DOI:
10.1039/C8RA04790A
(Paper)
RSC Adv., 2018,
8, 27610-27615
N2-selective alkylation of NH-1,2,3-triazoles with vinyl ethers via gold catalysis†
Received
5th June 2018
, Accepted 4th July 2018
First published on 2nd August 2018
Abstract
A new method was developed to synthesize N2-alkyl-substituted 1,2,3-triazoles via gold catalyzed alkylation of vinyl ethers with mono- and unsubstituted NH-1,2,3-triazoles and benzotriazole. A hydrogen bond between the oxygen atom of the vinyl ethers, activated via the gold catalyst, and the NH-1,2,3-triazoles was supposed to be generated, which selectively gave the N2-alkylation products.
Introduction
Substituted 1,2,3-triazoles are among the most important N-heterocyclic compounds and display a broad spectrum of applications in the areas of medicinal chemistry, agrochemicals, and materials science.1 1,2,3-Triazoles containing heterocyclic compounds serve as intermediates for the synthesis of biologically active compounds.2 Both thermal and Cu(I)/Ru(II)- catalyzed condensations of alkynes and azides provide an excellent approach to N1-substituted triazoles.3 However, the synthesis of N2-substituted triazoles remains a challenge.4,5 A particularly interesting subset of these compounds are N2-alkyl-1,2,3-triazoles, which possess a broad spectrum of antiherpetic, antiarrhythmic and antiviral activities.6 Ideally, the most direct route to N2-alkyl-1,2,3-triazoles involves nucleophilic substitution of alkylating reactants with NH-1,2,3-triazoles, however, a mixture of the isomeric products is often produced.7 Recently, the N2-selective alkylation reactions of alkyl halides with 1,2,3-triazoles have been achieved by incorporating C-4- and C-5-substituents on the heteroarenes, of which the synthetic utility was thus restricted by the substrate's steric requirements. Another route to the N2-alkyl-1,2,3-triazoles, addition of 1-sulfonyl-1,2,3-triazoles to olefins or vinyl ethers catalyzed by TsOH or gold catalyst, was reported by Chen and our group.8 Very recently, Chen and co-workers reported the highly N2-selective alkylation reactions of NH-1,2,3-triazoles with olefins mediated by NIS,9 in which the favored N1-type intermolecular hydrogen bonding connection during the transition state provides the coupling adduct with a high N2-selectively (Scheme 1a). Based on our previous report of gold catalyzed N2 alkylation of 1-sulfonyl-1,2,3-triazoles with vinyl ethers,8b we hope to extend this reaction involving the use of NH-1,2,3-triazoles as nucleophiles, in which the hydrogen bond between the oxygen atom of vinyl ethers activated via gold catalyst with NH-1,2,3-triazoles produces N2-alkyl-1,2,3-triazoles (Scheme 1b).
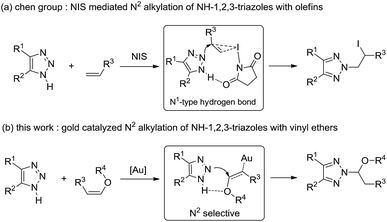 |
| Scheme 1 N2 alkylation of NH-1,2,3-triazoles. | |
Results and discussion
Based on our previous results with respect to the N2-selective gold-catalyzed alkylation of 1-sulfonyl-1,2,3-triazoles,8b we began our studies by exploring the reaction between NH-1,2,3-triazole (1a) and vinyl ether (2a). The optimization results are summarized in Table 1. To our delight, the desired N2-alkyl-1,2,3-triazole 3a was obtained in 95% yield in the presence of Ph3PAuCl (5 mol%)/AgNTf2 (5 mol%) in 1,2-dichloroethane (DCE) at 80 °C, without a N1-coupling adduct (Table 1, entry 1). In order to optimize the reaction conditions, different silver salts were screened, of which Ph3PAuCl/AgNTf2 was found to be the best silver combination (Table 1, entries 2 and 3). The catalyst ligands were then evaluated. The N-heterocyclic carbene ligand IPrAuCl showed moderate activity, while the phosphine ligand JohnphosAuCl was not favored in this transformation, affording 3a in only 30% yield (Table 1, entries 4 and 5). Further solvent optimization identified DCE to be the best reaction medium (Table 1, entries 6–9). The addition of 4 Å molecular sieves resulted in a decreased conversion into 3a in 69% yield after 22 hours (Table 1, entry 10), due to the possible gold catalyst poisons contained in molecular sieves.10 Variation of the number of equivalents of 2a from 3.0 to 2.0 lowed the conversion of 3a to 65% (Table 1, entry 11), which indicates that the excess of vinyl ether probably is necessary due to the high tendency of vinyl ethers to undergo cationic polymerization initiated by gold(I).11 Decreasing the catalyst loading to 2 mol% led to reduced reaction yield of 72% (Table 1, entry 12). In the control experiments, employing Ph3PAuCl and AgNTf2 separately gave none of the desired product (Table 1, entries 12 and 13) even after 24 hours.
