DOI:
10.1039/C8RA06860G
(Paper)
RSC Adv., 2018,
8, 41415-41421
Retracted Article: Down-regulation of HOXB5 inhibits TGF-β-induced migration and invasion in hepatocellular carcinoma cells via inactivation of the PI3K/Akt pathway
Received
16th August 2018
, Accepted 22nd November 2018
First published on 11th December 2018
Abstract
HOXB5, a member of the HOX gene family, is a developmental gene which encodes homeoproteins and is known to be a crucial player in development of enteric nervous systems. Recently, HOXB5 was reported to be associated with cancer progression. However, the specific effect of HOXB5 in hepatocellular carcinoma (HCC) remains unclear. In this study, we demonstrated the important role of HOXB5 in HCC. We showed that HOXB5 was up-regulated in HCC tissues and cell lines. Furthermore, down-regulation of HOXB5 inhibited TGF-β-induced HCC cell migration and invasion in vitro and suppressed tumor metastasis in vivo. We also found that the PI3K/Akt pathway partly accounted for the mechanisms underlying the inhibitory effect of HOXB5 down-regulation on TGF-β-induced HCC progression. Taken together, these findings demonstrated that down-regulation of HOXB5 inhibits TGF-β-induced migration and invasion in HCC cells via inactivation of the PI3K/Akt pathway. Thus, HOXB5 may be a novel therapeutic target for HCC treatment.
I. Introduction
Hepatocellular carcinoma (HCC), one of the most prevalent malignancies, features high aggressiveness and invasiveness.1,2 Ranking the second among all leading causes of cancer-related deaths, HCC results in over half a million deaths every year in the world.3 For early-stage HCC, radical resection and liver transplantation are available therapeutic approaches.4,5 Unfortunately, most HCC patients are diagnosed at an advanced stage and the prognosis of these patients remains poor due to a high probability of intrahepatic spread and extrahepatic metastasis.6,7 Therefore, there is an urgent need to identify metastasis-associated factors and explore molecular mechanisms underlying HCC metastasis.
TGF-β, a multifunctional cytokine, is implicated in a variety of cellular processes.8,9 Many studies have reported overexpression of TGF-β in different types of cancer cells.10 In addition, TGF-β has been demonstrated to function as a positive mediator of tumor metastasis.11–13 Thus, it may be a beneficial method to suppress or reverse the TGF-β-induced progression during treatment of cancer patients.
Homeobox genes, termed “HOX” genes, are a group of developmental genes which encode homeoproteins, small proteins functioning as transcriptional factors during the embryonic development.14–16 So far, 39 HOX genes have been identified in the human genome.17 These genes are located on four different chromosomes and are the homologue of homeotic complexes of Drosophila at both structural and functional levels.18 More importantly, it has been found that the protein products of these genes serve as transcriptional activators or repressors during cancer development.19 For example, HOXB5, a member of the HOX family, was demonstrated to be overexpressed in breast cancer tissues and promote cell growth and invasion.20 Similarly, HOXB5 expression was up-regulated in non-small cell lung cancer (NSCLC) tissues and HOXB5 down-regulation suppressed proliferation, migration, and invasion in NSCLC cells.21 These findings showed the critical role of HOXB5 in carcinogenesis. However, the specific effect of HOXB5 in HCC remains unclear.
In this study, we investigated the biological functions of HOXB5 in HCC cell migration and invasion as well as the molecular mechanisms underlying these processes. We found that HOXB5 was overexpressed in HCC tissues and cell lines. Moreover, down-regulation of HOXB5 inhibited TGF-β-induced HCC cell migration and invasion in vitro and suppressed tumor metastasis in vivo. We also demonstrated that the PI3K/Akt pathway may be part of the underlying mechanisms.
II. Results
Expression of HOXB5 is up-regulated in HCC tissues and cell lines
To examine the effect of HOXB5 on HCC, we measured its expression in 98 pairs of HCC tissues and matched non-cancerous tissues by RT-qPCR and western blot analysis. The results showed that the level of HOXB5 mRNA and protein expression in HCC tissues was remarkably higher than that in the normal tissues (Fig. 1A and B). We also detected the expression of HOXB5 in HCC cell lines. As shown in Fig. 1C and D, HOXB5 mRNA and protein expression were significantly up-regulated in Huh-7 and HepG2 cells in comparison with the normal liver cell line MIHA.
