DOI:
10.1039/C8SC02802H
(Edge Article)
Chem. Sci., 2018,
9, 7556-7561
Annulative π-extension of indoles and pyrroles with diiodobiaryls by Pd catalysis: rapid synthesis of nitrogen-containing polycyclic aromatic compounds†
Received
26th June 2018
, Accepted 2nd August 2018
First published on 9th August 2018
Abstract
A palladium-catalyzed one-step annulative π-extension (APEX) reaction of indoles and pyrroles that allows rapid access to nitrogen-containing polycyclic aromatic compounds is described. In the presence of palladium pivalate and silver carbonate, diverse indoles or pyrroles coupled with diiodobiaryls in a double direct C–H arylation manner to be transformed into the corresponding π-extended compounds in a single step. The newly developed catalytic system enables the use of various pyrroles and indoles as templates with a series of diiodobiaryls to provide structurally complicated and largely π-extended nitrogen-containing polycyclic aromatic compounds that are otherwise difficult to synthesize.
Introduction
With desirable electronic properties and diverse biological activities, nitrogen-containing fused aromatics have long been recognized as privileged structures in the fields of organic materials and pharmaceutical science.1 As these properties can be readily tuned via skeletal modification of the core N-heteroarene structure, significant efforts have been devoted to develop new synthetic approaches for π-extended nitrogen-containing polycyclic aromatic compounds (N-PACs). Representative classical approaches include (i) intramolecular carbon–nitrogen bond formation of biaryl amines,2 (ii) intramolecular carbon–carbon bond formation of diaryl amines,3 and (iii) stepwise functionalization and π-extension of indoles and pyrroles.4 However, these methods require the use of prefunctionalized heteroaromatics such as halogenated pyrroles, anilines and indoles, and stepwise transformations from unfuctionalized (hetero)aromatics. To achieve maximum efficiency in N-PAC construction, a more direct and ‘intuitive’ method for π-extension of unfunctionalized pyrroles and indoles is called for.
Recently, we have introduced several new one-step methods for the annulative π-extension (APEX) of unfuctionalized (hetero)aromatics (Fig. 1a).5–7 Because such APEX reactions directly transform easily available unfunctionalized (hetero)arenes to polycyclic aromatic hydrocarbons, nanographenes and π-extended heteroaromatics in a double direct C–H arylation manner, these protocols offer large benefits in the context of cost, simplicity, and step/atom economy.8
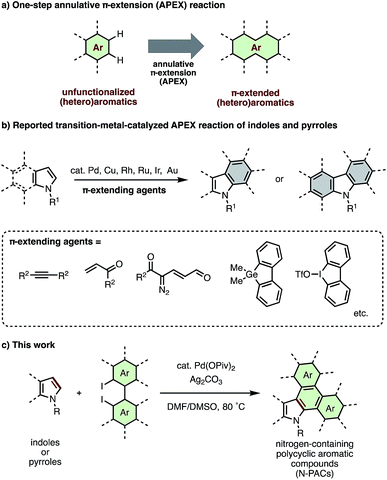 |
| Fig. 1 (a) General scheme of annulative π-extension (APEX) reaction of unfunctionalized (hetero)aromatics. (b) Previous transition-metal-catalyzed APEX reactions of indoles and pyrroles. (c) Palladium-catalyzed APEX reaction of indoles and pyrroles with diiodobiaryls (this work). | |
Recently, we7 and others9–14 have reported transition-metal-catalyzed APEX reactions of indoles and pyrroles using various π-extension units such as alkyne,9 alkene,7a,10 1-vinylpropargyl alcohols,11 α-diazocarbonyl compounds,12 α-bromochalcone,13 α-bromocinnamate,13 cyclic diaryliodonium salts,14 dibenzogermoles7b and diiodobiphenyls7c (Fig. 1b). However, these APEX reactions are limited in terms of lack of variety in π-extending agents, narrow substrate scope, and low functional group tolerance. Herein, we report a new catalytic APEX reaction that allows efficient pyrrole-to-indole, pyrrole-to-carbazole and indole-to-carbazole π-extensions. Our newly established catalytic system featuring palladium pivalate and silver carbonate in a mixed DMF/DMSO solvent system enabled the rapid synthesis of structurally complicated N-PACs from readily available unfunctionalized pyrroles/indoles and diiodobiaryls.
