DOI:
10.1039/C8RA02572J
(Paper)
RSC Adv., 2018,
8, 22313-22320
Synthesis and characterization of amino glucose-functionalized silica-coated NiFe2O4 nanoparticles: a heterogeneous, new and magnetically separable catalyst for the solvent-free synthesis of pyrano[3,2-c]chromen-5(4H)-ones
Received
26th March 2018
, Accepted 20th May 2018
First published on 19th June 2018
Abstract
A novel, efficient and one-pot multi-component procedure for the synthesis of simple pyrano[3,2-c]chromen-5(4H)-ones or pyrazolyl pyrano[3,2-c]chromen-5(4H)-ones via reaction of aryl aldehydes, acetophenones and 4-hydroxycoumarin promoted by amino glucose-functionalized silica-coated NiFe2O4 nanoparticles under solvent-free conditions without using any other harmful organic reagents was reported. The structure of this nanoparticle was characterized by transmission electron microscopies, X-ray diffraction and Fourier transform infrared spectroscopies. The catalyst could easily be separated from the reaction mixture by using an external magnetic field and it was reusable. The high purity of the desired products, eco-friendliness, short reaction time and easy workup procedure can be mentioned as the other advantages of this method.
Introduction
Pyranochromenes are a class of important heterocycles with a wide range of pharmaceutical and biological properties such as anti-coagulant, spasmolytic, analgesic, myorelaxant, anti-cancer, cytotoxic, anti-HIV, anti-microbial, anti-tuberculosis, diuretic and anti-anaphylactic activity.1–9 Moreover, they can be used as cognitive enhancers for the treatment of neurodegenerative diseases, including Huntington's disease, schizophrenia and myoclonus disease, amyotrophic lateral sclerosis, Alzheimer's disease, Parkinson's disease, AIDS associated dementia and Down's syndrome.1 They can act also as additives in perfumes, food, cosmetics, optical brighteners, dispersed fluorescence and laser dyes.10,11 So far, only a few methods have been reported for the synthesis of pyrano[3,2-c]chromen-5(4H)-ones.12–15
Multicomponent reactions (MCRs) are one of the most efficient tools in modern synthetic organic chemistry. They have advantages such as being fast, simple and easy to implement, with high atom efficiency. They offer a model for target- and diversity-oriented synthesis.16–20
Transition-metal nanoparticles are materials: containing a few tens to several thousand metal atoms, which are stabilized by ligands, polymers or dendrimers as protecting agents for their surfaces. They are heterogeneous catalysis that benefits from easy removal of catalyst materials and possible use at high temperatures, suffered for a long time from lack of selectivity and understanding of the mechanistic aspects that are indispensable in parameter improvements.21 Amino glucose-functionalized silica-coated NiFe2O4 nanoparticles have attracted a lot of chemists because of its unique properties such as chemical inertness, easy synthesis and functionalization, low toxicity and low cost, high surface area, small crystallite size, superior controlled selectivity, more active sites and facile removing with aid of external magnet from the reaction mixture.
Results and discussion
The multicomponent reaction of benzaldehydes, acetophenones and 4-hydroxycoumarin, simple in nature, is essentially a condensation reaction. The reaction can be assisted by activating the carbonyl group of aldehydes with an acidic species or by improving the nucleophilic property of 4-hydroxycoumarin in basic media. In continuation of our research for the green synthesis of heterocyclic compounds,22–29 herein we describe the synthesis of pyrano[3,2-c]chromen-5(4H)-ones using NiFe2O4@SiO2@amino glucose magnetic nanoparticle as a new catalyst (Scheme 1).
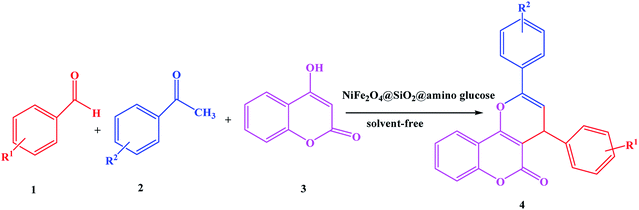 |
| Scheme 1 Multicomponent synthesis of pyrano[3,2-c]chromen-5(4H)-ones using NiFe2O4@SiO2@amino glucose. | |
As shown in Scheme 2, the NiFe2O4@SiO2@amino glucose magnetic nanoparticle was synthesized in three steps from commercially available materials. MNPs were coated by silica using a sol–gel process. The NiFe2O4@SiO2 core–shell structures were then sequentially treated with 3-chloropropyltrimethoxysilane. Next, it was treated with aminoglucose to obtain the amino glucose-functionalized silica-coated NiFe2O4 nanoparticles.