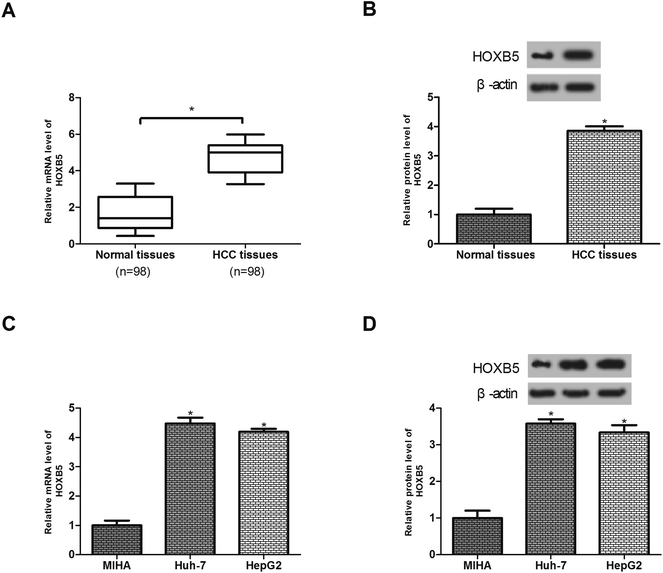 |
| Fig. 1 Expression of HOXB5 is up-regulated in HCC tissues and cell lines. (A and B) RT-qPCR and western blot analysis of HOXB5 expression in HCC tissues and matched normal tissues. (n = 98). (C and D) RT-qPCR and western blot analysis of HOXB5 expression in HCC cell lines (Huh-7 and HepG2) and the normal liver cell line MIHA. *p < 0.05. | |
Down-regulation of HOXB5 inhibits TGF-β-induced HCC cell migration and invasion in vitro
TGF-β is known as a promoter of cancer cell migration and invasion.22,23 To investigate whether HOXB5 was involved in TGF-β-induced HCC cell migration and invasion, the expression of HOXB5 was reduced by shRNA transfection in HCC cells. Knockdown of HOXB5 was verified by western blot analysis (Fig. 2A and B). Transwell assays were performed to examine cell migration and invasion. The results showed that HOXB5 knockdown inhibited Huh-7 cell migration and invasion and abrogated TGF-β-promoted cell migration and invasion (Fig. 2C and D). Similar effects were observed in HepG2 cells (Fig. 2E and F).
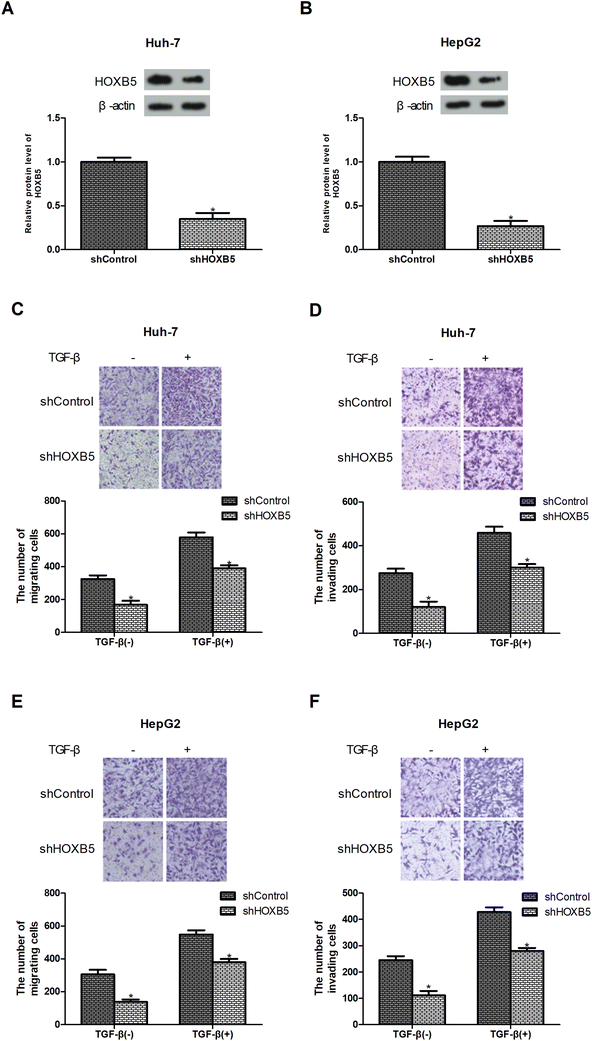 |
| Fig. 2 Down-regulation of HOXB5 inhibits TGF-β-induced HCC cell migration and invasion in vitro. (A and B) HOXB5 expression in Huh-7 and HepG2 cells were measured by western blot analysis after transfection of shHOXB5 or shControl. (C–F) Huh-7 and HepG2 cell migration and invasion were determined using the transwell assay after treatment with or without TGF-β (10 ng mL−1). *p < 0.05. | |
Down-regulation of HOXB5 inhibits TGF-β-induced HCC cell metastasis in vivo
