DOI:
10.1039/C8RA03940B
(Paper)
RSC Adv., 2018,
8, 20456-20461
Effects of the inhibitor of glutamate decarboxylase on the development and GABA accumulation in germinating fava beans under hypoxia-NaCl stress
Received
8th May 2018
, Accepted 23rd May 2018
First published on 5th June 2018
Abstract
Glutamate decarboxylase (GAD) is the key enzyme in GABA shunt, which catalyzes the α-decarboxylation of glutamate to produce GABA. A specific inhibitor for GAD is convenient to study the dynamic balances of GABA metabolism in plants. The inhibitor of GAD in germinated fava beans was screened, and its inhibitory effect on the growth and GABA accumulation in fava beans during germination under hypoxia-NaCl stress was investigated. The inhibitory effect of aminoxyacetate for fava bean GAD was better than those of other chemicals, and it increased with the increase in concentration in vivo. After aminoxyacetate (5 mM) application for 4 days during germination, the GAD activity in germinating fava beans was significantly inhibited by more than 90% in both organs. Meanwhile, the growth of fava bean sprouts was also slightly suppressed. Moreover, the GABA contents decreased by 43.9% and 81.5% in a 4 day-old cotyledon and embryo, respectively, under aminoxyacetate treatment compared with that in the control. In summary, these results showed that aminoxyacetate can serve as a specific inhibitor of GAD in plants. At least 43.9% and 81.5% of GABA in germinating fava beans under hypoxia-NaCl stress were synthesized via GABA shunt.
1. Introduction
Γ-Aminobutyric acid (GABA) is a ubiquitous four carbon, non-proteinaceous amino acid, which is one of the major inhibitory neurotransmitters in mammals.1 Recently, a huge amount of research has been done on the rapid accumulation of GABA levels in bean sprouts through seed germination under abiotic stresses, especially hypoxia and salt stress,2–5 as GABA can prevent certain forms of cancer and reduce the risk of cardiovascular diseases.6,7 In higher plants, the conserved metabolic pathways known as the GABA shunt and polyamine (PA) degradation pathways have been well studied. GABA is synthesized from alpha-decarboxylation of glutamate (Glu) catalyzed by glutamate decarboxylase (GAD, EC 4.1.1.15) in the cytosol; this process is called the GABA shunt.8 Meanwhile, polyamine (PA) oxidation is catalyzed by diamine oxidase (DAO, EC 1.4.3.6) and polyamine oxidase (PAO, EC 1.5.3.11) to form γ-amino butyraldehyde and then, γ-aminobutyraldehyde is converted by aminoaldehyde dehydrogenase (AMADH, EC 1.2.1.19) to produce GABA.9
Mechanisms have been put forward for the production of GABA, the dynamic balances of GABA shunt and PA degradation pathway in plants. At present, the effect of the PA degradation pathway on GABA accumulation was investigated by inhibiting DAO activity via aminoguanidine (AG).2,10 The reduction in GABA formation was considered to be due to PA degradation pathway after DAO activity was inhibited. It has been reported that about 30% of GABA accumulation is due to PA degradation in germinated fava beans under non-stress11 and hypoxia stress.2 However, there is no specific inhibitor for GAD to investigate the contribution of GABA shunt on GABA accumulation in plant tissues under stress, and the contribution rate of the GABA shunt to GABA accumulation is not clear, although the GABA shunt has been studied since it was first reported more than fifty years back.12 Furthermore, AG is a specific inhibitor of DAO rather than PAO and AMADH. More and more research has demonstrated that AG cannot inhibit DAO activity completely10,11,13 let alone PAO and AMADH, which are also key enzymes responsible for GABA synthesis in the polyamine degradation pathway.9 A specific inhibitor for GAD, as the only known rate-limiting synthase in GABA shunt, is convenient to study the accurate contribution ratio of the GABA shunt for GABA formation. Besides, further studies on the GABA shunt will undoubtedly provide a new understanding of the mechanism of GABA accumulation.
