DOI:
10.1039/C7SC05019D
(Edge Article)
Chem. Sci., 2018,
9, 2437-2442
Is the kernel–staples match a key–lock match?†
Received
23rd November 2017
, Accepted 28th January 2018
First published on 29th January 2018
Abstract
Metal nanoclusters provide excellent references for understanding metal nanoparticle surfaces, which remain mysterious due to the difficulty of atomically precise characterization. Although some remarkable advances have been achieved for understanding the structure of metal nanoclusters, it is still unknown if the inner kernel–outer staples match is a key–lock match and how the surface staples influence some of the properties of metal nanoclusters. Herein, we have developed an acid-induction method for synthesizing a novel gold nanocluster whose composition is determined to be Au42(TBBT)26 (TBBT: 4-tert-butylbenzenelthiolate) by ESI-MS and single-crystal X-ray crystallography (SCXC). SCXC also reveals that Au42(TBBT)26 has an identical kernel but different staples with an existing gold nanocluster Au44(TBBT)28, indicating that the kernel–staples match is not a key–lock match and the existence of homo-ligand–homo-kernel–hetero-staples phenomenon in metal nanoclusters provides some reference for understanding the growth or transformation of metal nanoclusters. Further experiments reveal that the staples greatly contribute to the stability of gold nanoclusters and influence their photoluminescence intensity and that minute differences in the interfacial structure can lead to enhanced stability and photoluminescence.
Introduction
Ligand-protected metal nanoparticles have received extensive interest not only for fundamental scientific research but also for their practical applications over the past several decades.1 However, the surfaces of the nanoparticles remain mysterious due to the difficulty of atomically precise characterization. The recently emergent metal nanoclusters2 provide excellent references for understanding metal nanoparticle surfaces because the compositions of the metal nanoclusters can be precisely determined by mass spectrometry and because the total structures of metal nanoclusters can be resolved by single-crystal X-ray crystallography (SCXC). To date, over a dozen metal nanoclusters2p,3 have been structurally resolved by SCXC, which reveals that metal nanoclusters consist of pure metal kernels and ligand-containing staples (herein, the bridging thiolates are also included in the staples for simplification). It is believed that the kernel is protected from aggregation or decomposition by the staples;4 thus, the staples should match the kernel, otherwise the nanocluster cannot be stable.
Moreover, some theoretical calculations indicate that the protecting staple motifs should fit the curvature of the kernel of the nanoclusters.5 Essential questions include the following: is this match a key–lock match (i.e., if the metal, the thiolate and the kernel are all fixed, are the staples exclusive)? If not, will a variation of the staples lead to great change in the stability and other properties (e.g., photoluminescence) of nanoclusters? These questions are important to understand the interaction between staples and the kernel, the staples' influence on the properties, and the growth (or transformation) mechanism of metal nanoclusters.6 To address these questions, we have developed a method to synthesize a novel gold nanocluster protected by 4-tert-butylbenzenelthiolate (TBBT), which has identical Au34 kernels but varied staples with an existing gold nanocluster-Au44(TBBT)28, and we compare the stability and photoluminescence properties between the two homo-kernelled gold nanoclusters.
Results and discussion
The synthesis of Au42(TBBT)26 nanoclusters was according to the method described by us in our previous study.3v Some modifications were made to this method, and the details are provided in the experimental section. Briefly, tetraoctylammonium bromide (TOAB) was dissolved in ethyl acetate in a tri-neck flask, and HAuCl4·4H2O was added to the solution. After the solution was stirred for 10 min, 4-tert-butylbenzenelthiol was added. When the color of the solution changed from deep red to yellow, an acetic acid-water mixture was added, and then, a cold water solution of NaBH4 was added all at once immediately. Please note that without the addition of acid, the Au42(TBBT)26 nanoclusters cannot be obtained, and very recently, we synthesized a rare aliphatic ligand-stabilized gold nanocluster