous locomotor activity test (SLT) in mice, and their hypnotic effects were determined to be synergistic with pentobarbital-induced sleep. The results showed that 4-hydroxybenzyl alcohol 3-furancarboxylic acid diester (2FHBA, 10 mg kg−1) exhibited the strongest sedative–hypnotic activity among HBA and its derivatives, and 2FHBA could reverse the insomnia caused by p-chlorophenylalanine (pCPA), flumazenil (FLU) and thiosemicarbazide (TSC). Meanwhile, 2FHBA and 5-hydroxytryptophan (5-HTP) showed a synergistic effect. The results suggested that 2FHBA might be a potential agent against insomnia, which might be mediated by the serotonergic and GABAergic systems.
1. Introduction
Today, almost half of human beings suffer from sleeping disturbance all over the world, not only old people but also young people, due to the excessive stress in their lives.1 Difficulty getting to sleep, easily waking up and poor sleep quality are all symptoms associated with insomnia, which appears to be very prevalent and seriously threatens human health. If there is no effective control, insomnia will develop into a major depressive disorder. Therefore, it is necessary to search for potent medicines with effective sedative and hypnotic activities. Natural products have become an important source of bioactive lead compounds for drug discovery, especially their derivatives through synthetic transformation or structural modification, which has occupied a leading position recently.2
Gastrodia elata (G. elata), recorded as the stem tubers of G. elata Blume in Chinese pharmacopoeia, has been found to possess a wide range of biological activities, including sedative and hypnotic,3,4 antiepileptic and anticonvulsive,5,6 anti-anxiety and antidepressant,7,8 neuroprotective,9 anti-cardio-cerebral-vascular disease,10 antipsychotic,11 anti-vertigo,12 anticoagulant and antithrombotic,13 anti-atherosclerotic,14 antihypertensive,15 anti-inflammatory and analgesic,16,17 memory improving and anti-aging,18 antivirus19 and antitumor20 activities and so on. 4-Hydroxybenzyl alcohol (HBA), also named as gastrodigenin, is the characteristic and main active component of G. elata. Gastrodin (GAS) is another chief active ingredient of G. elata, and studies have also reported that GAS is metabolized to HBA in the body and then plays pharmacological roles.21 Meanwhile, HBA was chosen as one of the standard compounds to evaluate the quality of G. elata.22 Modern pharmacological experiments have demonstrated that HBA apparently possesses the ability to adjust the central nervous system, including sedation,23 anti-convulsion,23 anti-depressant,24 and anti-neuroinflammation activities,25 facilitating memory and neuroprotection,26,27 and it is often used as a precursor compound through structural modification to discover more active compounds. It has been reported that the derivatives of HBA substituted with amino acids, phosphate and sulfonate display excellent hepatoprotective, anti-cancer and inhibition of rat testicular P-45017α activities, respectively.28–30
Carboxylic acids are usually selected to be synthesized with compounds with hydroxyl groups to obtain better biological activity esters. Some bioactive compounds have been synthesized by our team in recent years, like ergosteryl 2-naphthoate31 and arctigenin β-indolylacetate,32 which showed excellent anti-tumor effects. The aim of this study was to find new compounds with potentially sedative and hypnotic activities by synthesizing derivatives of HBA with carboxylic acids. Behavioral pharmacological tests, including the spontaneous locomotor activity test and the pentobarbital-induced sleep test, were employed to evaluate their biological activities. Furthermore, the possible mechanism was also devised.
2. Materials and method
2.1. Animals
Mice weighing 18–22 g were supplied by Changchun Yisi Experimental Animal Technology Co. Ltd. The animals were kept under standard laboratory conditions (temperature 25 ± 2 °C, relative humidity 60 ± 5%, 12 h light/12 h dark cycle with light on at 7:00 a.m.) with food and tap water available ad libitum for the duration of the study. After 1 week of acclimatization, all mice were randomly divided into different groups. All tests were performed in accordance with the Guide for Animal Experimentation of Jilin Agricultural University. The protocol was approved by the Jilin Agricultural University Institutional Animal Care and Use Committee. Every effort was made to minimize the number of animals used and any pain and discomfort experienced by the subjects.
2.2. Drugs and reagents
HBA, 3-furoic acid, 2-naphthoic acid, (Z)-9-octadecanoic acid (oleic acid), 2,4-hexadienoic acid (sorbic acid), octadecadienoic acid (linoleic acid), dodecanoic acid (lauric acid) and benzoic acid were purchased from Sinopharm Chemical Reagent Co. Ltd. (Shanghai, China). 4-Dimethylaminopyridine (DMAP) and 1-ethylethyl-3-(3-dimethyllaminopropyl) carbodiimide hydrochloride (EDCI) were purchased from Civi Chem Co. Ltd (Shanghai, China). The HBA derivatives were synthesized by us. Dichloromethane was acquired from Damao Chem Co. Ltd. (Tianjin, China). Diazepam (DZP) was provided by Zhenxing Pharma Co. Ltd. (Taiyuan, China). Sodium pentobarbital was purchased from Merck Co. Ltd. (Shanghai, China). The flumazenil (FLU) injection was produced by Lingkang Co. Ltd. (Hainan, China). p-Chlorophenylalanine (pCPA), thiosemicarbazide (TSC) and 5-hydroxytryptophan (5-HTP) were all purchased from Melone Pharma Co. Ltd. (Dalian, China). All the reagents used in this study were of analytical grade.
2.3. Synthesis
The experimental procedure is described taking 4-hydroxybenzyl alcohol 3-furoate (FHBA) and 4-hydroxybenzyl alcohol 3-furancarboxylic acid diester (2FHBA) as an example. 3-Furancarboxylic acid (6 mmol) and EDCI (8 mmol) were dissolved in 10 mL of dichloromethane and stirred for 10 minutes; then, 10 mL of dichloromethane solution containing HBA (4 mmol) and DMAP (4.8 mmol) was added to the mixture. After dissolution, the mixture was heated to reflux at 60 °C for 6 hours and the solvent was removed under reduced pressure. The residue was purified by silica gel chromatography and eluted with petroleum ether/acetone (6
:
1, v/v) to yield the product 2FHBA and FHBA. The synthesis of the other derivatives was similar to that of FHBA and 2FHBA.
2.4. Structural identification
The structures of the HBA derivatives were determined by IR, NMR and MS analysis. FTIR analysis was performed on a WGH-30/30A double beam infrared spectrophotometer (Gangdong Sci. & Tech. Development Co., Ltd. Tianjin, China). NMR spectra were recorded on a Varian Mercury 300 MHz NMR spectrometer equipped with an Oxford Instruments Ltd. superconducting magnet (Palo Alto, CA, USA). The HRMS was measured using a Q-Exactive Electrospray Orbitrap High Resolution Mass Spectrometer (Thermo-Fisher, USA). Melting point analysis was performed in an X-4 micro melting point apparatus (Tech Instrument Co., Ltd., Beijing, China). Specific conclusions are described in detail in the Results section.
2.5. Treatment
Ten derivatives of HBA and DZP were blended with 0.05% sodium carboxymethyl cellulose (CMC-Na)/physiological saline and administered 30 minutes prior to the locomotor activity test or pentobarbital administration. Sodium pentobarbital was dissolved in physiological saline, using 28 mg kg−1 (intraperitoneally, i.p.) as the sub-hypnotic dose and 42 mg kg−1 (i.p.) as the hypnotic dose, which could generate a 100% rate of sleep onset. pCPA (100 mg kg−1, i.p.) was administered continuously for 4 days to obtain the mouse insomnia model. After the insomnia model was successfully established, 2FHBA was administered for observation. TSC (8 mg kg−1, i.p.), diluted in 0.9% physiological saline, was injected 30 min before the administration of 2FHBA. 5-HTP (2.5 mg kg−1, i.p.) also diluted in 0.9% physiological saline was given 15 min prior to pentobarbital administration. FLU injection was also administrated 15 min prior to the administration of pentobarbital to obtain an insomnia model. All the drug solutions were freshly prepared before use.
2.6. Spontaneous locomotor activity test (SLT)
The sedative effects of HBA and its derivatives were assessed by detecting the spontaneous locomotor activity of mice. Locomotor activity and standing numbers could be calculated using the ZZ-6 locomotor activity tester (Taimeng Software Co. Ltd., Chengdu, China), which consisted of a microcomputer vehicle system and six separable reaction jars that possessed 36 points of infrared array probes. 30 min after oral administration of HBA and its derivatives and DZP respectively, mice were placed separately in the locomotor activity tester. After adapting for 5 min, the locomotor activity of each mouse was recorded for 5 min. After each testing session, the enclosure was thoroughly cleaned with 70% ethanol.