GAD has been identified and purified in various higher plants, and its enzymatic properties have been studied in detail.14–16 Considerable inhibitory potentials of certain reagents have been confirmed in vitro, particularly the inhibitory effects on purified plant GAD.14 These in vitro studies indicated that sulfhydryl compound and aminoxyacetate can inhibit the activity of purified GAD.14,17 To the best of our knowledge, very few studies have reported the effects of these reagents on the physiological functions of plants, especially the metabolic process of GABA. The aim of this study is to confirm the in vivo inhibitory effect of four selected inhibitors. Meanwhile, in case of the specific inhibitor applications, the changes in the growth and the contribution of GABA shunt in GABA accumulation of fava beans during germination under hypoxia-NaCl stress are investigated.
2. Experimental
2.1. Materials and reagents
The fava bean seeds (Qi Bean 2) were purchased from Jiangsu Academy of Agricultural Sciences (Nanjing, China). Aminoxyacetate, GABA, dimethylaminoazobenzene sulfonyl chloride and Glu were purchased from Sigma Chemical Co. (St Louis, MO, USA).
2.2. Material treatment and experimental design
Dried fava bean seeds were surface-sterilized by soaking in 1% (v/v) sodium hypochlorite for 15 min; then, they were washed and steeped in deionized water for 8 h at 30 °C. The soaked seeds were put into a culturing pallet (25 cm × 20 cm × 5 cm) and incubated in the dark at 30 °C for 48 h. The seeds were sprayed with deionized water every 8 h. Then, the pretreated fava beans were placed in sprout pots (6 cm diameter × 9 cm height) and germinated in a dark incubator at 30 °C for 4 days under hypoxia-NaCl stress (pH 3.5, 10 mM citrate buffer, 60 mM NaCl, dissolved oxygen concentration 5.5 mg L−1). The condition of hypoxia-NaCl treatment was chosen because of the high GABA content in germinated fava beans, as indicated in our previous study.18 Meanwhile, the culture solution contained 0.0, 2.5, 5.0, 7.5 and 10.0 mM of β-mercaptoethanol, dithiothreitol, L-cysteine and aminoxyacetate, respectively. Three independent biological experiments were performed. During the germination, the culture solution was replaced at 8 h intervals. The sprouts were collected, rinsed, frozen in liquid nitrogen, and stored at −20 °C until analyses.
Moreover, the pretreated fava bean seeds were germinated under hypoxia-NaCl stress as described above, and the culture solution contained 5.0 mM of aminoxyacetate. The control sprouts were germinated under the culture solution without inhibitors. The seeds were germinated for 0 d, 1 d, 2 d, 3 d and 4 d. Then, the sprouts were also collected, rinsed, frozen and stored for analyses.
2.3. Measurements of GABA and Glu contents
GABA and Glu were extracted from germinated fava beans and purified according to the procedure described by Bai et al.19 The residue was dissolved in 2 mL of 1 M sodium bicarbonate (pH 9.0) and centrifuged at 6000 g for 10 min. GABA and Glu contents were determined using the Agilent 1200 Series HPLC system with a ZORBAX Eclipse AAA reverse phase column (150 mm × 4.6 mm, 3.5 μm; Agilent, Palo Alto, Calif., USA), as described by Yang et al.2
2.4. GAD activity assay
GAD activity was determined according to the method described by Bai et al.19 The GABA content in the reaction solution was analyzed by the standard method. One unit of GAD activity was defined as the release of 1 μM of GABA produced from glutamate per 60 min at 40 °C. The GAD activity of plant tissues was defined as units of GAD activity of 1 g DW.
2.5. Measurements of sprout length and fresh weight
Sprout length was measured directly using a vernier caliper. Thirty sprouts were set as a sampling group for each measurement. For determination of fresh weight (FW) and dry weight (DW), 30 soybeans were weighed, followed by oven-drying at 80 °C for 48 h and then, the average weight was calculated.