Table 1 Condition optimization for the reaction of 1a with 2aa

|
Entry |
Cat. (mol%) |
Solvent |
Time (h) |
Yieldb (%) |
Unless noted, all reactions were carried out at the 0.1 mmol scale in 3 mL solvent with the addition of 5 mol% catalyst (1a/2a = 1/3) at 80 °C, NR = no reaction. Isolated yield. 100 mg 4 Å MS was added. 2 Equiv. 2a was added. No consumption of starting material 1a was seen after 24 h. |
1 |
Ph3PAuCl/AgNTf2 (5) |
DCE |
6 |
95 |
2 |
Ph3PAuCl/AgOTf (5) |
DCE |
6 |
81 |
3 |
Ph3PAuCl/AgSbF6 (5) |
DCE |
6 |
75 |
4 |
IPrAuCl/AgNTf2 (5) |
DCE |
6 |
79 |
5 |
JohnphosAuCl/AgNTf2 (5) |
DCE |
6 |
30 |
6 |
Ph3PAuCl/AgNTf2 (5) |
DCM |
6 |
34 |
7 |
Ph3PAuCl/AgNTf2 (5) |
CHCl3 |
6 |
24 |
8 |
Ph3PAuCl/AgNTf2 (5) |
CH3CN |
6 |
60 |
9 |
Ph3PAuCl/AgNTf2 (5) |
Toluene |
6 |
71 |
10c |
Ph3PAuCl/AgNTf2 (5) |
DCE |
22 |
69 |
11d |
Ph3PAuCl/AgNTf2 (5) |
DCE |
24 |
65 |
12 |
Ph3PAuCl/AgNTf2 (2) |
DCE |
24 |
72 |
13e |
Ph3PAuCl (5) |
DCE |
24 |
NR |
14e |
AgNTf2 (5) |
DCE |
24 |
NR |
With the optimized reaction conditions in hand, we examined the scope of this transformation by synthesizing a series N2-alkyl-1,2,3-triazoles. As shown in Table 2, various NH-1,2,3-triazoles were explored by using vinyl ether 2a as the reactants. First, various 4-aryl substituted NH-1,2,3-triazoles were explored. The NH-1,2,3-triazoles containing electron-donating functional groups, such as methyl, t-butyl, and methoxy, at the para position of the aromatic ring gave comparably good yields (80–87%) of the desired N2-alkyl-1,2,3-triazoles. However, 4-halogen phenyl substituted NH-1,2,3-triazoles gave the corresponding products in moderate yields, in which the more electron deficient triazole rings reduced their nucleophilicities. The reaction of 2-thienyl substituted NH-1,2,3-triazoles went smoothly, affording 3h in 77% yield. Moreover, 4-alkyl substituted NH-1,2,3-triazoles 1i and unsubstituted NH-1,2,3-triazole 1j could also provide the corresponding N2-alky-substituted 1,2,3-triazoles 3i and 3j in moderate yields. Benzotriazole 1k was also tested, giving 3k in 89% yield.
Table 2 Variation of the NH-1,2,3-triazoles 1a,b

|
Unless noted, all reactions were carried out at the 0.1 mmol scale in 3 mL solvent with the addition of 5 mol% catalyst (1/2a = 1/3) at 80 °C. Isolated yield. |
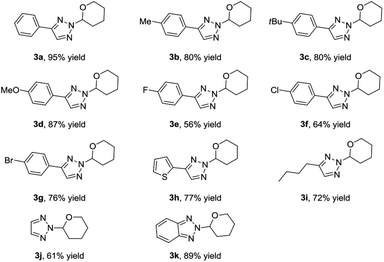 |
We next set out to explore the scope of vinyl ethers 2 in the presence of 4-phenyl substituted 1,2,3-triazol 1a (Table 3). First, cyclic vinyl ether 2,3-dihydrofuran 2b worked very well, giving 4b in 74% yield. Next, we investigated the linear vinyl ether's reactions. We found that mono-substituted and 1,2-disubstituted linear vinyl ether could be employed in this reaction and gave the desired products in good yields. Moreover, 1,1-disubstituted vinyl ether 2j could also provide the desired N2-alkyl-1,2,3-triazole 4j in 77% yield. This reaction was also efficient with alpha-angelica lactone, giving 4k in 93% yield.
Table 3 Variation of the vinyl ethers 2a,b

|
Unless noted, all reactions were carried out at the 0.1 mmol scale in 3 mL solvent with the addition of 5 mol% catalyst (1a/2 = 1/3) at 80 °C. Isolated yield. |
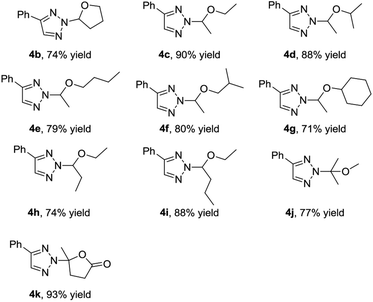 |
In order to understand the mechanism for the regioselective synthesis of N2-alkyl-1,2,3-triazoles, we performed two control experiments. First, we replaced vinyl ether with 4-tert-butylstyrene in the model reaction, no product was obtained. This may be due to the lower complexation of olefin with gold (Scheme 2(1)). Then, we performed this reaction in the presence of phenylacetylene, N1-vinyl substituted 1,2,3-triazole 7 was obtained in 70% yield, which was in accordance with our previous report (Scheme 2(2)).12 The two control experiments suggested that the N2-regioselectivity may be induced by the substrate vinyl ethers in this reaction.
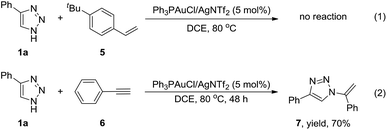 |
| Scheme 2 Control experiments. | |
Based on our experimental results and precedents in the literature, a proposed catalytic cycle for the N2-selective alkylation is presented in Scheme 3. Initial complexation of gold could occur via pathway A8a or B.13 Pathway A shows a one-step process involving the nucleophilic attack of the internal nitrogen of the NH-1,2,3-triazole 1a on Au(I)-coordinated vinyl ether 2a through the hydrogen bond interaction to form the alkyl gold intermediate. This subsequently undergoes protodemetalation14 giving the N2-alkyl-1,2,3-triazole 3a. Pathway B shows a two-step process invoking a triazole–Au complex, which then activated vinyl ether and subsequently undergoes N2-selective alkylation with the internal nitrogen of the NH-1,2,3-triazole 1a to give the alkyl gold intermediate. This subsequently undergoes protodemetalation14 giving the N2-alkyl-1,2,3-triazole 3a. However, pathway B might be unfavorable because the N2-vinyl substituted 1,2,3-triazole could not be observed in our control experiment (Scheme 2(2)).
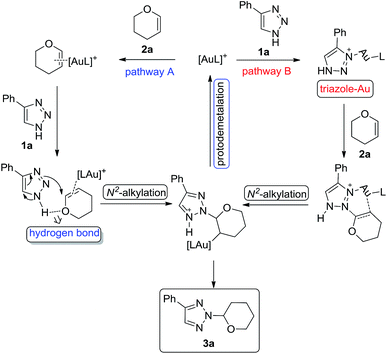 |
| Scheme 3 Proposed catalytic cycle for the N2-selective alkylation. | |
Conclusions
In summary, we have developed a new method to synthesis N2-alkyl-1,2,3-triazoles through gold catalyzed alkylation of vinyl ethers with NH-1,2,3-triazoles. The reaction is facile, high yielding and regioselective. The regioselectivity may be induced by the hydrogen bond between the oxygen atom of vinyl ethers with NH-1,2,3-triazole. The potential utilization and extension of this interesting synthetic methodology are currently underway.
