DOI:
10.1039/C8RA02018C
(Paper)
RSC Adv., 2018,
8, 23163-23170
An immunochromatographic dipstick as an alternate for monitoring of heroin metabolites in urine samples
Received
7th March 2018
, Accepted 10th June 2018
First published on 26th June 2018
Abstract
Heroin use and addiction pose serious risks and side effects due to overdose. Quantification of heroin in biological samples is challenging due to rapid deacetylation of heroin to its active metabolites. In this study, we report the quantification of metabolic degradation of heroin by-products in biological urine samples. The presence of the drug was monitored after oral administration of heroin at different time intervals. Various biophysical techniques, such as high performance liquid chromatography (HPLC) and mass spectrometry (MS) were used to evaluate the presence of the drug. A competitive fluorescence based immunoassay was developed with a limit of detection (LOD) up to 0.01 ng mL−1 and the IC50 value was 0.1 ng mL−1, while the dipstick assay shows a LOD up to 5 ng mL−1. Rapid detection of narcotic drugs was carried out for biological urine samples collected at various time points. Validation of the developed dipstick was carried out for the standard as well as the spiked urine samples by fluorescence based immunoassay (FIA), using anti-morphine antibodies. A strong correlation (R = 0.94) was obtained between the developed dipstick and FIA assay for biological urine samples collected at various time points. The developed immunochromatographic dipstick is highly sensitive, field applicable and cost effective, and can serve as a first choice for the monitoring of narcotic drugs in blood, urine and saliva in drug addicts and athletes.
Introduction
Heroin is a synthetic derivative obtained from morphine and extracted naturally from the seeds of poppy plants.1 Heroin experiences fast metabolism, and after ingestion, rapidly degrades into its constituent metabolites such as monoacetyl morphine (MAM), morphine and morphine glucouronide.2,3 Heroin has a short half-life of about 2–8 min and gets rapidly metabolized to MAM, which has a short half life of approximately 38 min and thus both are metabolically degraded in the blood.1,4,5 MAM is rapidly deacetylated to morphine with an average half-life of 20 min and thus can be detected in blood up to 1–2 h and in urine approximately up to 2–8 h.4 Unhindered heroin and morphine use has caused a substantial number of illness because of their easy accessibility as recreational drugs.6 These drug molecules are powerful groups of narcotic analgesics and possess a notable tendency for abuse in youngsters and athletes; therefore it is of prime importance to detect the presence of opiates in biological samples.1,6,7 Till date, many qualitative as well as quantitative techniques have been described for the detection of opiate drugs such as high-pressure liquid chromatography (HPLC), capillary electrophoresis (CE), thin-layer chromatography (TLC), gas chromatography (GC), and mass spectroscopy (MS).8–11 However, these techniques are time consuming, require many cleanup steps, and trained personnel, due to which immunosensor based methods have emerged as a better alternative as they have the potential to provide continuous data streams for the rapid and on-site detection of narcotic drugs.12 Specific binding between antibody and its antigen in immunosensors make them highly sensitive and specific and can thus be developed for the detection of various analytes such as pesticides, narcotic drugs, preeclampsia and cancer etc.13–23 Indirect competitive ELISA was done for the monitoring of papvarine in serum and injection samples with the limit of detection upto 0.25 and 0.06 ng mL−1.24 6-Monoacetyl morphine (MAM) screening was done in urine samples by cloned enzyme donor immunoassay upto 410–2010 μg mL−1.25 Fluorescence based immunoassays (FIA) have been developed for the detection and quantification of opiates with detection limit up to 0.01 ng mL−1.1 The anti-morphine antibody (Mor-Ab) was labeled with fluorescein isothiocyanate (FITC). Sensitive, specific, and robust nature of antibodies allow their use in various immunoassays based on dipstick, electrochemical, chemiluminescence and fluorescence for rapid detection and quantification of opiates.1,26–30 Signal amplification and increased sensitivity can be achieved with the use of nanomaterials. Gold nanoparticles (AuNPs) are useful as diagnostic tool due to their resonance properties.26 AuNPs provide scattering and oscillation of electrons in the conductive region.27,28 Functionalization of specific antibodies followed by their attachment onto AuNPs surface is governed by different molecular interactions such as covalent bond, electrostatic and hydrophobic bond. In this line, AuNPs based immunochromatographic kit was developed for chicken antibody (IgY) and single chain variable fragment (scFv) antibody for morphine detection. The Mor-Abs were labeled with AuNPs and IC50 values for MAM and morphine were around 13.3 ng mL−1 and 14.0 ng mL−1, respectively, with LOD upto 5 ng mL−1.26 Further enhancement in the sensitivity of immunoassay was achieved by single walled carbon nanotubes (SWCNT). Due to their exceptional charge transport characteristics, carbon nanotubes (CNTs) have immense importance in the area of biosensing. The developed SWCNT based real time field effect transistors (LG-FET) showed the LOD upto fg mL−1 for morphine.28
In present study, we report the metabolic degradation of heroin after oral administration in mice. For this purpose, hapten–protein conjugate (Mor-BSA) was used as antigen in immunochromatographic dipstick for rapid diagnosis of heroin and its metabolites in biological urine samples. The developed dipstick showed a good correlation of 0.94 with FIA for the urine samples collected at different time intervals. The obtained data was further validated by various biophysical and analytical techniques, such as HPLC, MS, ELISA and FIA. Our results confirmed that the developed dipstick, besides being cost effective and rapid, can be efficient for on-site monitoring of opiate drugs.