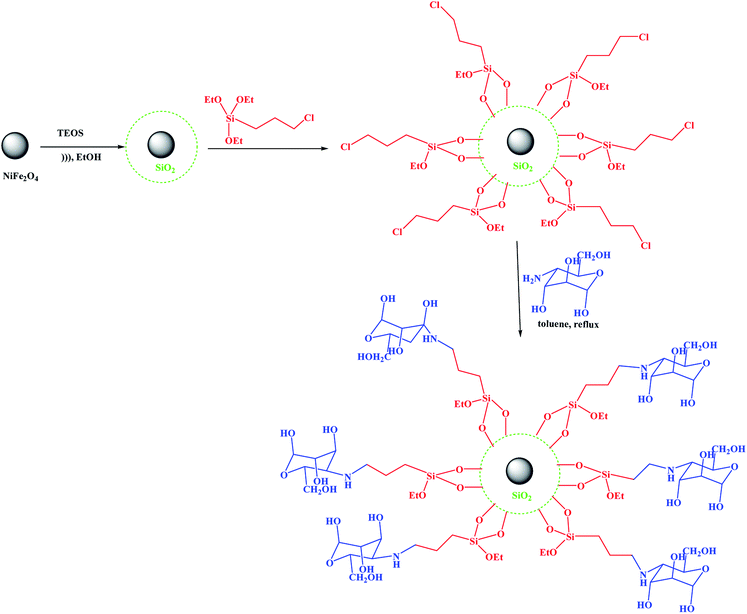 |
| Scheme 2 Stepwise synthesis pathway of NiFe2O4@SiO2@amino glucose. | |
In the FT-IR spectrum of the NiFe2O4@SiO2@amino glucose magnetic nanoparticle (Fig. 1), the absorption band of Fe–O was appeared in 590 cm−1, the absorption band of Si–O–Si in SiO2 shell were appeared in 1088, 912 and 512 cm−1. The peaks in region 2930, 3120 and 3415 cm− 1 refer to the stretching band of C–H aliphatic, OH stretching and NH stretching, in order.
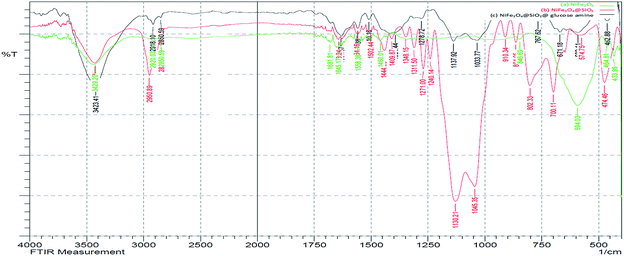 |
| Fig. 1 FT-IR spectra of (a) NiFe2O4, (b) NiFe2O4@SiO2, (c) NiFe2O4@SiO2@glucose amine. | |
The structure of NiFe2O4@SiO2@glucose amine was also analyzed by XRD analysis. In Fig. 2 the XRD patterns of NiFe2O4@SiO2@glucose amine and pure NiFe2O4 are illustrated. The comparison of the XRD patterns indicated that both patterns exhibits peaks at 30°, 36°, 45°, 50°, 54°, 58° and 62° which are representative of HNTs structure (JCPDS no. 54-0964) and broad peak in 10–30° is related to NiFe2O4 covered by SiO2.
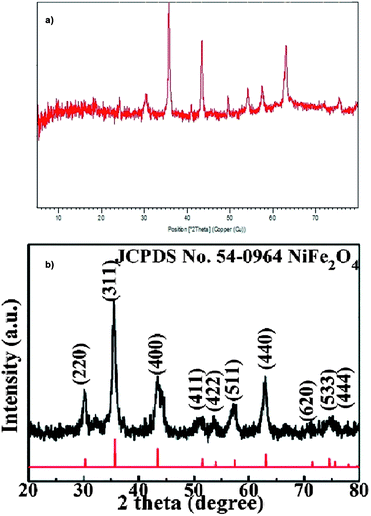 |
| Fig. 2 The XRD of (a) NiFe2O4@SiO2@glucose amine and (b) NiFe2O4. | |
Next, the morphology and nanoparticle size of the synthesized magnetic catalyst was characterized by Transmission Electron Microscope (TEM) (Fig. 3). As shown in Fig. 2, the catalyst particles possess near spherical morphology with average diameter of about 20–40 nm. Furthermore, TEM images show some aggregation, which was illustrated the successful grafting of the polymer on to magnetic nanoparticles.
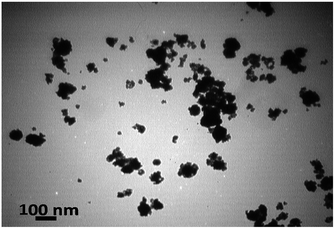 |
| Fig. 3 The TEM image of synthesized NiFe2O4@SiO2@glucose amine. | |
The most common method to determine concentration of acidic sites is temperature programmed desorption (TPD) of a basic probe molecule, mostly ammonia. For this test, the sample were pretreated at 573 K for 2 h. Afterwards, ammonia was adsorbed onto the surface of the samples at 393 K. The total amount of desorbed ammonia was determined by reaction with a diluted solution of sulphuric acid and titration with sodium hydroxide. The results are shown in Table 1. It can be seen that the numbers of acidic sites increase from NiFe2O4 and NiFe2O4@SiO2 to NiFe2O4@SiO2@glucose amine.
Table 1 Concentration of acidic sites determined by the method NH3-TPD
Catalyst |
NiFe2O4 |
NiFe2O4@SiO2 |
NiFe2O4@SiO2@glucose amine |
Acid surface sites (μmol g−1) |
180 |
315 |
1450 |
In order to evaluate the catalytic capability of the synthesized heterogeneous catalyst (NiFe2O4@SiO2@glucose amine) in organic reactions, we chose to examine its activity in a one-pot reaction for the synthesis of pyrano[3,2-c]chromen-5(4H)-ones.