Experimental
General methods
All reactions were performed using Schlenk-tubes, septa, and syringes under nitrogen atmosphere. Commercial reagents were used as supplied or purified by standard techniques where necessary. Column chromatography was performed using 200–300 mesh silica with the proper solvent system according to TLC analysis using KMnO4 stain and UV light to visualize the reaction components. Unless otherwise noted, nuclear magnetic resonance spectra were recorded on a Agilent 400 MHz spectrometer using CDCl3 with TMS as internal reference at room temperature. NMR data were reported as follows: chemical shift, multiplicity (s = singlet, d = doublet, t = triplet, m = multiplet and bs = broad singlet), coupling constant in Hz and integration. Chemical shifts for 13C NMR spectra were recorded in parts per million from tetramethylsilane using the central peak of deuterochloroform (77.0 ppm) as the internal standard. Melting points were determined on a WRS-1B digital melting point instrument. IR spectra were obtained on Nicoletisso FTIR using KBr disks. HR-MS spectral were determined on a Bruker Daltonics Bio TOF mass spectrometer. NH-1,2,3-triazoles 1a–1m were prepared according to the published methods.14 Vinyl ethers were obtained commercially and used without further purification.
General procedure for synthesis of compound 3a
To a Schlenk tube charged with nitrogen was added Ph3PAuCl/AgNTf2 (5 mol%) in dry DCE (3 mL). After three minutes, NH-1,2,3-triazole 1a (0.1 mmol), vinyl ether 2a (0.3 mmol) were added to the reaction. Then the reaction mixture was stirred at 80 °C for 6 h until complete consumption of starting material as monitored by TLC. Concentration of the reaction mixture in vacuo followed by purification through flash chromatography on silica gel column (hexane/EtOAc = 30/1) afforded N2-alkyl-substituted 1,2,3-triazole 3a.
4-Phenyl-2-(tetrahydro-2H-pyran-2-yl)-2H-1,2,3-triazole (3a). The spectroscopic data are in accordance with those reported.8bYield: 21.8 mg (95%); yellow oil liquid; Rf = 0.20 (hexane–EtOAc, 10
:
1). IR (neat): 3475, 2951, 1611, 1391, 1063 cm−1. 1H NMR (400 MHz, CDCl3): δ 7.92 (s, 1H), 7.82 (d, J = 7.7 Hz, 2H), 7.42 (t, J = 7.4 Hz, 2H), 7.35 (t, J = 6.9 Hz, 1H), 5.75 (d, J = 8.8 Hz, 1H), 4.08 (d, J = 11.3 Hz, 1H), 3.77 (t, J = 10.4 Hz, 1H), 2.52–2.45 (m, 1H), 2.15–2.10 (m, 2H), 1.74–1.72 (m, 2H), 1.69–1.63 (m, 1H). 13C NMR (100 MHz, CDCl3): δ 148.1, 131.5, 130.1, 128.8, 128.5, 126.1, 89.1, 67.5, 29.4, 24.7, 21.8.
2-(Tetrahydro-2H-pyran-2-yl)-4-(p-tolyl)-2H-1,2,3-triazole (3b). The spectroscopic data are in accordance with those reported.8bYield: 19.4 mg (80%); colorless oil liquid; Rf = 0.20 (hexane–EtOAc, 10
:
1). IR (neat): 3466, 2955, 1645, 1398, 1070 cm−1. 1H NMR (400 MHz, CDCl3): δ 7.89 (s, 1H), 7.71 (d, J = 8.0 Hz, 2H), 7.23 (d, J = 7.9 Hz, 2H), 5.73 (dd, J = 9.3, 2.4 Hz, 1H), 4.08 (d, J = 11.4 Hz, 1H), 3.80–3.73 (m, 1H), 2.54–2.44 (m, 1H), 2.38 (s, 3H), 2.17–2.08 (m, 2H), 1.78–1.72 (m, 2H), 1.68–1.62 (m, 1H). 13C NMR (100 MHz, CDCl3): δ 148.2, 138.5, 131.4, 129.5, 127.2, 125.9, 89.1, 77.3, 29.4, 24.8, 21.9, 21.3.
4-(4-(tert-Butyl)phenyl)-2-(tetrahydro-2H-pyran-2-yl)-2H-1,2,3-triazole (3c). The spectroscopic data are in accordance with those reported.8bYield: 22.8 mg (80%); colorless oil liquid; Rf = 0.20 (hexane–EtOAc, 10
:
1). IR (neat): 3470, 2970, 1644, 1490, 1092 cm−1. 1H NMR (400 MHz, CDCl3): δ 7.88 (s, 1H), 7.74 (d, J = 8.4 Hz, 2H), 7.44 (d, J = 8.3 Hz, 2H), 5.77–5.70 (m, 1H), 4.06 (d, J = 11.4 Hz, 1H), 3.76 (t, J = 9.7 Hz, 1H), 2.51–2.43 (m, 1H), 2.14–2.08 (m, 2H), 1.75–1.71 (m, 2H), 1.67–1.62 (m, 1H), 1.33 (s, 9H). 13C NMR (100 MHz, CDCl3): δ 151.7, 148.1, 131.5, 127.3, 125.9, 125.7, 89.1, 67.5, 34.7, 31.3, 29.4, 24.8, 21.9.