Results and discussion
We began our study by optimizing the reaction conditions for indole-to-carbazole extension of N-methylindole (1a) using 2,2′-diido-1,1′-biphenyl (2a) as a π-extending agent (Table 1). After extensive screening, we discovered that 1a (1.0 equiv.) coupled with 2a (1.5 equiv.) in the presence of Pd(OAc)2 (5 mol%) and Ag2CO3 (3.0 equiv.) at 80 °C in 7
:
3 mixture of dimethylformamide (DMF) and dimethylsulfoxide (DMSO) to provide N-methyldibenzo[a,c]carbazole (3aa) in 54% yield (entry 1). Use of palladium pivalate [Pd(OPiv)2] instead of Pd(OAc)2 improved the yield to 61% (entry 2), but other palladium sources such as PdCl2, PdI2, Pd(PPh3)4, Pd(OCOCF3)2 and Pd(CH3CN)4(BF4)2 failed to give more than trace amounts of product (entries 3–7). Decreasing the amount of Ag2CO3 to 1.5 equiv. (relative to 1a) further increased the yield of 3aa to 78% (entry 8). The use of silver carboxylate salts (AgOAc, AgOPiv, or AgOCOCF3) instead of Ag2CO3 resulted in much lower yield (entries 9–11). The silver cation itself was essential for this reaction; the use of Na2CO3, K2CO3 or Cs2CO3 instead of Ag2CO3 failed to give any product (see ESI† for details). The use of the DMF/DMSO mixed solvent system was important for obtaining maximum conversion; highly polar single solvents such as N,N-dimethylacetamide (DMAc), DMF, DMSO, CH3CN provided 3aa in diminished yield (29–10%, see ESI† for details), while less polar solvents such as 1,2-dichloroethane, 2,2,2-trifluoroethanol, 1,4-dioxane and toluene completely suppressed the reaction. Although higher reaction temperature accelerated the consumption of the starting material, the yield of 3aa was decreased (entries 12 and 13). Finally, we confirmed that the APEX reaction did not proceed in the absence of Pd catalyst or Ag2CO3 (entries 14 and 15). Although the use of additional ligands for Pd and the use of dibromobiphenyl in place of diiodobiphenyl as the π-extension reagent were also investigated, these modifications proved ineffective (see ESI† for details). Ultimately the conditions in entry 8 were deemed optimal for the present indole-to-carbazole APEX reaction.
Table 1 Screening of reaction conditions for the Pd-catalyzed indole-to-carbazole APEX reaction of N-methylindole (1a) and diiodobiphenyl (2a)
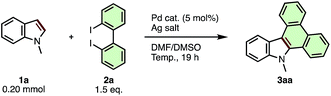
|
Entry |
Pd cat. |
Ag salt |
Temp. (°C) |
Yielda (%) |
Yield was determined by 1H NMR analysis using dodecane as an internal standard.
Reaction time was 1 h.
Isolated yield in the parenthesis. Piv = pivaloyl.
|
1 |
Pd(OAc)2 |
Ag2CO3 (3.0 eq.) |
80 |
54 |
2 |
Pd(OPiv)2 |
Ag2CO3 (3.0 eq.) |
80 |
61 |
3 |
PdCl2 |
Ag2CO3 (3.0 eq.) |
80 |
3 |
4 |
PdI2 |
Ag2CO3 (3.0 eq.) |
80 |
2 |
5 |
Pd(PPh3)4 |
Ag2CO3 (3.0 eq.) |
80 |
4 |
6 |
Pd(OCOCF3)2 |
Ag2CO3 (3.0 eq.) |
80 |
0 |
7 |
Pd(CH3CN)4(BF4)2 |
Ag2CO3 (3.0 eq.) |
80 |
0 |
8 |
Pd(OPiv)2 |
Ag2CO3 (1.5 eq.) |
80 |
78 (66)c |
9 |
Pd(OPiv)2 |
AgOAc (3.0 eq.) |
80 |
33 |
10 |
Pd(OPiv)2 |
AgOPiv (3.0 eq.) |
80 |
0 |
11 |
Pd(OPiv)2 |
AgOCOCF3 (3.0 eq.) |
80 |
0 |
12 |
Pd(OPiv)2 |
Ag2CO3 (1.5 eq.) |
100 |
57 |
13b |
Pd(OPiv)2 |
Ag2CO3 (1.5 eq.) |
100 |
58 |
14 |
None |
Ag2CO3 (1.5 eq.) |
80 |
0 |
15 |
Pd(OPiv)2 |
None |
80 |
0 |
A possible reaction mechanism of current indole-to-carbazole APEX reaction is shown in Scheme 1. Oxidative addition of 2a to palladium species (Pd(0) or Pd(II)) occurs to form biphenylylpalladium intermediate A.15 Then, the removal of iodide by silver salt may activate Pd complex A16 to form electron-deficient aryl-Pd species,17 which then react with indole at the C2 position to afford intermediate B. Through the control experiments on the C–H arylations of 1,2-dimethylindole and 1,3-dimethylindole with iodobenzene, the present APEX reaction seems to occur through the C2-arylation of indole rather than C3-arylation in the first step (see ESI† for details). Final step would be well-established Pd-catalyzed intramolecular C–H/C–I coupling to afford the cyclized compound 3aa.18
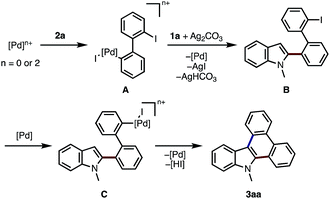 |
| Scheme 1 Proposed reaction mechanism for the Pd-catalyzed APEX reaction of N-methylindole (1a) with 2,2′-diiodo-1,1′-biphenyl (2a). | |
Under the optimized conditions, various types of π-extended carbazoles/indoles 3, 5 were prepared from the corresponding indole/pyrrole derivatives 1, 4 and diiodobiaryls 2. Scheme 2 illustrates the scope of applicable indole and pyrrole derivatives (1a–1m). N-Alkyl (2a, 2b), N-benzyl (2c), N-phenyl (2d) indoles and cross-linked lilolidine (2e) were converted smoothly to dibenzocarbazoles 3ba–3da in good to moderate yield, however the reaction of N-acetyl indole 2f did not provide the expected π-extension product 3fa. The presence of substituents at the 5-, 6-, or 7-positions of the indole ring was well-tolerated, giving various nitro- (3ga), cyano- (3ha, 3la), bromo- (3ia), methoxy- (3ja, 3ka), and benzyloxy-substituted (3ma) dibenzocarbazoles in moderate yields (40–62%). These results suggest that substituents on the benzene ring of indole do not critically affect the reaction progress. Interestingly, we found that the current APEX reaction between N-substituted pyrroles and 2a was mono-selective for the formation of dibenzoindoles 5aa and 5ba in 39% and 43% yields; only trace amounts of the di-APEX tetrabenzocarbazole products, the main products of our previous report,7c,19 were observed. As synthetic methods to prepare the dibenzo[e,g]indole skeleton remain limited and inefficient,20 the current APEX protocol provides a valuable, streamlined entry to this compound class.