To determine whether HOXB5 down-regulation could inhibit TGF-β-induced HCC cell metastasis in vivo, Huh-7 cells transfected with shHOXB5 or shControl with or without treatment of TGF-β (10 ng mL−1) were injected into the tail vein of nude mice. 30 days later, less metastatic nodules were found in shHOXB5 group while more metastatic nodules were detected in TGF-β1-treated group, in comparison with the control group (Fig. 3A and B).
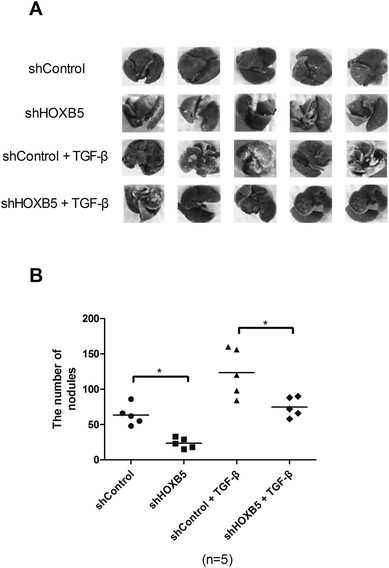 |
| Fig. 3 Down-regulation of HOXB5 inhibits TGF-β-induced HCC cell metastasis in vivo. (A) Representative images of lungs in nude mice were presented. (B) Quantification of metastatic lung nodules of nude mice in different groups. *p < 0.05. | |
Down-regulation of HOXB5 abrogates TGF-β-induced activation of the PI3K/Akt pathway in HCC cells
Previous studies have indicated that TGF-β could activate the PI3K/Akt pathway,24,25 so we investigated whether HOXB5 down-regulation inhibited TGF-β-induced PI3K/Akt pathway. As expected, TGF-β effectively increased phosphorylation of PI3K and Akt while HOXB5 down-regulation significantly blocked TGF-β-induced activation of the PI3K/Akt pathway in Huh-7 cells (Fig. 4A). To further confirm involvement of the PI3K/Akt pathway in the inhibitory effect of HOXB5 down-regulation on TGF-β-induced HCC cell migration and invasion, LY294002 (PI3K/Akt inhibitor) was used to treat Huh-7 cells. The results indicated that LY294002 obviously prevented TGF-β-induced HCC cell migration and invasion and this reversal effect was enhanced by HOXB5 down-regulation (Fig. 4B and C).
