DOI:
10.1039/C8SC02769B
(Edge Article)
Chem. Sci., 2018,
9, 7193-7197
A mild light-induced cleavage of the S–O bond of aryl sulfonate esters enables efficient sulfonylation of vinylarenes†
Received
24th June 2018
, Accepted 23rd July 2018
First published on 25th July 2018
Abstract
A new mode of S–O bond activation has been discovered, which constitutes novel reactivity of easily available and bench-stable arylsulfonate phenol esters. This protocol enables access to putative sulfonyl radical intermediates, which enable straightforward access to valuable vinyl sulfones.
Aryl sulfonate phenol esters are versatile synthetic intermediates in organic chemistry. A facile C–O bond cleavage under transition metal (TM)-catalyzed conditions makes them valuable alternatives to aryl halides in cross-coupling reactions (Scheme 1a).1 Meanwhile, the robustness of arylsulfonate groups under a variety of reaction conditions renders them useful protecting groups of phenols (Scheme 2b).2 In both scenarios, different forms of organosulfur byproducts are discarded. The ability to utilize these intermediates as sulfonylating reagents, on another hand, would offer an attractive alternative strategy toward incorporation of organosulfur motifs into organic molecules.3 Herein we report mild, light-induced TM-free activation of the S–O bond4 of aryl sulfonate phenol esters leading to the formation of putative sulfonyl radicals.5,9 Thus the generated reactive sulfone species add across the double bond of vinyl arenes and -heteroarenes to furnish valuable vinyl sulfones (Scheme 1c).
 |
| Scheme 1 Diverse reactivity of arylsulfonate phenol esters. | |
 |
| Scheme 2 Proposed reaction mechanism. | |
Within the framework of our ongoing studies on the development of light-induced synthetic methodologies,6 we unexpectedly discovered a sulfonylation of styrene 1a with arylsulfonate phenol ester 2a into vinyl sulfone 3a (Table 1). Vinyl sulfones have received considerable attention in recent years, as these motifs are featured in medicinally relevant structures,7 and also serve as useful reactive intermediates.8 Most of the established protocols toward synthesis of vinyl sulfones rely on employment of TM-catalysts and/or utilizing excess amounts of oxidants.9 In contrast, the observed sulfonylation reaction takes advantage of mild light-induced activation of the S–O bond, thus potentially providing an alternative route toward vinyl sulfones. Intrigued by the uncovered novel reactivity of arylsulfonate phenol esters and inspired by the importance of vinyl sulfones,8,9 we performed an optimization of this interesting transformation.10 It was found that the tosylate of commercially available meta-CF3 phenol is the best source of tosyl groups. The reaction proceeds best by 427 nm visible light irradiation of 0.1 M DMA solution in the presence of 3 equiv. of Cs2CO3 (entry 1). Introducing other electron-rich or -deficient substituents across the phenolic aromatic ring led to diminished yields (entries 2–5). The derivative of aliphatic alcohol showed no reactivity, thus indicating the necessity of an aromatic ester for the successful transformation (entry 6). Employment of DMSO instead of DMA was equally efficient (entry 7). Switching to other solvents commonly used in radical chemistry was not productive (entries 8–10). Lowering the amount of base played a detrimental role as well (entry 11), and addition of radical scavengers such as TEMPO or galvinoxyl completely suppressed this sulfonylation reaction (entries 12–13). Finally, the test experiment indicated that this reaction does not proceed in the absence of light (entry 14).
Table 1 Optimization of reaction parametersa
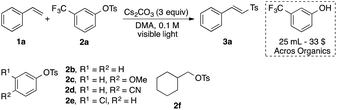
|
Entry |
Deviation from standard conditions |
Yieldb, % |
Reaction conditions: 1a (0.1 mmol), 2a (0.3 mmol), Cs2CO3 (0.3 mmol), DMA (0.1 M), and a 40 W 427 nm LED.
