DOI:
10.1039/C8RA04088E
(Paper)
RSC Adv., 2018,
8, 25961-25965
Copper(I) reagent-promoted hydroxytrifluoromethylation of enamides: flexible synthesis of substituted-3-hydroxy-2-aryl-3-(2,2,2-trifluoro-1-arylethyl)isoindolin-1-one†
Received
14th May 2018
, Accepted 2nd July 2018
First published on 19th July 2018
Abstract
A novel CuBr-catalyzed hydroxytrifluoromethylation reaction was investigated. Substituted 3-benzylidene-2-arylisoindolin-1-ones was reacted with sodium trifluoromethanesulfinate to afford substituted-3-hydroxy-2-aryl-3-(2,2,2-trifluoro-1-arylethyl)isoindolin-1-one. The reaction proceeded at 25 °C in air atmosphere in the absence of base and ligands. Our results indicate that trifluoromethyl free radical tends to attack a double bond rather than aryl in this reaction.
Introduction
Isoindoles are a series of notable nitrogen-containing compounds known for their bioactivity in nature.1 In particular, 3-hydroxyisoindolin-1-ones such as I and II are the core structural motifs of several compounds of medicinal value (Fig. 1). 3-Hydroxy isoindolin-1-ones are known for their use as diuretic and anticancer drugs.2 As substituted 3-benzylidene-2-arylisoindolin-1-ones have double bonds, we try to find a catalytic system for direct hydroxytrifluoromethylation of substituted 3-benzylidene-2-arylisoindolin-1-one.
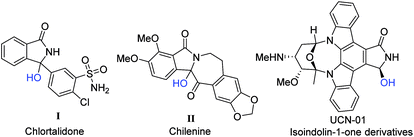 |
| Fig. 1 Bioactive and drug value compounds containing 3-hydroxyisoindolin-1-one motifs. | |
Hydroxytrifluoromethylation of organic molecules has become a research focus in the field of organic synthesis for its unique biological activities.3 In 1991, Langlois and co-workers reported the first use of CF3SO2Na as the trifluoromethyl radical source.4 Since then, a series of trifluoromethylation of olefins by using Langlois reagent has been published in the last twenty years.5 In these reactions, CF3SO2Na was excited by single electron oxidations to generate CF3 free radicals. The oxidative partners included TBHP,6 K2S2O8,7 PhI(OAc)2,8 DTBP,9 I2O5,10 metal (Cu, Mn),11 photoinducers12 and so on. There have only been several examples involving hydroxytrifluoromethylation of olefins to afford useful β-trifluoromethyl alcohols.13 Moreover, there were also studies of the metal-free-catalyzed hydroxytrifluoromethylation reactions of styrenes.14 Recently, manganese-catalyzed direct hydroxytrifluoromethylation reaction of styrene derivatives has been established.15 On the other hand, the CuCF3 system has been used for the synthesis of direct hydroxytrifluoromethylation reaction.16 Visible light promoted C–F functionalization has been developed under mild reaction condition.17 Until now, hydroxytrifluoromethylation of enamides has not been reported. As part of our research on the transition metal-catalyzed free radical reaction of substituted 3-benzylidene-2-arylisoindolin-1-one,18 this communication reports the first example of hydroxytrifluoromethylation reaction of 3-benzylidene-2-arylisoindolin-1-one (the special structure of enamide) with sodium trifluoromethanesulfinate catalyzed by CuBr in the presence of K2S2O8 (Scheme 1).