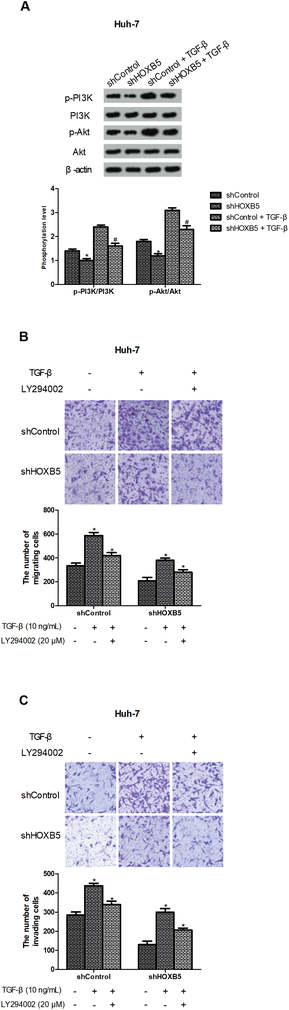 |
| Fig. 4 Down-regulation of HOXB5 abrogates TGF-β-induced activation of the PI3K/Akt pathway in HCC cells. (A) Western blot analysis of p-PI3K, PI3K, p-Akt and Akt in Huh-7 cells. (B and C) Huh-7 cell migration and invasion were determined using the transwell assay after treatment with TGF-β (10 ng mL−1) or LY294002 (20 μM). *p < 0.05 vs. shControl; #p < 0.05 vs. shControl + TGF-β. | |
III. Discussion
According to statistics, there has been an increasing incidence of HCC in the last two decades, but the prognosis remains unsatisfactory.3,5 Among HCC patients, tumor metastasis is ubiquitous, resulting in death of 90% of the patients.26 Thus, we need to make a maximum effort to explore novel therapeutic approaches targeting HCC metastasis.
HOXB5, a member of the HOX gene family, is known as a crucial player in development of the enteric nervous system.27,28 In addition, abnormalities in HOXB5 functions may cause Hirschsprung's disease.29,30 Recently, HOXB5 was reported to be associated with cancer progression. For example, Hong et al. reported that HOXB5 was overexpressed in gastric cancer tissues and induced cell migration and invasion.31 In a study on NSCLC, HOXB5 was targeted to regulate cell migration.32 All these observations indicated the tumor-promoting effect of HOXB5 in cancer progression. Consistently, our study found that HOXB5 was highly expressed in HCC tissues and cell lines. In addition, down-regulation of HOXB5 inhibited HCC cell migration and invasion in vitro. This result was further confirmed by our xenograft tumor assay which showed that HOXB5 down-regulation suppressed HCC cell metastasis in vivo.
TGF-β, a multifunctional cytokine, is a member of transforming growth factors.8,33 It has been demonstrated that TGF-β is capable of eliciting cellular phenotypic changes and acts as a tumor promoter or suppressor in a context-dependent manner.13 In the TGF-β pathway, inactivating mutation represents a mechanism underlying the evasion of tumor-inhibitory functions.34 But core aspects of the TGF-β signaling, in many types of cancers, are intact with amputated tumor-suppressive arms.35,36 In addition, TGF-β is able to enhance migratory and invasive capabilities of cancer cells, representing one of its tumor-promoting arms.37–39 It is a complex mechanism how TGF-β switches from a tumor promoter to a tumor suppressor.40 So far, many signaling pathways have been demonstrated to promote oncogenicity of the TGF-β signaling.41,42 In the present study, we found that TGF-β induced HCC cell migration and invasion while HOXB5 down-regulation abrogated the promoting effect, suggesting HOXB5 as a contributor to the oncogenic TGF-β. Given that HOXB5 is frequently up-regulated in various cancers, it may be another contextual cue promoting cancer progression driven by TGF-β.
The PI3K/Akt signaling pathway plays an important role in cancer migration and invasion.43–45 More importantly, the pathway could be activated or inhibited by TGF-β depending on cell types.46 In this study, we showed that TGF-β promoted HCC cell migration and invasion and this effect was mediated by the PI3K/Akt pathway. In addition, LY294002 (PI3K/Akt inhibitor) blocked TGF-β-induced cell migration and invasion in HCC cells and this reversal effect was potentiated after HOXB5 down-regulation. These results suggested that the PI3K/Akt pathway is essential for the inhibitory effect of HOXB5 down-regulation on TGF-β-induced HCC cell migration and invasion.
In conclusion, this study demonstrated the important role of HOXB5 in HCC. We found that HOXB5 was up-regulated in HCC tissues and cell lines. Furthermore, down-regulation of HOXB5 inhibited TGF-β-induced HCC progression partly through the PI3K/Akt pathway. Taken together, this study may provide a novel therapeutic target for HCC treatment.
IV. Materials and methods
Patients and tissue samples
Human HCC and matched non-cancerous tissues were obtained from 98 patients at Huaihe Hospital of Henan University (Kaifeng, China). Each patient received no adjuvant therapies before surgery. After collection, tissue samples were frozen in liquid nitrogen and stored at −80 °C. The study complied with all institutional and national guidelines, as per the Chinese Research Policy Statement: Ethical Conduct for Research Involving Humans, the protocol was approved by the Human Research Ethics Board of Huaihe Hospital (#47), and all participants provided written informed consent.