2.6. Statistical analysis
All results were expressed as mean values ± standard deviations of three replicates independent of the germinated fava beans. The data obtained were subjected to analysis of variance (ANOVA), and the mean differences were compared with Tukey's test; a p value at 0.05 was considered significant.
3. Results and discussion
3.1. Selection of GAD inhibitor
The present study illustrated the in vivo inhibitory effects of sulfhydryl compounds and aminoxyacetate on germinated fava bean GAD activity in various concentrations (Table 1). GAD activities in cotyledon and embryo of germinating fava beans showed a dose-dependent decrease with an increase in the concentrations of the selected inhibitors 0.0 mM onwards. In comparison with the results for the control (0.0 mM), the GAD activities in both organs were significantly inhibited, as was observed at 0.25 mM of all the inhibitors. After treatment with β-mercaptoethanol, dithiothreitol and L-cysteine, the GAD activity in the embryo was found to be higher than that in cotyledon at the same concentration, whereas the results were different under aminoxyacetate treatment. GAD activity in both organs significantly decreased up to 7.5 mM of β-mercaptoethanol, dithiothreitol and L-cysteine and then remained constant following any further increase in their levels (Table 1). The fava beans were germinated for 4 days under 5.0 mM of aminoxyacetate treatment, after which it was found that the GAD activities in embryo were 53.6%, 17.3% and 21.5% for the β-mercaptoethanol, dithiothreitol and L-cysteine treatments, respectively, at the same concentration. Moreover, the GAD activity was not detected in cotyledon and embryo of the germinated fava beans treated with 10.0 mmol L−1 aminoxyacetate. These results suggested that aminoxyacetate maybe the appropriate inhibitor for GAD activity during fava bean germination.
Table 1 Effect of sulfhydryl compound and aminoxyacetate on GAD activitya
Inhibitors |
Organs |
Concentration (mmol L−1) |
0.0 |
2.5 |
5.0 |
7.5 |
10.0 |
Lower case letters reflect the significance of differences in GAD activity among chemical concentrations (p < 0.05). |
β-Mercaptoethanol |
Cotyledon |
15.37 ± 0.55a |
3.12 ± 0.05b |
2.57 ± 0.08bc |
2.10 ± 0.02bc |
1.87 ± 0.06c |
Embryo |
18.88 ± 0.64a |
4.50 ± 0.10b |
3.45 ± 0.15c |
2.29 ± 0.03d |
1.98 ± 0.04d |
Dithiothreitol |
Cotyledon |
15.37 ± 0.55a |
9.33 ± 0.49b |
8.81 ± 0.60b |
5.90 ± 0.19c |
5.01 ± 0.68c |
Embryo |
18.88 ± 0.64a |
11.19 ± 0.58b |
10.67 ± 0.20b |
7.28 ± 0.29c |
6.93 ± 0.69c |
L-Cysteine |
Cotyledon |
15.37 ± 0.55a |
5.85 ± 0.32b |
5.33 ± 0.22b |
4.10 ± 0.12c |
3.02 ± 0.23c |
Embryo |
18.88 ± 0.64a |
9.60 ± 0.18b |
8.57 ± 0.36c |
4.97 ± 0.25d |
3.80 ± 0.64d |
Aminoxyacetate |
Cotyledon |
15.37 ± 0.55a |
2.85 ± 0.20b |
2.38 ± 0.02b |
0.78 ± 0.02c |
Not detected |
Embryo |
18.88 ± 0.64a |
3.12 ± 0.16b |
1.85 ± 0.03c |
0.79 ± 0.02d |
Not detected |
3.2. Changes in GABA and Glu contents and sprout lengths after inhibition of GAD activity
The GABA content significantly decreased (p < 0.05) in cotyledon and embryo of germinated fava beans exposed to aminoxyacetate (Table 2). After treatment with 5.0 mM aminoxyacetate, the GABA contents in cotyledon and embryo decreased by 66.03% and 90.32%, respectively, in comparison with the results for the untreated control. The Glu content showed a different response, that is, its content increased first at low concentrations of aminoxyacetate and then, it started markedly declining at higher concentrations of aminoxyacetate (Table 2). The Glu contents in cotyledon and embryo of 4 d germinated fava beans increased 1.56- and 1.53-fold, respectively, when compared with those of the control. The sprout length of the germinating fava bean, understandably, was progressively suppressed with the increase in aminoxyacetate concentration, whereas there was no significant difference found when the concentration of aminoxyacetate was above 5.0 mM (Table 2). The above-mentioned results suggested that the optimal inhibition concentration of aminoxyacetate in germinated fava beans was 5.0 mM.