4-(4-Methoxyphenyl)-2-(tetrahydro-2H-pyran-2-yl)-2H-1,2,3-triazole (3d). The spectroscopic data are in accordance with those reported.8bYield: 22.6 mg (87%); yellow oil liquid; Rf = 0.10 (hexane–EtOAc, 10
:
1). IR (neat): 3467, 2954, 1631, 1396, 1058 cm−1. 1H NMR (400 MHz, CDCl3): δ 7.83 (s, 1H), 7.73 (d, J = 8.5 Hz, 2H), 6.94 (d, J = 8.7 Hz, 2H), 5.71 (dd, J = 9.5, 2.6 Hz, 1H), 4.06 (d, J = 11.5 Hz, 1H), 3.83 (s, 3H), 3.74 (t, J = 11.2, 1H), 2.50–2.43 (m, 1H), 2.14–2.07 (m, 2H), 1.75–1.70 (m, 2H), 1.68–1.63 (m, 1H). 13C NMR (100 MHz, CDCl3): δ 159.9, 148.0, 131.1, 127.4, 122.8, 114.2, 89.1, 67.5, 55.3, 29.4, 24.8, 21.9.
4-(4-Fluorophenyl)-2-(tetrahydro-2H-pyran-2-yl)-2H-1,2,3-triazole (3e). The spectroscopic data are in accordance with those reported.8bYield: 13.8 mg (56%); colorless oil liquid; Rf = 0.20 (hexane–EtOAc, 10
:
1). IR (neat): 3477, 2959, 1617, 1397, 1094 cm−1. 1H NMR (400 MHz, CDCl3): δ 7.86 (s, 1H), 7.80–7.75 (m, 2H), 7.10 (t, J = 8.6 Hz, 2H), 5.72 (dd, J = 9.1, 2.0 Hz, 1H), 4.06 (d, J = 11.4 Hz, 1H), 3.75 (t, J = 10.7 Hz, 1H), 2.49–2.42 (m, 1H), 2.13–2.07 (m, 2H), 1.74–1.71 (m, 2H), 1.68–1.63 (m, 1H). 13C NMR (100 MHz, CDCl3): δ 162.9 (d, J = 246.5 Hz), 147.3, 131.3, 127.9 (d, J = 8.2 Hz), 126.4, 115.8 (d, J = 21.6 Hz), 89.2, 67.5, 29.4, 24.8, 21.9.
4-(4-Chlorophenyl)-2-(tetrahydro-2H-pyran-2-yl)-2H-1,2,3-triazole (3f). The spectroscopic data are in accordance with those reported.8bYield: 16.8 mg (64%); yellow oil liquid; Rf = 0.20 (hexane–EtOAc, 10
:
1). IR (neat): 3475, 2971, 1645, 1393, 1093 cm−1. 1H NMR (400 MHz, CDCl3): δ 7.90 (s, 1H), 7.76 (d, J = 8.3 Hz, 2H), 7.40 (d, J = 8.2 Hz, 2H), 5.77–5.72 (m, 1H), 4.08 (d, J = 11.8 Hz, 1H), 3.79 (d, J = 9.9 Hz, 1H), 2.51–2.43 (m, 1H), 2.16–2.09 (m, 2H), 1.77–1.72 (m, 2H), 1.69–1.66 (m, 1H). 13C NMR (100 MHz, CDCl3): δ 147.1, 134.4, 131.5, 129.0, 128.7, 127.3, 89.2, 67.5, 29.4, 24.8, 21.8.
4-(4-Bromophenyl)-2-(tetrahydro-2H-pyran-2-yl)-2H-1,2,3-triazole (3g). The spectroscopic data are in accordance with those reported.8bYield: 17.7 mg (76%); colorless oil liquid; Rf = 0.20 (hexane–EtOAc, 10
:
1). IR (neat): 3470, 2948, 1645, 1387, 1066 cm−1. 1H NMR (400 MHz, CDCl3): δ 7.93 (s, 1H), 7.72 (d, J = 8.4 Hz, 2H), 7.58 (d, J = 8.3 Hz, 2H), 5.79–5.74 (m, 1H), 4.10 (d, J = 11.7 Hz, 1H), 3.79 (d, J = 9.9 Hz 1H), 2.53–2.45 (m, 1H), 2.18–2.12 (m, 2H), 1.78–1.71 (m, 2H), 1.72–1.67 (m, 1H). 13C NMR (100 MHz, CDCl3): δ 147.1, 131.9, 131.5, 129.1, 127.6, 122.6, 89.2, 67.5, 29.4, 24.8, 21.8.
2-(Tetrahydro-2H-pyran-2-yl)-4-(thiophen-2-yl)-2H-1,2,3-triazole (3h). The spectroscopic data are in accordance with those reported.8bYield: 18.1 mg (77%); colorless oil liquid; Rf = 0.20 (hexane–EtOAc, 10
:
1). IR (neat): 3470, 2951, 1648, 1392, 1075 cm−1. 1H NMR (400 MHz, CDCl3): δ 7.80 (s, 1H), 7.64 (d, J = 1.4 Hz, 1H), 7.47 (d, J = 5.0 Hz, 1H), 7.39–7.34 (m, 1H), 5.71 (dd, J = 9.0, 1.9 Hz, 1H), 4.06 (d, J = 11.4 Hz, 1H), 3.74 (t, J = 10.6 Hz, 1H), 2.49–2.42 (m, 1H), 2.13–2.06 (m, 2H), 1.74–1.72 (m, 2H), 1.69–1.63 (m, 1H). 13C NMR (100 MHz, CDCl3): δ 144.3, 131.7, 131.5, 126.4, 126.0, 122.0, 89.1, 67.5, 29.4, 24.8, 21.9.
4-Butyl-2-(tetrahydro-2H-pyran-2-yl)-2H-1,2,3-triazole (3i). Yield: 15.1 mg (72%); colorless oil liquid; Rf = 0.20 (hexane–EtOAc, 10
:
1). IR (neat): 3464, 2931, 1658, 1266, 1092 cm−1. 1H NMR (400 MHz, CDCl3): δ 7.36 (s, 1H), 5.55 (dd, J = 9.5, 1.6 Hz, 1H), 3.98 (d, J = 11.6 Hz, 1H), 3.65 (t, J = 10.7 Hz, 1H), 2.62 (t, J = 7.8 Hz, 2H), 2.33 (dd, J = 20.2, 9.8 Hz, 1H), 2.00 (t, J = 14.0 Hz, 2H), 1.68–1.52 (m, 5H), 1.31 (dd, J = 14.9, 7.4 Hz, 2H), 0.86 (t, J = 7.3 Hz, 3H). 13C NMR (100 MHz, CDCl3): δ 149.3, 133.2, 88.8, 67.6, 31.2, 29.4, 25.2, 24.8, 22.3, 22.1, 13.8. HRMS (ESI): m/z [M + H]+ calcd for C11H20N3O+: 210.1601; found: 210.1599.
