DOI:
10.1039/C8RA06413J
(Paper)
RSC Adv., 2018,
8, 36133-36141
Inhibition of biofilm formation and quorum sensing mediated phenotypes by berberine in Pseudomonas aeruginosa and Salmonella typhimurium
Received
30th July 2018
, Accepted 12th October 2018
First published on 23rd October 2018
Abstract
Quorum sensing is involved in biofilm formation and modulates virulence factor production in pathogenic bacteria. Quorum sensing inhibitors can be used as novel intervention strategies for attenuating bacterial pathogenicity. Berberine is an isoquinoline alkaloid with pharmacological properties. The present study investigated the sub-inhibitory concentrations of berberine for inhibiting biofilm formation and quorum sensing regulated phenotypes in the bacterial pathogens Pseudomonas aeruginosa PA01 and Salmonella enterica serovar Typhimurium. Berberine inhibited quorum sensing regulated violacein production in C. violaceum. It reduced the pigment production in the wild type strain at 1.6 mg mL−1 by 62.67%. In the opportunistic pathogen, P. aeruginosa PA01, at sub-MIC, it showed significant antibiofilm activity in by reducing biomass by 71.70% (p < 0.05). It prevented biofilm formation and inactivated biofilm maturation in bacterial pathogens at the concentration ranging from 0.019 to 1.25 mg mL−1. In silico studies showed that berberine interacted with the quorum sensing signal receptors, LasR and RhlR. Furthermore, its anti-infective properties in S. Typhimurium were studied. At sub-inhibitory concentrations of 0.019 mg mL−1, it reduced biofilm formation in S. Typhimurium by 31.20%. It significantly prevented invasion and adhesion of Salmonella invasion in the colonic cell, HT 29 by 55.37% and 54.68%, respectively. It was capable of reducing in vivo virulence in Caenorhabditis elegans infected with Salmonella at 0.038 mg mL−1 by 65.38%. Our results suggest that berberine, previously recognised for its antimicrobial activity, could find potential application as an anti-biofilm and anti-infective agent based on its quorum sensing inhibitory activity.
Introduction
Quorum sensing is a cell density dependent mechanism used by bacteria to regulate gene expression. It is an essential component of biofilm physiology, as biofilms have a high concentration of bacterial cells.1 Quorum sensing or cell to cell communication is utilized by bacteria for biofilm initiation, maturation and dispersal and also for regulating metabolic activity and population density within the biofilm.2 Therefore, anti-biofilm strategies can be developed based on inhibition or interruption of the quorum sensing mechanism in bacteria.3
Apart from its role in biofilm formation, the quorum sensing mechanism is known to modulate virulence in pathogenic bacteria at molecular levels.4 Therefore, potential quorum sensing inhibitors could be developed as anti-infective agents against pathogenic bacteria. The phytochemicals having structural analogy to the AHLs compete with the signaling molecules to bind to the quorum sensing receptors. Hence, these compounds have the potential to be used as quorum sensing inhibitors.5 Phytochemicals such as furocoumarins have inhibited quorum sensing and biofilms in the pathogenic Vibrio harveyi, E. coli O157
:
H7, S. enterica sv Typhimurium and P. aeruginosa. Purified plant derived compounds including furocoumarins and bergamottin were shown to inhibit signal molecule production by 94.6–97.7% and modulate quorum sensing regulated phenotypes.6 Thus, compounds derived from plants and other dietary phytochemicals generally considered as safe can be screened for their effect on quorum sensing regulated phenotypes and further developed as quorum sensing inhibitors against the pathogens.
Berberine hydrochloride, a protoberberine alkaloid is a compound with various pharmacological effects including antimicrobial, antimalarial, anti-inflammatory, and anticancer activities. It has been used as a therapeutic agent in clinical applications due to its inherent low cytotoxicity.7 It is also widely used for the treatment of bacterial and fungal infections. At sub-MICs berberine inhibited MRSA biofilms in a concentration dependent manner. It has shown to inhibit MRSA amyloid fibril formation proving its efficacy as an agent for treating microbial-generated amyloid diseases.8 It was capable of inhibiting biofilms of Staphylococcus epidermidis, a clinically relevant pathogen involved in medical-device associated infections at sub-MICs.9 Similarly, berberine in combination with other antibiotics is known to reduce biofilm formation in clinical strains of S. aureus. It was capable of effectively downregulating the expression of the biofilm-associated sarA, cidA and icaA genes.10 Berberine has also inhibited biofilm formation and promoted biofilm dispersion in UTI causing Enterococcus faecalis.11 It is proved to be a good endodontic irrigant against multispecies biofilms of endodontic pathogens due to its bactericidal efficacy.12
In the present study, the phytochemical berberine belonging to the class isoquinoline alkaloids was studied at sub-inhibitory concentrations for the quorum sensing and biofilm inhibitory activity and anti-infective potential in Gram negative pathogens P. aeruginosa PA01 and S. enterica sv Typhimurium. The phytochemical was also tested for its ability to inhibit quorum sensing regulated phenotypes in biosensor strains of C. violaceum and P. aeruginosa PA01. The putative interaction of the compound with the quorum sensing signal receptors was analysed by computational studies. Further, its effect on virulence, invasion and pathogenicity in S. enterica sv Typhimurium was studied. The phytochemical berberine can be further developed into anti-biofilm and anti-infective agent based on its anti-quorum sensing mechanism.