Experimental
Materials and methods
Standard samples of heroin and morphine were granted by CFSL (the Central Forensic Science Laboratory), Chandigarh, India. MAM, M-3-G, and bovine serum albumin (BSA) was acquired from Sigma Aldrich Co., Delhi, India. 3,3′,5,5′-Tetramethylbenzidine (TMB) was procured from Bangalore Genei, India Pvt. Ltd (BGIP). ELISA plates (polystyrene made) were purchased from NUNC (A/S), Denmark. HPLC-grade solvents included acetonitrile A and B (CH3CN), ethyl acetate (CH3COOC2H5) and methanol (CH3OH). Buffer preparation (ph 6.0 ± 0.1) was done using a blend of 0.1 mol L−1 sodium acetate (CH3COONa) and 0.1 mol L−1 acetic acid (CH3COOH) with 50 g L−1 sodium fluoride (NaF) solution. Thermo Fisher Scientific, phosphate buffered saline (PBS) was also employed as washing buffer for ELISA plates in competitive immunoassay. Sample pad, absorption pad, and dipstick plastic unit was obtained from Advance Microdevices, Ambala, India.
All other reagents, chemicals and solvents availed in the experimental study were of high purity and analytical grade.
Standard solution preparation
1 mg mL−1 of all the pool and test solutions of heroin, morphine and MAM were prepared and stored at −20 °C when not in use. The standard solutions were prepared within 30 min of use to overcome the hydrolysis of chemicals. For the stabilization of heroin, acetate buffer was added immediately to thawed samples. The resulting solution was vortex mixed for 10 s, centrifuged for 10 min at 10
000 rpm and filtered with 20 μm filters.
Experimental animal
In this study, three sets of ten-week old female BALB/c mice were administered orally with heroin. For oral administration through injection, 22 mg kg−1 heroin hydrochloride (C21H24ClNO5) was prepared in saline. Samples of urine were equipped in 10 mL polypropylene tubes consisting 20 mM sodium acetate buffer (pH = 6.0) to stabilize heroin. On completion of heroin administration, collection of urine was done at 0 h, 2 h, 4 h, 6 h, 8 h and 10 h respectively. After collection, two sets of (biological and spiked) urine samples were immediately transferred on ice and centrifuged for 10 min at 10
000 rpm at 4 °C. The urine was frozen in dry ice/methanol and stored at −20 °C, until required. The present study was completed in strict agreement with the guidelines of Control and Supervision of Experiments on Animals (CPCSEA) for the use of laboratory animals. The approval was provided by Animal Ethics Committee of Institute of Microbial Technology (CSIR-IMTECH), Chandigarh, India.
Extraction of sample
The extracted urine samples were used for TLC. Spots were air dried, cut and dissolved in chloroform
:
isopropyl alcohol (3
:
1). The upper aqueous layer was separated and the step was repeated twice. The organic solvent fractions of all three extraction steps were clubbed together. These clubbed fractions were then passed through anhydrous sodium sulphate to remove the traces of water. The organic solvent was then evaporated completely and precipitate was resuspended in 50 μL of methanol and filtered through 0.22 μm filters before being used for further analysis.
