DOI:
10.1039/C8RA07283C
(Paper)
RSC Adv., 2018,
8, 35289-35293
An unexpected dual-response pH probe based on acridine†
Received
31st August 2018
, Accepted 10th October 2018
First published on 15th October 2018
Abstract
A new pH fluorescent probe 2,8-bis(acridin-9-ylethynyl)-6H,12H-5,11-methanodibenzo[b,f][1,5]diazocine (TBN), which has two acridine moieties attached to Tröger's base, is a useful fluorescent probe for monitoring extreme acidic and alkaline pH. TBN displays an excellent pH dependent behavior and responds linearly to extreme conditions in the pH ranges of 1.4–3.4 and 12.5–15.0. TBN can represent a novel type of fluorescent probe with perfect emission properties in extreme acidic and alkaline conditions by utilizing only one functional group.
Introduction
pH value plays a significant role in many fields, such as biological processes,1–3 environmental analysis,4,5 food production6,7 and life sciences.8–10 Moreover, as an important indication of cellular health, abnormal variation of pH may cause some serious diseases. Hence, the sensing and monitoring of pH is of great importance for understanding various pH-dependent physiological and pathological processes.
In recent years, a lot of methods have been applied to detect pH values, such as nuclear magnetic resonance,11,12 potentiometry,13,14 absorption spectroscopy15,16 and fluorescent probes.17,18 Among them, fluorescent probes have attracted much attention because of their quick response, excellent sensitivity, good selectivity, non-invasive detection and low cost.19 Till now, the improvements in synthetic methods have directed the development of fluorescent probes with many skeletons, including carbazole,20–22 BODIPY,23–25 rhodamine,26–28 cyanine,29 etc.
Most fluorescence-based pH probes can only exhibit response in weak acid range (pH = 4.5–6.0) or neutral range (pH = 6.8–7.4),30 but only a few fluorescent probes can work under extreme acidity or extreme alkalinity. Much less attention has been paid to fluorescent probes which are pH-sensitive in the lower pH region (pH < 5) or the higher pH region (pH > 9). Therefore, developing pH fluorescence probes with a wide detection range are becoming increasingly important.31
Tröger's base (TB), an old compound first synthesized in 1887, is still attracting wide attention owing to its unique properties as organic functional materials. Our group's recent research suggested that TB can be a good candidate to serve as a good electron-donating moiety in a fluorescent probe.32 Nowadays, increasing interest has been paid to acridine and its derivatives because of their biological activity and photophysical properties.33,34 However, the acridine chromophores were normally studied for acidic pH fluorescent probes.35 In this work, we reported a novel probe 2,8-diethynyl-6H,12H-5,11-methanodibenzo[b,f][1,5]diazocine (TBN) based on TB and acridine. Surprisingly, compound TBN is capable of measuring pH values at extreme conditions, specifically over the two pH ranges: 1.4–3.4 and 12.5–15.0, respectively. To the best of our knowledge, this is the first time that the acridine derivatives were used as an alkaline pH fluorescent probe.
Experimental section
Materials and apparatus
All chemicals were purchased from Adamas-beta® and TCI and used without further purification. 1H NMR and 13C NMR spectra were recorded on a Bruker AVANCE III MR spectrometer, and the chemical shifts (δ) were expressed in parts per million (ppm) and coupling constants (J) in Hertz. Mass spectra were determined with a Thermos Scientific Q Exactive LC-MS/MS system. The photoluminescence (PL) spectra were collected on a Lumina fluorescence spectrophotometer. Ultraviolet-visible (UV-vis) absorption spectra were performed on an Evolution 220 UV-vis spectrophotometer. Deionized water was obtained from a Milli-Q water purification system (Millipore). Thin layer chromatography (TLC) was performed on glass plates coated with 0.20 mm thickness of silica gel. pH values were measured using a PHSJ-3F pH meter (Shanghai LeiCi Device Works, Shanghai, China).