Initially, we observed that the reaction of 4-nitrobenzaldehyde with 4-hydroxycoumarin and acetophenone in NiFe2O4@SiO2@glucose amine could be a suitable choice for the synthesis of pyrano – chromene 4b. This protocol proceeds smoothly at room temperature to afford product 4b in fairly high yield. We have also carried out the sample reaction in the NiFe2O4, NiFe2O4@SiO2, SiO2 or in the absence of catalyst. On the other hand, variables affecting on the reaction yields such as the type of solvent, the amount of catalyst, different temperatures, and solvent-free conditions were studied (Table 2). As shown in Table 2, the reaction under solvent-free conditions is more efficient.
Table 2 Optimization of reaction condition
Entry |
Catalyst |
Catalyst amount |
Reaction condition |
Time (min) |
Yield (%) |
1 |
— |
— |
Reflux in EtOH |
720 |
Trace |
2 |
SiO2 |
0.05 g |
Stir, rt, EtOH |
750 |
15 |
3 |
NiFe2O4 |
0.05 g |
Stir, rt, EtOH |
720 |
24 |
4 |
NiFe2O4@SiO2 |
0.05 g |
Stir, rt, EtOH |
300 |
53 |
5 |
NiFe2O4@SiO2@glucose amine |
0.05 g |
Stir, rt, EtOH |
40 |
90 |
6 |
NiFe2O4@SiO2@glucose amine |
0.07 g |
Stir, rt, EtOH |
40 |
87 |
7 |
NiFe2O4@SiO2@glucose amine |
0.03 g |
Stir, rt, EtOH |
60 |
85 |
8 |
NiFe2O4@SiO2@glucose amine |
0.05 g |
Stir, rt, CH3CN |
60 |
76 |
9 |
NiFe2O4@SiO2@glucose amine |
0.05 g |
Stir, rt, H2O |
45 |
84 |
10 |
NiFe2O4@SiO2@glucose amine |
0.05 g |
Solvent free, rt |
25 |
96 |
11 |
NiFe2O4@SiO2@glucose amine |
0.05 g |
Solvent free, 80 °C |
25 |
94 |
To check the generality of this method, different derivatives of benzaldehydes and acetophenones were subjected to the reaction with 4-hydroxycoumarin. The results are summarized in Table 3.
Table 3 Synthesis of pyrano[3,2-c]chromen-5(4H)-ones using NiFe2O4@SiO2@glucose amine
Entry |
Benzaldehyde |
Acetophenones |
Product |
Time (min) |
Yield (%) |
Mp (°C) (Lit mp) |
Ref. |
All the isolated products were characterized on the basis of their elemental analyses, physical properties and IR, 1H, 13C NMR spectral analysis, HR-Ms or by direct comparison with authentic materials. Isolated yields. |
1 |
3-Nitro |
Acetophenone |
4a |
10 |
95 |
123–125 (122–123) |
14 |
2 |
4-Nitro |
Acetophenone |
4b |
10 |
98 |
228–230 (230–231) |
13 and 15 |
3 |
4-Hydroxy |
Acetophenone |
4c |
15 |
93 |
167–169 |
This work |
4 |
Benzaldehyde |
Acetophenone |
4d |
12 |
95 |
166–168 (170–171) |
12–15 and 30 |
5 |
4-Methoxy |
Acetophenone |
4e |
15 |
90 |
143–144 (143–144) |
12a,b and 30 |
6 |
4-Chloro |
4-Chloro |
4f |
10 |
94 |
238–240 (239–241) |
12 and 15 |
7 |
4-Chloro |
3-Nitro |
4g |
10 |
95 |
189–190 |
This work |
8 |
4-Methyl |
3-Nitro |
4h |
15 |
93 |
152–154 |
This work |
9 |
4-Chloro |
Acetophenone |
4i |
10 |
97 |
176–178 (176–177) |
13 and 14 |
10 |
Benzaldehyde |
4-Chloro |
4j |
10 |
96 |
145–147 (149–151) |
12 and 14 |
All of the synthesized compounds were characterized by IR, NMR, HR-Ms, elemental analysis and by comparison with authentic samples for known compounds.
In continuation of our study, we triggered to synthesize a new category of pyrazolylpyrano chromenes 4k–m and bis pyranochromene 4n as shown in Scheme 3.
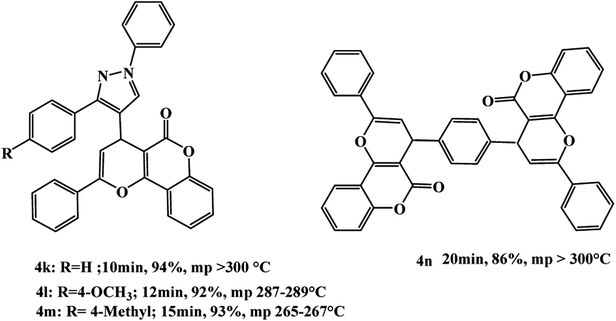 |
| Scheme 3 Synthesis of pyrazolyl and bis pyrano[3,2-c]chromenes. | |
A plausible mechanism for the formation of pyranochromene 4 is shown in Scheme 4. Initially, NiFe2O4@SiO2@glucose amine can increase the electrophilic character of the carbonyl species by virtue of its inherent Brønsted acidity. Nucleophilic addition of the enolic form of the ketone and subsequent dehydration can lead to chalcone 6. Then, Michael addition of 3 to 6 to produce intermediate 8, followed by simple condensation of the hydroxyl group with the carbonyl and dehydration, forms product 4.