Competitive-immunoassay
The competitive immunoassay studies were conducted by three different methods, namely, enzyme-linked immunosorbent assay (ELISA), fluorescent immunoassay (FIA) and dipstick based immunoassay (DIA), as described below. The steps were common for coating, washing and serial dilution for ELISA and FIA but not for DIA. Coating was done with Mor-BSA (1 μg mL−1) in carbonate buffer (20 mM, pH – 9.6) and incubated O/N at 4 °C. Washing was done with 1× PBST (0.05% Tween-20) for two times and 1× PBS (one time), after each incubation. Serial dilution of the spiked urine was prepared in PBS in the range of 1 pg mL−1 to 1 μg mL−1. Further methodology involved in DIA, ELISA, and FIA is described as follows:
Dipstick based immunoassay
An optical immunochromatographic dipstick kit was developed for the detection of narcotic drugs in urine samples collected at different time points, such as 0 h, 2 h, 4 h, 6 h and 8 h respectively. Preparation and characterization of Mor-Au-immunoprobe was done as described previously.6,23 The dipstick comprises of two pads, application and absorption pad, and the test line, made on nitrocellulose membrane (2 μL per line) accompanying conjugate of MAM–BSA, Mor–BSA and M-3-G-BSA (2.0 mg mL−1 in 3% methanol) and allocated by Easy Printer (Advance Microdevices, Ambala, India). Addition of samples was done on glass fabricated Mor-Ab-AuNPs immunoprobe at 1
:
200 dilution. Thereafter, nitrocellulose (NC) strip (absorption pad, sample pad and the NC membrane) was dried for 2 h in laminar and lodged into a cassette of plastic that was further employed as a dipstick. Various samples of urine (50 μL) were individually spiked with heroin, MAM and morphine. In other set of dipstick, biological samples collected at different time intervals, were added to sample well (50 μL per well). Competitive reaction occurred between opiate drugs and Mor-Ab-AuNPs immunoprobe. The resultant color showed an inverse relation with the quantity of analyte present in the sample via lateral flow in dipstick. For the quantification of results, a digital gel documentation system (CamiImager™ Ready, Alpha Innotech Corporation, USA) was employed for accessing the optical signals.
Enzyme-linked immunosorbent assay (ELISA)
Monoacetyl morphine–bovine serum albumin (MAM–BSA), morphine–bovine serum albumin (Mor–BSA) and morphine-3-glucouronide–bovine serum albumin (M3G–BSA) were coated in carbonate buffer (20 mM, pH – 9.6) and incubated O/N at 4 °C. Blocking was done to block non-specific binding sites with 5% defatted skimmed milk prepared in phosphate buffered saline (PBS, 200 μL per well) for 2 h at 37 °C. Spiked samples were prepared in urine with standard drugs (heroin, MAM, morphine and M-3-G) in the linear range of 1 pg mL−1 to 1 μg mL−1. Urine samples (100 μL each) collected at different time points, mixed with (0.1 μg mL−1) Mor-Ab and 100 μL was added to each well. Plates were incubated for 2 h, at 37 °C. 100 μL per well goat anti-rabbit-IgG-HRP (Biorad) was added as 2° antibody, at a dilution of 1
:
10
000 and 1 h incubation was done at 37 °C. 100 μL per well tetramethylbenzidine (TMB) substrate was added and incubated for 15 min at room temperature (RT). The reaction was stopped with 1 N H2SO4 (50 μL per well). The absorbance was measured at the wavelength of 450 nm by ELISA plate reader (Biotek- XS Plus) (Fig. 2). All washing steps were done twice in 1× PBS (pH 7.4) + 0.05% Tween-20 and once in 1× PBS (pH 7.4).