with an fcc structure by similar protocols except that acetic acid was replaced by nitric acid in the reaction, indicating that these synthesis protocols are not exclusive and can be developed to a method (tentatively dubbed acid-induction synthesis method) for synthesizing novel nanoclusters that are otherwise difficult to obtain. Herein, acetic acid could also be replaced by other acids (nitric acid, hydrochloric acid, etc.), however, it showed better reproducibility compared with the other investigated acids in this work. Acetic acid played at least two roles: on one hand, acetic acid increased the hydrolysis of NaBH4 and strengthened the reduction of NaBH4;7 on the other hand, it could weaken the interaction between Au and thiolate, reducing the reactivity of thiolate. Thus, the attack of acid probably influenced the kinetics and thermodynamics of the formation of nanocluster products8 similar to that in a previous work reported by Xie et al.9 who added a base instead to alter the reaction kinetics and thermodynamics. The growth of gold nanoclusters in the reaction was allowed to proceed for 2 h for enhancement in size.10 The crude product was collected and thoroughly washed with methanol, and Au42(TBBT)26 nanoclusters were separated by preparative thin-layer chromatography (PTLC).3j,11
The precise composition of the Au42(TBBT)26 nanocluster was determined by electrospray ionization mass spectrometry (ESI-MS).3g,3k,8,12 To impart charges, cesium acetate (CsOAC) was added to the nanocluster solution to form cationic cluster adducts with Cs+. The ESI-MS spectrum showed intense peaks centered at m/z 6418.44 (see Fig. S1†), corresponding to the [Au42(TBBT)26Cs2]2+ species (calculated: 6418.17 Da, deviation: 0.27 Da). Because the charge number was equal to the number of adducted Cs+ ions, the Au42(TBBT)26 nanoclusters were proposed to be charge-neutral, which is supported by the fact that no sound signals were found in the mass spectrum without the addition of CsOAC and that the counter ions were not found by SCXC. Dark rhombic single crystals were formed in a mixed solution of toluene and acetonitrile after 3 days. The total structure of Au42(TBBT)26 was resolved by SCXC. The Au42(TBBT)26 crystal structure adopts the triclinic space group P-1. Similar to its counterpart, Au44(TBBT)28,3p Au42(TBBT)26 has a pair of enantiomeric nanoclusters in the unit cell. The left-handed isomer was chosen to explore the details of the atom packing structure of Au42(TBBT)26 and Au44(TBBT)28.
The total structure of Au42(TBBT)26 is shown in Fig. 1A. The kernel of Au42(TBBT)26 is composed of four cuboctahedra, which are formed by 34 gold atoms, as shown in Fig. 1B, and it is identical to the kernel of Au44(TBBT)28 (ref. 3p) (see Fig. 1E; the total structure of Au44(TBBT)28 is shown in Fig. 1D). Please note that there are some other kernel assignments on the basis of different views.3p In another view, the two kernels adopt identical FCC packing as shown in Fig. 1C and F. However, although the two gold nanoclusters have identical Au34 kernels and protecting thiolate (TBBT), they have different staples. To better illustrate this, we give a detailed structural interpretation of the staples' species and adsorption sites (see Fig. 2).
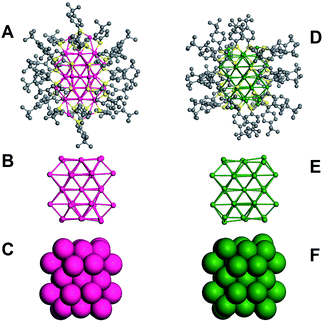 |
| Fig. 1 Total structure and Au34 kernels of Au42(TBBT)26 (A–C) and Au44(TBBT)28 (D–F). | |
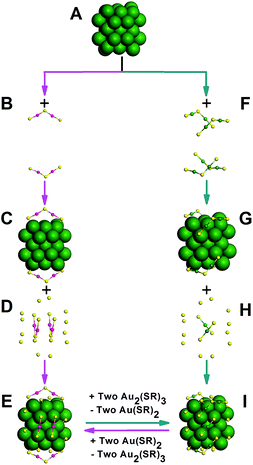 |
| Fig. 2 Surface staples comparison of Au42(TBBT)26 and Au44(TBBT)28: Au34 kernel (A); illustrating the anatomy of two Au2(TBBT)3, four Au(TBBT)2 and 12 TBBT capped on the kernel of Au42(TBBT)26 (from B to E); illustrating the anatomy of four Au2(TBBT)3, two Au(TBBT)2 and 12 TBBT capped on the kernel of Au44(TBBT)28 (from F to I). For clarity, C and H are omitted. Color labels: yellow = S; others = Au. | |
The kernel of Au44(TBBT)28 is protected by four Au2(TBBT)3 dimers at the top and bottom and two Au(TBBT)2 monomers at the waist (Fig. 2F–I).3p In contrast, there are two Au2(TBBT)3 dimers at the top and bottom of the Au42(TBBT)26 nanocluster and four Au(TBBT)2 monomers at the waist (Fig. 2B–E). Of note, there are also 12 bridging thiolates for every nanocluster (see Fig. 2D and H). In addition to quantitative differences in the staples, the surface arrangement of the staples is varied as mentioned above. In Au42(TBBT)26, the bridging thiolates and dimeric staples anchor the {100} facets (Fig. 3A), and each of the monomer staples anchor each {110} facet (Fig. 3C), with no staples anchoring the {111} facet (Fig. 3B). However, in Au44(TBBT)28, the {100}, {111} and {110} facets are protected by bridging thiolates, a dimeric staple and monomeric staple, respectively (Fig. 3D–F). Please note that each {111} facet is protected by one dimeric staple and each {100} square is protected by one bridging thiolate in the magic cluster series including Au28(TBB)20, Au36(TBBT)24, Au44(TBBT)28 and Au52(TBBT)32.3p