GC/MS yield.
|
1 |
None |
90 |
2 |
2b instead of 2a |
40 |
3 |
2c instead of 2a |
16 |
4 |
2d instead of 2a |
78 |
5 |
2e instead of 2a |
10 |
6 |
2f instead of 2a |
0 |
7 |
DMSO instead of DMA |
89 |
8 |
PhH instead of DMA |
0 |
9 |
PhCF3 instead of DMA |
0 |
10 |
MeCN instead of DMA |
15 |
11 |
Cs2CO3 (2 equiv.) instead of Cs2CO3 (3 equiv.) |
64 |
12 |
Galvinoxyl (2 equiv.) |
0 |
13 |
TEMPO (2 equiv.) |
0 |
14 |
No light, 60 to 100 °C |
0 |
The study on the generality of the transformation of this sulfonylation reaction (Table 2) showed that styrenes possessing electron-releasing (1b) and -withdrawing (1c, d) substituents at the para position all reacted well to give the corresponding vinyl sulfones in good yields. Pleasingly, Bpin moiety-containing 1e was compatible with the reaction conditions, delivering vinyl sulfone 3e in 62% yield. Substitution at the ortho- (3f) and meta- (3g) positions posed no problem as well. Various vinyl heteroarenes were found to be capable partners in this sulfonylation reaction. Thus fused N-, O- and S-containing vinylarenes provided the corresponding products 3h–3k efficiently. This protocol also works with vinyl pyridines, producing 3l–3n in excellent yields. The scale-up experiment proceeded smoothly to deliver vinyl sulfone 3l in 81% yield. Notably, mild reaction conditions provided N-sulfonyl vinyl imidazole (3o) in good yield. Importantly, this reaction can also be carried out with disubstituted styrenes to produce various trisubstituted sulfones 3p–3r in reasonable to good yields. Moreover, this reaction can be accomplished in a more complex setting to give access to a vinyl sulfone derivative of estrone (3s).
Table 2 Scope of vinyl arenesa

|
Reaction conditions: 1 (0.1 mmol), 2 (0.3 mmol), Cs2CO3 (0.3 mmol), DMA (0.1 M), and a 40 W 427 nm LED.
450 nm LED.
|
|
Next, the scope of the sulfone moiety was examined (Table 3). Gratifyingly, diverse substitution patterns of arylsulfonate phenol esters posed no problem for the developed protocol providing excellent yields of the respective vinyl sulfones 3t–3x. Importantly, this protocol may successfully be applied toward sulfone 3y featuring a 3,5-bis(trifluoromethyl)phenylsulfone fragment, a very useful synthon, for transition metal-catalyzed coupling reactions,11 as well as for the modified Julia olefination reaction.12 Heteroaryl sulfonates also participated well leading to products 3aa and 3ab in 95 and 69% yields, respectively.
Table 3 Scope of aryl sulfonate estersa

|
Reaction conditions: 1 (0.1 mmol), 2 (0.3 mmol), Cs2CO3 (0.3 mmol), DMA (0.1 M), and a 40 W 427 nm LED.
|
|
The proposed mechanism of this sulfonylation reaction is depicted in Scheme 2. The base-assisted13 formation of electron donor–acceptor (EDA) complex14A between arylsulfonate phenol ester 2 and a molecule of DMA occurs first.15 Upon excitation, this complex (A*) undergoes a single electron transfer (SET)16 leading to radical anion B, which fragments into sulfonyl radical D and phenoxide E. Addition of sulfonyl radical D to styrene5d produces benzyl radical F. In one scenario, the latter experiences intermolecular hydrogen atom transfer (HAT) with sulfonyl radical D, formed in parallel by cleavage of the S–O bond (B → D + E)17 or, alternatively, with the radical C, formed during the first SET step (A* → B), to produce the reaction product, vinyl sulfone 3a. Alternatively, electron catalysis may be operative.18 In that case, deprotonation of F produces anion-radical G, which after intermolecular SET with 2 produces 3a and radical-anion B, closing the catalytic cycle.19