2.7. Pentobarbital-induced sleep test
Experiments were carried out between 8:00 a.m. and 12:00 a.m. Following sodium pentobarbital injection, each mouse was observed for the onset of sleep. When the mice lost their righting reflex for over 60 s, they were considered to be asleep. The loss of the righting reflex was defined as a failure of the mouse to right itself for at least 15 s after being placed on its back. Sleep latency was recorded as the time of pentobarbital injection to the time of sleep onset, and the sleep duration was defined as the elapsed time between the righting reflex loss and recovery.
2.8. Acute toxicity test
The acute toxicity of 2FHBA was investigated according to the Organization for Economic Cooperation and Development guideline 423 (OECD423).33 40 mice were randomly divided into four groups with 5 males and 5 females per group. 2FHBA was blended with 0.05% sodium carboxymethyl cellulose (CMC-Na)/physiological saline and given orally only once at doses of 1000, 2000 and 5000 mg kg−1 for detailed assessment of signs of toxicity (e.g., hypo-activity, breathing difficulty, convulsion and abnormal movements). The control group only received the same volume of vehicle. All groups were carefully observed for mortality and changes in general behavior for the first 24 h and for a total of 14 days. The LD50 value was determined according to the method described by the OECD Guideline 423.
2.9. Biochemical measurements
The brains of the mice were washed with ice-cold physiological saline and homogenized, shaken for 10 s and centrifuged at 10
000 × g for 10 min at 4 °C. The monoamine neurotransmitters including 5-HT and GABA were measured according to the instructions of competitive enzyme-linked immunosorbent assay kits, which were purchased from US R&D Systems, Ltd. (Minneapolis, USA).
2.10. Statistical analysis
All values were expressed as mean ± S.D. The data were statistically analyzed using the t-test and one-way analyses of variance (ANOVAs), followed by Tukey's post-hoc multiple comparison test. A value of p < 0.05 was considered statistically significant.
3. Results
3.1. Chemistry
The HBA derivatives (Fig. 1) were obtained via the reaction of HBA with carboxylic acids in dichloromethane at a temperature of 60 °C by reflux. DMAP was used as a catalyst. EDCI reacts first with the acid to form an activated carboxylic acid and then this activated carboxylic acid reacts with HBA to afford the derivatives. The products were isolated using a silica gel column. IR and NMR were used to identify the molecular structure of the newly synthesized HBA esters. HRMS (ESI) was used to determine the molecular weight of the derivatives. In the IR spectra of the derivatives, the characteristic peaks of the carbonyl group from 1765 cm−1 to 1731 cm−1 indicated the presence of the ester. In the 1H-NMR spectra of the new HBA esters, the signal at 5.0–6.0 (s, 1H) disappeared, indicating that the hydroxyl was esterified; the signal at 9.0–9.5 (s, 1H) disappeared, suggesting that the phenolic hydroxyl was esterified. In the 13C-NMR spectra of all HBA esters, the presence of signals at 165.0–175.0 ppm suggests the introduction of ester bonds. By comparing the IR, 1H-NMR, 13C-NMR and HRMS spectra, it was shown that the esterification reaction was successful. In all, we got 4-hydroxybenzyl alcohol 3-furoate (FHBA), 4-hydroxybenzyl alcohol 3-furancarboxylic acid diester (2FHBA), 4-hydroxybenzyl alcohol 2-naphthoate (NHBA), 4-hydroxybenzyl alcohol 2-naphthoic acid diester (2NHBA), 4-hydroxybenzyl alcohol benzoate (BHBA), 4-hydroxybenzyl alcohol benzoic acid diester (2BHBA), 4-hydroxybenzyl alcohol oleic acid diester (OHBA), 4-hydroxybenzyl alcohol linoleic acid diester (LoHBA), 4-hydroxybenzyl alcohol sorbic acid diester (SHBA), and 4-hydroxybenzyl alcohol lauric acid diester (LHBA).
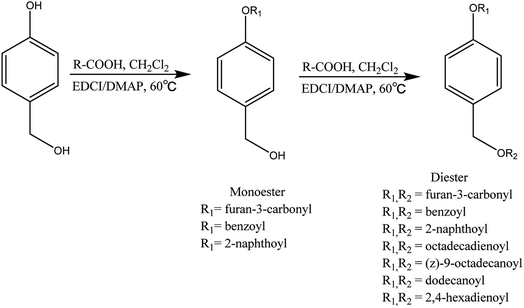 |
| Fig. 1 Compounds of the synthesis process and substituents. | |
3.1.1. 4-Hydroxybenzyl alcohol 3-furancarboxylic acid diester (2FHBA). Yield: 51.72%, white powder, melting point: 233–234 °C, HRMS (ESI) calcd for [M + H]+ C17H12O6+: 312.0634, found: 312.0639. IR spectrum: the peak at 3148 cm−1 is the stretching vibration peak of ArC–H; that at 2980 cm−1 is the hydrocarbon stretching vibration peak (νC–H) of CH2. The elongation vibration peak (νC
O) of the carbonyl group is shown at 1734 cm−1, suggesting the presence of the ester. The peak at 1685 cm−1 is the stretching vibration peak (νC
C) of C
C. The peaks at 1607 cm−1, 1575 cm−1, 1507 cm−1, and 1464 cm−1 are the characteristic absorption peaks of the benzene ring.1H-NMR (300 MHz, CDCl3) δ ppm: 8.199 (s, 1H, 17-H), 8.047 (s, 1H, 12-H), 7.509 (t, 1H, J = 1.5 Hz, 16-H), 7.478 (dt, 1H, J = 8.4, 2.7 Hz, 15-H), 7.431 (t, 2H, 3, 5-H), 7.212 (t, 1H, J = 2.7 Hz, 10-H), 7.189 (t, 1H, J = 1.8 Hz, 11-H), 6.876 (d, 1H, J = 1.8 Hz, 2-H), 6.773 (d, 1H, J = 2.1 Hz, 6-H), 5.297 (s, 2H, 7-H), 1.607–0.075 (others); 13C-NMR (75 MHz, CDCl3) δ ppm: 162.774 (C8), 161.204 (C13), 150.275 (C16), 148.699 (C11), 147.882 (C4), 144.057 (C17), 143.755 (C12), 133.613 (C1), 129.469 (C2, C6), 121.815 (C3, C5), 119.131 (C14), 118.681 (C9), 109.973 (C15), 109.784 (C10), 65.459 (C7).
3.1.2. 4-Hydroxybenzyl alcohol 3-furoate (FHBA). Yield: 47.65%, white powder, melting point: 145–146 °C, HRMS (ESI) calcd for [M + H]+ C12H10O4+: 218.0579, found: 218.0585. IR spectra: the peak at 3462 cm−1 is the stretching vibration peak (νOH) of the hydroxyl group; that at 3047 cm−1 is the hydrocarbon stretching vibration peak (νArC–H) of the benzene ring. The peak at 2884 cm−1 is the hydrocarbon stretching vibration peak (νC–H) of CH2. The telescopic vibration peak (νC
O) of the carbonyl group is at 1733 cm−1. The peak at 1651 cm−1 is the stretching vibration peak (νC
C) of olefin. The peaks at 1603 cm−1, 1507 cm−1, and 1455 cm−1 are the characteristic absorption peaks of the benzene ring.1H-NMR (300 MHz, CDCl3) δ ppm: 8.199 (m, 1H, 12-H), 7.506 (t, 1H, J = 1.8 Hz, 11-H), 7.429 (dt, 1H, J = 8.7, 2.1 Hz, 10-H), 7.191 (dt, 2H, J = 8.4, 2.4 Hz, 3, 5-H), 6.875 (dd, 2H, J = 1.8, 0.9 Hz, 2, 6-H), 5.292 (s, 1H, 7-OH), 4.698 (s, 2H, 7-H); 13C-NMR (75 MHz, CDCl3) δ ppm: 161.461 (C8), 149.551 (C11), 147.881 (C4), 143.983 (C12), 138.644 (C1), 129.417 (C2, C6), 127.942 (C3, C5), 121.572 (C9), 109.906 (C10), 64.373 (C7).