Synthesis and characterization of TBN
The synthetic route is depicted in Scheme 1. A mixture of 2,8-diethynyl-6H,12H-5,11-methanodibenzo[b,f][1,5]diazocine (130 mg, 0.48 mmol),36 9-bromoacridine37 (272 mg, 1.05 mmol), Pd(PPh3)4 (56 mg, 0.05 mmol) and CuI (10 mg, 0.05 mmol) were stirred at 85 °C under an atmosphere of N2 for 10 h. Ethyl acetate (10 mL) was added and the solution washed with water (3 × 10 mL) and brine (10 mL). The organic phase was separated, dried and evaporated. The reaction mixture was purified by column chromatography using dichloromethane and petroleum ether (4/1, v/v, Rf = 0.38) as the eluent to give a yellow solid. Yield: 53 mg (21%). 1H NMR (400 MHz, 1,1,2,2-tetrachloroethane-d2), δ (ppm): 4.34 (d, 2H, J = 17.3 Hz), 4.42 (s, 2H), 4.82 (d, 2H, J = 16.8 Hz), 7.29 (d, 2H, J = 8.3 Hz), 7.46 (s, 2H), 7.65 (t, 6H, J = 15.2 Hz), 7.84 (t, 4H, J = 14.8 Hz), 8.30 (d, 4H, J = 6.5 Hz), 8.52 (d, 4H, J = 8.6 Hz). 13C NMR (101 MHz, 1,1,2,2-tetrachloroethane-d2), δ (ppm): 58.42, 66.54, 83.57, 99.35, 105.36, 117.52, 120.17, 125.31, 126.21, 126.54, 128.18, 129.41, 130.49, 130.88, 131.27, 147.98, 149.32. HRMS (MALDI-TOF): [(M + H)+] calcd for C45H28N4: 625.2348; found: 625.2291.
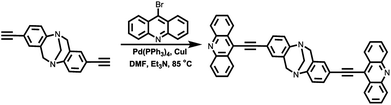 |
| Scheme 1 Synthetic route of TBN. | |
General procedure for spectroscopic measurements
The stock solution of TBN (10 μM) was prepared by dissolving TBN in a mixed solution (DMSO/H2O, v/v, 2/1). The metal ion solutions were prepared by using KCl, NaCl, CaCl2, MgCl2·6H2O, CuCl2·2H2O, MnCl2·4H2O, CoCl2·6H2O, NiCl2·6H2O, CdCl2, AlCl3, ZnCl2, CrCl3·6H2O, AgNO3, BaCl2, PbCl2, HgCl2. A small amount of HCl or NaOH was added to the solutions to modulate the pH values.
Results and discussion
Photophysical properties of probe TBN
In order to gain insight into the photophysical properties of the probe, we investigated the absorption and fluorescent spectra of TBN in various solvents with different polarities using 1.0 × 10−5 mol L−1 solutions. As is shown in Fig. 1, TBN shows similar absorption spectra and no obvious spectral shift were observed while the emission spectra exhibit quite different spectral lines which are not consistent with the general theoretical predictions of solvent effect.38 The reasons for the abnormal phenomenon are quite complicated and many factors such as changes in the probe's geometry, solute–solvent interactions and multichromophore aggregation can affect the solvatochromism.39 The acridine group and the amine on the TB skeleton act as electron acceptor and donor, respectively. This kind of structure enables each wing of TBN to form a D–π–A charge motif. Accordingly, the intense fluorescence of TBN in solution may owe to the effective intramolecular charge transfer (ICT) through strong push–pull interaction.
 |
| Fig. 1 Normalized (a) UV-vis and (b) PL spectra of TBN in solvents with different polarities. | |
UV-vis and fluorescence pH titrations
All samples in UV-vis and fluorescence experiments were performed in aqueous solution (2
:
1, DMSO/H2O, v/v) covering a broad spectrum of pH values ranging from 1.0 to 15.2 (Fig. 2 and 3). To better illustrate this issue, the absorption spectra were divided into two parts: one covers the pH from 15.2 to 7.0, the other covers the pH from 7.0 to 1.0 (Fig. S1†). As the pH is decreased from 15.2 to 7.0, the absorption intensity showed negligible increasing trend. Upon decreasing the pH from 7.0 to 1.0, the original absorption band at 437 nm decreased while the absorbance at around 353 nm, 372 nm and the absorption band between 470 nm and 503 nm gradually increased. In the meantime, the color was almost unchanged in alkaline pH. However, an apparent color change from viridescence to salmon pink was observed upon increasing the acidity which means that the probe has the potential to serve as a “naked-eye” colorimetric indicator for acidic pH.