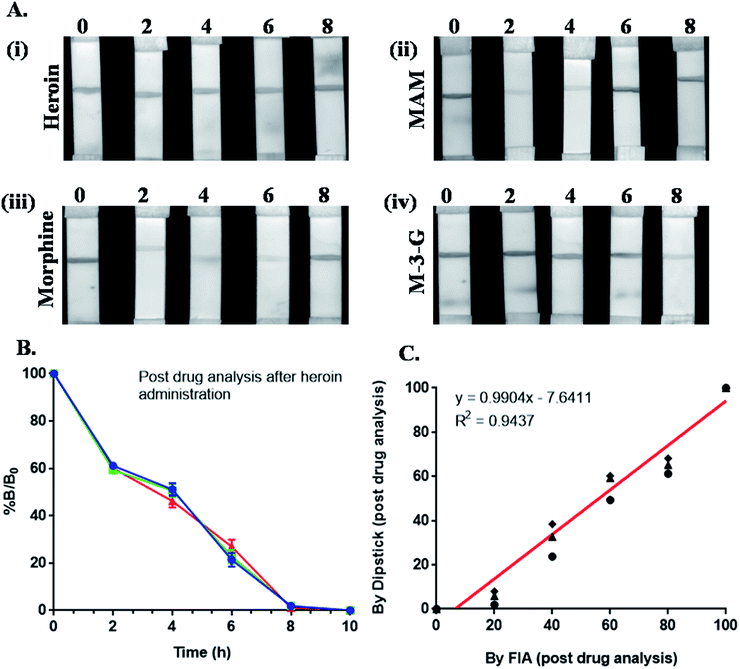 |
| Fig. 1 (A) Dipstick assay of biological urine samples collected at different time points (0 h, 2 h, 4 h, 6 h and 8 h); (B) % B/B0 graph of post heroin administration in mice at different time points; (C) standard graph showing a strong correlation of 0.94 between dipstick and FIA assay post heroin administration. | |
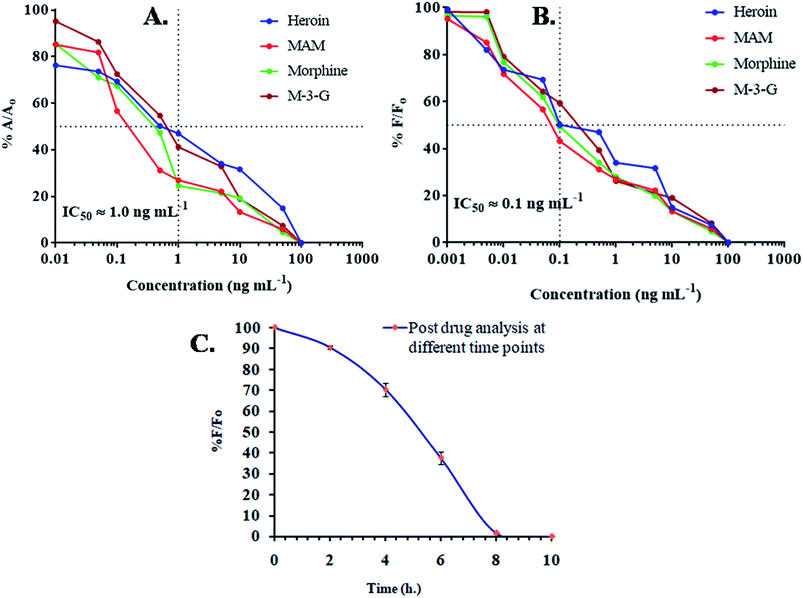 |
| Fig. 2 (A) competitive ELISA; (B) fluoroimmunoassay (FIA) of spiked urine samples; (C) competitive inhibition assay (FIA) of biological urine samples collected at different time points (0 h, 2 h, 4 h, 6 h, 8 h and 10 h) using (MAM–BSA), (Mor–BSA) and (M3G–BSA) conjugates as a coating antigen (0.1 μg mL−1); the buffer used for coating was carbonate buffer (20 mM, pH 9.6). | |
Fluoroimmunoassay (FIA)
ELISA plates were coated as described earlier. Spiked and biological samples were also prepared similarly and mixed with Mor-Ab-FITC solution (1
:
1000 in PBS) in equal volume. Preparation of standard drug solutions was done in PBS in linear range from 1 pg mL−1 to 1 μg mL−1 followed by plate incubation for 2 h at 37 °C. The counts were measured directly by fluorescence microtiter plate reader (Molecular Devices, USA) at excitation and emission wavelengths of 492 nm and 515 nm respectively.