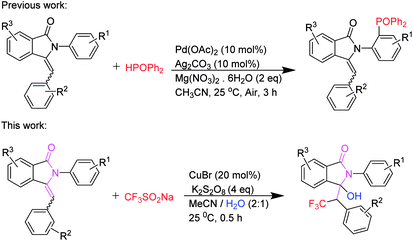 |
| Scheme 1 | |
Results and discussion
When the model reaction of 3-benzylidene-2-phenylisoindolin-1-one (1a) with sodium trifluoromethanesulfinate (2) was performed in CH3CN/H2O in the presence of oxidants such as TBHP, DTBP, Mn(OAc)3 and PhI(OAc)2, no desired products were obtained (Table 1, entries 1, 2, 3 and 4). After the addition of K2S2O8 (4 eq.), the reaction proceeded smoothly to afford the desired product, 3-hydroxy-2-phenyl-3-(2,2,2-trifluoro-1-phenylethyl)isoindolin-1-one (3a) in 48% yield (Table 1, entry 5). Further investigation of copper catalysts, the yield of 3a was improved to 72% when we used CuBr as the catalyst (Table 1, entries 9). On the other hand, when we use FeCl3 in place of CuBr, the reaction afforded the desired product in a lower yield (Table 1, entry 12). By screening polar mixed solvents such as DMSO/H2O DMF/H2O, THF/H2O, acetone/H2O, and a representative nonpolar solvent, toluene (Table 1, entries 15–19), we found that CH3CN/H2O (2
:
1) works best for the reaction. Apart from the above-mentioned factors, the effects of catalyst loading, reaction temperature and time were also investigated, and the optimal reaction conditions were determined to be room temperature reaction for 0.5 h in air atmosphere, with the addition of 20 mol% CuBr as catalyst, K2S2O8 as single electron oxidation regent and CH3CN/H2O as solvent (Table 1, entries 20–27).
Table 1 Optimization of the reaction conditionsa
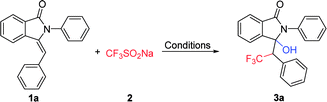
|
Entry |
Catalyst (%) |
Oxidant |
Temperature |
Solvent (66.7%) |
Yieldb |
Reaction conditions: 1a (1 mmol), 2, (3 mmol), CuBr, (0.2 mmol), K2S2O8 (4 mmol), solvent (10 ml), at 25 °C in air atmosphere, 30 min. Yields are given for isolated products. CH3CN/H2O = 5/1. CH3CN/H2O = 1/1. K2S2O8 (5 mmol) was added. K2S2O8 (3 mmol) was added. 1 h. In argon atmosphere. |
1 |
|
TBHP |
25 °C |
CH3CN |
N.D |
2 |
|
DTBP |
25 °C |
CH3CN |
N.D |
3 |
|
Mn(OAc)3 |
25 °C |
CH3CN |
N.D |
4 |
|
PhI(OAc)2 |
25 °C |
CH3CN |
N.D |
5 |
|
K2S2O8 |
25 °C |
CH3CN |
48% |
6 |
CuO (20) |
K2S2O8 |
25 °C |
CH3CN |
30% |
7 |
Cu(OAc)2 (20) |
K2S2O8 |
25 °C |
CH3CN |
55% |
8 |
CuCl (20) |
K2S2O8 |
25 °C |
CH3CN |
60% |
9 |
CuBr (20) |
K2S2O8 |
25 °C |
CH3CN |
72% |
10 |
CuI (20) |
K2S2O8 |
25 °C |
CH3CN |
28% |
11 |
CuBr2 (20) |
K2S2O8 |
25 °C |
CH3CN |
50% |
12 |
FeCl3 (20) |
K2S2O8 |
25 °C |
CH3CN |
45% |
13 |
Ag2CO3 (20) |
K2S2O8 |
25 °C |
CH3CN |
48% |
14 |
CuBr (20) |
K2S2O8 |
25 °C |
CH3CN |
48% |
15 |
CuBr (20) |
K2S2O8 |
25 °C |
DMSO |
6% |
16 |
CuBr (20) |
K2S2O8 |
25 °C |
DMF |
10% |
17 |
CuBr (20) |
K2S2O8 |
25 °C |
THF |
37% |
18 |
CuBr (20) |
K2S2O8 |
25 °C |
Acetone |
55% |
19 |
CuBr (20) |
K2S2O8 |
25 °C |
Toluene |
0% |
20 |
CuBr (20) |
K2S2O8 |
50 °C |
CH3CN |
65% |
21 |
CuBr (20) |
K2S2O8 |
80 °C |
CH3CN |
60% |
22 |
CuBr (20) |
K2S2O8 |
10 °C |
CH3CN |
66% |
23c |
CuBr (20) |
K2S2O8 |
25 °C |
CH3CN |
35% |
23d |
CuBr (20) |
K2S2O8 |
25 °C |
CH3CN |
28% |
23e |
CuBr (20) |
K2S2O8 |
25 °C |
CH3CN |
70% |
24f |
CuBr (20) |
K2S2O8 |
25 °C |
CH3CN |
46% |
25g |
CuBr (20) |
K2S2O8 |
25 °C |
CH3CN |
70% |
26h |
CuBr (20) |
K2S2O8 |
25 °C |
CH3CN |
70% |
27 |
CuBr (10) |
K2S2O8 |
25 °C |
CH3CN |
60% |
With the promising results obtained in the model reaction, we subsequently examined the substrate scope of 3-benzylidene-2-arylisoindolin-1-one under the optimized reaction conditions (20 mol% CuBr as catalyst, and K2S2O8 as oxidant in CH3CN/H2O (2
:
1) at 25 °C, for 0.5 h in air atmosphere).