3.1.3. 4-Hydroxybenzyl alcohol linoleic acid diester (LoHBA). Yield: 87.61%, light yellow oily liquid, HRMS (ESI) calcd for [M + H]+ C43H68O4+: 648.5118, found: 648.5126. IR spectra: the peak at 3022 cm−1 is the hydrocarbon stretching vibration peak (νArC–H) of the benzene ring. The peak at 2940 cm−1 is the stretching vibration peak (νas C–H) of CH2. The peak at 2867 cm−1 is the hydrocarbon stretching vibration peak (νs C–H) of CH2. The peaks at 1765 cm−1 and 1746 cm−1 are telescopic vibration peaks (νC
O) of the carbonyl group; those at 1611 cm−1, 1513 cm−1, and 1458 cm−1 are the telescopic vibration peaks of the benzene ring. The peaks at 1383 cm−1 and 1353 cm−1 are the deformation vibration peaks of the methyl group (δCH3).1H-NMR (300 MHz, CDCl3) δ ppm: 7.374 (d, 2H, J = 8.4 Hz, 3, 5-H), 7.079 (d, 2H, J = 8.4 Hz, 2, 6-H), 5.352 (m, 8H, 37, 35, 20, 19, 34, 38, 16, 17-H), 5.091 (s, 2H, 7-H), 2.794 (t, 4H, J = 5.7 Hz, 18, 35-H), 2.753 (t, 2H, J = 7.5 Hz, 27-H), 2.364 (t, 2H, J = 7.5 Hz, 9-H), 2.058 (t, 8H, J = 6.6 Hz, 15, 21, 33, 39-H), 1.773 (m, 4H, 10, 28-H), 1.301 (m, 24H, 11, 29, 13, 31, 14, 32, 22, 40, 23, 41, 24, 42-H), 0.888 (m, 6H, 25, 43-H); 13C-NMR (75 MHz, CDCl3) δ ppm: 173.441 (C8), 172.032 (C26), 150.529 (C4), 133.566 (C1), 130.120 (C16, C20), 129.952 (C34), 129.902 (C38), 129.323 (C2, C6), 128.031 (C17), 127.977 (C19), 127.847 (C35, C37), 121.619 (C3, C5), 65.327 (C7), 34.301 (C36), 34.221 (C9), 31.864 (C33), 31.477 (C39), 29.720 (C27), 29.629 (C23, C41), 29.536 (C14, C32), 29.479 (C13), 29.414 (C31), 29.276 (C22), 29.203 (C40), 29.102 (C12, C30), 29.039 (C11, C29), 27.155 (C15, C21), 25.588 (C18), 24.856 (C10, C28), 22.640 (C24), 22.529 (C42), 14.059 (C25), 14.019 (C43).
3.1.4. 4-Hydroxybenzyl alcohol lauric acid diester (LHBA). Yield: 91.33%, white powder, melting point: 280–281 °C, HRMS (ESI) calcd for [M + H]+ C31H52O4+: 488.3866, found: 488.3872. IR spectra: the peak at 2968 cm−1 is the stretching vibration peak (νas C–H) of CH3. The peak at 2932 cm−1 is the stretching vibration peak (νas C–H) of CH2, and that at 2860 cm−1 is the stretching vibration peak (νs C–H) of CH2. The telescopic vibration peaks (νC
O) of the carbonyl group are at 1761 cm−1 and 1740 cm−1. The peaks at 1602 cm−1, 1554 cm−1, 1506 cm−1 and 1467 cm−1 are the telescopic vibration peaks of the benzene ring. The peak at 1383 cm−1 is the deformation vibration peak of methyl group (δCH3).1H-NMR (300 MHz, CDCl3) δ ppm: 7.374 (d, 2H, J = 8.4 Hz, 3, 5-H), 7.079 (d, 2H, J = 8.4 Hz, 2, 6-H), 5.091 (s, 2H, 7-H), 2.572 (t, 2H, J = 7.5 Hz, 21-H), 2.363 (t, 2H, J = 7.5 Hz, 9-H), 1.771 (m, 4H, 10, 22-H), 1.251 (s, 32H, 11, 12, 13, 14, 15, 16, 17, 18, 23, 24, 25, 26, 27, 28, 29, 30-H), 0.899 (t, 6H, J = 6.3 Hz, 19, 31-H); 13C-NMR (75 MHz, CDCl3) δ ppm: 173.463 (C8), 172.065 (C20), 150.459 (C4), 133.497 (C1), 129.258 (C2, C6), 121.562 (C3, C5), 65.266 (C7), 34.257 (C9), 34.182 (C21), 31.788 (C17, C29), 29.475 (C13, C25), 29.330 (C14, C26), 29.211 (C15, C27), 29.127 (C12, C24), 29.007 (C16, C28), 28.979 (C11, C23), 24.807 (C10, C22), 22.562 (C18, C30), 13.981 (C19, C31).
3.1.5. 4-Hydroxybenzyl alcohol sorbic acid diester (SHBA). Yield: 82.38%, light yellow solid, melting point: 132–133 °C, HRMS (ESI) calcd for [M + H]+ C19H20O4+: 312.1362, found: 312.1369. IR spectra: the peak at 3047 cm−1 is the hydrocarbon stretching vibration peak (νArC–H) of the benzene ring. The peak at 2983 cm−1 is the stretching vibration peak (νC–H) of CH2. The peak at 2875 cm−1 is the stretching vibration peak (νC–H) of the CH3; those at 1761 cm−1 and 1740 cm−1 are the stretching vibration peaks (νC
O) of the carbonyl group; and that at 1647 cm−1 is νC
C, indicating the presence of olefin. The peaks at 1610 cm−1, 1509 cm−1, and 1450 cm−1 are the telescopic vibration peaks of the benzene ring.1H-NMR (300 MHz, CDCl3) δ ppm: 7.409 (d, 2H, J = 8.4 Hz, 10, 16-H), 7.260 (m, 2H, 3, 5-H), 7.132 (d, 2H, J = 8.4 Hz, 2, 6-H), 6.279 (m, 2H, 11, 17-H), 5.983 (d, 2H, J = 15.3 Hz, 12, 18-H), 5.835 (d, 2H, J = 15.6 Hz, 9, 15-H), 5.170 (s, 2H, 7-H), 1.906 (dd, 6H, J = 5.1, 6.9 Hz, 13, 19-H); 13C-NMR (75 MHz, CDCl3) δ ppm: 166.835 (C14), 165.349 (C8), 150.589 (C4), 146.826 (C16), 145.358 (C10), 140.489 (C12), 139.406 (C18), 133.503 (C1), 129.651 (C11), 129.621 (C17), 129.197 (C2, C6), 121.587 (C3, C5), 118.482 (C9), 117.833 (C15), 65.273 (C7), 18.515 (C13), 18.413 (C19).
3.1.6. 4-Hydroxybenzyl alcohol benzoic acid diester (2BHBA). Yield: 56.15%, white powder, melting point: 225–226 °C, HRMS (ESI) calcd for [M + H]+ C21H16O4+: 332.1048, found: 332.1057. IR spectra: the peak at 3076 cm−1 is the telescopic vibration peak of ArC–H, and the peak at 2974 cm−1 is the telescopic vibration peak (νC–H) of the CH2. The telescopic vibration peaks (νC
O) of the carbonyl group are at 1737 cm−1 and 1710 cm−1. The peaks at 1602 cm−1, 1507 cm−1, 1496 cm−1, and 1455 cm−1 are the telescopic vibration peaks of the benzene ring.1H-NMR (300 MHz, CDCl3) δ ppm: 8.223 (dt, 2H, J = 6.9, 3.0 Hz, 17, 21-H), 8.102 (dt, 2H, J = 6.9, 3.0 Hz, 10, 14-H), 7.671 (m, 6H, 19, 12, 18, 20, 11, 13-H), 7.260 (d, 2H, J = 2.1 Hz, 3, 5-H), 7.238 (d, 2H, J = 1.8 Hz, 2, 6-H), 5.384 (s, 2H, 7-H); 13C-NMR (75 MHz, CDCl3) δ ppm: 166.197 (C8), 164.922 (C15), 150.729 (C4), 133.602 (C19), 133.526 (C12), 132.949 (C1), 130.039 (C17, C21), 129.904 (C16), 129.567 (C9), 129.364 (C10, C14), 129.277 (C2, C6), 128.454 (C11, C13), 128.267 (C18, C20), 121.789 (C3, C5), 65.924 (C7).