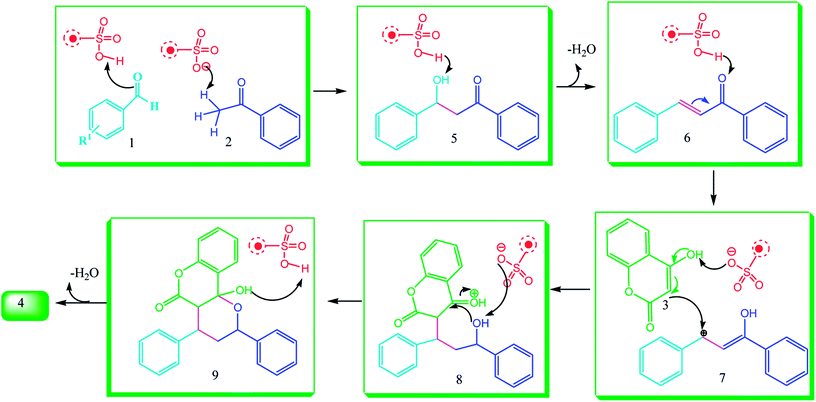 |
| Scheme 4 Proposed mechanism for the synthesis of pyranochromene 4. | |
We compared the efficiency of this method with recently reported methods (Table 4).
Table 4 Comparison of present method for the synthesis of 4d with some previous methods
Entry |
Condition |
Time (min) |
Yield (%) |
Ref. |
This pyranochromene was synthesized in a two step protocol, synthesis of chalcone followed by reaction with 4-hydroxycoumarin. |
1 |
MWI, 60 °C |
15 |
92 |
30 |
2 |
I2, AcOH, 100 °C |
50 |
98 |
14a |
3 |
AuCl3, AgOTf (3 mol%), reflux |
360 |
78 |
15a |
4 |
Ca(OTf)2, BuNPF6, 120 °C |
240 |
92 |
13a |
The recyclability and reusability of catalyst was studied in the model one-pot three-component reaction between 4-nitrobenzaldehyde, acetophenone and 4-hydroxycoumarin. At the end of the reaction, the separated catalyst can be reused after being washed with warm EtOH and drying at 80 °C. NiFe2O4@SiO2@glucose amine was used again for subsequent experiments under similar reaction conditions. The catalyst could be reused for the next cycle without any notable loss of its activity. Yields of the product decreased only slightly after reusing the catalyst five times.
Experimental section
Materials and methods
Chemicals were purchased from Merck and Fluka. All solvents used were dried and distilled according to standard procedures. Melting points were measured on an Electrothermal 9100 apparatus. IR spectra were determined on a Shimadzu FT-IR 8600 spectrophotometer. 1H and 13C NMR spectra were determined on a Bruker 400 DRX Avance instrument at 500 and 125 MHz. Elemental analyses were recorded on a Carlo-Erba EA1110CNNO-S analyser.
Thin layer chromatography (TLC) was carried out with ethyl acetate
:
n-hexane 1
:
4 on TLC Silica gel 60 F254.
Synthesis of silica-coated NiFe2O4 (NiFe2O4@SiO2 MNPs). Typically, 500 mg of the NiFe2O4 nanoparticle were dispersed by ultrasonic vibration in a mixture of ethanol (20 ml), deionized water (3 ml) and 1 ml of 25 wt% concentrated aqueous ammonia solution for 20 min. Subsequently, 0.7 ml of tetraethylorthosilicate (TEOS) was added dropwise. After stirring for 12 h at room temperature under N2 atmosphere, the products was collected from the solution using a magnet, and then washed several times with water and ethanol and dried at 25 °C under vacuum.
Synthesis of NiFe2O4@SiO2–Cl MNPs. 500 mg NiFe2O4@SiO2 nanoparticles were dispersed into 50 ml toluene and sonicated for 20 min, followed by the addition of 0.5 ml (3-chloropropyl)trimethoxysilane (CPTES). Then, the mixture was refluxed at 110 °C with continuous stirring for 12 h under a nitrogen flow. The resulting NiFe2O4@SiO2–Cl MNPs was collected by magnetic separation followed by washing with toluene and ethanol several times and drying at 60 °C for 6 h.
Synthesis of NiFe2O4@SiO2@amino glucose. 500 mg NiFe2O4@SiO2–Cl MNPs were dispersed into 50 ml toluene and sonicated for 30 min, followed by the addition of 0.5 ml glucose amine. Then, the mixture was refluxed at 110 °C with continuous stirring for 12 h under a nitrogen flow. The resulting functionalized NiFe2O4@SiO2@aminoglucose was collected by magnetic separation followed by washing with toluene and ethanol several times and drying at 80 °C for 8 h.