As shown in Table 2, electron-donating substituents such as methyl and methoxy groups on the aryl ring of substituted 3-benzylidene-2-arylisoindolin-1-one (1) facilitated the reaction to afford the hydroxytrifluoromethylation products (3) in moderate to good yields (Table 2, 65–83%, 3a–3d, 3h–3j, 3o–3p, and 3t). On the contrary, election-withdrawing groups such as F and Cl were unfavorable for the reaction and led to lower yields (Table 2, 34–67%, 3e–3g, 3k–3n and 3r–3s). We also found that when the substrate was 3-pentylidene-2-phenylisoindolin-1-one, the target product (3u) was in 85% yield but diastereomeric ratio is 1
:
1.
Table 2 Scope studies of 3-hydroxy-2-phenyl-3-(2,2,2-trifluoro-1-phenylethyl)isoindolin-1-onea
In order to understand the reaction mechanism, following control experiments were carried out. We repeated the reaction in the presence of radical quencher 2,2,6,6-tetramethylpiperidin-1-oxyl (TEMPO) and none of 3a was obtained (Scheme 2a). The result suggested that free radical were probably generated during the reaction. Furthermore, 3a was also not detected when the reaction was performed with the addition of butylated hydroxytoluene (BHT, 3.0 equiv.) under the standard conditions (Scheme 2b). Trifluoromethylation products was obtained when 1,1-diyldibenzene and Langlois reagent were carried out in standard condition (Scheme 2c). On the other hand, neither aryl amine nor benzylamine substrate produced the ortho-position C–H activated products (Scheme 2d).18 These results indicated that the reaction is only suitable for enamine substrates which have enough electron cloud density.
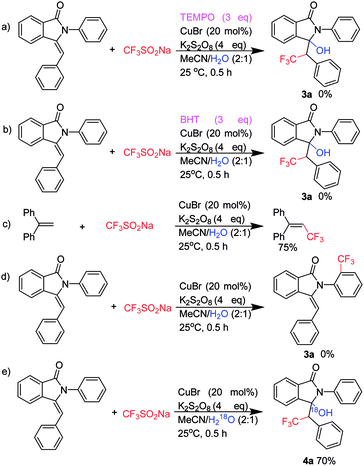 |
| Scheme 2 Control experiments. | |
As the hydroxytrifluoromethylation always took place under argon atmosphere in the above experiments, we wondered whether the reaction would proceed if isotopically labelled molecular H218O was used. Hence, we did further reactions (Scheme 2e). Surprisingly, the corresponding 18O-containing product 4a was obtained in 70%. These results further indicated that the oxygen source of this reaction is derived from H2O rather than oxygen gas.
On the basis of the mechanistic studies and experimental results, a plausible mechanism is proposed in Scheme 3.