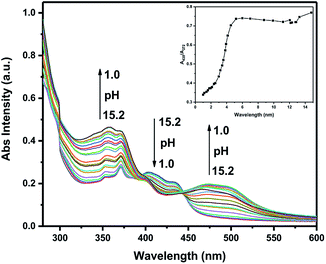 |
| Fig. 2 UV-vis absorption of TBN (10 μM in H2O/DMSO, v/v, 1/2) with different pH values (Inset: plot of ratio of I435/I372 as a function of pH). | |
 |
| Fig. 3 Fluorescence changes of TBN (10 μM) with (a) pH decreased from 15.2 to 11.1. Inset: the variation of fluorescence emission intensity at 480 nm of TBN with pH (pH 15.2–11.1), (b) pH decreased from 7.0 to 1.0. Inset: the variation of fluorescence emission intensity at 436 nm of TBN with pH (pH 7.0–1.0). Excitation at 320 nm, slit widths were set at 5 nm. | |
We found that probe TBN is responsive to both acid and alkaline which exceed our expectation. Because the emission spectra showed little change upon decreasing the pH from 11.1 to 7.0 (Fig. S2†), the emission spectra were also divided into two parts: one covers the pH from 15.2 to 11.1, the other covers the pH from 7.0 to 1.0 (Fig. 3). The TBN showed three emission peaks at 413 nm, 437 nm and 463 nm when the pH values are below 11.1. With the gradual increase of pH value from 11.1 to 15.2, the fluorescent intensity of TBN at 413 nm was reduced. Meanwhile, two peaks and a shoulder appeared at 453 nm, 480 nm and 509 nm. Additionally, the fluorescence changes under acid conditions were also examined. As shown in Fig. 3b, upon decreasing the pH value from 7.0 to 1.0, an evident decrease of the three fluorescent emission peaks was observed with no obvious spectral shift. The fluorescence change was well demonstrated in the fluorescence photos which were shown in the inset of Fig. S2(a).† Good linearity between fluorescent intensity and pH over the range 1.4 to 3.4 and 12.5 to 15.0 was observed by fluorescence titration experiments (Fig. 4). As a result, probe TBN can measure extreme pH values over the two pH ranges 1.0–7.0 and 11.1–15.2, respectively.
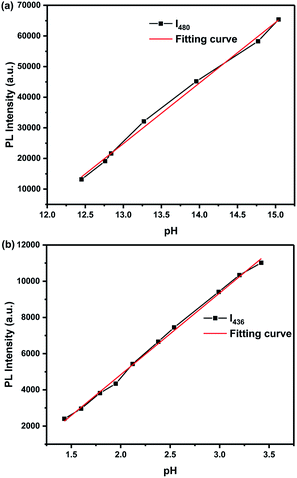 |
| Fig. 4 (a) Fluorescence intensity of probe TBN (10 μM, DMSO/H2O, v/v, 2/1) solution as a function of pH from 12.5 to 15.0. Y = −232351 + 19784X, R2 = 0.9952. (b) Fluorescence intensity of probe TBN (10 μM, DMSO/H2O, v/v, 2/1) solution as a function of pH from 1.4 to 3.4. Y = −4180 + 4510X, R2 = 0.9975. λex = 320 nm. | |
Stability, reversibility and selectivity of TBN
In order to determine the impact of time on this detection process, an estimation of the time-dependent fluorescence spectral changes of probe TBN at different pH values was carried out. As shown in Fig. S4,† the fluorescence emission responded immediately and the emission intensity remained stable over a long time.
The reversibility is considered as an essential property of a practical fluorescent probe, so the pH value was modulated repeatedly between 1.0 and 7.0, 11.1 and 15.2 five times, and the corresponding emissions were monitored. As shown in Fig. 5, when solutions containing probe TBN were changed to low or high pH, no obvious variation in the fluorescence intensity was observed after five cycles. As illustrated in Fig. S3,† the switch of fluorescence signals was also highly reversible between pH 15.2 and 1.0. Besides, the change of solution fluorescence is visible to the naked eyes. The fast and reversible response to pH fluctuation is necessary for real-time monitoring.
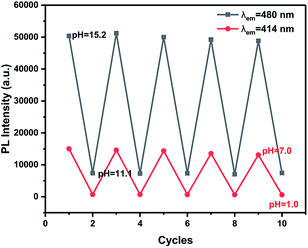 |
| Fig. 5 The pH reversibility of probe TBN. | |
The selectivity of probe TBN toward pH was studied by measuring the fluorescence responses to other biologically relevant species including some metal ions and bioactive small molecules at pH 13.8, 7.1, and 1.5, respectively. As shown Fig. 6, no noticeable change was observed in the fluorescence intensity of probe TBN with the addition of various interferents, suggesting that the high selectivity of probe TBN toward pH.