Table 2 Effect of aminoxyacetate on GABA content, Glu content and sprout length in hypoxia-NaCl stressed fava beana
Aminoxyacetate (mmol L−1) |
GABA content (mg g−1 DW) |
Glu content (mg g−1 DW) |
Sprout length (mm) |
Cotyledon |
Embryo |
Cotyledon |
Embryo |
|
Lower case letters reflect the significance of differences in GABA content, Glu content and sprout length among chemical concentrations (p < 0.05). |
0.0 |
1.56 ± 0.17a |
6.92 ± 0.41a |
68.45 ± 5.04b |
94.73 ± 12.65b |
10.5 ± 0.4a |
2.5 |
1.31 ± 0.10b |
1.91 ± 0.23b |
104.52 ± 11.26a |
99.42 ± 6.76b |
7.8 ± 0.2b |
5.0 |
0.96 ± 0.06c |
1.25 ± 0.12c |
106.93 ± 10.21a |
145.87 ± 19.58a |
6.9 ± 0.1c |
7.5 |
0.53 ± 0.08d |
0.67 ± 0.03d |
57.91 ± 6.05bc |
67.84 ± 5.87c |
6.6 ± 0.1c |
10.0 |
0.50 ± 0.02d |
0.64 ± 0.04d |
52.29 ± 5.81c |
65.48 ± 6.92c |
6.4 ± 0.2c |
3.3. Growth performance of germinated fava bean
To evaluate the effect of aminoxyacetate on the germinated fava bean, its sprout length and fresh weight were measured during germination (Fig. 1). Compared with that of the control, the sprout length of the fava bean decreased during germination in a aminoxyacetate-treated sprout (Fig. 1A). After germinating for 4 days under aminoxyacetate treatment, the sprout length was 65.7% of that of the control. Similarly, the inhibitory effect of aminoxyacetate was also observed on the fresh weight (Fig. 1B). The fresh weight increased significantly (p < 0.05) with germination time under treatment; however, after 4 days, aminoxyacetate addition resulted in a decrease by 12.9% compared with the results for the control. The growth of germinating fava beans was inhibited under aminoxyacetate treatment during germination.
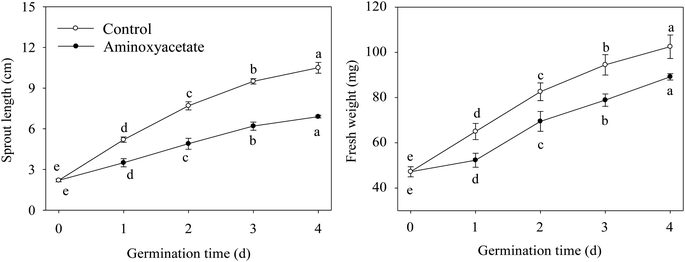 |
| Fig. 1 Effect of aminoxyacetate on sprout length and fresh weight of germinated fava bean. Lower case letters reflect the significance of differences in sprout length and fresh weight during germinating under their respective treatments (p < 0.05). | |
3.4. Changes in GAD activity in germinating fava bean
Under aminoxyacetate treatment, the GAD activities in cotyledon and embryo during germination are shown in Fig. 2. Under the normal hypoxia-NaCl stress condition, the GAD activity increased first and then decreased during germination, showing peak values at 3 d and 2 d of germination in cotyledon and embryo, respectively. As expected, aminoxyacetate treatment led to a continuous decrease in GAD activity, which was always maintained at a lower level during germination in both organs (Fig. 2). These facts proved that aminoxyacetate is a specific inhibitor of GAD and can be selected appropriately for the research regarding the mechanism and dynamic balances of GABA in higher plants.