2-(Tetrahydro-2H-pyran-2-yl)-2H-1,2,3-triazole (3j). Yield: 9.3 mg (61%); colorless oil liquid; Rf = 0.50 (hexane–EtOAc, 10
:
1). IR (neat): 3454, 2954, 1661, 1358, 1095 cm−1. 1H NMR (400 MHz, CDCl3): δ 7.68 (s, 2H), 5.73 (d, J = 9.1 Hz, 1H), 4.04 (d, J = 11.5 Hz, 1H), 3.77–3.71 (m, 1H), 2.48–2.39 (m, 1H), 2.09–2.06 (m, 2H), 1.73–1.65 (m, 3H). 13C NMR (100 MHz, CDCl3): δ 134.6, 88.9, 67.4, 29.4, 24.7, 21.8. HRMS (ESI): m/z [M + H]+ calcd for C7H12N3O+: 154.0975; found: 154.0968.
2-(Tetrahydro-2H-pyran-2-yl)-2H-benzo[d][1,2,3]triazole (3k). Yield: 18.1 mg (89%); colorless oil liquid; Rf = 0.10 (hexane–EtOAc, 10
:
1). IR (neat): 3467, 2940, 1664, 1274, 1086 cm−1. 1H NMR (400 MHz, CDCl3): δ 8.07 (d, J = 8.4 Hz, 1H), 7.74 (d, J = 8.3 Hz, 1H), 7.49 (t, J = 7.6 Hz, 1H), 7.38 (t, J = 7.7 Hz, 1H), 6.04 (dd, J = 8.2, 2.7 Hz, 1H), 3.95 (dd, J = 11.7, 4.6 Hz, 1H), 3.83–3.75 (m, 1H), 2.68–2.57 (m, 1H), 2.23–2.19 (m, 1H), 1.90–1.71 (m, 4H) 13C NMR (100 MHz, CDCl3): δ 127.4, 124.1, 119.9, 111.1, 85.6, 66.8, 29.3, 24.9, 21.6. HRMS (ESI): m/z [M + H]+ calcd for C11H14N3O+: 204.1131; found: 204.1130.
4-Phenyl-2-(tetrahydrofuran-2-yl)-2H-1,2,3-triazole (4b). The spectroscopic data are in accordance with those reported.8bYield: 15.9 mg (74%); colorless oil liquid; Rf = 0.20 (hexane–EtOAc, 10
:
1). IR (neat): 3474, 2979, 1645, 1381, 1074 cm−1. 1H NMR (400 MHz, CDCl3): δ 7.97 (s, 1H), 7.89 (d, J = 7.4 Hz, 2H), 7.52 (t, J = 7.6 Hz, 2H), 7.45 (t, J = 7.3 Hz, 1H), 6.43 (dd, J = 6.7, 2.3 Hz, 1H), 4.30 (dd, J = 14.1, 7.6 Hz, 1H), 4.16 (dd, J = 14.0, 7.7 Hz, 1H), 2.83–2.76 (m, 1H), 2.59–2.48 (m, 2H), 2.25–2.16 (m, 1H). 13C NMR (100 MHz, CDCl3): δ 148.0, 131.4, 130.3, 128.8, 128.5, 126.0, 92.3, 69.6, 31.4, 24.5.
2-(1-Ethoxyethyl)-4-phenyl-2H-1,2,3-triazole (4c). The spectroscopic data are in accordance with those reported.8bYield: 19.5 mg (90%); colorless oil liquid; Rf = 0.40 (hexane–EtOAc, 10
:
1). IR (neat): 3466, 3001, 1650, 1357, 1125 cm−1. 1H NMR (400 MHz, CDCl3): δ 8.07 (s, 1H), 7.98 (d, J = 7.1 Hz, 2H), 7.59 (t, J = 7.4 Hz, 2H), 7.51 (t, J = 7.4 Hz, 1H), 5.96 (q, J = 6.0 Hz, 1H), 3.75–3.68 (m, 1H), 3.58–3.50 (m, 1H), 2.00 (d, J = 6.0 Hz, 3H), 1.32 (t, J = 7.0 Hz, 3H). 13C NMR (100 MHz, CDCl3): δ 147.8, 131.3, 130.3, 128.8, 128.5, 126.0, 89.5, 64.6, 20.9, 14.7.
2-(1-Isopropoxyethyl)-4-phenyl-2H-1,2,3-triazole (4d). The spectroscopic data are in accordance with those reported.8bYield: 20.3 mg (88%); colorless oil liquid; Rf = 0.50 (hexane–EtOAc, 10
:
1). IR (neat): 3473, 2972, 1649, 1375, 1118 cm−1. 1H NMR (400 MHz, CDCl3): δ 8.02 (s, 1H), 7.95 (d, J = 7.1 Hz, 2H), 7.55 (t, J = 7.5 Hz, 2H), 7.47 (t, J = 7.4 Hz, 1H), 6.03 (q, J = 6.0 Hz, 1H), 3.87–3.77 (m, 1H), 1.94 (d, J = 6.0 Hz, 3H), 1.36 (d, J = 6.1 Hz, 3H), 1.07 (d, J = 6.2 Hz, 3H). 13C NMR (100 MHz, CDCl3): δ 147.7, 131.2, 130.3, 128.8, 128.5, 126.0, 87.4, 70.4, 22.7, 21.4, 21.3.