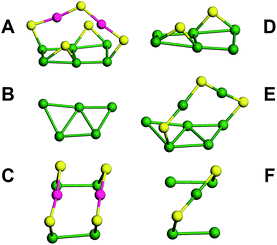 |
| Fig. 3 Staples and bridging thiolates on the crystalline facets of Au42(TBBT)26 and Au44(TBBT)28; bridging thiolates, a dimeric staple and monomeric staple on the {100}, {111} and {110} facets of Au42(TBBT)26, respectively (A, B and C); bridging thiolates, a dimeric staple and monomeric staple on the {100}, {111} and {110} facets of Au44(TBBT)28, respectively (D, E, and F). Color labels: yellow = S; others = Au. | |
The average Au–S bond length of the staples in Au42(TBBT)26 (2.31 Å) is slightly shorter than that in Au44(TBBT)28 (2.33 Å).3p The average Au–S–Au and S–Au–S bond angles of the staples in Au42(TBBT)26 (112.6° and 171.2°, respectively) are different from those in Au44(TBBT)28 (100.1° and 171.8°, respectively). Please note that the bridging thiolates are not considered in the above comparisons.
Based on the above discussion, Au42(TBBT)26 has an identical Au34 kernel but different staples, which indicates that the kernel–staples match is not a key–lock match and that the homo-ligand–homo-kernel–hetero-staples phenomenon exists in metal nanoclusters, which has not been previously reported to the best of our knowledge. Of note, it is known that the hetro-ligand–homo-kernel–hetero-stapled metal nanoclusters have been known for some time.3f,12b,13 The search for the homo-ligand–homo-kernel–hetero-staples phenomenon in metal nanoclusters indicates that the protecting staples on the kernel have some flexibility, which may provide some reference for understanding the growth or transformation of metal nanoclusters.6 The pair of homo-ligand–homo-kernel–hetero-stapled nanoclusters Au42(TBBT)26 and Au44(TBBT)28 is ideal materials to investigate the influence that the staples have on nanocluster properties without interferences from some other factors (e.g. ligands). As discussed above, it is believed that the staples protect the kernels from aggregation or decomposition. This is indeed indicated by our stability experiments, which reveal that Au44(TBB)28 in toluene is stable for at least 72 hours under 80 °C monitored by UV/vis/NIR spectrometry, whereas the less-stapled Au42(TBBT)26 is not stable for even 10 min under similar conditions (see Fig. 4). It is worth noting that there are some other perspectives to interpret the stability of metal nanoclusters.2a,14
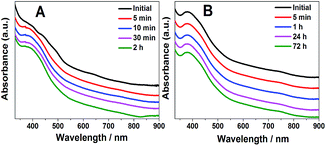 |
| Fig. 4 Thermostability of Au42(TBBT)26 (A) and Au44(TBBT)28 (B). | |
Photoluminescence is an intriguing phenomenon, and the photoluminescence of gold/silver nanoclusters has recently received increasing attention not only for fundamental scientific research but also for practical applications in a large range of fields such as sensors2i,2l,15 and biomedicine.2d,15f,16 For fundamental scientific research, studies on the photoluminescence mechanism of gold/silver nanoclusters are of major importance, and there have been some pre-eminent contributions from different groups. For instance, Murray's group17 found that the luminescence intensity of Au25(SR)18 increases linearly with the increasing number of polar ligands on the nanoclusters. Additionally, Wu and Jin18 suggested that surface ligands can influence the photoluminescence by charge transfer and direct delocalized electron donation. Moreover, Xie et al.19 found that aggregation-induced emission (AIE) also exists in gold nanoclusters, and Lee's group20 revealed that the rigidity of the Au(I)-thiolate shell (staple) is beneficial to the emission of gold nanoclusters. Recently, our group16g discovered that both interlocked Au4(SR)5 staples and strengthened interactions between the kernel and the thiolates contribute to the enhanced photoluminescence of Au24(SR)20 nanoclusters. Even more recently, theoretical calculations conducted by Aikens and colleagues showed that the photoluminescence of gaseous Au25(SR)18− originates from kernel-based electron transitions rather than charge-transfer or semi-ring states.21 Despite these distinguished