Cell lines and cell culture
Human HCC cell lines (Huh-7 and HepG2) and an immortalized normal liver cell line MIHA were purchased from the American Type Culture Collection (ATCC, Manassas, VA, USA). All cell lines were cultured in Dulbecco's modified Eagle's medium (DMEM; Sigma, St. Louis, MO, USA) supplemented with 10% fetal bovine serum (FBS; Sigma), 100 μg mL−1 streptomycin and 100 U mL−1 penicillin. The incubation condition was 37 °C in a humidified atmosphere with 5% CO2.
Quantitative real-time polymerase chain reaction (RT-qPCR)
The total RNA of tissues or cells was isolated using TRIzol reagent (Invitrogen, Carlsbad, CA, USA). The reverse transcription was performed using a reverse transcription kit (Takara, Tokyo, Japan) and RT-qPCR was performed using a LightCycler 96 Instrument (Roche, Basel, Switzerland). The following primers were used: HOXB5, 5′-CCAATTTCACCGAAATAGACG-3′ (forward) and 5′-CGGTCATATCATGGCTGATG-3′ (reverse); β-actin, 5′-CTTCTTGGGTATGGAATCCTG-3′ (forward) and 5′-TCAGGAGGAGCAATGATCTTG-3′ (reverse). The reaction conditions were as follows: 35 cycles of 95 °C for 30 s, 62 °C for 30 s and 72 °C for 1 min. Relative gene expression was normalized to β-actin and calculated using the 2−ΔΔCt method.
Western blot analysis
Tissues or cells were lysed in lysis buffer and centrifuged at 12
000 × g for 15 min. The supernatant was extracted, separated by 10% SDS-PAGE and transferred onto PVDF membranes (Millipore, Billerica, MA, USA). The membranes were blocked with 5% skimmed milk for 2 h and incubated overnight at 4 °C with primary antibodies against HOXB5, p-PI3K, PI3K, p-Akt, Akt and β-actin. Subsequently, the membranes were washed three times with TBST and then incubated with appropriate secondary antibodies. All antibodies were purchased from Santa Cruz Biotechnology (Santa Cruz, CA, USA). Protein bands were visualized using the ECL reagent (Pierce, Rockford, IL, USA) and the density was quantified by the ImageJ software (NIH, Bethesda, MD, USA).
Cell transfection
Lentiviral packaging of recombinant vectors of lenti-shHOXB5-LV3 or lenti-shControl-LV3 was produced in 293T cells and HCC cells were transfected with these lentivirus. 48 h later, the cells were treated with puromycin. After 2 weeks, stably transfected clones were selected. Transfection efficiency was confirmed by western blot analysis.
Transwell assay
Transwell chambers with polycarbonate membrane filters (8 μm pore) were used for cell migration and invasion assays. For the migration assay, 1 × 104 cells were resuspended in serum-free DMEM and added to the upper chamber. The lower chamber was filled with culture media containing 10% FBS. After incubation for 48 h, cells remaining on the upper surface of the filter were scraped away with a cotton swab and cells migrating to the lower surface of the filter were fixed and stained with 0.1% crystal violet. The number of migrating cells in four random fields was counted under a microscope (200×). The invasion assay was performed according to steps similar to those for the migration assay, except that Matrigel-coated filters were used.
In vivo tumor metastasis assay
Four-week-old male nude mice were obtained from Shanghai Laboratory Animal Center (Shanghai, China). Mice were randomly divided into four groups (n = 5) and handled with approval of the Animal Care and Use Committee of Henan University. 2 × 106 Huh-7 cells transfected with shHOXB5 or shControl with or without treatment of TGF-β (10 ng mL−1) were resuspended in PBS and then injected into the tail vein of nude mice. 30 days later, mice were killed and their lungs were removed. The metastatic lung nodules were counted under a microscope. This study was performed in strict accordance with the NIH guidelines for the care and use of laboratory animals (NIH Publication no. 85-23 Rev. 1985) and was approved by the Institutional Animal Care and Use Committee of Huaihe Hospital of Henan University (Kaifeng, China).