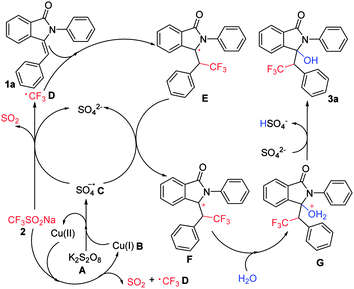 |
| Scheme 3 Proposed reaction mechanism. | |
Initially, the K2S2O8 (A) was excited by Cu(I) (B) to generate the intermediate SO4 radical anions (C), which then reacted with CF3SO2Na (2) to form trifluoromethyl free radical (D). D underwent addition with substrate (1a) to form key radical intermediate E. Thereafter, the radical intermediate E was oxidized by SO4 radical anions (C) which can regenerate SO42− to produce the cation intermediate F. Due to the presence of H2O, the cation intermediate F underwent nucleophilic addition to generated the corresponding intermediate G. The cation intermediate G underwent removing protons to generated the corresponding product 3a and HSO4−. Finally, Cu(II) was reduced to Cu(I) by CF3SO2Na (2) to complete the catalytic cycle.
Conclusions
In summary, we have developed a novel catalytic system for direct hydroxytrifluoromethylation of substituted 3-benzylidene-2-arylisoindolin-1-ones via a radical pathway. The reaction has a high regioselectivity as the CF3 free radical is prone to attacking a double bond rather than the aryl. The method has a broad scope and offers a good yield. The corresponding products are potentially useful in drug discovery.
Conflicts of interest
There are no conflicts to declare.
Acknowledgements
We gratefully acknowledge financial support from the Prospective Study Program of Jiangsu (BY2015039-08), the Project of Scientific and Technologic Infrastructure of Suzhou (SZS201708), and the Priority Academic Program Development of Jiangsu Higher Education Institutions.
Notes and references
- A. K. Mailyan, J. A. Eickhoff, A. S. Minakova, Z. Gu, P. Lu and A. Zakarian, Chem. Rev., 2016, 116, 4441 CrossRef PubMed.
-
(a) F.-G. Fang and S. J. Danishefsky, Tetrahedron Lett., 1989, 30, 2747 CrossRef;
(b) V. Fajardo, V. Elango, B. K. Cassels and M. Shamma, Tetrahedron Lett., 1982, 23, 39 CrossRef.
-
(a) I. Ojima, Fluorine in Medicinal Chemistry and Chemical Biology, Wiley-Blackwell, Chichester, 2009 CrossRef;
(b) M. Shimizu and T. Hiyama, Angew. Chem., Int. Ed., 2005, 44, 214 CrossRef PubMed;
(c) M. Schlosser, Angew. Chem., Int. Ed., 2006, 45, 5432 CrossRef PubMed;
(d) K. Müller, C. Faeh and F. Diederich, Science, 2007, 317, 1881 CrossRef PubMed;
(e) S. Purser, P. R. Moore, S. Swallow and V. Gouverneur, Chem. Soc. Rev., 2008, 37, 320 RSC;
(f) J. Wang, M. Sánchez-Roselló, J. L. Aceña, C. del Pozo, A. E. Sorochinsky, S. Fustero, V. A. Soloshonok and H. Liu, Chem. Rev., 2014, 114, 2432 CrossRef PubMed.
-
(a) B. R. Langlois, E. Laurent and N. Roidot, Tetrahedron Lett., 1992, 33, 1291 CrossRef;
(b) J.-L. Clavel, B. Langlois, E. Laurent and N. Roidot, Phosphorus, Sulfur Silicon Relat. Elem., 1991, 59, 169 CrossRef;
(c) B. R. Langlois, E. Laurent and N. Roidot, Tetrahedron Lett., 1991, 32, 7525 CrossRef.