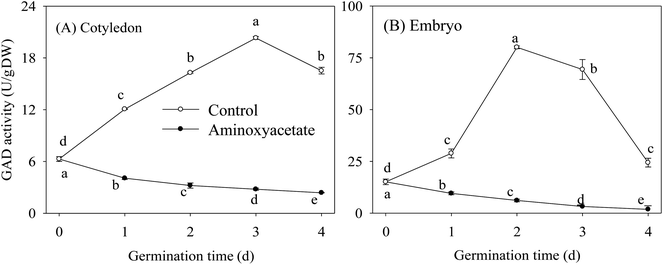 |
| Fig. 2 Effect of aminoxyacetate on GAD activity of germinated fava bean. Lower case letters reflect the significance of differences in GAD activity during germinating under their respective treatments (p < 0.05). | |
3.5. Changes of GABA and Glu contents in germinating fava bean
GABA contents in cotyledon and embryo both showed a continual and significant enhancement under normal hypoxia-NaCl stress during germination, and the GABA content was higher in the embryo when compared with that in the cotyledon (Fig. 3A and B). Under aminoxyacetate treatment, the GABA content gradually increased as the germination time was prolonged. Simultaneously, the aminoxyacetate treatment resulted in a noticeable decrease in GABA contents by 43.9% and 81.5% in the cotyledon and embryo, respectively, of 4 d germinated fava beans when compared with the results of the untreated control. In the early stage of germination, the Glu contents in fava beans continued to increase under both treatments, but the contents exhibited decrease in later stages. The Glu contents in cotyledon and embryo under aminoxyacetate treatment remained higher than those of untreated control during the whole germination process (Fig. 3C).
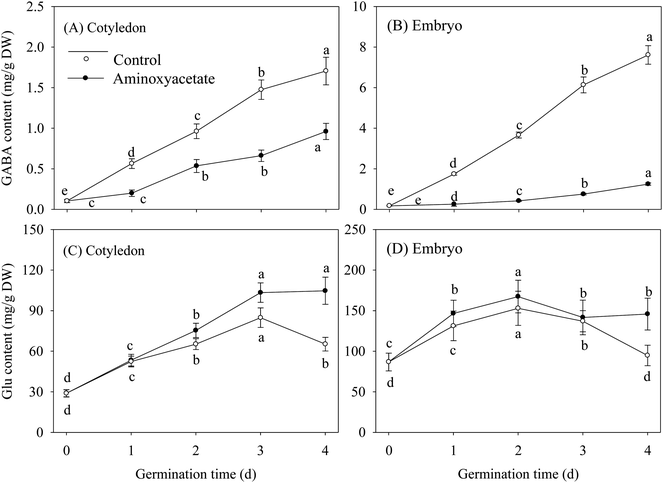 |
| Fig. 3 Effect of aminoxyacetate on GABA and Glu contents of germinated fava bean. Lower case letters reflect the significance of differences in GABA and Glu contents during germinating under their respective treatments (p < 0.05). | |
3.6. Discussion
In plants, GABA is synthesized via GABA shunt and polyamine degradation pathway. Abiotic stress can significantly increase the GAD and DAO activities and promote GABA accumulation during seed germination.8,9 The primary biosynthesis of GABA in plants is carried out by the action of cytosolic GAD.8 Studies on GAD are required for a better understanding of the metabolic mechanism of GABA in plants. There have been numerous studies that have investigated the regulation and activity of GAD in different plants;20–22 also, the enzymatic properties of plant GAD have been studied in detail.15–17 Plant GAD generally contains a C-terminal region known as the Ca2+/calmodulin (CaM)-binding domain.23 Hence, the study indicated that GAD activity can be inhibited by sulphydryl reagents, aminoxyacetate, calcium binding agents, 3-mercaptopropionate, etc.24–26 However, there is very less information about the effects of these inhibitors on the physiological and metabolic processes of GABA in plants. It was evident from our study that β-mercaptoethanol, dithiothreitol and L-cysteine showed dose-dependent inhibitory control abilities in fava bean GAD during germination. Consistent with previous in vitro results,2,25 the suppression effect of aminoxyacetate on GAD activity in germinating fava beans under hypoxia-NaCl stress was still better than those of other chemical inhibitors in the present study.