General procedure for preparation of pyrano[3,2-c]chromen-5(4H)-ones 4a–n. A mixture of aldehyde 1 (1.0 mmol), acetophenone derivative 2 (1.0 mmol), 4-hydroxycoumarin 3 (1.0 mmol) and 0.05 g NiFe2O4@SiO2@aminoglucose were stirred at room temperature under solvent free condition for the required reaction time according to Tables 2 and 3 After completion of the reaction, as indicated by TLC (TLC Silica gel 60 F254, ethyl acetate
:
n-hexane 1
:
4), the resulting mixture was diluted with hot ethanol (10 ml) and the catalyst separated by an external magnet and washed with hot distilled water (5 ml) and ethanol (3 ml) two times. The filtrate was cooled down to room temperature and the crude products which precipitated were collected and recrystallized from ethanol if necessary. All of the synthesized compounds were characterized by their physical constants, IR, 1H NMR, 13C NMR spectroscopy, HR-Ms and elemental analysis. Some derivatives of the pyranochromenes are known and were compared with authentic samples matching melting points and spectras.
Analytical data for the selected compounds.
4-(3-Nitrophenyl)-2-phenylpyrano[3,2-c]chromen-5(4H)-one (4a). White solid, mp: 123–125 °C, 1H NMR (DMSO-d6, 400 MHz): δ = 5.41 (s, 1H), 7.05–7.92 (m, 14H) ppm. 13C NMR (DMSO-d6, 100 MHz): δ = 34.34, 103.34, 105.65, 109.28, 111.62, 111.74, 114.72, 115.82, 119.03, 119.38, 121.92, 127.72, 130.29, 135.87, 137.63, 139.72, 142.49, 145.83, 147.87, 151.54, 154.75, 162.09 ppm. FT-IR (KBr): 3077, 2938, 1707 (C
O), 1613, 1495, 1510 and 1388 (NO2), 1237 (C–O) cm−1. HRMs (m/z 397.1). Anal calc. for C24H15NO5: C, 72.54; H, 3.80; N, 3.52. Found: C, 72.51; H, 3.83; N, 3.55.
4-(4-Nitrophenyl)-2-phenylpyrano[3,2-c]chromen-5(4H)-one (4b). White solid, mp: 228–230 °C, 1H NMR (DMSO-d6, 400 MHz): δ = 5.42 (s, 1H), 7.23–7.97 (m, 14H) ppm. 13C NMR (DMSO-d6, 100 MHz): δ = 37.82, 103.60, 105.92, 109.54, 111.98, 111.19, 115.04, 116.04, 119.82, 121.62, 130.59, 133.72, 137.82, 139.48, 142.80, 145.27, 147.48, 151.38, 154.73, 162.24 ppm. FT-IR (KBr): 2918, 1663 (C
O), 1523 and 1356 (NO2), 1600, 1400, 1233 (C–O) cm−1. HRMs (m/z 397.1). Anal calc. for C24H15NO5: C, 72.54; H, 3.80; N, 3.52. Found: C, 72.53; H, 3.81; N, 3.54.
4-(4-Hydroxyphenyl)-2-phenylpyrano[3,2-c]chromen-5(4H)-one (4c). White solid, mp: 167–169 °C, 1H NMR (DMSO-d6, 400 MHz): δ = 5.4 (s, 1H), 6.88–7.92 (m, 14H), 9.8 (s, 1H, phenolic O–H) ppm. 13C NMR (DMSO-d6, 100 MHz): δ = 37.63, 103.56, 105.78, 109.72, 111.04, 111.37, 115.84, 116.50, 119.24, 121.97, 130.21, 133.83, 137.72, 139.05, 142.756, 143.64, 149.62, 151.58, 154.48, 160.48 ppm. FT-IR (KBr): 3239, 3069, 2930, 1707 (C
O), 1572, 1486, 1237 (C–O) cm−1. HRMs (m/z 368.1). Anal alc. for C24H16O4: C, 78.25; H, 4.38. Found: C, 78.21; H, 4.40.
2,4-Diphenylpyrano[3,2-c]chromen-5(4H)-one (4d). White solid, mp: 166–168 °C, 1H NMR (DMSO-d6, 400 MHz): δ = 5.41 (s, 1H), 6.78–7.64 (m, 15H) ppm. 13C NMR (DMSO-d6, 100 MHz): δ = 36.71, 103.12, 105.21, 109.11, 111.13, 111.38, 114.78, 115.03, 121.45, 126.76, 128.14, 135.87, 142.09, 143.91, 145.12, 145.67, 147.16, 151.09, 153.98, 165.41 ppm. FT-IR (KBr): 3099, 2987, 1665 (C
O), 1609, 1487, 1234 (C–O) cm−1. HRMs (m/z 352.1). Anal calc. for C24H16O3: C, 81.80; H, 4.58. Found: C, 81.81; H, 4.56.
4-(4-Methoxyphenyl)-2-phenylpyrano[3,2-c]chromen-5(4H)-one (4e). White solid, mp: 143–144 °C, 1H NMR (DMSO-d6, 400 MHz): δ = 3.50 (s, 3H), 5.59 (s, 1H), 7.19–8.17 (m, 14H) ppm. 13C NMR (DMSO-d6, 100 MHz): δ = 36.65, 54.58, 103.27, 105.82, 109.09, 111.27, 111.83, 114.62, 115.24, 123.74, 125.87, 129.87, 135.87, 142.38, 143.84, 145.63, 145.43, 151.87, 152.41, 153.49, 163.09 ppm. FT-IR (KBr): 2938, 1666 (C
O), 1495, 1388, 1261 (C–O), 1102 cm−1. HRMs (m/z 382.12). Anal calc. for C25H18O4: C, 78.52; H, 4.74. Found: C, 78.51; H, 4.71.