contributions, the understanding of the photoluminescence mechanism of gold/silver nanoclusters is far from complete, and a major puzzle lies in the real roles of the kernel and staples in emission. This issue is challenging because the kernels and staples cannot be isolated and investigated separately. Thus, the finding of homo-ligand–homo-kernel–hetero-stapled gold nanoclusters might provide an opportunity to understand this issue. Although homo-kernelled, Au42(TBBT)26 and Au44(TBBT)28 (ref. 3p) have only slight difference in staples and show big difference in emission intensities: the more-stapled Au44(TBBT)28 exhibits ∼2 times stronger photoluminescence than the less-stapled Au42(TBBT)26 (see Fig. 5), indicating that the staples greatly contribute to the photoluminescence intensity of metal nanoclusters, which is also supported by the previous observations that ligands12c,18 and solvents3t have important influence on the photoluminescence intensities of metal nanoclusters. The two nanoclusters have different excitation spectra, however, they have a close maximum excitation wavelength at ∼470 nm (Fig. S2†). They have also similar emission profiles when excited at 514 nm although their emission intensities are pre-eminently different (Fig. 5). In addition, the photoluminescence lifetimes of Au44(TBBT)28 (15.59 ns (15.23%) and 71.99 ns (84.77%)) are close to those of Au42(TBBT)26 (14.96 ns (13.10%) and 89.00 ns (86.90%)) (see Fig. S3†). Taken together, these results indicate that the two nanoclusters have a similar photoluminescence mechanism. Please note that a high Au(I)/Au(0) ratio in the nanoclusters cannot be the cause of stronger photoluminescence observed in our research because Au42(TBBT)26 has a higher Au(I)/Au(0) ratio than Au44(TBBT)28 (see Fig. S4†) but less photoluminescence intensity than that of Au44(TBBT)28.19
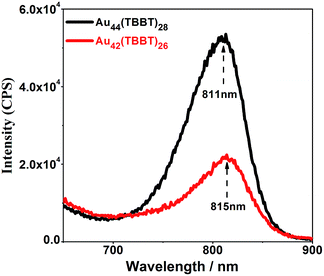 |
| Fig. 5 Photoluminescence spectra of Au44(TBBT)28 and Au42(TBBT)26. | |
Conclusions
In summary, an acid-induction method was developed to synthesize a novel gold nanocluster Au42(TBBT)26 whose composition was determined by using ESI-MS and SCXC and whose structure was resolved by SCXC. The as-obtained gold nanocluster had an identical Au34 kernel but reduced staples compared with those of the existing Au44(TBBT)28 nanocluster, indicating that the kernel–staples match was not a key–lock match and the existence of homo-ligand–homo-kernel–hetero-staples phenomenon in metal nanoclusters. The two Au-SR units' reduction led to a decreased stability of Au42(TBBT)26 compared with that of Au44(TBBT)28, thus experimentally confirming the importance of the staples in protecting the kernels of metal nanoclusters from aggregation or decomposition. Furthermore, the reduced staples resulted in a photoluminescence decrease by ∼2 times, indicating that the staples greatly influenced the photoluminescence intensity of metal nanoclusters. The novelty and significance of this work lie in the following: (i) an acid-induction method was developed to synthesize a novel gold nanocluster Au42(TBBT)26; (ii) the structure of Au42(TBBT)26 was resolved by SCXC; (iii) the structure comparison between Au44(TBBT)28 and Au42(TBBT)26 indicated the existence of homo-ligand–homo-kernel–hetero-staples phenomenon in metal nanoclusters and that the kernel–staples match is not a key–lock match, which might have some important implication for understanding the growth or transformation of metal nanoclusters; (iv) it experimentally confirmed that the staples stabilize the gold nanoclusters; and (v) it was first unambiguously demonstrated that the staples greatly influence the photoluminescence intensity without interferences from other factors (e.g. ligands), which may have important implications on the understanding of the photoluminescence mechanism of metal nanoclusters. It is expected that our work will trigger more studies on the synthesis, structures and properties of metal nanoclusters and have important implications on understanding the kernel–staples interaction, staples-properties correlation and growth (or transformation) mechanism of metal nanoparticles.
Conflicts of interest
There are no conflicts to declare.
Acknowledgements
The authors would like to thank the National Natural Science Foundation of China (no. 21222301, 21528303, 21603234, 21771186, 21171170, 21501182 and 21601193), and the CAS/SAFEA International Partnership Program for Creative Research Teams for financial support.