2-(1-Butoxyethyl)-4-phenyl-2H-1,2,3-triazole (4e). The spectroscopic data are in accordance with those reported.8bYield: 19.4 mg (79%); colorless oil liquid; Rf = 0.50 (hexane–EtOAc, 10
:
1). IR (neat): 3475, 2956, 1634, 1381, 1129 cm−1. 1H NMR (400 MHz, CDCl3): δ 7.89 (s, 1H), 7.81 (d, J = 7.1 Hz, 2H), 7.42 (t, J = 7.4 Hz, 2H), 7.34 (t, J = 7.3 Hz, 1H), 5.77 (q, J = 6.0 Hz, 1H), 3.49 (dt, J = 9.2, 6.7 Hz, 1H), 3.31 (dt, J = 9.4, 6.6 Hz, 1H), 1.82 (d, J = 6.0 Hz, 3H), 1.53–1.45 (m, 2H), 1.33–1.24 (m, 2H), 0.83 (t, J = 7.4 Hz, 3H). 13C NMR (100 MHz, CDCl3): δ 147.8, 131.2, 130.3, 128.8, 128.5, 126.0, 89.7, 68.9, 31.2, 20.8, 19.1, 13.7.
2-(1-Isobutoxyethyl)-4-phenyl-2H-1,2,3-triazole (4f). The spectroscopic data are in accordance with those reported.8bYield: 19.6 mg (80%); colorless oil liquid; Rf = 0.50 (hexane–EtOAc, 10
:
1). IR (neat): 3468, 2959, 1648, 1381, 1126 cm−1. 1H NMR (400 MHz, CDCl3): δ 7.89 (s, 1H), 7.81 (d, J = 7.1 Hz, 2H), 7.42 (t, J = 7.4 Hz, 2H), 7.34 (t, J = 7.4 Hz, 1H), 5.76 (q, J = 6.0 Hz, 1H), 3.26 (dd, J = 9.2, 6.9 Hz, 1H), 3.08 (dd, J = 9.2, 6.6 Hz, 1H), 1.82 (d, J = 6.0 Hz, 3H), 1.78 (dd, J = 13.4, 6.7 Hz, 1H), 0.85 (d, J = 6.7 Hz, 3H), 0.80 (d, J = 6.7 Hz, 3H). 13C NMR (100 MHz, CDCl3): δ 147.7, 131.2, 130.4, 128.8, 128.5, 126.0, 89.9, 75.8, 28.2, 20.8, 19.2, 19.1.
2-(1-(Cyclohexyloxy)ethyl)-4-phenyl-2H-1,2,3-triazole (4g). The spectroscopic data are in accordance with those reported.8bYield: 19.3 mg (71%); colorless oil liquid; Rf = 0.50 (hexane–EtOAc, 10
:
1). IR (neat): 3465, 2948, 1644, 1383, 1102 cm−1. 1H NMR (400 MHz, CDCl3): δ 7.88 (s, 1H), 7.81 (d, J = 7.1 Hz, 2H), 7.42 (t, J = 7.4 Hz, 2H), 7.34 (t, J = 7.4 Hz, 1H), 5.93 (q, J = 6.0 Hz, 1H), 3.40–3.34 (m, 1H), 2.00–1.98 (m, 1H), 1.80 (d, J = 6.0 Hz, 3H), 1.71–1.70 (m, 1H), 1.63–1.56 (m, 1H), 1.48–1.33 (m, 3H), 1.23–1.10 (m, 4H). 13C NMR (100 MHz, CDCl3): δ 147.7, 131.1, 130.4, 128.8, 128.5, 126.0, 87.2, 76.2, 32.7, 31.4, 25.5, 24.0, 23.9, 21.4.
2-(1-Ethoxypropyl)-4-phenyl-2H-1,2,3-triazole (4h). The spectroscopic data are in accordance with those reported.8bYield: 17.1 mg (74%); colorless oil liquid; Rf = 0.50 (hexane–EtOAc, 10
:
1). IR (neat): 3465, 2963, 1650, 1381, 1107 cm−1. 1H NMR (400 MHz, CDCl3): δ 7.90 (s, 1H), 7.82 (d, J = 7.1 Hz, 2H), 7.42 (t, J = 7.4 Hz, 2H), 7.34 (t, J = 7.4 Hz, 1H), 5.52 (t, J = 6.7 Hz, 1H), 3.57–3.50 (m, 1H), 3.43–3.36 (m, 1H), 2.21 (p, J = 7.4 Hz, 2H), 1.15 (t, J = 7.0 Hz, 3H), 0.88 (t, J = 7.5 Hz, 3H). 13C NMR (100 MHz, CDCl3): δ 147.9, 131.3, 130.3, 128.8, 128.5, 126.0, 94.5, 64.7, 28.0, 14.7, 9.0.
2-(1-Ethoxybutyl)-4-phenyl-2H-1,2,3-triazole (4i). The spectroscopic data are in accordance with those reported.8bYield: 21.6 mg (88%); colorless oil liquid; Rf = 0.50 (hexane–EtOAc, 10
:
1). IR (neat): 3469, 2962, 1647, 1381, 1112 cm−1. 1H NMR (400 MHz, CDCl3): δ 7.89 (s, 1H), 7.81 (d, J = 7.2 Hz, 2H), 7.42 (t, J = 7.4 Hz, 2H), 7.34 (t, J = 7.3 Hz, 1H), 5.60 (t, J = 6.7 Hz, 1H), 3.57–3.49 (m, 1H), 3.43–3.35 (m, 1H), 2.19–2.147 (m, 2H), 1.45–1.35 (m, 1H), 1.28–1.21 (m, 1H), 1.15 (t, J = 7.0 Hz, 3H), 0.93 (t, J = 7.4 Hz, 3H). 13C NMR (100 MHz, CDCl3): δ 147.8, 131.3, 130.3, 128.8, 128.5, 126.0, 93.0, 64.7, 36.7, 18.0, 14.7, 13.5.
2-(2-Ethoxypropan-2-yl)-4-phenyl-2H-1,2,3-triazole (4j). Yield: 16.7 mg (77%); colorless oil liquid; Rf = 0.30 (hexane–EtOAc, 10
:
1). IR (neat): 3442, 2940, 1661, 1384, 1072 cm−1. 1H NMR (400 MHz, CDCl3): δ 7.92 (s, 1H), 7.84 (d, J = 7.2 Hz, 2H), 7.44 (t, J = 7.5 Hz, 2H), 7.37 (d, J = 7.4 Hz, 1H), 3.10 (s, 3H), 1.98 (s, 6H). 13C NMR (100 MHz, CDCl3): δ 147.5, 131.0, 128.8, 128.4, 126.0, 93.0, 50.8, 25.7. HRMS (ESI): m/z [M + H]+ calcd for C12H16N3O+: 218.1288; found: 218.1289.