2,4-Bis(4-chlorophenyl)pyrano[3,2-c]chromen-5(4H)-one (4f). White solid, mp: 238–240 °C, 1H NMR (DMSO-d6, 400 MHz): δ = 6.10 (s, 1H), 7.07–8.09 (m, 13H) ppm. 13C NMR (DMSO-d6, 100 MHz): δ = 36.80, 103.72, 105.47, 109.71, 111.38, 111.06, 113.49, 115.05, 123.29, 123.93, 129.04, 135.62, 142.40, 143.21, 143.63, 145.63, 148.38, 151.09, 152.32, 161.32 ppm. FT-IR (KBr): 2934, 1675 (C
O), 1605, 1495, 1221 (C–O), 1098, 1016 cm−1. HRMs (m/z 420.03). Anal calc. for C24H14Cl2O3: C, 68.43; H, 3.35. Found: C, 68.41; H, 3.37.
4-(4-Chlorophenyl)-2-(3-nitrophenyl)pyrano[3,2-c]chromen-5(4H)-one (4g). White solid, mp: 189–190 °C, 1H NMR (DMSO-d6, 400 MHz): δ = 5.64 (s, 1H), 7.37–8.09 (m, 10H), 8.32 (s, 1H), 8.58 (s, 1H), 8.83 (s, 1H) ppm. 13C NMR (DMSO-d6, 100 MHz): δ = 36.29, 103.72, 105.48, 109.76, 111.39, 111.38, 115.41, 116.03, 119.61, 121.92, 130.04, 131.32, 133.72, 137.43, 139.85, 142.28, 144.93, 145.38, 147.72, 151.48, 154.05, 162.51 ppm. FT-IR (KBr): 3065, 2934, 1667 (C
O), 1609, 1572, 1392, 1245 (C–O), 1171 (C–Cl) cm−1. HRMs (m/z 431.06). Anal calc. for C24H14ClNO5: C, 66.75; H, 3.27; N, 3.24. Found: C, 66.71; H, 3.29; N, 3.27.
2-(3-Nitrophenyl)-4-p-tolylpyrano[3,2-c]chromen-5(4H)-one (4h). White solid, mp: 152–154 °C, 1H NMR (DMSO-d6, 400 MHz): δ = 2.31 (s, 3H), 5.61 (s, 1H), 7.11–8.11 (m, 8H), 8.25 (d, J = 8.2 Hz, 1H), 8.53–8.59 (m, 1H), 8.79 (s, 1H) ppm. 13C NMR (DMSO-d6, 100 MHz): δ = 31.29, 37.58, 103.40, 105.78, 109.72, 111.49, 111.48, 113.87, 116.28, 119.98, 121.76, 130.28, 131.73, 133.28, 133.69, 137.68, 139.29, 142.72, 145.63, 147.59, 151.73, 154.21, 161.82 ppm. FT-IR (KBr): 3089, 2922, 1675 (C
O), 1604, 1527, 1495, 1347, 1216 (C–O) cm−1. HRMs (m/z 411.11). Anal calc. for C25H17NO5: C, 72.99; H, 4.16; N, 3.40. Found: C, 72.98; H, 4.19; N, 3.41.
4-(4-Chlorophenyl)-2-phenylpyrano[3,2-c]chromen-5(4H)-one (4i). White solid, mp: 176–178 °C, 1H NMR (DMSO-d6, 400 MHz): δ = 5.63 (s, 1H), 7.14–7.83 (m, 9H), 7.89–8.02 (m, 2H), 8.14 (d, J = 8.0 Hz, 2H), 8.72 (s, 1H) ppm. 13C NMR (DMSO-d6, 100 MHz): δ = 36.46, 103.21, 105.51, 109.12, 110.93, 111.21, 113.63, 118.09, 119.27, 123.27, 130.05, 131.18, 133.72, 136.20, 138.51, 142.72, 145.63, 151.06, 154.18, 160.93 ppm. FT-IR (KBr): 3087, 2987, 1673 (C
O), 1613, 1565, 1475, 1323, 1256 (C–O) cm−1. HRMs (m/z 386.07). Anal calc. for C24H15ClO3: C, 74.52; H, 3.91. Found: C, 74.58; H, 3.99.
2-(4-Chlorophenyl)-4-phenylpyrano[3,2-c]chromen-5(4H)-one (4j). White solid, mp: 145–147 °C, 1H NMR (DMSO-d6, 400 MHz): δ = 5.58 (s, 1H), 7.27–7.85 (m, 8H), 8.01 (d, J = 7.8 Hz, 2H), 8.09 (d, J = 7.8 Hz, 2H), 8.23–8.32 (m, 1H), 8.74 (s, 1H) ppm. 13C NMR (100 MHz, DMSO-d6) δ (ppm): 36.51, 103.18, 105.93, 109.62, 111.04, 111.32, 113.61, 118.17, 119.40, 123.36, 130.12, 131.14, 133.63, 136.72, 138.90, 142.24, 145.05, 151.20, 156.82, 161.14 ppm. FT-IR (KBr): 3011, 2965, 1679 (C
O), 1600, 1545, 1432, 1278 (C–O) cm−1. HRMs (m/z 386.05). Anal calc. for C24H15ClO3: C, 74.52; H, 3.91. Found: C, 74.54; H, 3.95.