Materials and methods
Bacterial strains and chemicals
Chromobacterium violaceum (ATCC 12472), C. violaceum CV026, P. aeruginosa PAO1 and P. aeruginosa PAO1-JP2 were used for determining the quorum sensing inhibitory potential of the phytochemicals. C. violaceum (ATCC 12472) is a wild type strain whereas C. violaceum CV026 is a mutant, unable to produce signaling molecules but responds to short chain of AHLs. P. aeruginosa PAO1 is a wild type and P. aeruginosa PAO1-JP2 is lasI and rhII mutants unable to produce AHLs. The strains were cultured in Luria Bertani media at 28 °C. Salmonella enterica var typhimurium a clinical isolate was used for anti-infective study. The pathogen was grown and maintained in nutrient broth at 37 °C. The nematode Caenorhabditis elegans used for antivirulence studies was grown in nematode growth media at 25 °C. E. coli OP50 was cultured in LB media at 37 °C.
Berberine chloride used in the study was purchased from Sigma-Aldrich (B251-5G, St. Louis, USA). Its purity is >98%. The QS signal molecule N-butanyl-L-homoserine lactone (C4-HSL) and QS inhibitor furanone C30 purchased from Sigma-Aldrich, India were used in the experiments.
Determination of MIC
The minimum inhibitory concentration of berberine was determined in the Mueller–Hinton broth using a broth microdilution method.13 The MIC of the phytochemical against the bacteria used in the assays were assessed in the concentration ranging from 1.250 to 0.019 mg mL−1. The MIC was recorded as the lowest concentration exhibiting complete inhibition of visible growth and confirmed by the addition of tetrazolium trichloride dye. All the experiments in the present study were performed at sub-MIC concentrations of the phytochemical.
Quorum sensing inhibitory activity of berberine
Inhibition of violacein production in C. violaceum. Quorum sensing inhibitory activity of the phytochemicals was quantified by measuring the inhibition of violacein production in C. violaceum (ATCC 12472) and C. violaceum CV026.14 LB broth supplemented with sub-inhibitory concentration of berberine was inoculated with 200 μL of C. violaceum culture (WT) and incubated at 28 °C. Violacein extraction was carried out from the phytochemical treated culture and control sample by adding 200 μL of 10% SDS, vortexing for 15 s and incubated at room temperature for 5 min. The cell lysate was treated with 900 μL of water-saturated butanol (50 mL of n-butanol mixed with 10 mL distilled water), vortexed for 5 s and centrifuged at 13
000g for 5 min. The absorbance of butanol phase containing violacein was measured at 585 nm. The percentage inhibition of violacein production was calculated.Similar studies were performed with C. violaceum CV026 biosensor strain. Overnight grown C. violaceum CV026 culture was inoculated in LB medium supplemented with C4-HSL and the sub-inhibitory concentration of berberine. After incubation, the violacein was extracted and quantified as previously described. The quorum sensing inhibitory activity was indicated by reduction in violacein production.
Inhibition of swimming, swarming and twitching motility. Motility assay was carried out by adding sub-inhibitory concentration of berberine into the motility media and inoculating with 5 μL of bacterial broth culture (108 CFU mL−1). The swim plates contained 0.3% agar and was inoculated with bacterial culture directly into the center of the LB media to evaluate motility in the semisolid agar. Swarm plates were prepared with 0.5% agar and inoculum placed in the center of agar surface to evaluate bacterial motility across the surface. Twitching motility plates contained 1% agar and bacteria culture was inoculated deep into the media. The diameters of the all three motility zones were measured after incubation at 37 °C for 24 h.15
Antibiofilm activity of berberine
The biofilm inhibitory activity of berberine was studied in P. aeruginosa PAO1 and S. enterica sv Typhimurium. Its ability to inhibit biofilm formation at 0.5 MIC was assessed by the crystal violet assay.16 In brief, 100 μL of 0.5 MIC equivalent phytochemical and 100 μL of bacteria cultures (absorbance = 0.4 at 600 nm) grown in tryptic soy broth was added to individual wells of a sterile flat-bottomed 96-well polystyrene microtitre plates microlitres. The plates were incubated for 24 h at 37 °C for biofilm formation. The experiment was carried out in triplicates.