Further transformations of selected vinyl sulfones obtained highlight their synthetic usefulness (Scheme 3). Thus, conjugate Michael addition of pyrrolidine and benzenemethanethiol to vinyl sulfone 3z delivered adducts 4 and 5 in virtually quantitative yields. Upon subjecting to free radical conditions, 3z underwent substitution of the phenylsulfone group with the super silyl moiety giving rise to valuable vinyl silane 6
20 in 76% yield. Hydrogenation of 3y proceeded uneventfully producing alkylsulfone 7 in good yield. Versatility of the obtained vinyl sulfones may be further exemplified by transformations toward 8–11, which have been featured in reported protocols.21–24
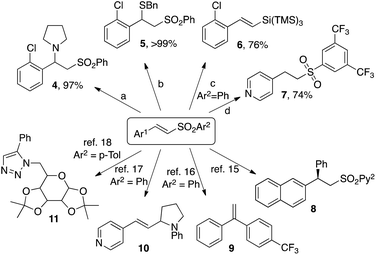 |
| Scheme 3 Transformations of obtained vinyl sulfones. Conditions: (a) 3z (0.1 mmol), pyrrolidine (2.4 mmol), 70 °C. (b) 3z (0.1 mmol), BnSH (0.4 mmol), Et3N (0.15 mmol), MeOH (0.25 M), rt (c) 3z (0.1 mmol), (TMS)3SiH (0.3 mmol), AIBN (0.25 mmol), benzene (0.05 M), reflux. (d) 3y (0.1 mmol), Pd/C (0.005 mmol), hydrogen gas (balloon). | |
Conclusions
In summary, we have uncovered a novel reactivity of arylsulfonate phenol esters, featuring mild visible light-induced cleavage of the S–O bond, which gives rise to putative sulfonyl radicals. The latter are capable of addition across various aromatic and heteroaromatic alkenes providing valuable vinyl sulfones. It is believed that this novel reactivity of arylsulfonate esters will find application in synthesis.
Conflicts of interest
The authors declare no conflict of interest.
Acknowledgements
This work was supported by the National Institutes of Health (GM120281) and the National Science Foundation (CHE-1663779).
Notes and references
-
(a)
A. Littke, in Modern Arylation Methods, Ed. L. Ackermann, Wiley-VCH, Weinheim, 2009, p. 25 Search PubMed;
(b) H. Zeng, Z. Qiu, A. Dominguez-Huerta, Z. Haerne, Z. Chen and C.-J. Li, ACS Catal., 2017, 7, 510 CrossRef;
(c) D. Kalyani, Pure Appl. Chem., 2014, 86, 315 Search PubMed;
(d) B.-J. Li, D.-G. Yu, C.-L. Sun and Z.-J. Shi, Chem.–Eur. J., 2011, 17, 1728 CrossRef PubMed.
-
P. G. M. Wuts, T. W. Greene, in Greene's Protective Groups in Organic Synthesis, Wiley-Interscience, 4th edn, 2007, p. 421 Search PubMed.
-
(a) A. Wimmer and B. Konig, Beilstein J. Org. Chem., 2018, 14, 54 CrossRef PubMed;
(b) C. Shen, P. Zhang, Q. Sun, S. Bai, T. S. A. Hor and X. Liu, Chem. Soc. Rev., 2015, 44, 291 RSC;
(c) K. L. Dunbar, D. H. Scharf, A. Litomska and C. Hertweck, Chem. Rev., 2017, 117, 5521 CrossRef PubMed;
(d) P. Chauhan, S. Mahajan and D. Enders, Chem. Rev., 2014, 114, 8807 CrossRef PubMed;
(e) T. Kondo and T. Mitsudo, Chem. Rev., 2000, 100, 3205 CrossRef PubMed;
(f) I. Beletskaya and V. P. Ananikov, Chem. Rev., 2011, 111, 1596 CrossRef PubMed;
(g) C. C. Eichman and J. P. Stambuli, Molecules, 2011, 16, 590 CrossRef PubMed.
- For examples of S–O bond cleavage leading to reactive organosulfur species, see:
(a) G. L'Haermine and D. J. Pasto, J. Org. Chem., 1990, 55, 5815 CrossRef;
(b) F. Cottard and D. J. Pasto, J. Org. Chem., 1994, 59, 4642 CrossRef;
(c) T. Kawamura, P. J. Crusic and J. K. Kochi, Tetrahedron Lett., 1972, 40, 4075 CrossRef;
(d) I. W. Still and M. T. Thomas, Tetrahedron Lett., 1970, 48, 4225 CrossRef;
(e) D. D. Gregory and W. S. Jenks, J. Org. Chem., 1998, 63, 3859 CrossRef;
(f) A. P. Darmanyan, D. D. Gregory, Y. Guo and W. S. Jenks, J. Phys. Chem. A, 1997, 101, 6855 CrossRef;
(g)
Y. Guo, W. S. Jenks, J. Org. Chem. 1995, 60, 5480.
-
(a) D. Zheng, J. Yu and J. Wu, Angew. Chem. Int. Ed., 2016, 55, 11925 (
Angew. Chem.