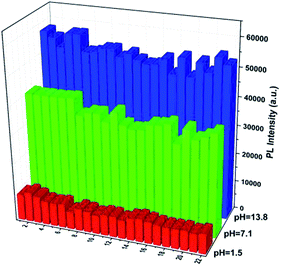 |
| Fig. 6 Fluorescence intensity of 10 μM TBN at different pH in the presence of various species: (1) blank; (2) K+ (150 mM); (3) Na+ (150 mM); (4) Ca2+ (0.2 mM); (5) Mg2+ (2 mM); (6) Zn2+ (0.2 mM); (7) Cu2+ (0.2 mM); (8) Mn2+ (0.2 mM); (9) Co2+ (0.2 mM); (10) Cr3+ (0.2 mM); (11) Cd2+ (0.2 mM); (12) Ni2+ (0.2 mM); (13) Al3+ (0.2 mM); (14) Hg2+(0.2 mM); (15) histidine (0.2 mM); (16) tyrosine (0.2 mM); (17) arginine (0.2 mM); (18) glutamic acid (0.2 mM); (19) lysine (0.2 mM); (20) threonine (0.2 mM); (21) glycine (0.2 mM); (22) glucose (0.2 mM). Fluorescence intensity at 480 nm at pH 13.8, fluorescence intensity at 436 nm of pH 7.1 and 1.5. | |
Proposed mechanism
In order to get insight into the sensing mechanism of probes TBN, 1H NMR experiments were carried out under acidic and alkaline conditions in DMSO-d6. As illustrated in Fig. 7, upon addition of hydrochloric acid, the chemical of H1–H4 exhibited an apparent downfield shift. However, the chemical shifts of H8–H10 had little change, which indicated that the protonation occurred at the N atoms in acridine rather than TB skeleton. The downfield chemical shift of these protons was obviously due to H+ binding with N atom of acridine, which enhanced the electron-withdrawing effect, and decreased the electron density of the aromatic ring, leading to the downfield shift of the proton signals. Under the alkaline conditions, all the proton signals of the conjugation system showed little change. To understand this phenomenon better, we introduced 2,8-diethynyl-6H,12H-5,11-metanodibenzo[b,f][1,5]diazocine (TBAN1) to make a comparison.40 Interestingly, we found that TBAN1 showed no response to pH (Fig. S5†). Therefore, it can be concluded that the pH response does not from carbon–carbon triple bonds and the N atoms in the TB skeleton of TBN. Moreover, considering the little shift of the alkaline conditions and negligible increasing trend of absorption spectra when the pH decreased from 15.2 to 7.0, it can be regarded as merely a weak interaction between hydroxyl ions and TBN molecules. It was reported41 that acridine emits blue fluorescence only in protic solvents at pH > 11. Weller42 concluded only the hydrogen-bonded complex between excited acridine and solvent molecules can emit the blue fluorescence. In our case, hydrogen-bonded complex has not been formed in solutions when pH < 11.1, TBN molecules in the ground state and protonated TBN ions showed purple fluorescence upon irradiation under this condition. In extreme alkaline solutions (pH > 11.1), a rise of the basicity leading to the formation of hydrogen-bonded complex between excited TBN dye and solvent molecules which emit intense blue fluorescence upon irradiation.
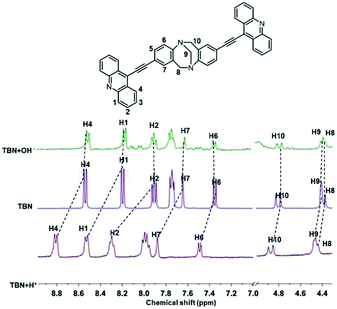 |
| Fig. 7 Partial 1H NMR spectrum of TBN, TBN + H+ and TBN + OH− in DMSO-d6. | |
Conclusion
In conclusion, we have designed and synthesized an acridine-based fluorescent pH probe TBN with excellent selectivity, good stability and high sensitivity. This probe can monitor not only extreme acidic pH but also extreme alkaline pH. The pH titrations demonstrated that probe TBN showed an apparent emission decline as the pH decreased from 7.0 to 1.0 and an obvious emission enhancement as the pH increased from 11.1 to 15.2. This probe responds linearly to the extreme acidic range of 1.4–3.4 and to the extreme alkaline range of 12.5–15.0. It is imperative that TBN can represent a novel type of fluorescent probe with perfect emission property in the extreme acidic and alkaline conditions by utilizing only one functional group.