Not surprisingly, 5.0 mmol L−1 of aminoxyacetate not only inhibited GAD activity but also suppressed the growth of fava bean sprouts during germination, since the GABA shunt has been proven to play an important role in plant carbon and nitrogen partitioning and development.8,20 Moreover, Barbosa et al. suggested that the plant GAD plays a critical role in the regulation of pH, mineral acquisition and nitrogen uptake.27 GAD activity may also have a relationship with the earlier diversification and evolution of vascular plants.28 In the present research, aminoxyacetate inhibited 90.1% and 94.5% of GAD activities in cotyledon and embryo, respectively, of 4 d germinated fava beans. Subsequently, the GABA contents in the cotyledon and embryo decreased by 43.9% and 81.5%, respectively, compared with those of the control. It can be concluded that in cotyledon and embryo of the germinating fava beans under hypoxia-NaCl stress, the GABA shunt provided at least 43.9% and 81.5% of GABA formation as GAD activity was not inhibited completely. Previous researchers have found that between 30% and 40% of GABA accumulation is contributed by the polyamine degradation pathway in germinated fava beans under hypoxia stress by the inhibition of DAO activity via AG.11 Meanwhile, AG inhibited 39% and 24.5% of GABA formation in NaCl-treated soybeans, as noted in the paper by Xing et al.10 and our previous research,29 respectively. This difference may be due to different abiotic stresses and sampling positions. The mechanism of GABA in higher plants depends on the severity and duration of the stress and the growth conditions as well as the developmental stages of the plants. Furthermore, Glu is the direct substrate for GABA accumulation in the GABA shunt. Hence, in the present study, the Glu contents in both organs of aminoxyacetate-treated fava beans were increased compared with those of the control during germination, since the GAD activity was significantly inhibited.
4. Conclusions
Aminoxyacetate can be the most appropriate inhibitor of GAD activity in vivo, and it can be conveniently used to study the contribution ratio of GABA shunt and polyamine degradation pathway for GABA formation. It can be inferred that at least 43.9% and 81.5% of GABA accumulations were supplied by the GABA shunt in cotyledon and embryo, respectively, under hypoxia-NaCl stress.
Conflicts of interest
There are no conflicts of interest to declare.
Acknowledgements
This work was financially supported by the Natural Science Foundation of China (31501401), Natural Science Foundation of Jiangsu Province (BK 20150448) and Natural Science Foundation of Colleges and Universities in Jiangsu Province (15KJB550010) and Postgraduate Research & Practice Innovation Program of Jiangsu Province (SJLX18_0616)
References
- M. G. Erlander and A. J. Tobin, The structural and functional heterogeneity of glutamic acid decarboxylase: a review, Neurochem. Res., 1991, 16, 215–226 CrossRef PubMed.