5-Methyl-5-(4-phenyl-2H-1,2,3-triazol-2-yl)dihydrofuran-2(3H)-one (4k). The spectroscopic data are in accordance with those reported.8bYield: 22.6 mg (93%); yellow oil liquid; Rf = 0.20 (hexane–EtOAc, 10
:
1). IR (neat): 3465, 2978, 1740, 1635, 1380 cm−1. 1H NMR (400 MHz, DMSO): δ 8.43 (s, 1H), 7.87 (d, J = 7.2 Hz, 2H), 7.46 (t, J = 7.4 Hz, 2H), 7.39 (t, J = 7.3 Hz, 1H), 3.09–2.96 (m, 2H), 2.84–2.75 (m, 1H), 2.68–2.60 (m, 1H), 2.14 (s, 3H). 13C NMR (100 MHz, DMSO): δ 176.0, 148.4, 133.2, 129.8, 129.5, 129.4, 126.4, 97.9, 33.7, 29.2, 25.1.
4-Phenyl-1-(1-phenylvinyl)-1H-1,2,3-triazole (7). The spectroscopic data are in accordance with those reported.12Yield: 17.2 mg (70%); yellow oil liquid; Rf = 0.20 (hexane–EtOAc, 10
:
1). IR (neat): 3038, 2934, 1496, 1459, 756 cm−1. 1H NMR (400 MHz, CDCl3): δ 7.99–7.97 (m, 2H), 7.93 (s, 1H), 7.60–7.45 (m, 8H), 6.00 (d, J = 1.0 Hz, 1H), 5.69 (d, J = 0.9 Hz, 1H). 13C NMR (100 MHz, CDCl3): δ 147.6, 143.0, 134.6, 130.2, 129.9, 128.9, 128.8, 128.3, 127.4, 125.8, 119.8, 109.4.
Conflicts of interest
There are no conflicts to declare.
Acknowledgements
This work was financially supported by the Project of Science and Technology Department of Sichuan Province (No. 2018JY0319) and the Fundamental Research Funds of Central Universities, Southwest Minzu University (2018NZD05).
Notes and references
-
(a) D. Astruc, L. Y. Liang, A. Rapakousiou and J. Ruiz, Acc. Chem. Res., 2012, 45, 630–640 CrossRef PubMed;
(b) C. H. Chu and R. H. Liu, Chem. Soc. Rev., 2011, 40, 2177–2188 RSC;
(c) M. G. Finn and V. V. Fokin, Chem. Soc. Rev., 2010, 39, 1231–1232 RSC;
(d) Y. H. Lau, P. J. Rutledge, M. Watkinson and M. H. Todd, Chem. Soc. Rev., 2011, 40, 2848–2866 RSC;
(e) T. Muller and S. Brase, Angew. Chem., Int. Ed., 2011, 50, 11844–11845 CrossRef PubMed.
-
(a) H. Wamhoff, in Comprehensive Heterocyclic Chemistry, ed. A. R. Katritzky and C. W. Rees, Pergamon, Oxford, UK, 1984, vol. 5, pp. 669–732 Search PubMed;
(b) W.-Q. Fan and A. R. Katritzky, in Comprehensive Heterocyclic Chemistry II, ed. A. R. Katritzky, C. W. Rees and E. F. V. Scriven, Elsevier Science, Oxford, UK, 1996, vol. 4, pp. 1–126 Search PubMed.
-
(a) R. Huisgen, 1,3-Dipolar Cycloaddition Chemistry, ed. A. Padwa, Wiley, New York, 1984 Search PubMed;
(b) B. C. Boren, S. Narayan, L. K. Rasmussen, L. Zhang, H. T. Zhao, Z. Y. Lin, G. C. Jia and V. V. Fokin, J. Am. Chem. Soc., 2008, 130, 8923–8930 CrossRef PubMed;
(c) H. C. Kolb, M. G. Finn and K. B. Sharpless, Angew. Chem., Int. Ed., 2001, 40, 2004–2021 CrossRef;
(d) M. M. Majireck and S. M. Weinreb, J. Org. Chem., 2006, 71, 8680–8683 CrossRef PubMed;
(e) V. V. Rostovtsev, L. G. Green, V. V. Fokin and K. B. Sharpless, Angew. Chem., Int. Ed., 2002, 41, 2596–2599 CrossRef;
(f) C. W. Tornoe, C. Christensen and M. Meldal, J. Org. Chem., 2002, 67, 3057–3064 CrossRef PubMed;
(g) P. Wu and V. V. Fokin, Aldrichimica Acta, 2007, 40, 7–17 Search PubMed;
(h) L. Zhang, X. G. Chen, P. Xue, H. H. Y. Sun, I. D. Williams, K. B. Sharpless, V. V. Fokin and G. C. Jia, J. Am. Chem. Soc., 2005, 127, 15998–15999 CrossRef PubMed.
- Selected examples of N2-arylation:
(a) Y. X. Liu, W. M. Yan, Y. F. Chen, J. L. Petersen and X. D. Shi, Org. Lett., 2008, 10, 5389–5392 CrossRef PubMed;
(b) A. B. Lopes, P. Wagner, R. de Souza, N. L. Germain, J. Uziel, J. J. Bourguignon, M. Schmitt and L. S. M. Miranda, J. Org. Chem., 2016, 81, 4540–4549 CrossRef PubMed;
(c) S. Ueda, M. J. Su and S. L. Buchwald, Angew. Chem., Int. Ed., 2011, 50, 8944–8947 CrossRef PubMed;
(d) X. J. Wang, L. Zhang, H. Lee, N. Haddad, D. Krishnamurthy and C. H. Senanayake, Org. Lett., 2009, 11, 5026–5028 CrossRef PubMed;
(e) J. Wen, L. L. Zhu, Q. W. Bi, Z. Q. Shen, X. X. Li, X. Li, Z. Wang and Z. L. Chen, Chem.–Eur. J., 2014, 20, 974–978 CrossRef PubMed.