High performance liquid chromatography
High performance liquid chromatography (HPLC) consisted of Shimadzu LC-20AD binary pump (Shimadzu Corporation, Kyoto, Japan). 20 μL sample was injected in an injector (SIL-10 A/10AVP) with a microsyringe (SIL-10ADvp) to Luna 5 μm C18 column (CTO-10AS; 250 mm × 4.60 mm, particle size 5 μm). UV-absorbance was detected at 214 nm with UV-Vis detector (SPD-10A/10Avp) (Fig. 4). Gradient based elution was initiated using a mixture of (5 mM) ammonium acetate in water (pH 4.0) and acetonitrile A and B, (for 2 min 95% A and 5% B, 2–8 min 85% A and 15% B, 8–11 min 20% A and 80% B, 11–15 min 95% A and 5% B). Rate of flow of the mobile phase was 1 mL min−1 and 15 min was the total run time. The degree of column's heat was 25 °C. Subsequently, the microsyringe was rinsed with 100% methanol after every injection. Standard drugs (heroin, MAM, morphine and M-3-G) were run before application of unknown sample.
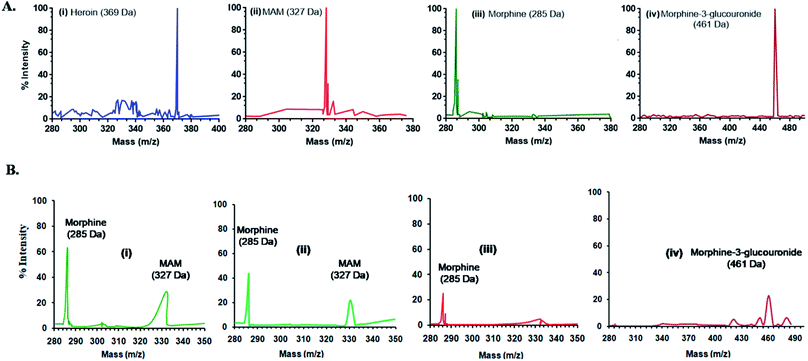 |
| Fig. 3 (A) Standard mass spectra (MS) of heroin, MAM, morphine and M-3-G (left to right) and (B) mass spectra of biological urine samples collected post 2 h, 4 h, 6 h and 8 h of heroin administration in the subject (mice) (left to right). | |
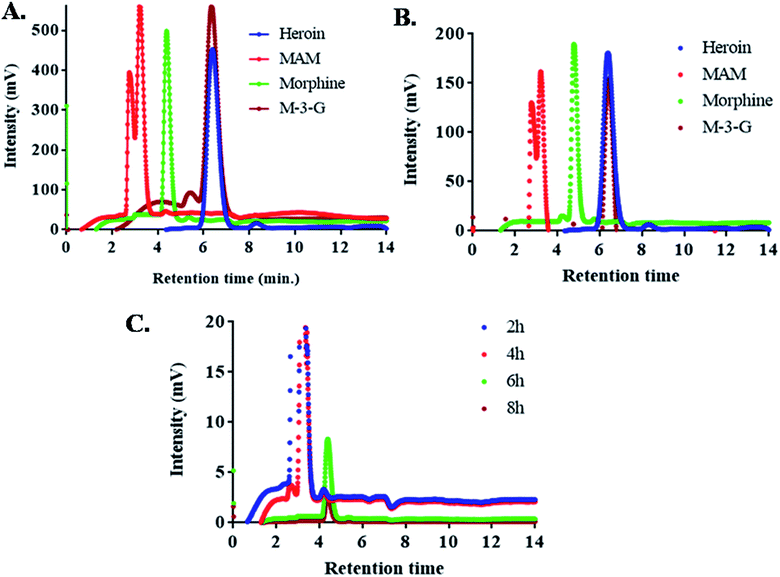 |
| Fig. 4 (A and B) Typical chromatogram of heroin, MAM, morphine and M-3-G in standard buffer and spiked urine. The X-axis shows the retention time (RT) and the Y-axis shows the intensity of the signal in milli volt (mV). Each of the individual chromatogram peaks represents separation of individual compounds from the sample mixture; (C) chromatogram of urine samples collected after 2 h, 4 h, 6 h and 8 h respectively. | |
Mass spectra
The mass ion defect of all quantitative ions (base peak) for standards and tests was determined with an unextracted standard and extracted urine samples, at different time points. For mass spectrometry (MS), two sets of urine samples i.e. spiked and biological were used in methanol. The Voyager-DESTR mass spectrometer was run in ion selective mode. The monitoring was performed for all the standards such as heroin, MAM, morphine and morphine-3-glucouronide (M-3-G) as well as for the test samples at varied time points (0 h, 2 h, 4 h, 6 h and 8 h) respectively and mixed in solution of sinapinic acid (15 mg mL−1 in CH3CN) in equimolar amounts. Following the application of samples onto a probe of stainless steel having 0.5 μL of 0.1% TFA, the samples were dried at ambient temperature, and transferred to analyzer for mass analysis. Then values were achieved with 50 shots per sample at 30 kV and analyzed via system linked software.