, 2016
, 128
, 12104
) CrossRef PubMed;
(b)
M. P. Bertrand, C. Ferreri, in Radicals in Organic Synthesis, Ed. P. Renaud, M. Sibi, Wiley-VCH, Weinheim, 2001, vol. 2, p. 485 Search PubMed;
(c) X.-Q. Pan, J.-P. Zou, W.-B. Yi and W. Zhang, Tetrahedron, 2015, 71, 7481 CrossRef;
(d) O. Mozziconacci, M. Tamba, K. Bobrowski, G. Kciuk, M. P. Bertrand, S. Gastaldi, V. I. Timokhin and C. Chatgilialoglu, J. Phys. Chem. A, 2012, 116, 7623 CrossRef PubMed;
(e) K. Gilmore, B. Gold, R. J. Clark and I. V. Alabugin, Aust. J. Chem., 2013, 66, 336 CrossRef.
-
(a) M. Ratushnyy, M. Parasram, Y. Wang and V. Gevorgyan, Angew. Chem. Int. Ed., 2018, 57, 2712 (
Angew. Chem.
, 2018
, 130
, 2742
) CrossRef PubMed;
(b) P. Chuentragool, M. Parasram, Y. Shi and V. Gevorgyan, J. Am. Chem. Soc., 2018, 140, 2465 CrossRef PubMed;
(c) D. Kurandina, M. Rivas, M. Radzhabov and V. Gevorgyan, Org. Lett., 2018, 20, 357 CrossRef PubMed;
(d) M. Parasram, P. Chuentragool, Y. Wang, Y. Shi and V. Gevorgyan, J. Am. Chem. Soc., 2017, 139, 14857 CrossRef PubMed;
(e) D. Kurandina, M. Parasram and V. Gevorgyan, Angew. Chem. Int. Ed., 2017, 56, 14212 (
Angew. Chem.
, 2017
, 129
, 14400
) CrossRef PubMed;
(f) M. Parasram, P. Chuentragool, D. Sarkar and V. Gevorgyan, J. Am. Chem. Soc., 2016, 138, 6340 CrossRef PubMed.
-
(a) S. Y. Woo, J. H. Kim, M. K. Moon, S.-H. Han, S. K. Yeon, J. W. Choi, B. K. Jang, H. J. Song, Y. G. Kang, J. W. Kim, J. Lee, D. J. Kim, O. Hwang and K. D. Park, J. Med. Chem., 2014, 57, 1473 CrossRef PubMed;
(b) B. A. Frankel, M. Bentley, R. G. Kryger and D. G. McCafferty, J. Am. Chem. Soc., 2004, 126, 3404 CrossRef PubMed;
(c) S. Liu, B. Zhou, H. Yang, Y. He, Z.-X. Jiang, S. Kumar, L. Wu and Z.-Y. Zhang, J. Am. Chem. Soc., 2008, 130, 8251 CrossRef PubMed;
(d) L. Ni, X. S. Zheng, P. K. Somers, L. K. Hoong, R. R. Hill, E. M. Marino, K.-L. Suen, U. Saxena and C. Q. Meng, Bioorg. Med. Chem. Lett., 2003, 13, 745 CrossRef PubMed;
(e) D. C. Meadows and J. Gervay-Hague, Med. Res. Rev., 2006, 26, 793 CrossRef PubMed;
(f) J. Morales-Sanfrutos, J. Lopez-Jamarillo, M. Ortega-Munoz, A. Megia-Fernandez, F. Perez-Balderas, F. Hernandez-Mateo and F. Santoyo-Gonzalez, Org. Biomol. Chem., 2010, 8, 667 RSC;
(g) A. F. Kisselev, W. A. van der Linden and H. S. Overkleeft, Chem. Biol., 2012, 19, 99 CrossRef PubMed.