The biofilm inhibition by phytochemical was confirmed as a result of anti-QS property by using the control strain P. aeruginosa PAO1-JP2. Overnight grown culture was grown in TSB was adjusted to get 1.5 × 108 CFU mL−1 and 190 μL culture was added to individual wells of a sterile 96 well microtiter plate. Further, the signaling molecules (10 μL of 10 μM 3-oxo-C12 HSL or C4-HSL) were added to each well with sub-MIC of berberine. Control wells contained only media, cells without AHLs, cells with AHLs but without berberine and cells with berberine without AHLS. After incubation at 37 °C for 24 h the biofilm formation was determined by crystal violet technique.17
Fluorescence microscopy
Biofilms were studied using fluorescence microscopy techniques.18 Bacterial cells (108 CFU mL−1) grown in tryptic soy broth was dispensed into sterile Petriplates (90 mm) containing sterilized cover slips (22 × 48 mm) treated with and without sub-MIC of the phytochemicals. The plates were incubated at 37 °C under static conditions for the development of biofilms. After the 24 h incubation period, the cover slips were stained with 20 μL acridine orange dye (1%) (Sigma-Aldrich, Switzerland). The stained glass cover slips were incubated for 30 min under dark condition, rinsed with sterile water to remove excess stain, air dried and further visualized with fluorescence microscopy at 40× air objective and (Axio Scope-Carl Zeiss, Germany). The software AxioVision 4.6 was used for digital processing of the images (Carl Zeiss, Germany).
Salmonella – adhesion and invasion assays
HT 29 a human colon cell line was procured from NCCS, Pune (India). The viability of cells in presence of berberine was tested by 3-(4,5-dimethyl-2-thiazolyl)-2,5-diphenyl-2H-tetrazolium bromide (MTT) assay.19 The assay was performed using EzCount MTT Cell assay Kit (Himedia, India). Cells were cultured in DMEM with 10% FBS and 1% penicillin–streptomycin at 5% CO2 at 37 °C. On reaching 90% confluency, cells were seeded in 96-well plates. 200 μL of diluted cell suspension (1 × 105 cell per mL) was added to each well. The cells were incubated for 24 h. After the incubation period, different concentrations of berberine 0.5 to 20 μL were added to the cells, followed by incubation for 24 h. 20 μL of MTT dye (5 mg mL−1) was added to each well. The plates were gently shaken and incubated for 4 h. The media containing MTT dye solution was removed, 200 μL of solubilizing solution was added to solubilize the formed formazan. The absorbance was measured at 570 nm. Cytotoxicity was calculated and expressed as percentage inhibition.
The adhesion and invasion assay were performed in HT 29 cell line using S. Typhimurium.20 Briefly, the cell adhesion involved centrifuging overnight grown S. Typhimurium cultures resuspended it in DMEM to obtain 1 × 108 CFU mL−1. The bacterial culture and different concentrations of phytochemicals were added to monolayers of HT29 cells grown in 6 well microtitre plates and incubated for 1 h at 37 °C. The wells were washed thrice with PBS. The cells were lysed using 1% Triton X-100 on incubation at room temperature for 20 min. The lysated suspensions were serially diluted and 100 μL of dilution was plated on trypticase soy agar. After incubation for 24 h at 37 °C, the bacterial colonies were used to calculate the adhesion rate. Similarly, in the invasion assay the HT29 cell monolayers were washed once with PBS after 1 h of incubation with S. Typhimurium resuspended in DMEM with different concentrations of berberine. Further, it was incubated for 30 min with 1 mL DMEM supplemented with 100 μg mL−1 gentamicin to kill extracellular bacteria. The wells were washed with PBS and lysed with 1% Triton X-100. The suspension was serially diluted and plated on TSA. The number of invaded or intracellular bacteria was calculated by counting the colonies on TSA plates.
In vivo virulence of S. Typhimurium in Caenorhabditis elegans (N2) model
S. Typhimurium was grown overnight in nutrient broth at 37 °C. The overnight bacterial culture was re-suspended in S Basal liquid assay media and 800 μL of the bacterial suspension was aliquoted into each well of a 96-well flat-bottomed plate. Berberine at 0.019 and 0.038 mg mL−1 and furanone (1 μM) was added to the wells. Twenty L4-staged worms were added to each well and were incubated at 23 °C for 24 h. Each well was examined with a microscope to observe the infection phenotype in C. elegans. Infected worms were also transferred to unseeded NGM plates to verify the phenotype. Worms were considered paralysed when neither body twitching nor pharyngeal pumping could be observed. Percentage of paralysed worms were calculated to determine anti-virulence effect of S. Typhimurium.21
Molecular docking studies
The crystal structures of P. aeruginosa LasR (PDB ID: 2UV0) as well as a homology model of P. aeruginosa RhlR were employed for setting-up molecular docking protocols. Prior to this, the protein coordinates were optimized through suitable bond-order assignments, water molecules elimination, addition of hydrogen atoms, internal hydrogen bonds refinement, restrained minimization etc. through OPLS 2005 force-field. Docking grids were generated within 20 Å from the ligand binding site (or equivalent site in case of the homology model). The small molecules (compounds) were energy minimized through LigPrep and then docked in to the generated grid employing extra precision (XP) mode of Glide 6.2. All docked molecules were finally processed through a post-docking minimization protocol of the same software. The images were prepared using PyMOL 1.0.
Homology modeling
The target sequence of full-length P. aeruginosa RhlR (1–241) was retrieved from UniProt (Acc. code: P54292). This was further subjected to homology model building employing ‘knowledge-based’ protocol of Prime 3.5. The generated model was further optimized through MacroModel 10.3 in a simulated aqueous environment and employing OPLS 2005 force-field. The final model was observed to have a reasonable Ramachandran plot and only few atypical side-chain conformations. All software names mentioned above are products of Schrödinger LLC.22
Statistical analysis
All the experiments were performed in triplicates and the data were reported as means + standard deviation. Student's t test was used to analyze the differences between control and test. One-way analysis of variance (ANOVA) was used in analyzing differences in the different assays. The p values <0.05 were considered statistically significant. The statistical analysis was carried out using IBM SPSS Statistics 18.