3.1.7. 4-Hydroxybenzyl alcohol benzoate (BHBA). Yield: 35.17%, colorless flaky crystals, melting point: 139–140 °C, HRMS (ESI) calcd for [M + H]+ C14H12O3+: 228.0786, found: 228.0793. IR spectra: the peak at 3508–3102 cm−1 is the stretching vibration peak (νOH) of the hydroxyl group; that at 3052 cm−1 is the telescopic vibration peak (νArC–H) of benzene. The peak at 2932 cm−1 is the stretching vibration peak (νC–H) of the CH2. The telescopic vibration peak (νC
O) of the carbonyl group is 1737 cm−1; the peaks at 1602 cm−1, 1587 cm−1, 1507 cm−1 and 1455 cm−1 are the telescopic vibration peaks of the benzene ring.1H-NMR (300 MHz, CDCl3) δ ppm: 8.219 (dt, 2H, J = 7.2, 1.2 Hz, 10, 14-H), 7.669 (m, 3H, 12, 11, 13-H), 7.447 (dt, 2H, J = 7.8, 2.1 Hz, 3, 5-H), 7.254 (s, 1H, 7-OH), 7.228 (dt, 2H, J = 8.4, 2.7 Hz, 2, 6-H), 4.706 (s, 2H, 7-H); 13C-NMR (75 MHz, CDCl3) δ ppm: 165.085 (C8), 150.162 (C4), 138.399 (C1), 133.452 (C12), 130.001 (C10, C14, C9), 128.399 (C2, C6), 127.948 (C11, C13), 121.629 (C3, C5), 64.581 (C7).
3.1.8. 4-Hydroxybenzyl alcohol 2-naphthoic acid diester (2NHBA). Yield: 58.23%, white powder, melting point: 407–408 °C, HRMS (ESI) calcd for [M + H]+ C29H20O4+: 432.1362, found: 432.1366. IR spectra: the peak at 3074 cm−1 is the telescopic vibration peak (νArC–H) of benzene. The peak at 2952 cm−1 is the stretching vibration peak (νC–H) of the CH2. The peaks at 1740 cm−1 and 1731 cm−1 are the stretching vibration peaks (νC
O) of the carbonyl group. The peaks at 1601 cm−1, 1560 cm−1, 1509 cm−1, and 1466 cm−1 are the telescopic vibration peaks of the benzene ring.1H-NMR (300 MHz, CDCl3) δ ppm: 8.793 (s, 1H, 25-H), 8.657 (s, 1H, 10-H), 8.215 (m, 6H, 20, 19, 16, 21, 15, 24-H), 8.124 (dd, 2H, J = 1.8, 6.9 Hz, 11, 14-H), 8.019 (m, 2H, 22, 23-H), 7.661 (m, 2H, 12, 13-H), 7.336 (d, 2H, J = 2.1 Hz, 3, 5-H), 7.314 (d, 2H, J = 1.8 Hz, 2, 6-H), 5.460 (s, 2H, 7-H); 13C-NMR (75 MHz, CDCl3) δ ppm: 166.466 (C8), 165.196 (C17), 150.921 (C4), 135.756 (C14-15), 135.513 (C20–21), 133.711 (C1, C10–11, C24–25), 132.401 (C10, C25), 131.909 (C11), 131.174 (C24), 129.565 (C2, C6), 129.409 (C18), 129.313 (C13), 128.598 (C22), 128.348 (C15), 128.238 (C20), 128.138 (C9), 127.758 (C14), 127.689 (C21), 126.787 (C12), 126.594 (C23), 125.346 (C16), 125.201 (C19), 121.948 (C3, C5), 66.179 (C7).
3.1.9. 4-Hydroxybenzyl alcohol 2-naphthoate (NHBA). Yield: 38.11%, white flaky crystals, melting point: 242–243 °C, HRMS (ESI) calcd for [M + H]+ C18H14O3+: 278.0943, found: 278.0949. IR spectra: the peak at 3394 cm−1 is the stretching vibration peak (νOH) of the hydroxyl group; that at 3070 cm−1 is the stretching vibration peak of ArC–H. The peak at 2878 cm−1 is the stretching vibration peak (νC–H) of the CH2. The telescopic vibration peak (νC
O) of the carbonyl group is at 1737 cm−1. The peaks at 1601 cm−1, 1507 cm−1, and 1466 cm−1 are the telescopic vibration peaks of the benzene ring.1H-NMR (300 MHz, CDCl3) δ ppm: 8.783 (s, 1H, 10-H), 8.206 (d, 2H, J = 1.8 Hz, 15, 16-H), 8.177 (d, 2H, J = 1.8 Hz, 14, 11-H), 8.011 (m, 2H, 12, 13-H), 7.470 (dt, 2H, J = 8.7, 2.4 Hz, 3, 5-H), 7.281 (dt, 2H, J = 8.7, 2.4 Hz, 2, 6-H), 4.721 (s, 2H, 7-H); 13C-NMR (75 MHz, CDCl3) δ ppm: 165.325 (C8), 150.324 (C4), 138.495 (C1), 135.722 (C14-15), 132.386 (C10–11), 131.841 (C10), 129.377 (C11), 128.541 (13), 128.296 (C2, C6), 128.048 (C9), 127.726 (C15), 126.742 (C14), 126.556 (C12), 125.326 (C16), 121.755 (C3, C5), 64.669 (C7).
3.1.10. 4-Hydroxybenzyl alcohol oleic acid diester (OHBA). Yield: 93.04%, light yellow oily liquid, HRMS (ESI) calcd for [M + H]+ C43H72O4+: 652.5431, found: 652.5437. IR spectra: the peak at 3070 cm−1 is the stretching vibration peak (νArC–H) of the benzene. The peak at 2860 cm−1 is the stretching vibration peak (νC–H) of CH2. The telescopic vibration peaks (νC
O) of the carbonyl group are at 1763 cm−1 and 1743 cm−1. The peaks at 1605 cm−1, 1505 cm−1, and 1467 cm−1 are the telescopic vibration peaks of the benzene ring. The peak at 1380 cm−1 is the deformation vibration peak of the methyl group (δCH3).1H-NMR (300 MHz, CDCl3) δ ppm: 7.374 (dt, 2H, J = 8.4, 2.7 Hz, 3, 5-H), 7.078 (dt, 1H, J = 8.7, 2.7 Hz, 2, 6-H), 5.359 (m, 4H, 16, 17, 34, 35-H), 5.09 (s, 2H, 7-H), 2.547 (t, 2H, J = 7.2 Hz, 27-H), 2.339 (t, 2H, J = 7.5 Hz, 9-H), 2.034 (m, 8H, 15, 18, 33, 36-H), 1.748 (m, 4H, 10, 28-H), 1.300 (m, 40H, 11, 29, 13, 31, 20, 38, 21, 39, 14, 32, 19, 37, 12, 30, 22, 23, 24, 40, 41, 42-H), 0.888 (m, 6H, 25, 43-H); 13C-NMR (75 MHz, CDCl3) δ ppm: 173.497 (C8), 172.093 (C26), 150.518 (C4), 133.555 (C1), 129.969 (C16), 129.916 (C17), 129.679 (C34), 129.634 (C35), 129.332 (C2, C6), 121.625 (C3, C5), 65.343 (C7), 34.313 (C9, C15, C18), 34.234 (C27, C33, C36), 31.853 (C23, C41), 29.714 (C14, C19, C32, C37), 29.625 (C20, C21, C38, C39), 29.529 (C13, C31), 29.469 (C12, C30), 29.268 (C22, C40), 29.035 (C11, C29), 25.578 (C10), 25.479 (C28), 22.629 (C24), 22.519 (C42), 14.057 (C25), 14.017 (C43).
3.2. Spontaneous locomotor activity test (SLT)
Spontaneous motor activity is usually considered as an effective index of alertness or a decrease in motor activity that leads to sedation,34 as a result of the reduced excitability of the central nervous system. Therefore, the spontaneous locomotor activities of mice treated with the newly synthesized HBA esters were detected using a ZZ-6 locomotor activity tester to determine their sedative effects. The number of autonomous activities was recorded after oral administration of the different HBA esters (20 mg kg−1) and diazepam (3 mg kg−1). Fig. 2A shows that the 2FHBA, SHBA, 2NHBA, NHBA, and OHBA groups displayed obvious sedative effects compared with the vehicle group (p < 0.05). However, 2FHBA (20 mg kg−1) exhibited the strongest sedative activity. Therefore, a series of dosages (5 mg kg−1, 10 mg kg−1, 20 mg kg−1 and 50 mg kg−1) of 2FHBA were investigated, and the results (Fig. 2B) demonstrated that 10 mg kg−1 of 2FHBA showed the best sedative effect, and the sub-sedative dose was 5 mg kg−1.