Notes and references
-
(a) M.-C. Daniel and D. Astruc, Chem. Rev., 2004, 104, 293–346 CrossRef CAS PubMed;
(b) N. L. Rosi and C. A. Mirkin, Chem. Rev., 2005, 105, 1547–1562 CrossRef CAS PubMed;
(c) G. Schmid, Chem. Soc. Rev., 2008, 37, 1909–1930 RSC;
(d) A. R. Tao, S. Habas and P. Yang, Small, 2008, 4, 310–325 CrossRef CAS.
-
(a) M. Walter, J. Akola, O. Lopez-Acevedo, P. D. Jadzinsky, G. Calero, C. J. Ackerson, R. L. Whetten, H. Gronbeck and H. Hakkinen, Proc. Natl. Acad. Sci. U. S. A., 2008, 105, 9157–9162 CrossRef CAS PubMed;
(b) R. Jin, Nanoscale, 2010, 2, 343–362 RSC;
(c) J. F. Parker, C. A. Fields-Zinna and R. W. Murray, Acc. Chem. Res., 2010, 43, 1289–1296 CrossRef CAS PubMed;
(d) S. Choi, R. M. Dickson and J. Yu, Chem. Soc. Rev., 2012, 41, 1867–1891 RSC;
(e) Y. Lu and W. Chen, Chem. Soc. Rev., 2012, 41, 3594–3623 RSC;
(f) G. Li and R. Jin, Acc. Chem. Res., 2013, 46, 1749–1758 CrossRef CAS PubMed;
(g) T. Dainese, S. Antonello, J. A. Gascón, F. Pan, N. V. Perera, M. Ruzzi, A. Venzo, A. Zoleo, K. Rissanen and F. Maran, ACS Nano, 2014, 8, 3904–3912 CrossRef CAS PubMed;
(h) S. Knoppe and T. Bürgi, Acc. Chem. Res., 2014, 47, 1318–1326 CrossRef CAS PubMed;
(i) A. Mathew and T. Pradeep, Part. Part. Syst. Charact., 2014, 31, 1017–1053 CrossRef CAS;
(j) S. Yamazoe, K. Koyasu and T. Tsukuda, Acc. Chem. Res., 2014, 47, 816–824 CrossRef CAS PubMed;
(k) Y. Wang, H. Su, C. Xu, G. Li, L. Gell, S. Lin, Z. Tang, H. Häkkinen and N. Zheng, J. Am. Chem. Soc., 2015, 137, 4324–4327 CrossRef CAS PubMed;
(l) N. Xia, J. Yang and Z. Wu, Nanoscale, 2015, 7, 10013–10020 RSC;
(m) M.-B. Li, S.-K. Tian, Z. Wu and R. Jin, Chem. Mater., 2016, 28, 1022–1025 CrossRef CAS;
(n) M. A. Tofanelli, K. Salorinne, T. W. Ni, S. Malola, B. Newell, B. Phillips, H. Hakkinen and C. J. Ackerson, Chem. Sci., 2016, 7, 1882–1890 RSC;
(o) N. Yan, L. Liao, J. Yuan, Y.-j. Lin, L.-h. Weng, J. Yang and Z. Wu, Chem. Mater., 2016, 28, 8240–8247 CrossRef CAS;
(p) X.-K. Wan, J.-Q. Wang, Z.-A. Nan and Q.-M. Wang, Sci. Adv., 2017, 3, e1701823 CrossRef PubMed.