Results and discussion
Determination of minimum inhibitory concentration
In the present study, the phytochemical berberine was studied for the quorum sensing modulatory and anti-biofilm activity. The MIC of the phytochemical against the tested bacteria were determined at a concentration ranging from 0.019 to 2.50 mg mL−1 (Table 1). Sub-inhibitory concentration of the phytochemicals were used in the study as they are known to inhibit quorum sensing regulated phenotypes, without affecting the growth of the bacteria. The absorbance of the growth of the phytochemical treated and control bacteria did not show any significant difference.
Table 1 Determination of MIC of berberine against bacterial pathogens
Pathogens |
MIC (mg mL−1) |
C. violaceum |
2.50 |
C. violaceum CV026 |
2.50 |
P. aeruginosa PA01 |
1.250 |
P. aeruginosa PA01-JP2 |
0.156 |
S. Typhimurium |
0.076 |
Quorum sensing inhibitory activity of berberine
Inhibition of violacein production. The quorum sensing inhibitory potential of berberine was screened using the biosensor bacteria C. violaceum. Violacein pigment production in C. violaceum (WT) is regulated by the CviIR-an acylhomoserine lactone based quorum sensing system. The compounds capable of inhibiting violacein production at sub inhibitory concentration without reducing the growth of the bacteria are considered potential quorum sensing inhibitors. These can interfere with the CviR–AHL complex and prevent expression of the vio genes required for the production of violacein. Similarly, in C. violaceum CV026 the biosensor strain produces violacein only in presence of exogenous signal molecules such as C4-HSL. The QS inhibitors can prevent violacein production in C. violaceum CV026 even in presence of the autoinducers.23 Berberine was capable of inhibiting production of the pigment violacein in both the wildtype and mutant of C. violaceum at the tested concentrations (Fig. 1). Berberine reduced violacein production in the wildtype by 62.67% at a concentration of 1.6 mg mL−1. In C. violaceum CV026, the pigment production was reduced by 73.52% at the same concentration. Previously, it has been shown that cinnamaldehyde at minimal concentration was capable of inhibiting acyl homoserine lactone (3-oxo-C6-HSL and 3-oxo-C12-HSL) and autoinducer 2 mediated quorum sensing.24 Similarly, curcumin at concentrations ranging from 25–100 μg mL−1 has been shown to inhibit hexanoyl homoserine lactone mediated quorum sensing in C. violaceum and formation of biofilms by uropathogens.25 However, there are no reports of the quorum sensing inhibitory potential of the phytochemical berberine. Phytochemicals having structural homology to the acyl homoserine lactones may act as competitive inhibitors and prevent the signal reception or decrease the expression of the signal synthases, and thus inhibit expression of quorum sensing regulated phenotypes.26
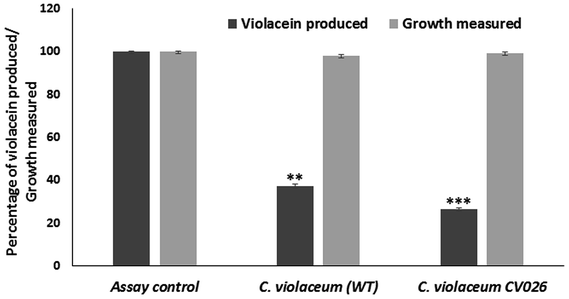 |
| Fig. 1 Effect of sub-inhibitory concentration of berberine on quorum sensing mediated violacein production in C. violaceum (wild type) and C. violaceum CV026. | |
Inhibition of swimming, swarming and twitching motility. Berberine inhibited both swimming and swarming motility in P. aeruginosa PA01. It had no inhibitory effect on twitching motility in P. aeruginosa PA01 (Fig. 2). Berberine did not interfere with swimming, swarming and twitching motility in S. enterica sv Typhimurium. It significantly inhibited swarming motility in P. aeruginosa PA01 by 33.34% at 0.625 mg mL−1. Berberine exhibited maximum inhibition in swimming motility at 0.312 mg mL−1. The antibacterial activity of the phytochemical berberine has been asserted previously. However, there are no reports to date on its effect on virulence factor production in pathogenic bacteria. In bacteria, motility is required for microbial surface colonization, biofilm formation and pathogenesis. Motility is also known to be regulated by quorum sensing mechanism in P. aeruginosa.15 Berberine exerted inhibitory effect on swimming and swarming motility in P. aeruginosa PA01 at sub-MIC. Quorum sensing inhibitory compounds such as halogenated furanones are known to inhibit swarming motility in pathogenic bacteria.27 The phytochemicals capable of affecting bacterial motility can be used to prevent bacterial surface colonization.