(4k). Off white solid, mp: >300 °C, 1H NMR (DMSO-d6, 400 MHz): δ = 5.63 (s, 1H), 7.07 (d, J = 7.6 Hz, 2H), 7.11–8.03 (m, 18H), 8.12 (s, 1H) ppm. 13C NMR (DMSO-d6, 100 MHz): δ = 56.9, 101.9, 105.6, 111.0, 111.7, 112.9, 117.7, 119.4, 119.8, 121.1, 123.3, 129.5, 129.7, 131.0, 131.6, 133.4, 133.7, 135.0, 135.4, 138.0, 138.2, 139.5, 140.4, 145.8, 151.7, 153.7, 163.0 ppm. FT-IR (KBr): 2973, 1721 (C
O), 1672, 1632, 1481, 1209 (C–O), 1108 cm−1. HRMs (m/z 494.16). Anal calc. for C33H22N2O3: C, 80.15; H, 4.48; N, 5.66; found: C, 80.10; H, 4.41, N, 5.67.
(4l). Off white solid, mp: 287–289 °C, 1H NMR (DMSO-d6, 400 MHz): δ = 2.31 (s, 3H), 5.59 (s, 1H), 7.11 (d, J = 7.8 Hz, 2H), 7.13–8.05 (m, 17H), 8.09 (s, 1H) ppm. 13C NMR (DMSO-d6, 100 MHz): δ = 43.5, 57.3, 101.3, 103.6, 111.4, 111.8, 113.6, 117.9, 119.8, 120.2, 121.0, 128.7, 129.8, 129.9, 130.5, 131.4, 133.3, 133.9, 135.3, 135.5, 139.0, 139.8, 139.9, 140.5, 143.6, 150.5, 153.4, 161.4 ppm. FT-IR (KBr): 2991, 1709 (C
O), 1657, 1641, 1492, 1235 (C–O), 1128 cm−1. HR-Ms (508.18 m/z). Anal calc. for C34H24N2O3: C, 80.30; H, 4.76; N, 5.51; found: C, 80.24; H, 4.76, N, 5.57.
(4m). Off white solid, mp: 265–267 °C, 1H NMR (DMSO-d6, 400 MHz): δ = 3.63 (s, 3H), 5.41 (s, 1H), 7.10 (d, J = 7.8 Hz, 2H), 7.13 (d, J = 7.6 Hz, 2H), 7.15–8.08 (m, 15H), 8.14 (s, 1H) ppm. 13C NMR (DMSO-d6, 100 MHz): δ = 56.9, 59.6, 101.6, 105.9, 111.6, 112.7, 113.5, 118.0, 119.0, 119.7, 120.6, 125.7, 129.3, 129.7, 130.6, 131.5, 133.9, 135.5, 135.7, 137.4, 138.5, 139.7, 140.0, 143.7, 148.9, 151.2, 153.4, 161.3 ppm. FT-IR (KBr): 2962, 1709 (C
O), 1652, 1641, 1493, 1226 (C–O), 1170 cm−1. HR-Ms (524.17 m/z). Anal calc. for C34H24N2O4: C, 77.85; H, 4.61; N, 5.34. Found: C, 77.84; H, 4.66, N, 5.32.
(4n). White solid, mp: >300 °C, 1H NMR (DMSO-d6, 400 MHz): δ = 5.44 (s, 2H), 7.10–7.14 (m, 4H), 7.16–7.34 (m, 4H), 7.136–8.05 (m, 16H) ppm. 13C NMR (DMSO-d6, 100 MHz): δ = 56.9, 101.6, 104.8, 111.2, 111.7, 113.0, 119.6, 119.9, 125.4, 129.6, 129.9, 131.6, 133.2, 135.8, 142.0, 151.5, 152.6, 161.0. FT-IR (KBr): 3057, 2983, 1723 (C
O), 1655, 1647, 1462, 1219 (C–O), 1151 cm−1. HR-Ms (m/z 657.23). Anal calc. for C44H33O6: C, 80.35; H, 5.06. Found: C, 80.34; H, 5.11.
Conclusion
In conclusion, we have developed NiFe2O4@SiO2@glucose amine as a new, mild and efficient avenue for the synthesis of pyrano[3,2-c]chromen-5(4H)-ones. This nano particles were synthesized for the first time and can act as a promoter to activate the substrate molecule for the synthesis of pyrano[3,2-c]chromen-5(4H)-ones. The operational simplicity, the excellent yields of products, ease of separation and recyclability of the magnetic catalyst, waste reduction and high selectivity are the main advantages of this method. Furthermore, this new avenue is cheap and environmentally benign. This novel concept is expected to use to development of more benign reactions.
Conflicts of interest
There are no conflicts to declare.
Acknowledgements
Financial support from the Research Council of Payame Noor University of Rasht and Roodsar branch is sincerely acknowledged.