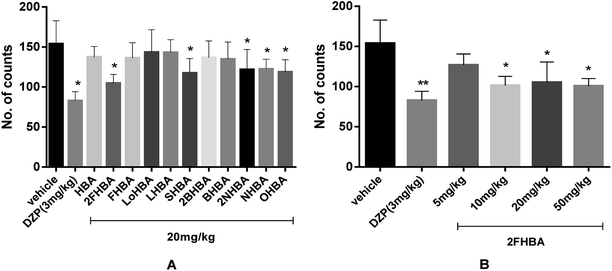 |
| Fig. 2 The sedative effect of HBA esters on spontaneous locomotor activity (A); the sedative effect of different doses of 2FHBA on spontaneous locomotor activity (B) (10 in each group). *p < 0.05 and **p < 0.01 compared with the vehicle group. | |
3.3. Screening of the HBA derivatives for hypnotic activity
Sodium pentobarbital (28 mg kg−1 or 42 mg kg−1) was used to record the falling asleep rate, sleep latency and sleep duration time. As we can see from Table 1, DZP, the positive control, showed the strongest hypnotic activity, in which the ratio of mice falling asleep was 100% (p < 0.01). Meanwhile, the 2FHBA group administered in combination with sodium pentobarbital (28 mg kg−1) also performed well, with 90% of the mice falling asleep (p < 0.01). The others did not exhibit an obvious effect. Fig. 3 shows that 2FHBA, SHBA, 2BHBA and 2NHBA combined with pentobarbital sodium (42 mg kg−1) significantly increased the sleep duration in mice (Fig. 3B, p < 0.05), but the reduction in sleep latency was not obvious except for that with 2FHBA (Fig. 3A). From the above results, we concluded that 2FHBA displayed the best hypnotic activity among the new esters.
Table 1 The effect of HBA esters on the sleep onset of mice treated with a sub-hypnotic dose of pentobarbital (28 mg kg−1)a
Group |
Dosage (mg kg−1, i.g.) |
No. falling asleep/total |
Sleep onset (%) |
Statistical analysis by chi-square test (the χ2 test); ##p < 0.01 compared with the vehicle group. |
Vehicle |
— |
1/10 |
10 |
DZP |
3 |
10/10 |
100## |
HBA |
20 |
1/10 |
10 |
2FHBA |
20 |
9/10 |
90## |
FHBA |
20 |
1/10 |
10 |
LoHBA |
20 |
1/10 |
10 |
LHBA |
20 |
1/10 |
10 |
SHBA |
20 |
1/10 |
10 |
2BHBA |
20 |
0/10 |
0 |
BHBA |
20 |
0/10 |
0 |
2NHBA |
20 |
2/10 |
20 |
NHBA |
20 |
0/10 |
0 |
OHBA |
20 |
0/10 |
0 |
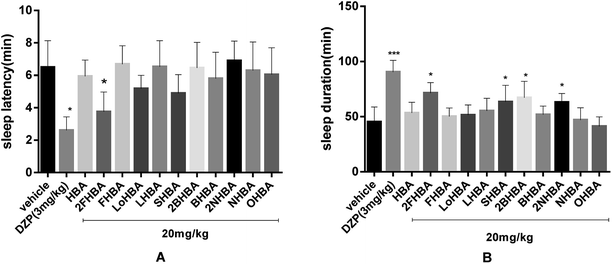 |
| Fig. 3 The hypnotic effect of HBA esters (2FHBA, FHBA, LoHBA, LHBA, SHBA, 2BHBA, BHBA, 2NHBA, NHBA, and OHBA) co-administered with pentobarbital (42 mg kg−1) on sleep in mice (10 in each group): the sleep latency (A) and the sleep duration (B); *p < 0.05, **p < 0.01 and ***p < 0.001 compared with the vehicle group. | |
3.4. Effect of 2FHBA on sleep in pentobarbital-treated mice
A series of doses of 2FHBA (5 mg kg−1, 10 mg kg−1, 20 mg kg−1 and 50 mg kg−1) were designed to evaluate its hypnotic activity in combination with sodium pentobarbital (42 mg kg−1). The results showed that 10 mg kg−1 and 20 mg kg−1 of 2FHBA significantly prolonged the sleeping time (p < 0.05, Fig. 4B), but only administration of 10 mg kg−1 significantly decreased the sleep latency of the mice (p < 0.05, Fig. 4A). Furthermore, the hypnotic effect of 2FHBA was dose-dependent, but this dependence is not obvious. 10 mg kg−1 of 2FHBA showed specific hypnotic activity, while 5 mg kg−1 represented the sub-hypnotic dose. These two doses were used in the following mechanism research.
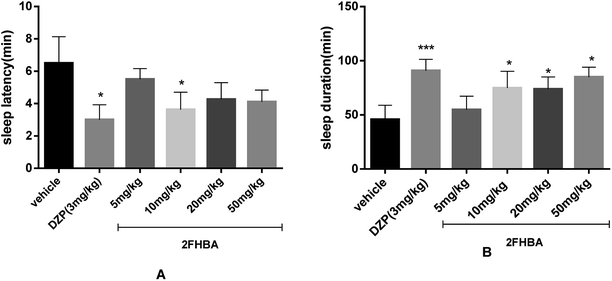 |
| Fig. 4 The hypnotic effect of 2FHBA at different doses (5 mg kg−1, 10 mg kg−1, 20 mg kg−1 and 50 mg kg−1) co-administered with pentobarbital (42 mg kg−1) in mice (10 in each group): the sleep latency (A) and the sleep duration (B); *p < 0.05, **p < 0.01 and ***p < 0.001 compared with the vehicle group. | |
3.5. Effect of 2FHBA on pCPA-induced insomnia in pentobarbital-treated mice
Fig. 5 shows that after 4 days of pCPA treatment (100 mg kg−1, i.p.), the sleep latency of mice was increased, and the sleep duration was reduced (p < 0.05), suggesting that a successful insomnia model was established. After administration of 2FHBA (10 mg kg−1), the sleep latency was significantly shortened (Fig. 5A, p < 0.05), but there was no statistically significant change in sleep duration, although it was prolonged (Fig. 5B).
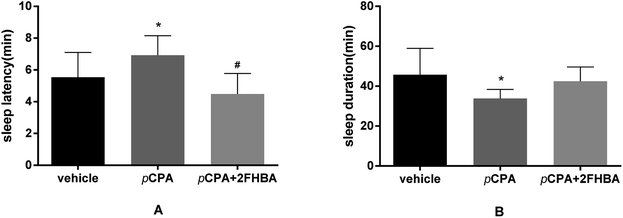 |
| Fig. 5 Effect of 2FHBA (10 mg kg−1) on pCPA-induced insomnia in pentobarbital-treated mice (10 in each group): the sleep latency (A) and sleep duration (B); all values are presented as mean ± SD of the mean. *p < 0.05 and **p < 0.01 compared with the vehicle group. | |
3.6. Synergic effects of 2FHBA and 5-HTP on sleep induced by pentobarbital
In order to determine whether the hypnotic effect of 2FHBA was related to the serotonergic system, synergistic administration of sub-hypnotic doses of 2FHBA (5 mg kg−1) and 5-HTP (2.5 mg kg−1) was performed. As shown in Fig. 6, the sleep latency was significantly reduced (p < 0.01), and the sleep duration was significantly prolonged (p < 0.001). Combined with the results of the pCPA-induced model, this suggests that the serotonergic system might be involved in the hypnotic effects of 2FHBA.