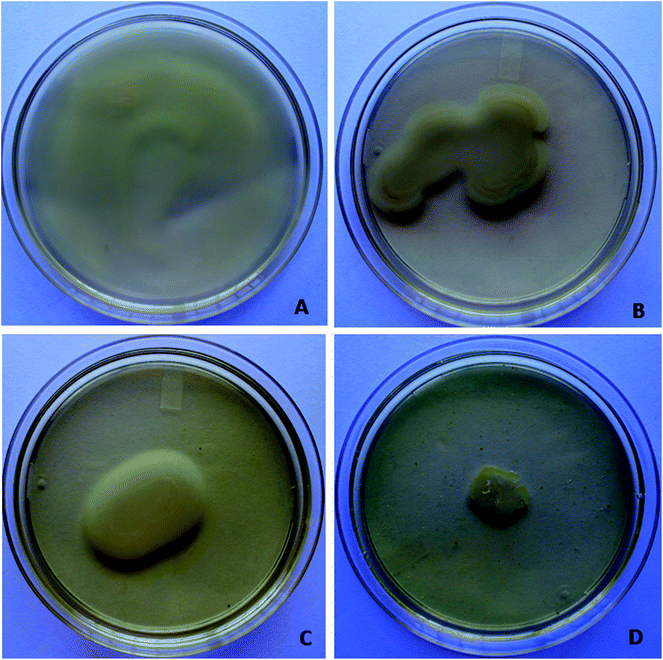 |
| Fig. 2 Inhibitory effect of berberine on swimming and swarming motility in P. aeruginosa PA01. Swimming (A) and swarming motility (B) control in P. aeruginosa PA01; swimming (C) and swarming motility (D) in P. aeruginosa PA01 at sub-inhibitory concentration of berberine. | |
Antibiofilm activity of berberine
Biofilm biomass – crystal violet assay. Berberine was screened for its anti-biofilm potential in the pathogenic bacteria using crystal violet microtitre plate assay (CV-MTP) at sub MIC (Fig. 3). Berberine showed significant anti-biofilm potential against both the pathogens. It showed significant inhibitory activity against attachment and biofilm formation in P. aeruginosa PA01 at 0.625 mg mL–1 by 71.70%. At 0.019 mg mL−1, it inhibited biofilms of S. enterica sv Typhimurium by 31.20%. Thus, it is clear from the above study that berberine was capable of significantly inhibiting bacterial biofilms (p < 0.05). Further, the QS inhibitory effect of berberine on P. aeruginosa PA01 JP-2 was studied at 0.078 mg mL−1. The biosensor forms weak biofilms but in presence of AHLs it forms moderate to strong biofilms. However, the addition of berberine reduced the biofilm formation of P. aeruginosa PA01 JP-2 in presence of both the signaling molecules C4-HSL and 3-oxo-C12 HSL by 65.50 and 60.74%, respectively (Fig. 3). Thus, the biofilm inhibitory activity of berberine at sub-inhibitory concentrations is due to its anti-quorum sensing property.
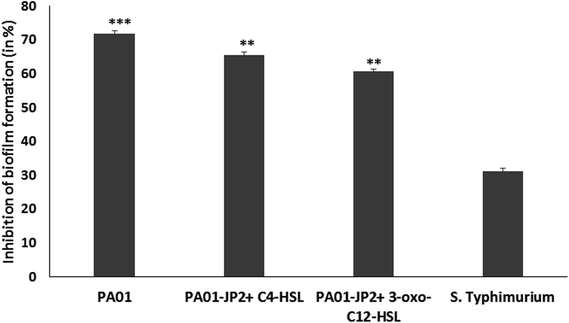 |
| Fig. 3 Anti-biofilm activity of sub-MIC of berberine on P. aeruginosa PA01 (WT and mutant) and S. Typhimurium assessed by crystal violet microtiter plate assay. | |
Fluorescence microscopy analysis
Microscopy studies revealed the effect of the phytochemical berberine on bacterial attachment, colonization of surface and formation of mature biofilms. Berberine was able to inhibit attachment of P. aeruginosa PA01 cells but at a higher concentration of 0.625 mg mL−1 (Fig. 4). Though berberine was unable to inhibit initial cell attachment in S. Typhimurium, however, it was capable of disrupting the pre-formed biofilms. At a concentration of 0.019 mg mL−1, it disrupted colonies of S. enterica sv Typhimurium and prevented them from developing into mature biofilms (Fig. 4). Berberine without affecting bacterial growth significantly inhibited biofilm formation in the pathogens by inhibiting adherence to substratum, interfering with EPS production and by reducing metabolic activity leading to prevention of biofilm maturation. Though their activity on initial attachment of S. Typhimurium was low, they could disrupt biofilm formation during maturation stage which is probably due to the inhibition of EPS production. Quorum sensing mechanism is known to be involved not only in the initiation but also in the maturation of bacterial biofilms. EPS production in biofilms required for the development of biofilm architecture and maturation has been demonstrated to be under quorum sensing regulon.28 Therefore, it can be concluded that the phytochemical berberine at sub-inhibitory concentrations inhibited the attachment of cells to substratum and further colonization of the P. aeruginosa and disrupted S. Typhimurium biofilms due to its quorum sensing inhibitory potential.