-
(a) P. D. Jadzinsky, G. Calero, C. J. Ackerson, D. A. Bushnell and R. D. Kornberg, Science, 2007, 318, 430–433 CrossRef CAS PubMed;
(b) M. W. Heaven, A. Dass, P. S. White, K. M. Holt and R. W. Murray, J. Am. Chem. Soc., 2008, 130, 3754–3755 CrossRef CAS PubMed;
(c) M. Zhu, C. M. Aikens, F. J. Hollander, G. C. Schatz and R. Jin, J. Am. Chem. Soc., 2008, 130, 5883–5885 CrossRef CAS PubMed;
(d) G. Li, Z. Lei and Q.-M. Wang, J. Am. Chem. Soc., 2010, 132, 17678–17679 CrossRef CAS PubMed;
(e) C. Zeng, H. Qian, T. Li, G. Li, N. L. Rosi, B. Yoon, R. N. Barnett, R. L. Whetten, U. Landman and R. Jin, Angew. Chem., Int. Ed., 2012, 51, 13114–13118 CrossRef CAS PubMed;
(f) A. Desireddy, B. E. Conn, J. Guo, B. Yoon, R. N. Barnett, B. M. Monahan, K. Kirschbaum, W. P. Griffith, R. L. Whetten, U. Landman and T. P. Bigioni, Nature, 2013, 501, 399–402 CrossRef CAS PubMed;
(g) D. Crasto, S. Malola, G. Brosofsky, A. Dass and H. Hakkinen, J. Am. Chem. Soc., 2014, 136, 5000–5005 CrossRef CAS PubMed;
(h) X.-K. Wan, S.-F. Yuan, Z.-W. Lin and Q.-M. Wang, Angew. Chem., Int. Ed., 2014, 53, 2923–2926 CrossRef CAS PubMed;
(i) C. P. Joshi, M. S. Bootharaju, M. J. Alhilaly and O. M. Bakr, J. Am. Chem. Soc., 2015, 137, 11578–11581 CrossRef CAS PubMed;
(j) S. B. Tian, Y. Z. Li, M. B. Li, J. Y. Yuan, J. L. Yang, Z. K. Wu and R. C. Jin, Nat. Commun., 2015, 6, 8667 CrossRef CAS PubMed;
(k) X.-K. Wan, Q. Tang, S.-F. Yuan, D.-e. Jiang and Q.-M. Wang, J. Am. Chem. Soc., 2015, 137, 652–655 CrossRef CAS PubMed;
(l) C. Zeng, Y. Chen, K. Kirschbaum, K. Appavoo, M. Y. Sfeir and R. Jin, Sci. Adv., 2015, 1, e1500045 Search PubMed;
(m) M. J. Alhilaly, M. S. Bootharaju, C. P. Joshi, T. M. Besong, A.-H. Emwas, R. Juarez-Mosqueda, S. Kaappa, S. Malola, K. Adil, A. Shkurenko, H. Häkkinen, M. Eddaoudi and O. M. Bakr, J. Am. Chem. Soc., 2016, 138, 14727–14732 CrossRef CAS PubMed;
(n) L. Liao, S. Zhuang, C. Yao, N. Yan, J. Chen, C. Wang, N. Xia, X. Liu, M. B. Li, L. Li, X. Bao and Z. Wu, J. Am. Chem. Soc., 2016, 138, 10425–10428 CrossRef CAS PubMed;
(o) H. Yang, Y. Wang, X. Chen, X. Zhao, L. Gu, H. Huang, J. Yan, C. Xu, G. Li, J. Wu, A. J. Edwards, B. Dittrich, Z. Tang, D. Wang, L. Lehtovaara, H. Häkkinen and N. Zheng, Nat. Commun., 2016, 7, 12809 CrossRef CAS PubMed;
(p) C. Zeng, Y. Chen, K. Iida, K. Nobusada, K. Kirschbaum, K. J. Lambright and R. Jin, J. Am. Chem. Soc., 2016, 138, 3950–3953 CrossRef CAS PubMed;
(q) J.-L. Zeng, Z.-J. Guan, Y. Du, Z.-A. Nan, Y.-M. Lin and Q.-M. Wang, J. Am. Chem. Soc., 2016, 138, 7848–7851 CrossRef CAS PubMed;
(r) T. Chen, S. Yang, J. Chai, Y. Song, J. Fan, B. Rao, H. Sheng, H. Yu and M. Zhu, Sci. Adv., 2017, 3, e1700956 CrossRef PubMed;
(s) H. Dong, L. Liao, S. Zhuang, C. Yao, J. Chen, S. Tian, M. Zhu, X. Liu, L. Li and Z. Wu, Nanoscale, 2017, 9, 3742–3746 RSC;
(t) Z. Gan, J. Chen, J. Wang, C. Wang, M.-B. Li, C. Yao, S. Zhuang, A. Xu, L. Li and Z. Wu, Nat. Commun., 2017, 8, 14739 CrossRef CAS PubMed;
(u) L. Liao, S. Zhuang, P. Wang, Y. Xu, N. Yan, H. Dong, C. Wang, Y. Zhao, N. Xia, J. Li, H. Deng, Y. Pei, S.-K. Tian and Z. Wu, Angew. Chem., Int. Ed., 2017, 56, 12644–12648 CrossRef CAS PubMed;
(v) S. Zhuang, L. Liao, M.-B. Li, C. Yao, Y. Zhao, H. Dong, J. Li, H. Deng, L. Li and Z. Wu, Nanoscale, 2017, 9, 14809–14813 RSC.
- Y. Pei, Y. Gao, N. Shao and X. C. Zeng, J. Am. Chem. Soc., 2009, 131, 13619–13621 CrossRef CAS PubMed.