Statistical analysis
Data were collected from three independent experiments and shown as means ± standard deviation (SD). Statistical analysis was performed using SPSS 13.0 software. Student's t-test was used for comparisons between two groups and one-way ANOVA was used for comparisons between three or more groups. P < 0.05 was considered statistically significant.
Conflicts of interest
The authors declare that they have no conflicts of interest.
Acknowledgements
This study was supported by the Scientific and Technological Project of Henan Province in China (No. 182102310367).
References
- A. Ananthakrishnan, V. Gogineni and K. Saeian, Epidemiology of Primary and Secondary Liver Cancers, Semin. Intervent. Radiol., 2006, 23, 47–63 CrossRef PubMed.
- V. V. Sahasrabuddhe, M. Z. Gunja, B. I. Graubard, B. Trabert, L. M. Schwartz, Y. Park, A. R. Hollenbeck, N. D. Freedman and K. A. Mcglynn, Nonsteroidal Anti-inflammatory Drug Use, Chronic Liver Disease, and Hepatocellular Carcinoma, J. Natl. Cancer Inst., 2012, 104, 1808–1814 CrossRef CAS PubMed.
- A. Jemal, F. Bray, M. M. Center, J. J. Ferlay, E. Ward and D. Forman, Global cancer statistics, Ca-Cancer J. Clin., 2011, 61, 69–90 CrossRef.
- A. Villanueva, New strategies in hepatocellular carcinoma: genomic prognostic markers, Clin. Cancer Res., 2010, 16, 4688–4694 CrossRef CAS.
- J. Belghiti and D. Fuks, Liver resection and transplantation in hepatocellular carcinoma, Liver Cancer, 2012, 1, 71–82 CrossRef CAS PubMed.
- H. Wang, C. Zhang, L. Xu, K. Zang, Z. Ning, J. Feng, H. Chi, X. Zhu and Z. Meng, Bufalin suppresses hepatocellular carcinoma invasion and metastasis by targeting HIF-1α via the PI3K/AKT/mTOR pathway, Oncotarget, 2016, 7, 20193–20208 Search PubMed.
- C. L. Chaffer and R. A. Weinberg, A perspective on cancer cell metastasis, Science, 2011, 331, 1559–1564 CrossRef CAS PubMed.
- D. A. Lawrence, Transforming growth factor-beta: a general review, Eur. Cytokine Network, 1996, 7, 363–374 CAS.
- E. Z. Ying, A special issue on TGF-β signaling and biology, Cell Biosci., 2011, 1, 1 CrossRef PubMed.
- M. H. Barcelloshoff and R. J. Akhurst, Transforming growth factor-β in breast cancer: too much, too late, Breast Cancer Res., 2009, 11, 202 CrossRef PubMed.
- A. Hata, Y. Shi and J. Massagué, TGF-beta signaling and cancer: structural and functional consequences of mutations in Smads, Mol. Med. Today, 1998, 4, 257–262 CrossRef CAS PubMed.
- M. Oft, K. H. Heider and H. Beug, TGF-β signaling is necessary for carcinoma cell invasiveness and metastasis, Curr. Biol., 1998, 8, 1243–1252 CrossRef CAS.
- B. Bierie and H. L. Moses, TGF beta: the molecular Jekyll and Hyde of cancer, Nat. Rev. Cancer, 2006, 6, 506–520 CrossRef CAS PubMed.
- J. Nourse, Chromosomal translocation t(1;19) results in synthesis of a homeobox fusion mRNA that codes for a potential chimeric transcription factor, Cell, 1990, 60, 535–545 CrossRef CAS.
- M. P. Kamps, C. Murre, X. H. Sun and D. Baltimore, A new homeobox gene contributes the DNA binding domain of the t(1;19) translocation protein in pre-B ALL, Cell, 1990, 60, 547–555 CrossRef CAS.
- W. J. Gehring and Y. Hiromi, Homeotic genes and the homeobox, Annu. Rev. Genet., 1986, 20, 147–173 CrossRef CAS PubMed.
- F. H. Ruddle, J. L. Bartels, K. L. Bentley, C. Kappen, M. T. Murtha and J. W. Pendleton, Evolution of Hox Genes, Annu. Rev. Genet., 1994, 28, 423–442 CrossRef CAS PubMed.