-
(a) N. S. Simpkins, Tetrahedron, 1990, 46, 6951 CrossRef;
(b) D. C. Meadows and J. Gervay-Hague, Med. Res. Rev., 2006, 26, 793 CrossRef PubMed;
(c) A. L. Krasovskiy, E. S. Balenkova and V. G. Nenajdenko, Tetrahedron, 2007, 63, 12481 CrossRef;
(d) M. G. Braun, B. Quiclet-Sire and S. Z. Zard, J. Am. Chem. Soc., 2011, 133, 15954 CrossRef PubMed;
(e) Q. Shu and Y. Lu, Angew. Chem. Int. Ed., 2010, 49, 7753 (
Angew. Chem.
, 2010
, 122
, 7919
) CrossRef PubMed;
(f) J.-N. Desroisers and A. B. Charette, Angew. Chem. Int. Ed., 2007, 46, 5955 (
Angew. Chem.
, 2007
, 119
, 6059
) CrossRef PubMed;
(g) J.-N. Desrosiers, W. S. Bechara and A. B. Charette, Org. Lett., 2008, 10, 2315 CrossRef PubMed;
(h) X. Liu, X. Chen and J. T. Mohr, Org. Lett., 2015, 17, 3572 CrossRef PubMed;
(i) J. Fritz and D. J. Wardrop, Org. Lett., 2006, 8, 3659 CrossRef PubMed;
(j) F. Hof, A. Schutz, C. Fah, S. Meyer, D. Bur, J. Liu, D. E. Goldberg and F. Diederich, Angew. Chem. Int. Ed., 2006, 45, 2138 (
Angew. Chem.
, 2006
, 118
, 2193
) CrossRef PubMed;
(k) M. N. Noshi, A. El-awa, E. Torres and P. L. Fuchs, J. Am. Chem. Soc., 2007, 129, 11242 CrossRef PubMed;
(l) B. Fisher, R. J. Lepage, J. M. White, Y. Ye, E. H. Krenske and M. A. Rizzacasa, Org. Biomol. Chem., 2017, 15, 5529 RSC;
(m) E. Rodrigo, I. Alonso, J. L. Garcia Ruano and M. B. Cid, J. Org. Chem., 2016, 81, 10887 CrossRef PubMed;
(n) Z. Li, R. Yazaki and T. Ohshima, Org. Lett., 2016, 18, 3350 CrossRef PubMed;
(o) U. Sankar, C. V. S. Kumar, V. Subramanian, K. K. Balasubramanian and S. Mahalakshimi, J. Org. Chem., 2016, 81, 2340 CrossRef PubMed.
-
(a) Y. Fang, Z. Luo and X. Xu, RSC Adv., 2016, 6, 59661 RSC;
(b) N.-W. Liu, S. Liang and G. Manolikakes, Synthesis, 2016, 1939 Search PubMed;
(c) A. Garcia-Dominguez, S. Muller and C. Nevado, Angew. Chem. Int. Ed., 2017, 56, 9949 (
Angew. Chem.
, 2017
, 129
, 10081
) CrossRef PubMed;
(d) Y. Xi, B. Dong, E. J. McClain, Q. Wang, T. L. Gregg, N. G. Akhmedov, J. L. Peterson and X. Shi, Angew. Chem. Int. Ed., 2014, 53, 4657 (
Angew. Chem.
, 2014
, 126
, 4745
) CrossRef PubMed;
(e) H. Wang, Q. Lu, C.-W. Chiang, Y. Luo, J. Zhou, G. Wang and A. Lei, Angew. Chem. Int. Ed., 2017, 56, 595 (
Angew. Chem.
, 2017
, 129
, 610
) CrossRef PubMed . For examples of photoredox synthesis of vinyl sulfones, see ;
(f) A. U. Meyer, S. Jager, D. P. Hari and B. Konig, Adv. Synth. Catal., 2015, 357, 2050 CrossRef;
(g) S. Cai, Y. Xu, D. Chen, L. Li, Q. Chen, M. Huang and W. Weng, Org. Lett., 2016, 18, 2990 CrossRef PubMed.
- See the ESI† for full optimization details.