-
(a) D.-e. Jiang, M. L. Tiago, W. Luo and S. Dai, J. Am. Chem. Soc., 2008, 130, 2777–2779 CrossRef CAS PubMed;
(b) S. Yamazoe, S. Takano, W. Kurashige, T. Yokoyama, K. Nitta, Y. Negishi and T. Tsukuda, Nat. Commun., 2016, 7, 10414 CrossRef CAS PubMed.
- Q. Yao, X. Yuan, V. Fung, Y. Yu, D. T. Leong, D. E. Jiang and J. Xie, Nat. Commun., 2017, 8, 927 CrossRef PubMed.
- M. M. Kreevoy and J. E. C. Hutchins, J. Am. Chem. Soc., 1972, 94, 6371–6376 CrossRef CAS.
- Z. Wu, M. A. MacDonald, J. Chen, P. Zhang and R. Jin, J. Am. Chem. Soc., 2011, 133, 9670–9673 CrossRef CAS PubMed.
- X. Yuan, B. Zhang, Z. Luo, Q. Yao, D. T. Leong, N. Yan and J. Xie, Angew. Chem., Int. Ed., 2014, 53, 4623–4627 CrossRef CAS PubMed.
- Z. Wu, J. Suhan and R. Jin, J. Mater. Chem., 2009, 19, 622–626 RSC.
-
(a) S. Tian, C. Yao, L. Liao, N. Xia and Z. Wu, Chem. Commun., 2015, 51, 11773–11776 RSC;
(b) C. Yao, J. Chen, M.-B. Li, L. Liu, J. Yang and Z. Wu, Nano Lett., 2015, 15, 1281–1287 CrossRef CAS PubMed;
(c) C. Yao, S. Tian, L. Liao, X. Liu, N. Xia, N. Yan, Z. Gan and Z. Wu, Nanoscale, 2015, 7, 16200–16203 RSC;
(d) L. Liao, C. Yao, C. Wang, S. Tian, J. Chen, M.-B. Li, N. Xia, N. Yan and Z. Wu, Anal. Chem., 2016, 88, 11297–11301 CrossRef CAS PubMed.
-
(a) Y. Negishi, K. Nobusada and T. Tsukuda, J. Am. Chem. Soc., 2005, 127, 5261–5270 CrossRef CAS PubMed;
(b) K. M. Harkness, Y. Tang, A. Dass, J. Pan, N. Kothalawala, V. J. Reddy, D. E. Cliffel, B. Demeler, F. Stellacci, O. M. Bakr and J. A. McLean, Nanoscale, 2012, 4, 4269–4274 RSC;
(c) Y. Yu, Z. Luo, D. M. Chevrier, D. T. Leong, P. Zhang, D.-e. Jiang and J. Xie, J. Am. Chem. Soc., 2014, 136, 1246–1249 CrossRef CAS PubMed;
(d) Y. Chen, C. Zeng, D. R. Kauffman and R. Jin, Nano Lett., 2015, 15, 3603–3609 CrossRef CAS PubMed;
(e) A. Dass, S. Theivendran, P. R. Nimmala, C. Kumara, V. R. Jupally, A. Fortunelli, L. Sementa, G. Barcaro, X. Zuo and B. C. Noll, J. Am. Chem. Soc., 2015, 137, 4610–4613 CrossRef CAS PubMed;
(f) X.-K. Wan, S.-F. Yuan, Q. Tang, D.-e. Jiang and Q.-M. Wang, Angew. Chem., Int. Ed., 2015, 54, 5977–5980 CrossRef CAS PubMed;
(g) M. S. Bootharaju, C. P. Joshi, M. R. Parida, O. F. Mohammed and O. M. Bakr, Angew. Chem., Int. Ed., 2016, 55, 922–926 CrossRef CAS PubMed;
(h) C. Zeng, Y. Chen, K. Kirschbaum, K. J. Lambright and R. Jin, Science, 2016, 354, 1580–1584 CrossRef CAS PubMed;
(i) M. A. Aljuhani, M. S. Bootharaju, L. Sinatra, J. M. Basset, O. F. Mohammed and O. M. Bakr, J. Phys. Chem. C, 2017, 121, 10681–10685 CrossRef CAS.
-
(a) H. Yang, Y. Wang, H. Huang, L. Gell, L. Lehtovaara, S. Malola, H. Häkkinen and N. Zheng, Nat. Commun., 2013, 4, 2422 Search PubMed;
(b) Y. Chen, C. Liu, Q. Tang, C. Zeng, T. Higaki, A. Das, D. E. Jiang, N. L. Rosi and R. Jin, J. Am. Chem. Soc., 2016, 138, 1482–1485 CrossRef CAS PubMed;
(c) W. Du, S. Jin, L. Xiong, M. Chen, J. Zhang, X. Zou, Y. Pei, S. Wang and M. Zhu, J. Am. Chem. Soc., 2017, 139, 1618–1624 CrossRef CAS PubMed.