Results and discussion
Degradation of heroin post oral administration
Table of content highlights the degradation pathway of heroin after oral administration, resulting into generation of MAM to morphine, and then to morphine-3-glucouronide (M-3-G) and morphine-6-glucouronide (M-6-G). The presence of three active sites (C3, C4 and N17) plays a major role in deacetylation of heroin. To minimize the process of deacetylation, the extraction drugs were done immediately after sample collection. Consequently, the standards were chosen (heroin, MAM, morphine and M-3-G) on the basis of degradation pathway for recognition of unknown compounds released after degradation of heroin. Urine samples were collected after 0 h, 2 h, 4 h, 6 h and 8 h respectively. Presence of narcotic drugs was analyzed by dipstick immunoassay. Further validation of the obtained data was done by ELISA, FIA, HPLC and MS.
Dipstick based immunoassay
The presence of heroin and its major metabolites were detected via efficient immunochromatographic assay using Mor-Abs-AuNPs as an immunoprobe (Fig. 1). A series of blocking agents were used previously, including polyvinyl alcohol (PVA), polyethylene glycol (PEG), polyvinyl pyrrolidone (PVP), skimmed milk as well as bovine serum albumin (BSA) but they were associated with drooping effect on the strip due to its cloggy property and affects the tangential capillary action. Therefore, blocking was not carried out. Drug detection was mediated via competitive reaction between the test line, i.e., MAM–BSA, morphine–BSA and M-3-G–BSA conjugate and the target analyte. Linear range of concentration (1 to 10
000 ng mL−1) was prepared for heroin, morphine, MAM and M-3-G and LOD was 5 ng mL−1 and IC50 was approx 20 ng mL−1. Urine samples were added (collected at different time points) directly on sample pad to analyze, if the drug was present or absent. A strong correlation of 0.94 between dipstick and FIA assay for morphine in biological urine samples was noted. The optical signal for the presence of heroin was obtained at 2 and 4 h for MAM and morphine, 6 h for morphine, 8 h for M-3-G. This adds to the fact that Mor-Abs can be successfully used for rapid and on-site detection of opiates in biological samples.
Table 1 Comparison of sensitivity and specificity of various morphine specific immunoassays in spiked urine samples
S. No. |
Type of immunoassay |
Linear range (μg mL−1) |
LOD (ng mL−1) |
IC50 (ng mL−1) |
1 |
Dipstick |
1–10 |
5 |
20 |
2 |
ELISA |
0.01–1 |
0.1 |
1.0 |
3 |
FIA |
0.01–1 |
0.01 |
0.1 |
The intensity of the signal (I) was normalized as % B/B0 that represents the following normalization equation:
% B/B0 = I − Iex/I0 − Iex × 100 |
where,
I is the signal measured for a certain analyte concentration,
I0 is signal recorded for the blank/zero standard, and
Iex is signal at the excess of certain analyte.
Enzyme immunoassay
Using the same samples, the obtained data was further validated by ELISA. ELISA based IC50 value was 1.0 ng mL−1 and in dipstick assay, up to 20 ng mL−1 (Fig. 2A). The sensitivity difference can be neglected, as the obtained values differ due to different matrix effects. IC50 or critical inhibition values (50% A/A0) were achieved by enzyme-linked immunoassay and incorporation of given equation
%A/A0 = A − Aex/A0 − Aex × 100 |
where A, A0 and Aex signify sample absorbance, hapten at zero concentration, and hapten at excess concentration, respectively.
Fluorescence immunoassay
Further enhancement in sensitivity was achieved by FIA. Mor-Ab was labeled with FITC. IC50 values were achieved by competitive inhibition assay and normalization of fluorescence intensity and incorporation of given equation
% F/F0 = F − Fex/F0 − Fex × 100 |
where F, F0 and Fex signify sample fluorescence intensities, hapten at zero concentration, and hapten at excess concentration, respectively.