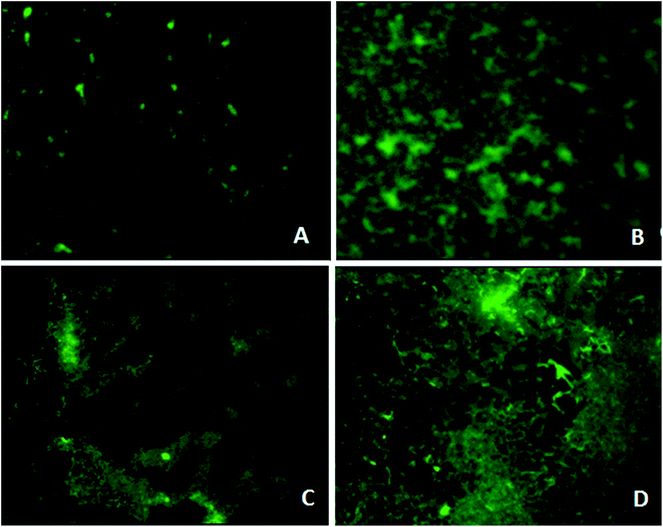 |
| Fig. 4 Fluorescence microscopy analysis of biofilm formation in P. aeruginosa in presence of 0.5× MIC of berberine (A), and untreated control (B); and in S. Typhimurium in presence of 0.5× MIC of berberine (C), and untreated control (D). | |
Anti-infective property
Further, berberine was studied for its anti-virulence property in S. Typhimurium. Berberine was tested for cytotoxicity in HT 29 colon cell line by treating it with the phytochemical for 24 h and measuring the cell viability by MTT assay. Berberine from 0.005 to 0.02 mg mL−1 had no adverse effect on the tested mammalian cell line (Fig. 5). The same concentrations were used to study the effect of berberine on S. Typhimurium adhesion and invasion. In a concentration dependent manner, berberine was capable of significantly reducing both adhesion and invasion of S. Typhimurium in the mammalian colon cells. At the highest tested concentration of 0.02 mg mL−1, berberine reduced adhesion and invasion of S. Typhimurium by 54.68 and 55.37%, respectively (Fig. 5). Previously, it has been reported that sub-inhibitory concentrations of phytochemicals punicalagin and carvacrol significantly reduced Salmonella invasion without affecting adhesion to the intestinal epithelial cells.20,29
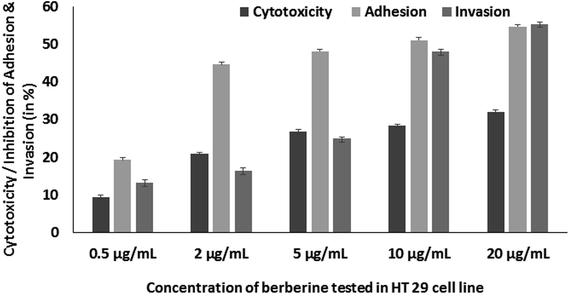 |
| Fig. 5 Concentration dependent effect of berberine on HT 29 cell line cytotoxicity, adhesion and invasion of S. Typhimurium in HT 29 cell line. | |
C. elegans is used as an animal infection model to study the pathogenicity of bacteria. L4-staged C. elegans were infected with S. Typhimurium, without or with the addition of berberine and furanone. Infected worms were incubated at 23 °C for 24 h and were examined for the infection phenotype. They were observed for paralysed state as neither body twitching nor pharyngeal pumping occurs due to severe infection. C. elegans infected with S. Typhimurium showed high paralysis of around 85%, whereas it was observed that berberine and furanone reduced nematode paralysis. Berberine showed a dose dependent reduction in C. elegans infection. At 0.038 mg mL−1, it reduced nematode paralysis by 65.38% (p < 0.05). Furanone, a QS inhibitor showed significant reduction in S. Typhimurium induced nematode paralysis and infection (Fig. 6).
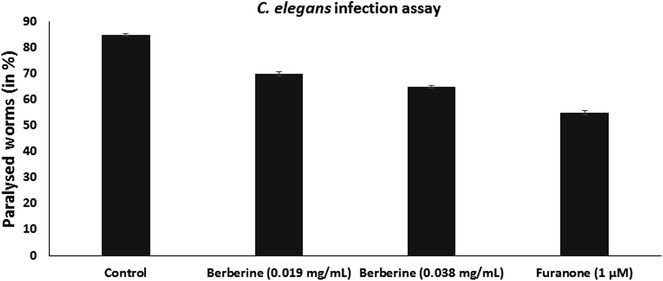 |
| Fig. 6 Effect on berberine (0.019 mg mL−1 and 0.038 mg mL−1) and synthetic QS inhibitor furanone (1 μM) on S. Typhimurium infection in C. elegans animal model evaluated by decrease in number of paralysed worms. | |
S. Typhimurium pathogenesis depends upon a number of factors including virulence factor production, invasion into host and intracellular proliferation. Therefore, alternative strategies such as use of anti-virulence agents, anti-infectives and anti-pathogenic drugs have to be used in the pathogen control. As these agents are used at sub-inhibitory concentration, they impose less selective power for resistance among pathogens.