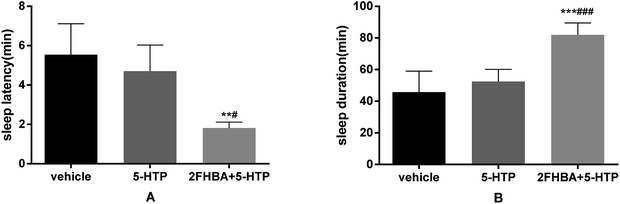 |
| Fig. 6 Synergistic effect of 2FHBA (5 mg kg−1) with 5-HTP (2.5 mg kg−1) on pentobarbital-treated sleep in mice (10 in each group): the sleep latency (A) and sleep duration (B); all values are presented as the mean ± SD. *p < 0.05, **p < 0.01 and ***p < 0.001 compared with the vehicle group; #p < 0.05, ##p < 0.01 and ###p < 0.001 compared with the vehicle model group treated with 5-HTP. | |
3.7. Effect of 2FHBA on the hypnotic-reversing action of flumazenil in pentobarbital-treated mice
Fig. 7 shows that administration of FLU (8 mg kg−1) alone slightly prolonged the sleep latency and shortened the sleep duration. In addition, the hypnotic effect of 2FHBA (10 mg kg−1, p < 0.05) was inhibited by FLU compared with the vehicle group, which was similar to the effect of DZP (3 mg kg−1) co-administered with FLU.
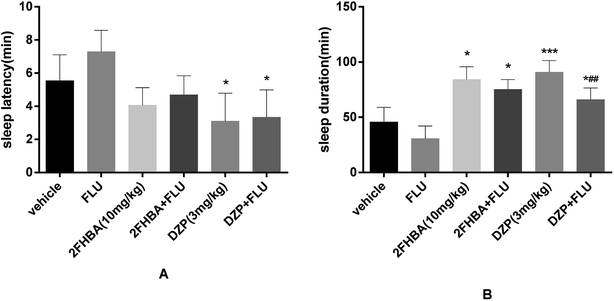 |
| Fig. 7 Effect of 2FHBA (10 mg kg−1) on FLU-induced insomnia in pentobarbital-treated mice (10 in each group): the sleep latency (A) and sleep duration (B); all values are presented as mean ± SD. *p < 0.05 and **p < 0.01 compared with the vehicle group; #p < 0.05 and ##p < 0.01, compared with the diazepam group (3 mg kg−1). | |
3.8. Effect of 2FHBA on thiosemicarbazide-induced sleep disturbance in mice
In this study, we could see an obvious insomnia model induced by TSC alone (8 mg kg−1, p < 0.05). However, 2FHBA (10 mg kg−1) reversed the TSC-induced insomnia. Compared with the TSC group, the sleep latency of the co-administration group was significantly shortened (p < 0.05, Fig. 8A) and the duration of sleep was significantly prolonged (p < 0.01, Fig. 8B). The above results suggested that the hypnotic effect of 2FHBA was related to the GABAergic system.
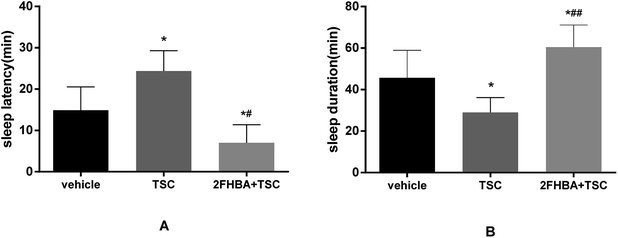 |
| Fig. 8 Effect of 2FHBA (10 mg kg−1) on TSC-induced insomnia in pentobarbital-treated mice (10 in each group): the sleep latency (A) and sleep duration (B); all values are presented as mean ± SD. *p < 0.05 and **p < 0.01 compared with the vehicle group; #p < 0.05 and ##p < 0.01 compared with the TSC-treated group. | |
3.9. Acute toxicity test results
In the acute toxicity study, 2FHBA (1000, 2000 and 5000 mg kg−1, i.g.) did not produce any mortality in the mice during the observation period. The 1000 and 2000 mg kg−1 treated groups did not show any adverse clinical signs compared to the control group. Meanwhile, the dose of 5000 mg kg−1 seemed to affect the female mice on the first day, as this group showed a small decrease in their intake of food and water, but this symptom gradually disappeared in the subsequent days. As the acute administration of 2FHBA did not produce death at the maximum dose of 5000 mg kg−1, the LD50 could not be estimated, and it was considered to be greater than 5000 mg kg−1. The early acute toxicity study of HBA and GAS also showed non-toxicity.35 Therefore, we considered 2FHBA to be non-toxic.
3.10. Effects of 2FHBA on the levels of 5-HT and GABA in drug-treated mice
To verify whether the sedative–hypnotic effect of 2FHBA was connected with the 5-HTergic and GABAergic systems, we detected the levels of 5-HT and GABA in the brains of the mice in the relevant administration groups. As Fig. 9A shows, the content of 5-HT was obviously increased in the 2FHBA group (p < 0.01). In the 2FHBA combined with pretreatment with pCPA group, the 5-HT level was obviously increased (p < 0.05) compared with that in the pCPA group. In the 5-HTP group, the content of 5-HT was higher than that in the control group (p < 0.01). In the combined use of 2FHBA and 5-HTP group, the 5-HT level was higher than that in the 5-HTP group (p < 0.01). Fig. 9B shows that the levels of GABA in the brains of the mice administered with 2FHBA were higher than the others, especially the control group (p < 0.05). The GABA level of 2FHBA combined with FLU was higher than that with FLU alone (p < 0.05). The level of GABA in the 2FHBA combined with TSC group was higher than that in the TSC group (p < 0.05).
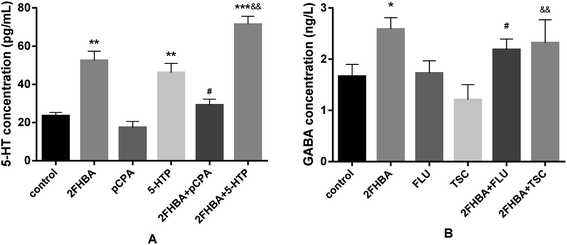 |
| Fig. 9 The content of 5-HT in the brains of mice in the 2FHBA group, pCPA group, 5-HTP group, 2FHBA + pCPA group and 2FHBA+5-HTP group (A). The content of GABA in the brains of mice in the 2FHBA group, FLU group, TSC group, 2FHBA + FLU group and 2FHBA + TSC group (B). The values represent the mean ± SD (n = 10 in each group), compared with the control group, *p < 0.05, **p < 0.01 and ***p < 0.001; compared with the pCPA (100 mg kg−1, i.g.) (FLU (B) (8 mg kg−1)) group, #p < 0.05; and compared with the 5-HTP (2.5 mg kg−1) (TSC (B) (8 mg kg−1)) group, &p < 0.05 and &&p < 0.01. One-way ANOVA, and a post-hoc Tukey test were used. | |
4. Discussion
In this study, the original chemical structure of HBA was modified by reaction with organic acids in dichloromethane. We obtained 10 new compounds, which were screened for sedative and hypnotic activity for the first time. As is well-known, sleep is a sophisticated physiological process which is affected by a network of neuronal systems and is hard to reproduce in vitro.36,37 In this study, the sedative effects of the derivatives were determined through artificial screening using the spontaneous locomotor activity test. In addition, the classical behavioral pharmacology method, the pentobarbital-induced sleep test, was conducted to evaluate the hypnotic effect of the newly synthesized HBA esters. The present study showed that 2FHBA and SHBA significantly decreased the locomotor activity and potentiated the hypnotic effect of sodium pentobarbital, indicating their central nervous system depressant activity. Ultimately, 2FHBA, which had the strongest sedative and hypnotic activity, was selected for the subsequent experiments. Based on the results of dose screening, 10 mg kg−1 was selected as the effective hypnotic dose and 5 mg kg−1 was selected as the sub-hypnotic dose.
The hippocampus plays an important role in sleep activity. Since serotonergic projections in the hippocampus are diffuse in nature,38 the local effects of 5-HT are largely dependent on the 5-HT concentration. The hippocampus also modulates emotional processing in the central nervous system (CNS)39 via diffuse serotonergic projections from the midbrain raphe nuclei.38 In fact, 5-HT imbalances are often correlated with psychological disorders such as insomnia, anxiety, depression, and psychosis.40–42 Interestingly, 5-HT receptors also form homodimers or heterodimers among themselves or other G-protein-coupled receptors (GPCRs), respectively, which greatly enhances the complexity of their functions.43 pCPA is an inhibitor of 5-HT synthesis.44 In this study, the content of 5-HT in the brain was significantly decreased after pCPA was used alone, while 2FHBA increased the content of 5-HT. There was no doubt that the increase in 5-HT level contributed significantly to the sedative and hypnotic effect.45 5-HTP, which is the rate-limiting precursor in 5-HT biosynthesis, is used as an oral supplement to enhance serotonin levels in humans.46 In this study, co-administration of 5-HTP with 2FHBA significantly shortened the sleep latency and increased the duration of sleep in mice, and the content of 5-HT in the brains of the mice increased significantly compared with that in the control group, suggesting that 2FHBA exerted its sedative and hypnotic effect via the 5-HT system.