- M. P. Scott, Vertebrate homeobox gene nomenclature, Cell, 1992, 71, 551–553 CrossRef CAS PubMed.
- S. Bhatlekar, J. Z. Fields and B. M. Boman, HOX genes and their role in the development of human cancers, J. Mol. Med., 2014, 92, 811–823 CrossRef CAS.
- J. Y. Lee, H. Hur, H. J. Yun, Y. Kim, S. Yang, S. I. Kim and M. H. Kim, HOXB5 Promotes the Proliferation and Invasion of Breast Cancer Cells, Int. J. Biol. Sci., 2015, 11, 701–711 CrossRef CAS PubMed.
- B. Zhang, N. Li and H. Zhang, Knockdown of Homeobox B5 (HOXB5) Inhibits Cell Proliferation, Migration, and Invasion in Non-Small Cell Lung Cancer Cells Through Inactivation of the Wnt/β-Catenin Pathway, Oncol. Res., 2017, 26, 37–44 CrossRef PubMed.
- E. Fransvea, U. Angelotti, S. Antonaci and G. Giannelli, Blocking transforming growth factor-beta up-regulates E-cadherin and reduces migration and invasion of hepatocellular carcinoma cells, Hepatology, 2008, 47, 1557–1566 CrossRef CAS PubMed.
- K. H. Wrighton, X. Lin and X. Feng, Phospho-control of TGF-β superfamily signaling, Cell Res., 2009, 19, 8–20 CrossRef CAS.
- K. Mitsuo, P. Sumanth, W. Mei, Y. Hang, L. Linda, N. Indu, G. Amanda, N. Yoshimi, S. Hitoshi and T. Ivan, TGF-β activates Akt kinase via a microRNA-dependent amplifying circuit targeting PTEN, Nat. Cell Biol., 2009, 11, 881–889 CrossRef.
- K. C. Chen, C. Y. Chen, C. J. Lin, T. Y. Yang, T. H. Chen, L. C. Wu and C. C. Wu, Luteolin attenuates TGF-β1-induced epithelial–mesenchymal transition of lung cancer cells by interfering in the PI3K/Akt–NF-κB–Snail pathway, Life Sci., 2013, 93, 924–933 CrossRef CAS PubMed.
- S. Sheng and M. Qiao, Ab, metastasis and AKT activation, J. Cell. Physiol., 2009, 7, 2991–2996 Search PubMed.
- V. C. Lui, W. W. Cheng, T. Y. Leon, D. K. Lau, M. M. Garcia-Barcelo, X. P. Miao, M. K. Kam, M. T. So, Y. Chen and N. A. Wall, Perturbation of hoxb5 signaling in vagal neural crests down-regulates ret leading to intestinal hypoganglionosis in mice, Gastroenterology, 2008, 134, 1104–1115 CrossRef CAS PubMed.
- B. Lizen, B. Hutlet, D. Bissen, D. Sauvegarde, M. Hermant, M.T. Ahn and F. Gofflot, J. Comp. Neurol., 2017, 525, 1155–1175 CrossRef CAS PubMed.
- J. J. Zhu, M. K. Kam, M. M. Garcia-Barceló, P. K. Tam and V. C. Lui, HOXB5 binds to multi-species conserved sequence (MCS+9.7) of RET gene and regulates RET expression, Int. J. Biochem. Cell Biol., 2014, 51, 142–149 CrossRef CAS PubMed.
- J. Zhu, M. M. Garcia-Barcelo, P. K. Tam and V. C. Lui, HOXB5 cooperates with NKX2-1 in the transcription of human RET, PLoS One, 2011, 6, e20815 CrossRef CAS PubMed.
- C. S. Hong, O. Jeong, Z. Piao, C. Guo, M. R. Jung, C. Choi and Y. K. Park, HOXB5 induces invasion and migration through direct transcriptional upregulation of β-catenin in human gastric carcinoma, Biochem. J., 2015, 472, 393–403 CrossRef CAS PubMed.
- X. Gao, H. Zhao, C. Diao, X. Wang, Y. Xie, Y. Liu, J. Han and M. Zhang, miR-455-3p serves as prognostic factor and regulates the proliferation and migration of non-small cell lung cancer through targeting HOXB5, Biochem. Biophys. Res. Commun., 2017, 1074–1080 Search PubMed.