Molecular docking
Docking studies were performed to screen the interaction of berberine with the quorum sensing receptors. The receptors after binding with the signals act as transcriptional regulators. The phytochemical were docked against the quorum sensing signal receptors LasR and RhlR (Fig. 7). The docking analysis revealed that had better interaction with the RhlR receptors than the LasR protein as shown by the docking scores and the interaction between the ligand and the receptors (Table 2).
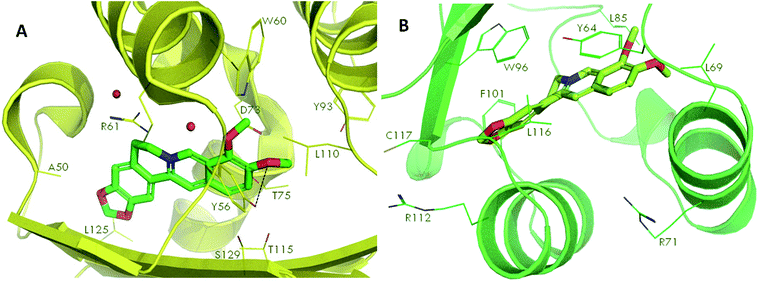 |
| Fig. 7 Molecular docking of phytochemical berberine with QS signal receptors, LasR (A) and RhlR (B). | |
Table 2 Molecular docking analysis of the phytochemicals with the RhlR and LasR receptors
Compounda |
Glide 6.2 b extra precision (XP) docking scores |
P. aeruginosa RhlRc |
P. aeruginosa LasRd |
Structures downloaded from PubChem. Molecular docking software (Product of Schrödinger LLC). Docked in an in-house generated homology model (template: PDB ID - 4LFU, sequence identity 40%). Docked in PDB ID - 2UV0, chain H (Quorum sensing signaling molecule binding site). |
Berberine |
−10.1 |
−5.9 |
The leucine (L125), tyrosine (Y56), threonine (T75) and asparagine (N73) of LasR and leucine (L116), phenylalanine (F101), tyrosine (Y64), leucine (L85) of RhlR, interacted with berberine. Thus, the amino acids which were involved in bond formation to provide efficient interaction of the ligands with the receptors was identified using the docking analysis. Therefore, by the docking studies it can be concluded that the phytochemical berberine can interact with the quorum sensing receptors. This phytochemical can be used as the lead molecule for the development of new drugs to inhibit quorum sensing in LuxR homologues utilizing pathogenic bacteria.
Thus, in silico studies based on molecular docking analysis have supported the quorum sensing inhibitory behavior of berberine. In our study, docking analysis revealed the possible interactions between berberine and the QS receptors. Similarly, in P. aeruginosa PAO1 LasR and RhlR are receptors for the quorum sensing signal molecules 3oxo-C12 HSL and C4 HSL, respectively. Docking studies were used to explore the probable active sites in these transcriptional regulators with berberine. The overall docking results of the phytochemical with the receptors revealed that the conserved residues tyrosine, leucine, arginine, threonine, asparaginine and phenylalanine were involved in bond formation with the phytochemical. Berberine had strong interaction with the signal receptors which might be the reason for their quorum sensing modulatory property as exhibited by them in the inhibition of violacein production in C. violaceum and in disrupting motility and biofilms of P. aeruginosa.
Berberine is an isoquinolone alkaloid present in plants of the genus Coptis, Hydrastis and Berberis and these plants have been consumed as a food source and used for their medicinal properties.30 The present study, demonstrates that berberine has biofilm inhibitory and anti-quorum sensing property, apart from other therapeutic potentials.
Conclusion
The phytochemical berberine at sub-inhibitory concentration was capable of modulating quorum sensing regulated phenotypes and inhibited biofilm formation in the QS biosensor organisms C. violaceum and P. aeruginosa PA01 as determined by in vitro and in silico analysis. Further, berberine was capable of reducing adhesion, invasion and infection in S. Typhimurium. Thus, berberine could find potential application as anti-infective agents to control P. aeruginosa and S. Typhimurium infections.
Conflicts of interest
There are no conflicts to declare.
Acknowledgements
The authors thank Prof. Kalai Mathee, Florida International University, USA for providing the biosensor strains used in the study and CGC Centre, University of Minnesota for C. elegans nematode and E. coli OP50. The authors thank Indian Council of Medical Research for awarding fellowship to the first author (Grant No. F.No. 80/709/2011-ECD I). The authors acknowledge Institution of Excellence, University of Mysore for providing fluorescence microscopy imaging facility and DST-PURSE, University of Mysore for Cell culture facility.