-
(a) Y. Pei, Y. Gao and X. C. Zeng, J. Am. Chem. Soc., 2008, 130, 7830–7832 CrossRef CAS PubMed;
(b) Z. K. Wu and R. C. Jin, Chem.–Eur. J., 2011, 17, 13966–13970 CrossRef CAS PubMed.
-
(a) W. Guo, J. Yuan and E. Wang, Chem. Commun., 2009, 3395–3397, 10.1039/B821518A;
(b) Y. Liu, K. Ai, X. Cheng, L. Huo and L. Lu, Adv. Funct. Mater., 2010, 20, 951–956 CrossRef CAS;
(c) S. W. Yang and T. Vosch, Anal. Chem., 2011, 83, 6935–6939 CrossRef CAS PubMed;
(d) M. Wang, Z. Wu, J. Yang, G. Wang, H. Wang and W. Cai, Nanoscale, 2012, 4, 4087–4090 RSC;
(e) X. Yuan, Z. Luo, Y. Yu, Q. Yao and J. Xie, Chem.–Asian J., 2013, 8, 858–871 CrossRef CAS PubMed;
(f) L. Zhang and E. Wang, Nano Today, 2014, 9, 132–157 CrossRef CAS.
-
(a) C.-A. J. Lin, T.-Y. Yang, C.-H. Lee, S. H. Huang, R. A. Sperling, M. Zanella, J. K. Li, J.-L. Shen, H.-H. Wang, H.-I. Yeh, W. J. Parak and W. H. Chang, ACS Nano, 2009, 3, 395–401 CrossRef CAS PubMed;
(b) M. A. H. Muhammed, P. K. Verma, S. K. Pal, R. C. A. Kumar, S. Paul, R. V. Omkumar and T. Pradeep, Chem.–Eur. J., 2009, 15, 10110–10120 CrossRef CAS PubMed;
(c) L. Shang, S. Dong and G. U. Nienhaus, Nano Today, 2011, 6, 401–418 CrossRef CAS;
(d) L. Zhang, J. Zhu, Z. Zhou, S. Guo, J. Li, S. Dong and E. Wang, Chem. Sci., 2013, 4, 4004–4010 RSC;
(e) Z. Luo, K. Zheng and J. Xie, Chem. Commun., 2014, 50, 5143–5155 RSC;
(f) X. Zhang, F.-G. Wu, P. Liu, N. Gu and Z. Chen, Small, 2014, 10, 5170–5177 CAS;
(g) Z. Gan, Y. Lin, L. Luo, G. Han, W. Liu, Z. Liu, C. Yao, L. Weng, L. Liao, J. Chen, X. Liu, Y. Luo, C. Wang, S. Wei and Z. Wu, Angew. Chem., Int. Ed., 2016, 55, 11567–11571 CrossRef CAS PubMed;
(h) J. Yang, N. Xia, X. Wang, X. Liu, A. Xu, Z. Wu and Z. Luo, Nanoscale, 2016, 8, 13078 RSC.
- D. Lee, R. L. Donkers, G. Wang, A. S. Harper and R. W. Murray, J. Am. Chem. Soc., 2004, 126, 6193–6199 CrossRef CAS PubMed.
- Z. Wu and R. Jin, Nano Lett., 2010, 10, 2568–2573 CrossRef CAS PubMed.
- Z. Luo, X. Yuan, Y. Yu, Q. Zhang, D. T. Leong, J. Y. Lee and J. Xie, J. Am. Chem. Soc., 2012, 134, 16662–16670 CrossRef CAS PubMed.
- K. Pyo, V. D. Thanthirige, K. Kwak, P. Pandurangan, G. Ramakrishna and D. Lee, J. Am. Chem. Soc., 2015, 137, 8244–8250 CrossRef CAS PubMed.
- K. L. Weerawardene and C. M. Aikens, J. Am. Chem. Soc., 2016, 138, 11202–11210 CrossRef CAS PubMed.
Footnotes |
† Electronic supplementary information (ESI) available: CCDC 1587211. For ESI and crystallographic data in CIF or other electronic format see DOI: 10.1039/c7sc05019d |
‡ S. Zhuang, Dr L. Liao, Y. Zhao contributed equally to this work. |
|
This journal is © The Royal Society of Chemistry 2018 |
Click here to see how this site uses Cookies. View our privacy policy here.