- Selected examples of N2-allylation:
(a) S. Kamijo, T. Jin, Z. B. Huo and Y. Yamamoto, J. Org. Chem., 2004, 69, 2386–2393 CrossRef PubMed;
(b) S. Kamijo, T. N. Jin, Z. B. Huo and Y. Yamamoto, J. Am. Chem. Soc., 2003, 125, 7786–7787 CrossRef PubMed;
(c) K. Xu, N. Thieme and B. Breit, Angew. Chem., Int. Ed., 2014, 53, 7268–7271 CrossRef PubMed;
(d) W. M. Yan, Q. Y. Wang, Y. F. Chen, J. L. Petersen and X. D. Shi, Org. Lett., 2010, 12, 3308–3311 CrossRef PubMed.
- Examples of N2-alkylated triazoles in biological application:
(a) B. E. Blass, K. Coburn, W. Lee, N. Fairweather, A. Fluxe, S. D. Wu, J. M. Janusz, M. Murawsky, G. M. Fadayel, B. Fang, M. Hare, J. Ridgeway, R. White, C. Jackson, L. Djandjighian, R. Hedges, F. C. Wireko and A. L. Ritter, Bioorg. Med. Chem. Lett., 2006, 16, 4629–4632 CrossRef PubMed;
(b) C. D. Cox, M. J. Breslin, D. B. Whitman, J. D. Schreier, G. B. McGaughey, M. J. Bogusky, A. J. Roecker, S. P. Mercer, R. A. Bednar, W. Lemaire, J. G. Bruno, D. R. Reiss, C. M. Harrell, K. L. Murphy, S. L. Garson, S. M. Doran, T. Prueksaritanont, W. B. Anderson, C. Y. Tang, S. Roller, T. D. Cabalu, D. H. Cui, G. D. Hartman, S. D. Young, K. S. Koblan, C. J. Winrow, J. J. Renger and P. J. Coleman, J. Med. Chem., 2010, 53, 5320–5332 CrossRef PubMed;
(c) O. S. Kanishchev, G. P. Gudz, Y. G. Shermolovich, N. V. Nesterova, S. D. Zagorodnya and A. V. Golovan, Nucleosides Nucleotides Nucleic Acids, 2011, 30, 768–783 CrossRef PubMed;
(d) M. Whiting, J. Muldoon, Y. C. Lin, S. M. Silverman, W. Lindstrom, A. J. Olson, H. C. Kolb, M. G. Finn, K. B. Sharpless, J. H. Elder and V. V. Fokin, Angew. Chem., Int. Ed., 2006, 45, 1435–1439 CrossRef PubMed;
(e) L. Zhang, Z. B. Li, X. J. Wang, N. Yee and C. H. Senanayake, Synlett, 2012, 1052–1056, DOI:10.1055/s-0031-1290770.
-
(a) Y. F. Chen, Y. X. Liu, J. L. Petersen and X. D. Shi, Chem. Commun., 2008, 3254–3256, 10.1039/b805328f;
(b) J. Kalisiak, K. B. Sharpless and V. V. Fokin, Org. Lett., 2008, 10, 3171–3174 CrossRef PubMed;
(c) X. J. Wang, K. Sidhu, L. Zhang, S. Campbell, N. Haddad, D. C. Reeves, D. Krishnamurthy and C. H. Senanayake, Org. Lett., 2009, 11, 5490–5493 CrossRef PubMed;
(d) X. J. Wang, L. Zhang, D. Krishnamurthy, C. H. Senanayake and P. Wipf, Org. Lett., 2010, 12, 4632–4635 CrossRef PubMed.
-
(a) J. W. Shi, L. L. Zhu, J. Wen and Z. L. Chen, Chin. J. Org. Chem., 2016, 37, 1222–1226 Search PubMed;
(b) T. Ma, C. Y. Sun, X. Yuan, X. X. Li and Z. G. Zhao, RSC Adv., 2017, 7, 1062–1066 RSC.
- L.-L. Zhu, X.-Q. Xu, J.-W. Shi, B.-L. Chen and Z. Chen, J. Org. Chem., 2016, 81, 3568–3575 CrossRef PubMed.
- M. Kumar, G. B. Hammond and B. Xu, Org. Lett., 2014, 16, 3452–3455 CrossRef PubMed.
-
(a) A. S. K. Hashmi, S. Schafer, V. Goker, C. D. Eisenbach, K. Dirnberger, Z. Zhao-Karger and P. Crewdson, Aust. J. Chem., 2014, 67, 500–506 CrossRef;
(b) F. Nzulu, S. Telitel, F. Stoffelbach, B. Graff, F. Morlet-Savary, J. Lalevee, L. Fensterbank, J. P. Goddard and C. Ollivier, Polym. Chem., 2015, 6, 4605–4611 RSC;
(c) J. Urbano, A. J. Hormigo, P. de Fremont, S. P. Nolan, M. M. Diaz-Requejo and P. J. Perez, Chem. Commun., 2008, 759–761, 10.1039/b716145j;
(d) F. Nzulu, A. Bontemps, J. Robert, M. Barbazanges, L. Fensterbank, J. P. Goddard, M. Malacria, C. Ollivier, M. Petit, J. Rieger and F. Stoffelbach, Macromolecules, 2014, 47, 6652–6656 CrossRef.
- C. Y. Sun, X. Yuan, Y. Li, X. X. Li and Z. G. Zhao, Org. Biomol. Chem., 2017, 15, 2721–2724 RSC.
- H. F. Duan, S. Sengupta, J. L. Petersen, N. G. Akhmedov and X. D. Shi, J. Am. Chem. Soc., 2009, 131, 12100–12102 CrossRef PubMed.
- T. Jin, S. Kamijo and Y. Yamamoto, Eur. J. Org. Chem., 2004, 3789–3791, DOI:10.1002/ejoc.20040442.
Footnote |
† Electronic supplementary information (ESI) available. See DOI: 10.1039/c8ra04790a |
|
This journal is © The Royal Society of Chemistry 2018 |
Click here to see how this site uses Cookies. View our privacy policy here.