Conflicts of interest
There are no conflicts to declare.
Acknowledgements
The authors thank the help from Professor Rui Liu in Tongji University on the testing of fluorescence and UV-vis spectra. We gratefully acknowledge the financial support from the state National Natural Science Foundation of China (Grant No. 51403157) and the Fundamental Research Funds for the Central Universities.
Notes and references
- W. Niu, L. Fan, M. Nan, Z. Li, D. Lu, M. Wong, S. Shuang and C. Dong, Anal. Chem., 2015, 87, 2788 CrossRef CAS PubMed.
- N. I. Georgiev, A. I. Said, R. A. Toshkova, R. D. Tzoneva and V. B. Bojinov, Dyes Pigm., 2019, 160, 28 CrossRef CAS.
- Y. Ge, A. Liu, J. Dong, G. Duan, X. Cao and F. Li, Sens. Actuators, B, 2017, 247, 46 CrossRef CAS.
- R. S. Spaulding, M. D. DeGrandpre, J. C. Beck, R. D. Hart, B. Peterson, E. H. Carlo, P. S. Drupp and T. R. Hammar, Environ. Sci. Technol., 2014, 48, 9573 CrossRef CAS PubMed.
- C. Jeschke, C. Falagán, K. Knöller, M. Schultze and M. Koschorreck, Environ. Sci. Technol., 2013, 47, 14018 CrossRef CAS PubMed.
- O. Schlüter, B. Rumpold, T. Holzhauser, A. Roth, R. F. Vogel, W. Quasigroch, S. Vogel, V. Heinz, H. Jäger, N. Bandick, S. Kulling, D. Knorr, P. Steinberg and K. Engel, Mol. Nutr. Food Res., 2017, 61, 1600520 CrossRef PubMed.
- A. Terpou, A. Gialleli, L. Bosnea, M. Kanellaki, A. A. Koutinas and G. R. Castro, LWT--Food Sci. Technol., 2017, 79, 616 CrossRef CAS.
- Y. Yue, F. Huo, S. Lee, C. Yin and J. Yoon, Analyst, 2017, 142, 30 RSC.
- J. Chao, K. Song, Y. Zhang, C. Yin, F. Huo, J. Wang and T. Zhang, Talanta, 2018, 189, 150s CrossRef PubMed.
- J. Chao, K. Song, H. Wang, Z. Li, Y. Zhang, C. Yin, F. Huo, J. Wang and T. Zhang, RSC Adv., 2017, 7, 964 RSC.
- R. V. Shchepin, D. A. Barskiy, A. M. Coffey, T. Theis, F. Shi, W. S. Warren, B. M. Goodson and E. Y. Chekmenev, ACS Sens., 2016, 1, 640 CrossRef CAS PubMed.
- G. A. Rothbauer, E. A. Rutter, C. Reuter-Seng, S. Vera, E. J. Billiot, Y. Fang, F. H. Billiot and K. F. Morris, J. Surfactants Deterg., 2018, 21, 139 CrossRef CAS.
- L. Truche, E. F. Bazarkina, G. Berger, M. Caumon, G. Bessaque and J. Dubessy, Geochim. Cosmochim. Acta, 2016, 177, 238 CrossRef CAS.
- Z. Guo, Y. Wang, A. Yang and G. Yang, Soft Matter, 2016, 12, 6669 RSC.
- R. G. Zhang, S. G. Kelsen and J. C. Lamanna, J. Appl. Physiol., 1990, 68, 1101 CrossRef CAS PubMed.
- J. Han and K. Burgess, Chem. Rev., 2010, 110, 2709 CrossRef CAS PubMed.
- F. Zhao, L. Zhang, A. Zhu, G. Shi and Y. Tian, Chem. Commun., 2016, 52, 3717 RSC.
- A. R. Sarkar, C. H. Heo, L. Xu, H. W. Lee, H. Y. Si, J. W. Byun and H. M. Kim, Chem. Sci., 2016, 7, 766 RSC.