- For recent examples of difunctionalizing trifluoromethylation of olefins by using Langlois reagent, see:
(a) H.-Y. Zhang, W. Huo, C. Ge, J. Zhao and Y. Zhang, Synlett, 2017, 28, 962 CrossRef;
(b) V. R. Yatham, Y. Shen and R. Martin, Angew. Chem., Int. Ed., 2017, 56, 10915 CrossRef PubMed;
(c) Z. Wu, D. Wang, Y. Liu, L. Huan and C. Zhu, J. Am. Chem. Soc., 2017, 139, 1388 CrossRef PubMed;
(d) Z.-Q. Liu and D. Liu, J. Org. Chem., 2017, 82, 1649 CrossRef PubMed;
(e) W. Kong, H. An and Q.-L. Song, Chem. Commun., 2017, 53, 8968 RSC;
(f) S. Jana, A. Verma, R. Kadu and S. Kumar, Chem. Sci., 2017, 8, 6633 RSC;
(g) J. Fang, Z.-K. Wang, S.-W. Wu, W.-G. Shen, G.-Z. Ao and F. Liu, Chem. Commun., 2017, 53, 7638 RSC;
(h) L. Zhu, L.-S. Wang, B. Li, B. Fu, C.-P. Zhang and W. Li, Chem. Commun., 2016, 52, 6371 RSC;
(i) X.-L. Yu, J.-R. Chen, D.-Z. Chen and W.-J. Xiao, Chem. Commun., 2016, 52, 8275 RSC;
(j) B. Yang, X.-H. Xu and F.-L. Qing, Chin. J. Chem., 2016, 34, 465 CrossRef;
(k) B. Li, D. Fan, C. Yang and W.-J. Xia, Org. Biomol. Chem., 2016, 14, 5293 RSC;
(l) H. Fu, S.-S. Wang and Y.-M. Li, Adv. Synth. Catal., 2016, 358, 3616 CrossRef;
(m) B. Yang, X.-H. Xu, F.-L. Qing and F.-L. Qing, Org. Lett., 2015, 17, 1906 CrossRef PubMed;
(n) L. Zhang, Z. Li and Z.-Q. Liu, Org. Lett., 2014, 16, 3688 CrossRef PubMed;
(o) F. Yang, P. Klumphu, Y.-M. Liang and B. H. Lipshutz, Chem. Commun., 2014, 50, 936 RSC;
(p) W. Wei, J. Wen, D. Yang, X. Liu, M. Guo, R. Dong and H. Wang, J. Org. Chem., 2014, 79, 4225 CrossRef PubMed;
(q) Y. Lu, Y. Li, R. Zhang, K. Jin and C.-Y. Duan, J. Fluorine Chem., 2014, 161, 128 CrossRef;
(r) D. J. Wilger, N. J. Gesmundo and D. A. Nicewicz, Chem. Sci., 2013, 4, 3160 RSC;
(s) X.-Y. Jiang and F.-L. Qing, Angew. Chem., Int. Ed., 2013, 52, 14177 CrossRef PubMed;
(t) Z. Hang, Z. Li and Z. Q. Liu, Org. Lett., 2014, 16, 3648 CrossRef PubMed;
(u) Q. Lefebvre, Synlett, 2017, 28, 19 CrossRef;
(v) C. Zhang, Adv. Synth. Catal., 2014, 356, 2895 CrossRef.