-
(a) J. C.-H. Yim, M. Nambo and C. M. Crudden, Org. Lett., 2017, 19, 3715 CrossRef PubMed;
(b) M. Nambo, E. C. Keske, J. P. G. Rygus, J. C.-H. Yim and C. M. Crudden, ACS Catal., 2017, 7, 1108 CrossRef.
-
(a) D. A. Alonso, M. Varea and C. Najera, Tetrahedron Lett., 2004, 45, 573 CrossRef;
(b) D. A. Alonso, M. Fuensanta, E. Gomez-Bengoa and C. Najera, Adv. Synth. Catal., 2008, 350, 1823 CrossRef;
(c) D. A. Alonso, M. Fuensanta, E. Gomez-Bengoa and C. Najera, Eur. J. Org. Chem., 2008, 2915 CrossRef;
(d) D. A. Alonso, M. Fuensanta, C. Najera and M. Varea, J. Org. Chem., 2005, 70, 6404 CrossRef PubMed.
- R. P. Woodbury and M. W. Rathke, J. Org. Chem., 1977, 42, 1688 CrossRef.
-
(a) B. Liu, C.-H. Lim and G. M. Miyake, J. Am. Chem. Soc., 2017, 139, 13616 CrossRef PubMed;
(b) E. Arceo, I. D. Jurberg, A. Alvarez-Fernandez and P. Melchiorre, Nat. Chem., 2013, 5, 750 CrossRef PubMed.
- The UV-vis analysis of the reaction components indicated that the DMA solution of arylsulfonate phenol ester 2 and Cs2CO3 is capable of light absorption. For details, see the ESI.†.
- For examples of enolates as single electron donors, see:
(a) K. J. Emery, T. Tuttle and J. A. Murphy, Org. Biomol. Chem., 2017, 15, 8810 RSC;
(b) F. Cumine, S. Zhou, T. Tuttle and J. A. Murphy, Org. Biomol. Chem., 2017, 15, 3324 RSC;
(c) E. Doni, S. Zhou and J. A. Murphy, Molecules, 2015, 20, 1755 CrossRef PubMed and references therein.
- Monitoring the reaction by GC/MS showed that production of 1 equivalent of vinyl sulfone 3a consumes 2 equivalents of arylsulfonate phenol ester 2a.
- A. Studer and D. P. Curran, Nat. Chem., 2014, 6, 765 CrossRef PubMed.
- This pathway, however, does not account for the necessity of employment of 3 equivalents of arylsulfonate ester 2. See the ESI† for details..
-
(a) J. M. Kanabus-Kaminska, J. A. Hawari, D. Griller and C. Chatgilialoglu, J. Am. Chem. Soc., 1987, 109, 5268 CrossRef;
(b) D. Griller, M. Lesage and C. Chatgilialoglu, J. Org. Chem., 1988, 53, 3642 CrossRef;
(c) A. Alberti and C. Chatgilialoglu, Tetrahedron, 1990, 46, 3963 CrossRef;
(d) C. Chatgilialoglu, Acc. Chem. Res., 1992, 25, 188 CrossRef;
(e) C. Chatgilialoglu, Chem.–Eur. J., 2008, 14, 2310 CrossRef PubMed.
-
(a) P. Mauleon, I. Alonso, M. Rodriguez Rivero and J. C. Carretero, J. Org. Chem., 2007, 72, 9924 CrossRef PubMed;
(b) T. Nishimura, Y. Takiguchi and T. Hayashi, J. Am. Chem. Soc., 2012, 134, 9086 CrossRef PubMed.
- K. Yoshida and T. Hayashi, J. Am. Chem. Soc., 2003, 125, 2872 CrossRef PubMed.
-
(a) A. Noble and D. W. C. MacMillan, J. Am. Chem. Soc., 2014, 136, 11602 CrossRef PubMed;
(b) D. R. Heitz, K. Rizwan and G. A. Molander, J. Org. Chem., 2016, 81, 7308 CrossRef PubMed.
- D. Sahu, S. Dey, T. Pathak and B. Ganguly, Org. Lett., 2014, 16, 2100 CrossRef PubMed.
Footnote |
† Electronic supplementary information (ESI) available. See DOI: 10.1039/c8sc02769b |
|
This journal is © The Royal Society of Chemistry 2018 |
Click here to see how this site uses Cookies. View our privacy policy here.