On the basis of a semi-log plot, a standard arc for indirect type FIA was obtained using standard samples in the linear range of 0.01 pg mL−1 and 1000 ng mL−1. Heroin and its metabolites showed LOD upto 0.01 ng mL−1. It was seen that the designed immunoassay for fluorescence, incorporating anti-morphine-Ab, not only possessed wide specificity but was highly sensitive towards heroin, MAM, morphine and M-3-G. Unlike current known chromatographic techniques, the developed assay proved to be far more sensitive, as the LOD in the prior for heroin was 1 ng mL−1, 5 ng mL−1 for MAM and morphine, and 9.7 ng mL−1 for M-3-G (Fig. 2B). A comparative Table 1 showed the linear range, limit of detection, and IC50 of the different assays developed in spiked urine samples.
Mass spectrometry and high performance liquid chromatography
Fig. 3 and 4 shows the MS spectra and HPLC chromatogram where the mass and purity was analyzed with standard samples. Further urine samples were collected at different time points 2, 4, 6 and 8 h to observe the degradation pathway of heroin and its major metabolites. At 2 and 4 h, two peaks of MAM and morphine were found in urine samples. While at 6 h, major concentration of morphine was present and at 8 h, M-3-G traces were found that further proves the degradation pathway in table of contents.
HPLC chromatogram showed the degradation pattern in a similar manner as shown by Mass spectra. Fig. 4A showed the spiked samples chromatogram in buffer, while urine samples were spiked with heroin, MAM, morphine and M-3-G (Fig. 4B). Fig. 4C showed the peaks observed after 2, 4, 6, and 8 h of urine sample collected after heroin administration in mice. Mass spectra, HPLC data are in close agreement with dipstick based lateral flow immunoassay. It further validated our results that the color developed in dipstick is in accordance with MS and HPLC at 2 and 4 h (MAM and morphine), 6 h (morphine), and 8 h (M-3-G) in biological sample.
Conclusion
This work reported the development of various immunoanalytical approaches to trace the metabolic degradation of heroin post administration. Biological urine samples were analyzed by dipstick and further validated by ELISA, FIA, HPLC and MS. The sensitivity of each immunoassay was compared. The highest LOD was obtained in FIA, up to 0.01 ng mL−1. The main drawback in FIA is quenching that can be overcome by dipstick. Dipstick based immunoassay provides cost effective, rapid and optical detection, but again sensitivity comes as an issue. Therefore, the developed immunoassays can be used, according to the person requirement for the monitoring of opiate drugs in biological urine samples.
Conflicts of interest
There are no conflicts to declare.
Acknowledgements
The authors are grateful for the funding support provided by Central Forensic Science Laboratory (CFSL), Chandigarh, India. We also acknowledge Institute of Microbial Technology (CSIR-IMTECH), Chandigarh, India for providing the research and animal facility. We greatly acknowledge the research grant DST/ECR/2016/000075 from Department of Science and Technology-Science and Engineering Research Board (DST-SERB), New Delhi, India for providing support to carry out the research work.
References
- S. Gandhi, P. Sharma, N. Capalash, R. S. Verma and C. R. Suri, Anal. Bioanal. Chem., 2008, 392, 215–222 CrossRef PubMed.
- A. Maas, B. Madea and C. Hess, Drug Test. Anal., 2018, 10, 54–71 CrossRef PubMed.
- L. G. Franken, A. D. Masman, B. C. M. de Winter, B. C. P. Koch, F. P. M. Baar, D. Tibboel, T. V. Gelder and R. A. A. Mathot, Clin. Pharmacokinet., 2016, 55, 697–709 CrossRef PubMed.
- A. Maas, M. Krämer, K. Sydow, P. S. Chen, T. Dame, F. Musshoff, B. W. K. Diehl, B. Madea and C. Hess, Drug Test. Anal., 2017, 9, 470–478 CrossRef PubMed.
- K. M. Rentsch, G. A. Kullak-Ublick, C. Reichel, P. J. Meier and K. Fattinger, Clin. Pharmacol. Ther., 2001, 70, 237–246 CrossRef PubMed.
- S. Gandhi, N. Caplash, P. Sharma and C. R. Suri, Biosens. Bioelectron., 2009, 25, 502–505 CrossRef PubMed.
- G. Das, J. Clin. Pharmacol., 1993, 33, 296–310 CrossRef PubMed.