- E. M. Chambaz, S. Souchelnitskiy, S. Pellerin, G. Defaye, C. Cochet and J. J. Feige, Transforming Growth Factor-βs: A Multifunctional Cytokine Family, Horm. Res. Paediatr., 1996, 45, 222–226 CrossRef CAS.
- G. Guasch, M. Schober, H. A. Pasolli, E. B. Conn, L. Polak and E. Fuchs, Loss of TGFbeta signaling destabilizes homeostasis and promotes squamous cell carcinomas in stratified epithelia, Cancer Cell, 2007, 12, 313–327 CrossRef CAS PubMed.
- M. Joan, TGFbeta in Cancer, Cell, 2008, 134, 215–230 CrossRef PubMed.
- L. Sun, Tumor-suppressive and promoting function of transforming growth factor beta, Front. Biosci., 2004, 9, 1925–1935 CrossRef CAS.
- I. Matsuura, K. N. Chiang, C. Y. Lai, D. He, G. Wang, R. Ramkumar, T. Uchida, A. Ryo, K. Lu and F. Liu, Pin1 Promotes Transforming Growth Factor-β-induced Migration and Invasion, J. Biol. Chem., 2010, 285, 1754–1764 CrossRef CAS PubMed.
- J. J. Northey, Z. Dong, E. Ngan, A. Kaplan, W. R. Hardy, T. Pawson and P. M. Siegel, Distinct Phosphotyrosine-dependent Functions of the ShcA Adaptor Protein are Required for Transforming Growth Factor β (TGFβ)-induced Breast Cancer Cell Migration, Invasion, and Metastasis, J. Biol. Chem., 2013, 288, 5210–5222 CrossRef CAS PubMed.
- D. B. Kong, F. Chen and N. Sima, Focal adhesion kinases crucially regulate TGFβ-induced migration and invasion of bladder cancer cells via Src kinase and E-cadherin, OncoTargets Ther., 2017, 10, 1783–1792 CrossRef CAS PubMed.
- S. Lin, A mechanism for the switch of transforming growth factorbeta from a tumor suppressor to a tumor promoter in cancer progression, Dissertations & theses, Gradworks, 2012.
- E. Piek and A. B. Roberts, Suppressor and oncogenic roles of transforming growth factor-β and its signaling pathways in tumorigenesis, Adv. Cancer Res., 2001, 83, 1–54 CrossRef CAS.
- D. A. Chapnick, L. Warner, J. Bernet, T. Rao and X. Liu, Partners in crime: the TGFβ and MAPK pathways in cancer progression, Cell Biosci., 2011, 1, 42 CrossRef CAS.
- X. Zhang, H. Shi, H. Tang, Z. Fang, J. Wang and S. Cui, miR-218 inhibits the invasion and migration of colon cancer cells by targeting the PI3K/Akt/mTOR signaling pathway, Int. J. Mol. Med., 2015, 35, 1301–1308 CrossRef CAS PubMed.
- R. Zhou, L. Xu, M. Ye, M. Liao, H. Du and H. Chen, Formononetin inhibits migration and invasion of MDA-MB-231 and 4T1 breast cancer cells by suppressing MMP-2 and MMP-9 through PI3K/AKT signaling pathways, Horm. Metab. Res., 2014, 46, 753 CrossRef CAS PubMed.
- H. Niu, B. Wu, Y. Peng, H. Jiang, Y. Zhang, J. Wang and P. He, RNA interference-mediated knockdown of RhoGDI2 induces the migration and invasion of human lung cancer A549 cells via activating the PI3K/Akt pathway, Tumor Biol., 2015, 36, 409–419 CrossRef CAS PubMed.
- S. Lamouille and R. Derynck, Cell size and invasion in TGF-β–induced epithelial to mesenchymal transition is regulated by activation of the mTOR pathway, J. Cell Biol., 2007, 178, 437–451 CrossRef CAS PubMed.
Footnote |
† These authors contributed equally to this work. |
|
This journal is © The Royal Society of Chemistry 2018 |
Click here to see how this site uses Cookies. View our privacy policy here.