References
- M. M. Heravi, B. Alimadadi Jani, F. Derikvand, F. F. Bamoharram and H. A. Oskooie, Catal. Commun., 2008, 10, 272 CrossRef.
- L. Bonsignore, G. Loy, D. Secci and A. Calignano, Eur. J. Med. Chem., 1993, 28, 517 CrossRef.
- G. Cingolani, F. Gualtieri and M. Pigini, J. Med. Chem., 1969, 12, 531 CrossRef PubMed.
- J. Y. C. Wu, W. F. Fong, J. X. Zhang, C. H. Leung, H. L. Kwong, M. S. Yang, D. Li and H. Y. Cheung, Eur. J. Pharmacol., 2003, 473, 9 CrossRef PubMed.
- F. W. Perrella, S. F. Chen, D. L. Behrens, R. F. Kaltenbach and S. P. Seitz, J. Med. Chem., 1994, 37, 2232 CrossRef PubMed.
- N. R. Emmadi, K. Atmakur, G. K. Chityal, S. Pombala and J. B. Nanubolu, Bioorg. Med. Chem. Lett., 2012, 22, 7261 CrossRef PubMed.
- Y. Kashman, K. R. Gustafson, R. W. Fuller, J. H. Cardellina, J. B. Mcmahon, M. J. Currens, R. W. Buckheit, S. H. Hughes, G. M. Cragg and M. R. Boyd, J. Med. Chem., 1992, 35, 2735 CrossRef PubMed.
- A. D. Patil, A. J. Freyer, D. S. Eggleston, R. C. Haltiwanger, M. F. Bean, P. B. Taylor, M. J. Caranfa, A. L. Breen and H. R. Bartus, J. Med. Chem., 1993, 36, 4131 CrossRef PubMed.
- D. C. Mungra, M. P. Patel, D. P. Rajani and R. G. Patel, Eur. J. Med. Chem., 2011, 46, 4192 CrossRef PubMed.
- M. Zabradnik, “The Production and Application of Fluorescent Brightening Agents”, John Wiley and Sons, New York, NY, 1992, vol. 1, p. 15 Search PubMed.
- R. D. Murray; J. Mendez and S. A. Brown, “The Natural Coumarins: Occurrence, Chemistry and Biochemistry”, John Wiley and Sons, New York, NY, 1982, vol. 7, p. 430 Search PubMed.
- M. Gohain, H. T. Johannes and C. B. B. Barend, Tetrahedron Lett., 2013, 54, 3773 CrossRef.
- Y. Srinivasarao, S. Pyare Lal and G. Singh, Tetrahedron Lett., 2015, 56, 1649 CrossRef.
- A. Naseem and B. Venkata Babu, Synth. Commun., 2013, 43, 3044 CrossRef.
- L. Yunkui, Z. Jie, Q. Jianqiang, B. Jiang and X. Zhenyuan, J. Org. Chem., 2011, 76, 9096 CrossRef PubMed.
- P. A. Wender, Nat. Prod. Rep., 2014, 31, 433 RSC.
- A. Z. Halimehjani, I. N. Namboothiri and S. E. Hooshmand, RSC Adv., 2014, 4, 48022 RSC.
- A. Z. Halimehjani, I. N. Namboothiri and S. E. Hooshmand, RSC Adv., 2014, 4, 51794 RSC.
- V. Estévez, M. Villacampa and J. C. Menéndez, Chem. Soc. Rev., 2014, 43, 4633 RSC.
- A. Domling, W. Wang and K. Wang, Chem. Rev., 2012, 112, 3083 CrossRef PubMed.
- S. M. Vahdat, Sh. Ghafouriraz and S. Baghery, J. Chem. Sci., 2014, 126, 579 CrossRef.
- M. Nikpassand, L. Zare Fekri, L. Karimian and M. Rassa, Curr. Org. Synth., 2015, 12, 358 CrossRef.
- M. Nikpassand, L. Zare Fekri and P. Farokhian, Ultrason. Sonochem., 2016, 28, 341 CrossRef PubMed.
- L. Zare Fekri, M. Nikpassand and K. Hasanpour, Curr. Org. Synth., 2015, 12, 76 CrossRef.
- L. Zare Fekri, M. Nikpassand and R. Maleki, J. Mol. Liq., 2016, 222, 77 CrossRef.
- M. Nikpassand, L. Zare and M. R. Mousavi, Lett. Org. Chem., 2012, 9, 375 CrossRef.
- M. Nikpassand, L. Z. Fekri and S. Sanagou, Dyes Pigm., 2017, 136, 140 CrossRef.
- L. Zare, N. Mahmoodi, A. Yahyazadeh, M. Mamaghani and K. Tabatabaeian, J. Heterocycl. Chem., 2011, 48, 864 CrossRef.
- L. Zare, N. O. Mahmoodi, A. Yahyazadeh and M. Mamaghani, Synth. Commun., 2011, 41, 2323 CrossRef.
- M. Veeranarayana Reddy, B. Siva Kumar, K. Taek Lim, B. Gwon Cho and Y. Tae Jeong, Tetrahedron Lett., 2016, 57, 476 CrossRef.
|
This journal is © The Royal Society of Chemistry 2018 |
Click here to see how this site uses Cookies. View our privacy policy here.