- R. Yang, Q. Guo and Z. Gu, GABA shunt and polyamine degradation pathway on γ-aminobutyric acid accumulation in germinating fava bean (Vicia faba L.) under hypoxia, Food Chem., 2013, 136, 152–159 CrossRef PubMed.
- Y. Yin, R. Yang, Q. Guo and Z. Gu, NaCl stress and supplemental CaCl2 regulating GABA metabolism pathways in germinating soybean, Eur. Food Res. Technol., 2014, 238, 781–788 CrossRef.
- Y. Guo, R. Yang, H. Chen, Y. Song and Z. Gu, Accumulation of γ-aminobutyric acid in germinated soybean (Glycine max L.) in relation to glutamate decarboxylase and diamine oxidase activity induced by additives under hypoxia, Eur. Food Res. Technol., 2012, 234, 679–687 CrossRef.
- X. Yan, P. Wang, T. Zhou, Z. Gu and R. Yang, NaCl-CaCl2 treatment enhancing nutritional and functional quality of mung bean sprouts, Emir. J. Food Agric., 2017, 29, 123–131 CrossRef.
- D. Del Rio, A. Rodriguez-Mateos, J. P. Spencer, M. Tognolini, G. Borges and A. Crozier, Dietary (poly) phenolics in human health: structures, bioavailability, and evidence of protective effects against chronic diseases, Antioxid. Redox Signaling, 2013, 18, 1818–1892 CrossRef PubMed.
- E. García-Martín, G. Esguevillas, M. Serrador, H. Alonso-Navarro, F. Navacerrada, G. Amo, E. García-Albea, J. A. Agúndez and F. J. Jiménez-Jiménez, Gamma-aminobutyric acid (GABA) receptors GABRA4, GABRE, and GABRQ gene polymorphisms and risk for migraine, J. Neural Transm., 2018, 4, 1–10 Search PubMed.
- N. Bouche and H. Fromm, GABA in plants: just a metabolite?, Trends Plant Sci., 2004, 9, 110–115 CrossRef PubMed.
- B. J. Shelp, G. G. Bozzo, C. P. Trobacher, A. Zarei, K. L. Deyman and C. J. Brikis, Hypothesis/review: Contribution of putrescine to 4-aminobutyrate (GABA) production in response to abiotic stress, Plant Sci., 2012, 193, 130–135 CrossRef PubMed.
- S. G. Xing, Y. B. Jun, Z. W. Hau and L. Y. Liang, Higher accumulation of gamma-aminobutyric acid induced by salt stress through stimulating the activity of diarnine oxidases in Glycine max (L.) Merr. roots, Plant Physiol. Biochem., 2007, 45, 560–566 CrossRef PubMed.
- R. Yang, H. Chen and Z. Gu, Factors influencing diamine oxidase activity and γ-aminobutyric acid content of fava bean (Vicia faba L.) during germination, J. Agric. Food Chem., 2011, 59, 11616–11620 CrossRef PubMed.
- C. Dent, W. Stepka and F. Steward, Detection of the free amino acids of plant cells by partition chromatography, Nature, 1947, 160, 682–683 CrossRef PubMed.
- Y. Guo, H. Chen, Y. Song and Z. Gu, Effects of soaking and aeration treatment on γ-aminobutyric acid accumulation in germinated soybean (Glycine max L.), Eur. Food Res. Technol., 2011, 232, 787–795 CrossRef.
- R. Yang, Y. Yin, Q. Guo and Z. Gu, Purification, properties and cDNA cloning of glutamate decarboxylase in germinated faba bean (Vicia faba L.), Food Chem., 2013, 138, 1945–1951 CrossRef PubMed.
- S. H. Oh, W. G. Choi, I. T. Lee and S. J. Yun, Cloning and characterization of a rice cDNA encoding glutamate decarboxylase, BMB Rep., 2005, 38, 595–601 CrossRef.