- For recent examples of TBHP activate Langlois reagent to liberate CF3 radicals, see:
(a) d. W. A. van, M. Hribersek and N. Selander, Org. Lett., 2017, 19, 2374 CrossRef PubMed;
(b) Y. A. Konik, M. Kudrjashova, N. Konrad, S. Kaabel, I. Jarving, M. Lopp and D. G. Kananovich, Org. Biomol. Chem., 2017, 15, 4635 RSC;
(c) H. Jiang, W. Huang, Y. Yu, S. Yi, J. Li and W.-Q. Wu, Chem. Commun., 2017, 53, 7473 RSC;
(d) R. C. Simon, E. Busto, V. Resch, W. Kroutil, N. Richter and K. N. Houk, Nat. Commun., 2016, 7, 13323 CrossRef PubMed;
(e) X. Zhang, P. Huang, Y. Li and C. Duan, Org. Biomol. Chem., 2015, 13, 10917 RSC;
(f) K. Zhang, X.-H. Xu and F.-L. Qing, J. Org. Chem., 2015, 80, 7658 CrossRef PubMed;
(g) K. Monir, A. K. Bagdi, M. Ghosh and A. Hajra, J. Org. Chem., 2015, 80, 1332 CrossRef PubMed;
(h) Y.-R. Liu, H.-Y. Tu and X.-G. Zhang, Synthesis, 2015, 47, 3460 CrossRef;
(i) H.-L. Hua, Y.-T. He, Y.-F. Qiu, Y.-X. Li, B. Song, P. Gao, X.-R. Song, D.-H. Guo, X.-Y. Liu and Y.-M. Liang, Chem.–Eur. J., 2015, 21, 1468 CrossRef PubMed;
(j) J. Yin, Y. Li, R. Zhang, K. Jin and C. Duan, Synthesis, 2014, 46, 607 CrossRef;
(k) M. Wu, X. Ji, W. Dai and S. Cao, J. Org. Chem., 2014, 79, 8984 CrossRef PubMed;
(l) J. C. Fennewald and B. H. Lipshutz, Green Chem., 2014, 16, 1097 RSC;
(m) S. R. Dubbaka, S. Nizalapur, A. R. Atthunuri, M. Salla and T. Mathew, Tetrahedron, 2014, 70, 2118 CrossRef;
(n) M. Presset, D. Oehlrich, F. Rombouts and G. A. Molander, J. Org. Chem., 2013, 78, 12837 CrossRef PubMed;
(o) D. Musumeci, C. Irace, R. Santamaria and D. Montesarchio, Med. Chem. Commun., 2013, 4, 1405 RSC;
(p) Z. Li, Z. Cui and Z.-Q. Liu, Org. Lett., 2013, 15, 406 CrossRef PubMed;
(q) Y. Li, L. Wu, H. Neumann and M. Beller, Chem. Commun., 2013, 49, 2628 RSC;
(r) Y. Ye, S. A. Kunzi and M. S. Sanford, Org. Lett., 2012, 14, 4979 CrossRef PubMed;
(s) Y. Ji, T. Brueckl, R. D. Baxter, Y. Fujiwara, I. B. Seiple, S. Su, D. G. Blackmond and P. S. Baran, Proc. Natl. Acad. Sci. U. S. A., 2011, 108, 14411 CrossRef PubMed.
- For recent examples of K2S2O8 activate Langlois reagent to liberate CF3 radicals, see:
(a) D. Wang, J. Fang, G.-J. Deng and H. Gong, ACS Sustainable Chem. Eng., 2017, 5, 6398 CrossRef;
(b) Q. Lu, C. Liu, Z. Huang, Y. Ma, J. Zhang and A.-W. Lei, Chem. Commun., 2014, 50, 14101 RSC;
(c) D. Wang, G.-J. Deng, S. Chen and H. Gong, Green Chem., 2016, 18, 5967 RSC;
(d) C. Shen, J. Xu, B. Ying and P.-F. Zhang, ChemCatChem, 2016, 8, 3560 CrossRef;
(e) H.-L. Huang, H. Yan, G.-L. Gao, C. Yang and W. Xia, Asian J. Org. Chem., 2015, 4, 674 CrossRef;
(f) J. Liu, S. Zhuang, Q. Gui, X. Chen, Z. Yang and Z. Tan, Eur. J. Org. Chem., 2014, 3196 CrossRef;
(g) T. Patra, A. Deb, S. Manna, U. Sharma and D. Maiti, Eur. J. Org. Chem., 2013, 5247 CrossRef;
(h) A. Deb, S. Manna, A. Modak, T. Patra, S. Maity and D. Maiti, Angew. Chem., Int. Ed., 2013, 52, 9747 CrossRef PubMed.