References
- M. R. Parsek and E. P. Greenberg, Trends Microbiol., 2005, 13, 27–33 CrossRef CAS PubMed
. - S. Asad and S. M. Opal, Crit. Care, 2008, 12, 236–246 CrossRef PubMed
. - M. Simoes, L. C. Simoes and M. J. Vieira, LWT–Food Sci. Technol., 2010, 43, 573–583 CrossRef CAS
. - U. M. Pinto, V. E. de Souza, M. L. Martins and M. C. D. Vanetti, Food Control, 2007, 18, 1322–1327 CrossRef CAS
. - M. Teplitski, U. Mathesius and K. P. Rambaugh, Chem. Rev., 2011, 11, 100–116 CrossRef PubMed
. - B. Girennavar, M. L. Cepeda, K. A. Soni, A. Vikram, P. Jesudhasan and G. K. Jayaprakasha, Int. J. Food Microbiol., 2008, 125, 204–208 CrossRef CAS PubMed
. - J. G. Chung, L. T. Wu, C. B. Chu, J. Y. Jan, C. C. Ho, M. F. Tsou, H. F. Lu, G. W. Chen, J. G. Lin and T. F. Wang, Food Chem. Toxicol., 1999, 37, 319–326 CrossRef CAS PubMed
. - M. Chu, M. B. Zhang, Y. C. Liu, J. R. Kang, Z. Y. Chu, K. L. Yin, L. Y. Ding, R. Ding, R. X. Xiao, Y. N. Yin, X. Y. Liu and Y. D. Wang, Sci. Rep., 2016, 6, 24748 CrossRef CAS PubMed
. - X. Wang, X. Yao, Z. Zhu, T. Tang, K. Dai, I. Sadovskaya, S. Flahaut and S. Jabbouri, Int. J. Antimicrob. Agents, 2009, 34, 60–66 CrossRef PubMed
. - N. Guo, X. Zhao, W. Li, C. Shi, R. Meng, Z. Liu and L. Yu, J. Med. Microbiol., 2015, 64, 891–900 CrossRef CAS PubMed
. - L. Chen, Q. Bu, H. Xu, Y. Liu, P. She, R. Tan and Y. Wu, Microbiol. Res., 2016, 186–187, 44–51 CrossRef CAS PubMed
. - Q. Xie, B. R. Johnson, C. S. Wenckus, M. I. Fayad and C. D. Wu, J. Endod., 2012, 38, 1114–1117 CrossRef PubMed
. - S. Jadhav, R. Shah, M. Bhave and E. A. Palombo, Food Control, 2013, 29, 125–130 CrossRef CAS
. - R. S. Blosser and K. M. Gray, J. Microbiol. Methods, 2004, 40, 47–55 CrossRef
. - C. O'May and N. Tufenkji, Appl. Environ. Microbiol., 2011, 77, 3061–3067 CrossRef PubMed
. - M. Sandasi, C. M. Leonard and A. M. Viljoen, Lett. Appl. Microbiol., 2010, 50, 30–35 CrossRef CAS PubMed
. - M. O. Vandeputte, M. Kiendrebeogo, S. Rajaonson, B. Diallo, A. Mol, M. E. Jaziri and M. Baucher, Appl. Environ. Microbiol., 2010, 76, 243–253 CrossRef PubMed
. - E. Peeters, H. J. Nelis and T. Coenye, J. Microbiol. Methods, 2008, 72, 157–165 CrossRef CAS PubMed
. - J. McCauley, A. Zivanovic and D. Skropeta, Methods Mol. Biol., 2013, 1055, 191–205 CrossRef CAS PubMed
. - G. Li, C. Yan, Y. Xu, Y. Feng, Q. Wu, X. Lv, B. Yang, X. Wang and X. Xiaa, Appl. Environ. Microbiol., 2014, 80, 6204–6211 CrossRef PubMed
. - S. Puiac, X. Sem, A. Negrea and M. Rhen, Int. J. Antimicrob. Agents, 2011, 38, 409–416 CrossRef CAS PubMed
. - S. Gnanendra, S. Anusuya and J. Natarajan, J. Mol. Model., 2012, 18, 4709–4719 CrossRef CAS PubMed
. - L. R. Swem, D. L. Swem, C. T. O'Loughlin, R. Gatmaitan, B. Zhao, S. M. Ulrich and B. L. Bassler, Mol. Cell, 2009, 35, 143–153 CrossRef CAS PubMed
. - C. Niu, S. Afre and E. S. Gilbert, Lett. Appl. Microbiol., 2006, 43, 489–494 CrossRef CAS PubMed
. - I. A. S. V. Packiavathy, P. Agilandeswari, K. S. Musthafa, S. K. Pandian and A. V. Ravi, Food Res. Int., 2012, 45, 85–92 CrossRef CAS
. - D. A. Vattem, K. K. Mihali, S. H. Crixell and R. J. Mclean, Fitoterapia, 2007, 78, 302–310 CrossRef CAS PubMed
. - M. Givskov, R. de Nys and M. Manefield, J. Bacteriol., 1996, 178, 6618–6622 CrossRef CAS PubMed
. - T. R. De Kievit, R. Gillis, S. Marx, C. Brown and B. H. Iglewski, Appl. Environ. Microbiol., 2001, 67, 1865–1873 CrossRef CAS PubMed
. - J. Inamuco, A. K. Veenendaal, S. A. Burt, J. A. Post, J. L. Tjeerdsma-van Bokhoven, H. P. Haagsman and E. J. Veldhuizen, Vet. Microbiol., 2012, 157, 200–207 CrossRef CAS PubMed
. - Y. L. Siow, L. Sarna and O. Karmin, Food Res. Int., 2011, 44, 2409–2417 CrossRef CAS
.
|
This journal is © The Royal Society of Chemistry 2018 |
Click here to see how this site uses Cookies. View our privacy policy here.