In addition to 5-HT, GABA is also considered as another important neurotransmitter associated with sleep modulation.47 The γ-aminobutyric acid-A (GABAA) receptor is a ligand-gated ion channel that modulates the levels of anxiety, insomnia, and memory.48 The GABAA receptor is a heteropentameric membrane protein that forms a GABA-gated chloride channel and is composed of several classes of subunits (α, β, γ, δ, and ε).49,50 Pharmacological studies suggested that classic benzodiazepines and other GABAA receptor positive modulators reduce anxiety and improve sleeping.51 Diazepam, as a representative sedative–hypnotic drug, releases GABA through binding with the GABAA-BZD receptor. In contrast, flumazenil as a specific benzodiazepine-derived (BZD) antagonist, can effectively induce insomnia. In this study, 2FHBA exhibited a stable hypnotic effect. We sought to determine the possible mechanisms of action. The hypnotic effects of 2FHBA were blocked by the GABAA receptor antagonist flumazenil, which indicated that the hypnotic activity of 2FHBA was attributed to the modulation of the GABAA-BZD receptor through the GABAergic system. As a central nervous stimulant, thiosemicarbazide is an inhibitor of glutamate decarboxylase, and its application will cause a reduction in the GABA content in the brain.34 From the above results, it was shown that 2FHBA can significantly improve insomnia caused by TSC, and 2FHBA with TSC treatment can significantly increase the content of GABA in the brain, which demonstrated that the hypnotic effect of 2FHBA was related to the GABAergic system. In order to assess the potential druggability of 2FHBA, the acute toxicity of 2FHBA was detected, and the results showed that the mice did not exhibit any signs of toxicity or mortality despite the dose being up to 5000 mg kg−1, so we considered 2FHBA to be safe.
5. Conclusions
In summary, 10 HBA derivatives were synthesized with simple control, and their sedative–hypnotic activities were evaluated. The results showed that 2FHBA showed the strongest sedative–hypnotic effects, and its hypnotic effect might be related to the serotonergic system and GABAergic system. Overall, this study demonstrated that the structural modification of HBA by esterification might be an effective method for its application in altering central nervous system function. 2FHBA has potential to be developed as an agent for insomnia therapy.
Conflicts of interest
The authors declare no conflict of interest.
Abbreviations
EDCI | 1-Ethylethyl-3-(3-dimethyllaminopropyl) carbodiimide hydrochloride |
DMAP | 4-Dimethylaminopyridine |
HBA | 4-Hydroxybenzyl alcohol |
2FHBA | 4-Hydroxybenzyl alcohol 3-furancarboxylic acid diester |
FHBA | 4-Hydroxybenzyl alcohol 3-furoate |
LoHBA | 4-Hydroxybenzyl alcohol linoleic acid diester |
SHBA | 4-Hydroxybenzyl alcohol sorbic acid diester |
LHBA | 4-Hydroxybenzyl alcohol lauric acid diester |
BHBA | 4-Hydroxybenzyl alcohol benzoate |
2BHBA | 4-Hydroxybenzyl alcohol benzoic acid diester |
2NHBA | 4-Hydroxybenzyl alcohol 2-naphthoic acid diester |
NHBA | 4-Hydroxybenzyl alcohol 2-naphthoate |
OHBA | 4-Hydroxybenzyl alcohol oleic acid diester |
SLT | Spontaneous locomotor activity test |
DZP | Diazepam |
pCPA | p-Chlorophenylalanine |
BZD | Benzodiazepine-derived |
5-HT | 5-Hydroxytryptamine |
5-HTP | 5-Hydroxytryptophan |
GABA | γ-Aminobutyric acid |
GABAA | γ-Aminobutyric acid-A |
FLU | Flumazenil |
TSC | Thiosemicarbazide |
CNS | Central nervous system |
GPCRs | G-protein-coupled receptors |
Acknowledgements
This work was supported by the Jilin Provincial Science and Technology Development Project (20180520060JH), the Jilin Provincial Education Department Fund Project (JJKH20170321KJ), the National Key Research and Development Project (2016YFC0500300) and the Jilin Provincial Key Scientific and Technological Project (20170204017YY).
References
- K. B. Hong, Y. Park and H. J. Suh, Sleep-promoting effects of the GABA/5-HTP mixture in vertebrate models, Behav. Brain Res., 2016, 310, 36–41 CrossRef PubMed.
- H. Wen, et al., Methyl 2-(2-(4-formylphenoxy)acetamido)-2-substituted acetate derivatives: a new class of acetylcholinesterase inhibitors, Bioorg. Med. Chem. Lett., 2007, 17(8), 2123–2125 CrossRef PubMed.
- Y. Zhang, et al., NHBA isolated from Gastrodia elata exerts sedative and hypnotic effects in sodium pentobarbital-treated mice, Pharmacol., Biochem. Behav., 2012, 102(3), 450–457 CrossRef PubMed.
- Y. Shi, et al., N(6)-(3-methoxyl-4-hydroxybenzyl) adenine riboside induces sedative and hypnotic effects via GAD enzyme activation in mice, Pharmacol., Biochem. Behav., 2014, 126, 146–151 CrossRef PubMed.
- C. L. Hsieh, et al., Gastrodia elata modulated activator protein 1 via c-Jun N-terminal kinase signaling pathway in kainic acid-induced epilepsy in rats, J. Ethnopharmacol., 2007, 109(2), 241–247 CrossRef PubMed.
- J. H. Ha, et al., 4-Hydroxybenzaldehyde from Gastrodia elata B1. is active in the antioxidation and GABAergic neuromodulation of the rat brain, J. Ethnopharmacol., 2000, 73(1–2), 329–333 CrossRef PubMed.
- J. W. Jung, et al., Anxiolytic-like effects of Gastrodia elata and its phenolic constituents in mice, Biol. Pharm. Bull., 2006, 29(2), 261–265 Search PubMed.
- S. H. Lin, et al., Down-regulation of Slit-Robo pathway mediating neuronal cytoskeletal remodeling processes facilitates the antidepressive-like activity of Gastrodia elata Blume, J. Agric. Food Chem., 2014, 62(43), 10493–10503 CrossRef PubMed.
- C. F. Ng, et al., The Aqueous Extract of Rhizome of Gastrodia elata Protected Drosophila and PC12 Cells against Beta-Amyloid-Induced Neurotoxicity, J. Evidence-Based Complementary Altern. Med., 2013, 516741 Search PubMed.
- E. Descamps, et al., Experimental stroke protection induced by 4-hydroxybenzyl alcohol is cancelled by bacitracin, Neurosci. Res., 2009, 64(2), 137–142 CrossRef PubMed.
- E. J. Shin, et al., Effects of Gastrodia elata Bl on phencyclidine-induced schizophrenia-like psychosis in mice, Curr. Neuropharmacol., 2011, 9(1), 247–250 CrossRef PubMed.
- Y. U. Lei, Y. S. Shen and H. C. Miao, Study on the Anti-vertigo Function of Polysaccharides of Gastrodia elata and Polysaccharides of Armillaria mellea, Chin. J. Inf. Tradit. Chin. Med., 2006, 8(13), 29–36 Search PubMed.
- C. S. Ding, et al., Study of a glycoprotein from Gastrodia elata: its effects of anticoagulation and antithrombosis, Zhongguo Zhongyao Zazhi, 2007, 32(11), 1060–1064 Search PubMed.
- T. Y. Jung, et al., Protective effects of several components of Gastrodia elata on lipid peroxidation in gerbil brain homogenates, Phytother. Res., 2007, 21(10), 960–964 CrossRef PubMed.
- O. H. Lee, et al., Effects of Acidic Polysaccharides from Gastrodia Rhizome on Systolic Blood Pressure and Serum Lipid Concentrations in Spontaneously Hypertensive Rats Fed a High-Fat Diet, Int. J. Mol. Sci., 2012, 13(1), 698–709 CrossRef PubMed.