- For recent examples of PhI(OAc)2 activate Langlois reagent to liberate CF3 radicals, see:
(a) Z. Tan, S. Zhang, Y. Zhang, Y. Li, M. Ni and B.-N. Feng, J. Org. Chem., 2017, 82, 9384 CrossRef PubMed;
(b) Z. Wu, Y. He, C. Ma, X. Zhou, X. Liu, Y. Li, T. Hu, P. Wen and G. Huang, Asian J. Org. Chem., 2016, 5, 724 CrossRef;
(c) L. Shi, X. Yang, Y. Wang, H. Yang and H. Fu, Adv. Synth. Catal., 2014, 356, 1021 CrossRef;
(d) X. Xu and F. Liu, Org. Chem. Front., 2017, 4, 2306 RSC.
-
(a) L.-H. Wu, K. Zhao, Z.-L. Shen and T.-P. Loh, Org. Chem. Front., 2017, 4, 1872 RSC;
(b) P. Huang, Y. Li, X. Fu, R. Zhang, K. Jin, W. Wang and C. Duan, Tetrahedron Lett., 2016, 57, 4705 CrossRef.
- X.-J. Shang, Z. Li and Z.-Q. Liu, Tetrahedron Lett., 2015, 56, 233 CrossRef.
-
(a) P.-Z. Zhang, C.-K. Li, G.-Y. Zhang, L. Zhang, Y.-J. Jiang and J.-P. Zou, Tetrahedron, 2016, 72, 3250 CrossRef;
(b) X.-H. Cao, X. Pan, P.-J. Zhou, J.-P. Zou and O. T. Asekun, Chem. Commun., 2014, 50, 3359 RSC;
(c) H.-B. Yang and N. Selander, Org. Biomol. Chem., 2017, 15, 1771 RSC.
- For selected examples of photoinduced Langlois reagent liberate CF3 radicals, see:
(a) S. Corsico, M. Fagnoni and D. Ravelli, Photochem. Photobiol. Sci., 2017, 16, 1375 RSC;
(b) B. Chang, H. Shao, P. Yan, W. Qiu, Z. Weng and R. Yuan, ACS Sustainable Chem. Eng., 2017, 5, 334 CrossRef;
(c) L. Li, X. Mu, W. Liu, Y. Wang, Z. Mi and C.-J. Li, J. Am. Chem. Soc., 2016, 138, 5809 CrossRef PubMed.
- For recent examples of hydroxyltrifluoromethylation of olefins, see:
(a) Y. Yasu, T. Koike and M. Akita, Angew. Chem., Int. Ed., 2012, 51, 9567 CrossRef PubMed;
(b) X.-Z. Luo, H.-Q. Luo, Z.-P. Zhang and W. Dong, Synlett, 2014, 25, 1307 CrossRef;
(c) Y. Yang, Y. Liu, Y. Jiang, Y. Zhang and D. A. Vicic, J. Org. Chem., 2015, 80, 6639 CrossRef PubMed;
(d) C. Liu, Q. Lu, Z. Huang, J. Zhang, F. Liao, P. Peng and A.-W. Lei, Org. Lett., 2015, 17, 6034 CrossRef PubMed;
(e) X. Yang, L. He and G. C. Tsui, Org. Lett., 2017, 19, 2446 CrossRef PubMed.
- X.-Z. Luo, H.-Q. Luo, Z.-P. Zhang and W. Dong, Synlett, 2014, 25, 1307 CrossRef.
- Y. Yang, Y. Liu, Y. Jiang, Y. Zhang and D. A. Vicic, J. Org. Chem., 2015, 80, 6639 CrossRef PubMed.
- X. Yang, L. He and G. C. Tsui, Org. Lett., 2017, 19, 2446 CrossRef PubMed.
- Y. Yasu, T. Koike and M. Akita, Angew. Chem., Int. Ed., 2012, 51, 9567 CrossRef PubMed.
- P. Shi, Q. Wang, X. Zeng, Y.-S. Zhao and R.-S. Zeng, RSC Adv., 2017, 7, 54277 RSC.
Footnote |
† Electronic supplementary information (ESI) available. See DOI: 10.1039/c8ra04088e |
|
This journal is © The Royal Society of Chemistry 2018 |
Click here to see how this site uses Cookies. View our privacy policy here.