- Y. Ni and J. Wu, Org. Biomol. Chem., 2014, 12, 3774 RSC.
- L. Fan, S. Gao, Z. Li, W. Niu, W. Zhang, S. Shuang and C. Dong, Sens. Actuators, B, 2015, 221, 1069 CrossRef CAS.
- F. Miao, G. Song, Y. Sun, Y. Liu, F. Guo, W. Zhang, M. Tian and X. Yu, Biosens. Bioelectron., 2013, 50, 42 CrossRef CAS PubMed.
- H. Lu, B. Xu, Y. Dong, F. Chen, Y. Li, Z. Li, J. He, H. Li and W. Tian, Langmuir, 2010, 26, 6838 CrossRef CAS PubMed.
- J. Zhang, M. Yang, C. Li, N. Dorh, F. Xie, F. Luo, A. Tiwari and H. Liu, J. Mater. Chem. B, 2015, 3, 2173 RSC.
- J. Qiu, S. Jiang, H. Guo and F. Yang, Dyes Pigm., 2018, 157, 351 CrossRef CAS.
- L. Wang, M. Cui, H. Tang and D. Cao, Anal. Methods, 2018, 10, 1633 RSC.
- S. Shen, X. Chen, X. Zhang, J. Miao and B. Zhao, J. Mater. Chem. B, 2015, 3, 919 RSC.
- H. Yu, G. Li, B. Zhang, X. Zhang, Y. Xiao, J. Wang and Y. Song, Dyes Pigm., 2016, 133, 93 CrossRef CAS.
- X. Zhao, X. Chen, S. Shen, D. Lia, S. Zhou, Z. Zhou, Y. Xiao, G. Xia, J. Miao and B. Zhao, RSC Adv., 2014, 4, 50318 RSC.
- Y. Yue, F. Huo, S. Lee, C. Yin, J. Yoon, J. Chao, Y. Zhang and F. Cheng, Chem.–Eur. J., 2017, 22, 1239 CrossRef PubMed.
- J. Chao, H. Wang, K. Song, Z. Li, Y. Zhang, C. Yin, F. Huo, J. Wang and T. Zhang, Tetrahedron, 2016, 72, 8342 CrossRef CAS.
- J. Chao, H. Wang, Y. Zhang, Z. Li, Y. Liu, F. Huo, C. Yin and Y. Shi, Anal. Chim. Acta, 2017, 975, 52 CrossRef CAS PubMed.
- C. Yuan, Y. Zhang, H. Xi and X. Tao, RSC Adv., 2017, 7, 55577 RSC.
- C. Felip-León, O. Martínez-Arroyo, S. Díaz-Oltra, J. F. Miravet, N. Apostolova and F. Galindo, Bioorg. Med. Chem. Lett., 2018, 28, 869 CrossRef PubMed.
- L. R. Daghigh, M. Pordel and A. Davoodnia, J. Chem. Res., 2014, 38, 202 CrossRef CAS.
- S. Chemate, Y. Erande, D. Mohbiya and N. Sekar, RSC Adv., 2016, 6, 84319 RSC.
- W. Li and T. Michinobu, Macromol. Chem. Phys., 2016, 217, 863 CrossRef CAS.
- E. C. Constable, C. E. Housecroft, M. Neuburger and C. X. Schmitt, Polyhedron, 2006, 25, 1844 CrossRef CAS.
- J. J. Aaront and M. MAAFI, Spectrochim. Acta, 1995, 51A, 603 CrossRef.
- D. Zhang, Y. Gao, J. Dong, Q. Sun, W. Liu, S. Xue and W. Yang, Dyes Pigm., 2015, 113, 307 CrossRef CAS.
- L. Xu, Y. Li, X. Yan and C. Yuan, Tetrahedron, 2018, 74, 4738 CrossRef CAS.
- E. J. Bowen, N. J. Holder and G. B. Woodger, J. Phys. Chem., 1962, 66, 2491 CrossRef CAS.
- A. Weller, Z. Elektrochem., 1957, 61, 956 CAS.
Footnote |
† Electronic supplementary information (ESI) available. See DOI: 10.1039/c8ra07283c |
|
This journal is © The Royal Society of Chemistry 2018 |
Click here to see how this site uses Cookies. View our privacy policy here.