- M. T. Contreras, A. F. Hernandez, M. Gonzalez, S. Gonzalez, R. Ventura, A. Pla, J. L. Valverde, J. Segura and R. Torre, Forensic Sci. Int., 2006, 164, 168–171 CrossRef PubMed.
- M. R. Hutchinson and A. A. Somogyi, Eur. J. Pharmacol., 2002, 453, 27–32 CrossRef PubMed.
- J. Segura, R. Ventura and C. Jurado, J. Chromatogr. B: Biomed. Sci. Appl., 1998, 713, 61–90 CrossRef.
- F. Tames, I. D. Watson, W. Morden and I. D. Wilson, J. Chromatogr. B., 1999, 729, 341–346 CrossRef.
- S. Carreiro, D. Smelson, M. Ranney, K. J. Horvath, R. W. Picard, E. D. Boudreaux, R. Hayes and E. W. Boyer, J. Med. Toxicol., 2015, 11, 73–79 CrossRef PubMed.
- A. P. Turner, Chem. Soc. Rev., 2013, 42, 3184–3196 RSC.
- P. Sharma, S. Gandhi, A. Chopra, N. Sekar and C. R. Suri, Anal. Chim. Acta, 2010, 676, 87–92 CrossRef PubMed.
- C. R. Suri, R. Boro, Y. Nangia, S. Gandhi, P. Sharma, N. Wangoo, R. Kumar and G. S. Shekhawat, Trends Anal. Chem., 2009, 28, 29–39 CrossRef.
- C. R. Suri, J. Kaur, S. Gandhi and G. S. Shekhawat, J. Nanotechnol., 2008, 19, 235502 CrossRef PubMed.
- I. P. M. Wijaya, S. Gandhi, J. N. Tey, N. Wangoo, G. S. Shekhawat, C. R. Suri, I. Rodriguez and S. G. Mhaisalkar, Appl. Phys. Lett., 2009, 95, 073704 CrossRef.
- I. P. M. Wijaya, T. J. Nie, S. Gandhi, R. Boro, A. Palaniappan, G. W. Hau, I. Rodriguez, C. R. Suri and S. G. Mhaisalkar, Lab Chip, 2010, 10, 634–638 RSC.
- S. Thakur, S. Gandhi, A. K. Paul and C. R. Suri, Sens. Transducers J., 2011, 131, 91–100 Search PubMed.
- S. Gandhi, P. Sharma, N. Capalash and C. R. Suri, BioImpacts, 2015, 5, 207–213 CrossRef PubMed.
- P. Suman, S. Gandhi, P. Kumar and K. Garg, Am. J. Reprod. Immunol., 2017, 77, 1–10 CrossRef PubMed.
- S. Gandhi, H. Arami and K. M. Krishnan, Nano Lett., 2016, 16, 3668–3674 CrossRef PubMed.
- S. Eissa and M. Zourob, Microchim. Acta, 2017, 184, 2281–2289 CrossRef.
- J. Yan, J. Mi, J. He, Z. Guo, M. Zhao and W. Chang, Talanta, 2005, 66, 1005–1011 CrossRef PubMed.
- C. George, S. George and S. Parmar, J. Anal. Toxicol., 2002, 26, 233–235 CrossRef PubMed.
- S. Gandhi, I. Banga, P. K. Maurya and S. A. Eremin, RSC Adv., 2018, 8, 1511–1518 RSC.
- S. Gandhi, A. Talan, A. Mishra, S. A. Eremin, J. Narang and A. Kumar, Biosens. Bioelectron., 2018, 105, 14–21 CrossRef PubMed.
- J. N. Tey, S. Gandhi, I. P. M. Wijaya, A. Palaniappan, J. Wei, I. Rodriguez, C. R. Suri and S. G. Mhaisalkar, Small, 2010, 6, 993–998 CrossRef PubMed.
- X. H. Qi, J. Q. Mi, X. X. Zhang and W. B. Chang, Electrochem. Commun., 2005, 7, 227–232 CrossRef.
- S. Singh, P. Mishra, I. Banga, A. S. Parmar, P. P. Tripathi and S. Gandhi, BioImpacts, 2018, 8, 57–62 Search PubMed.
|
This journal is © The Royal Society of Chemistry 2018 |
Click here to see how this site uses Cookies. View our privacy policy here.