- G. Baum, Y. Chen, T. Arazi, H. Takatsuji and H. Fromm, A plant glutamate decarboxylase containing a calmodulin binding domain. Cloning, sequence, and functional analysis, J. Biol. Chem., 1993, 268, 19610–19617 Search PubMed.
- P. V. Taberner, M. J. Pearce and J. C. Watkins, The inhibition of mouse brain glutamate decarboxylase by some structural analogues of L-glutamic acid, Biochem. Pharmacol., 1977, 26, 345–349 CrossRef PubMed.
- R. Yang, Y. Yin, L. Guo and Z. Gu, Sequence analysis of diamine oxidase gene from fava bean and its expression related to γ-aminobutyric acid accumulation in seeds germinating under hypoxia-NaCl stress, J. Sci. Food Agric., 2014, 94, 1585–1591 CrossRef PubMed.
- Q. Y. Bai, M. Q. Chai, Z. X. Gu, X. H. Cao, Y. Li and K. L. Liu, Effects of components in culture medium on glutamate decarboxylase activity and gamma-aminobutyric acid accumulation in foxtail millet (Setaria italica L.) during germination, Food Chem., 2009, 116, 152–157 CrossRef.
- Y. Seher, O. Filiz and B. Melike, Gamma-amino butyric acid, glutamate dehydrogenase and glutamate decarboxylase levels in phylogenetically divergent plants, Plant Syst. Evol., 2013, 299, 403–412 CrossRef.
- F. J. Turano and T. K. Fang, Characterization of two glutamate decarboxylase cDNA clones from Arabidopsis, Plant Physiol., 1998, 117, 1411–1421 CrossRef PubMed.
- W. A. Snedden, T. Arazi, H. Fromm and B. J. Shelp, Calcium/calmodulin activation of soybean glutamate decarboxylase, Plant Physiol., 1995, 108, 543–549 CrossRef PubMed.
- M. Takayama, C. Matsukura, T. Ariizumi and H. Ezura, Activating glutamate decarboxylase activity by removing the autoinhibitory domain leads to hyper γ-aminobutyric acid (GABA) accumulation in tomato fruit, Plant Cell Rep., 2017, 36, 103–116 CrossRef PubMed.
- F. Roberts, P. V. Taberner and R. G. Hill, The effect of 3-mercaptopropionate, an inhibitor of glutamate decarboxylase, on the levels of GABA and other amino acids, and on presynaptic inhibition in the rat cuneate nucleus, Neuropharmacology, 1978, 17, 715–720 CrossRef PubMed.
- T. Tsushida and T. Murai, Conversion of glutamic acid to γ-aminobutyric acid in tea leaves under anaerobic conditions, Agric. Biol. Chem., 1987, 51, 2865–2871 Search PubMed.
- R. Yang, Y. Yin, Q. Guo and Z. Gu, Purification, properties and cDNA cloning of glutamate decarboxylase in germinated faba bean (Vicia faba L.), Food Chem., 2013, 138, 1945–1951 CrossRef PubMed.
- J. M. Barbosa, N. K. Singh, J. H. Cherry and R. D. Locy, Nitrate uptake and utilization is modulated by exogenous γ-aminobutyric acid in Arabidopsis thaliana seedlings, Plant Physiol. Biochem., 2010, 48, 443–450 CrossRef PubMed.
- J. J. Molina-Rueda, M. B. Pascual, F. M. Cánovas and F. Gallardo, Characterization and developmental expression of a glutamate decarboxylase from maritime pine, Planta, 2010, 232, 1471–1483 CrossRef PubMed.
- Y. Yin, R. Yang and Z. Gu, Organ-specific proteomic analysis of NaCl-stressed germinating soybeans, J. Agric. Food Chem., 2014, 62, 7233–7244 CrossRef PubMed.
|
This journal is © The Royal Society of Chemistry 2018 |
Click here to see how this site uses Cookies. View our privacy policy here.