- J. Y. Lee, et al., Anti-inflammatory action of phenolic compounds from Gastrodia elata root, Arch. Pharmacal Res., 2006, 29(10), 849–858 CrossRef.
- S. M. Hwang, et al., Anti-inflammatory effect of Gastrodia elata rhizome in human umbilical vein endothelial cells, Am. J. Chin. Med., 2009, 37(02), 395–406 CrossRef PubMed.
- Y. Hu, C. Li and W. Shen, Gastrodin alleviates memory deficits and reduces neuropathology in a mouse model of Alzheimer's disease, Neuropathology, 2014, 34(4), 370–377 Search PubMed.
- X. Tong, et al., WSS45, a sulfated α-D-glucan, strongly interferes with Dengue 2 virus infection in vitro, Acta Pharmacol. Sin., 2010, 31(5), 585–592 CrossRef PubMed.
- G. Shu, et al., Gastrodin stimulates anticancer immune response and represses transplanted H22 hepatic ascitic tumor cell growth: involvement of NF-κB signaling activation in CD4+ T cells, Toxicol. Appl. Pharmacol., 2013, 269(3), 270–279 CrossRef PubMed.
- W. Zhang, Y. X. Sheng and J. L. Zhang, Determination and pharmacokinetics of gastrodin and p-hydroxybenzylalcohol after oral administration of Gastrodia elata Bl. extract in rats by high-performance liquid chromatography-electrospray ionization mass spectrometric method, Phytomedicine, 2008, 15(10), 844–850 CrossRef PubMed.
- Chinese Pharmacopoeia Commission, Pharmacopoeia of the People's Republic of China, 2015, p. 58 Search PubMed.
- Y. J. Mo and D. Shi-Xian, Pharmacological study on Gastrodia elata Blume.2. effects of synthetic gastrodin and its genin on the heart and small intestine, Acta Bot. Yunnanica, 1980, 2(2), 230–234 Search PubMed.
- W. C. Chen, et al., Anti-depressant effects of Gastrodia elata Blume and its compounds gastrodin and 4-hydroxybenzyl alcohol, via the monoaminergic system and neuronal cytoskeletal remodeling, J. Ethnopharmacol., 2016, 182, 190–199 CrossRef PubMed.
- B. W. Kim, et al., Modulation of LPS-stimulated neuroinflammation in BV-2 microglia by Gastrodia elata: 4-hydroxybenzyl alcohol is the bioactive candidate, J. Ethnopharmacol., 2012, 139(2), 549–557 CrossRef PubMed.
- M. W. Laschke, et al., 4-Hydroxybenzyl alcohol: a novel inhibitor of tumor angiogenesis and growth, Life Sci., 2013, 93(1), 44–50 CrossRef PubMed.
- S. S. Yu, et al., Neuroprotective effect of 4-hydroxybenzyl alcohol against transient focal cerebral ischemia via anti-apoptosis in rats, Brain Res., 2010, 1308(3), 167–175 CrossRef PubMed.
- Q. L. Guo, et al., 4-Hydroxybenzyl-substituted glutathione derivatives from Gastrodia elata, J. Asian Nat. Prod. Res., 2015, 17(5), 439–454 CrossRef PubMed.
- R. Kodela, et al., Synthesis and biological activity of acetyl-protected hydroxybenzyl diethyl phosphates (EHBP) as potential chemotherapeutic agents, Bioorg. Med. Chem. Lett., 2011, 21(23), 7146–7150 CrossRef PubMed.
- S. Ahmed, et al., Synthesis and biochemical evaluation of a range of sulfonated derivatives of 4-hydroxybenzyl imidazole as highly potent inhibitors of rat testicular 17alpha-hydroxylase/17,20-lyase (P-450(17alpha)), Bioorg. Med. Chem. Lett., 2009, 19(16), 4698–4701 CrossRef PubMed.
- L. Zha, et al., Analysis of parameters of serum concentration and pharmacokinetic of liposome and aqueous solution of total ginsenoside of ginseng stems and leaves in rats, Zhongguo Zhongyao Zazhi, 2017, 1957–1963 Search PubMed.
- E. B. Cai, et al., The synthesis and evaluation of arctigenin amino acid ester derivatives, Chem. Pharm. Bull., 2016, 64(10), 1466–1473 CrossRef PubMed.
- OECD, Guidelines for the Testing of Chemicals/Section 4: Health Effects Test No. 423, Acute Oral Toxicity – Acute Toxic Class Method, Organisation for Economic Co-operation and Development, Paris, France, 2002 Search PubMed.
- Y. Öztürk, et al., Effects of Hypericum perforatum L. and Hypericum calycinum L. extracts on the central nervous system in mice, Phytomedicine, 1996, 3(2), 139–146 CrossRef.
- S. X. Deng and Y. Q. Mo, Pharmacological studies on Gastrodia elata Bluma.1. the sedative and anticonvulsant effects of synthetic gastrodin and its genin, Acta Bot. Yunnanica, 1979, 2(1), 66–73 Search PubMed.
- P. M. Fuller, J. J. Gooley and C. B. Saper, Neurobiology of the sleep-wake cycle: sleep architecture, circadian regulation, and regulatory feedback, J. Biol. Rhythms, 2006, 21(6), 482–493 CrossRef PubMed.
- M. Zhu, et al., Application of radioligand receptor binding assays in the search for CNS active principles from Chinese medicinal plants, J. Ethnopharmacol., 1996, 54(2–3), 153–164 CrossRef PubMed.
- R. Y. Moore and A. E. Halaris, Hippocampal innervation by serotonin neurons of the midbrain raphe in the rat, J. Comp. Neurol., 1975, 164(2), 171–183 CrossRef PubMed.
- D. M. Bannerman, et al., Regional dissociations within the hippocampus—memory and anxiety, Neurosci. Biobehav. Rev., 2004, 28(3), 273–283 CrossRef PubMed.
- E. Dale, et al., Effects of serotonin in the hippocampus: how SSRIs and multimodal antidepressants might regulate pyramidal cell function, CNS Spectr., 2016, 21(2), 143–161 CrossRef PubMed.
- M. A. Geyer and F. X. Vollenweider, Serotonin research: contributions to understanding psychoses, Trends Pharmacol. Sci., 2008, 29(9), 445–453 CrossRef PubMed.
- K. Herrickdavis, Functional Significance of Serotonin Receptor Dimerization, Exp. Brain Res., 2013, 230(4), 375–386 CrossRef PubMed.
- A. R. Green, Neuropharmacology of 5-hydroxytryptamine, Br. J. Pharmacol., 2006, 147(S1), S145–S152 CrossRef PubMed.
- A. A. Borbély and H. U. Neuhaus, Sleep-deprivation: effects on sleep and EEG in the rat, J. Comp. Physiol., 1979, 133(1), 71–87 CrossRef.
- R. G. Peñalva, et al., Effect of sleep and sleep deprivation on serotonergic neurotransmission in the hippocampus: a combined in vivo microdialysis/EEG study in rats, Eur. J. Neurosci., 2003, 17(9), 1896–1906 CrossRef.
- C. P. Lynn-Bullock, et al., The effect of oral 5-HTP administration on 5-HTP and 5-HT immunoreactivity in monoaminergic brain regions of rats, J. Chem. Neuroanat., 2004, 27(2), 129–138 CrossRef PubMed.
- A. N. Bateson, Further potential of the GABA receptor in the treatment of insomnia, Sleep Med., 2006, 7(1), S3–S9 CrossRef.
- J. Liu, et al., GABA and 5-HT systems are implicated in the anxiolytic-like effect of spinosin in mice, Pharmacol., Biochem. Behav., 2015, 128, 41–49 CrossRef PubMed.
- E. A. Barnard, et al., Molecular Biology of the GABAA Receptor, Adv. Exp. Med. Biol., 1988, 295–308 Search PubMed.
- R. W. Olsen and W. Sieghart, International Union of Pharmacology. LXX. Subtypes of gamma-aminobutyric acid(A) receptors: classification on the basis of subunit composition, pharmacology, and function. Update, Pharmacol. Rev., 2008, 60(3), 243–260 CrossRef PubMed.
- C. Belzung and G. Griebel, Measuring normal and pathological anxiety-like behaviour in mice: a review, Behav. Brain Res., 2001, 125(1–2), 141–149 CrossRef PubMed.
|
This journal is © The Royal Society of Chemistry 2018 |
Click here to see how this site uses Cookies. View our privacy policy here.