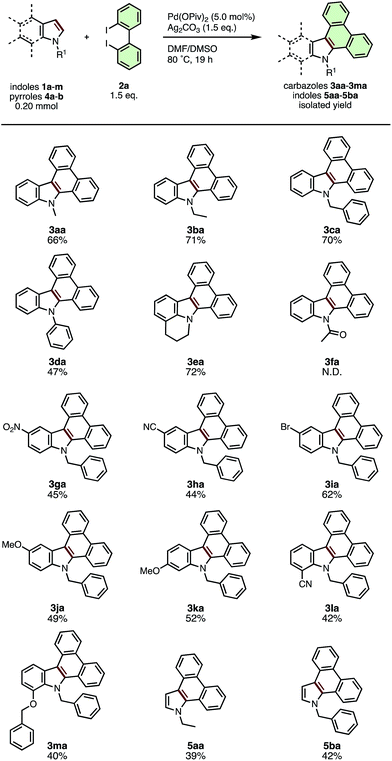 |
| Scheme 2 Substrate scope of indoles and pyrroles in the APEX reaction with 2,2′-diiodo-1,1′-biphenyl (2a). | |
The scope of diiodobiaryls in the current APEX reaction is shown in Scheme 3. The reaction of N-methylindole (2a) with unsymmetrical 4-chloro-2,2′-diiodo-1,1′-biphenyl (2b) gave a 1
:
1.4 regioisomeric mixture of 3ab and 3ab′ in 94% combined yield. APEX reactions of 1a and 4a with 4,4′-dibromo-2,2′-diiodo-1,1′-biphenyl (2c) smoothly occurred to give dibromodibenzocarbazole 3ac and dibromodibenozoindole 5cc in 81% and 44% yield, respectively. To our delight, the reaction of 1a with 2,2′-diiodo-1,1′-binaphthalene (2d) gave dinaphthocarbazole 3ad containing a [5]helicene moiety in 25% yield, whose helical structure was confirmed by X-ray crystallographic analysis. As this example clearly demonstrates, the late-stage attachment of complex, extended polyaromatic units is one of the most remarkable characteristics in the present APEX reaction.
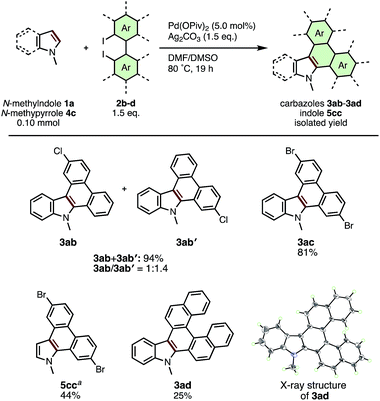 |
| Scheme 3 Substrate scope of diiodobiaryls. a0.20 mmol scale. | |
To demonstrate the power of the current APEX reaction to build complex, unsymmetrical N-PACs from simple starting materials, we employed a two-step sequence to synthesize tetrabenzocarbazole 6, a compound difficult to prepare via known methods (Scheme 4). First, APEX reaction of N-methylpyrrole (4c) with 2a was carried out to give the corresponding N-methyldibenzoindole (5ca) in 37% yield. Notably, this reaction did not give double-APEX product which is the major product in the previously developed APEX reaction of N-phenylpyrrole.8b Then, 5ca was further reacted with 4,4′-dibromo-2,2′-diiodo-1,1′-biphenyl (2c) by using Pd(CH3CN)4(BF4)2/AgOPiv/TfOH catalytic system8b to give the desired product 6 in 33% yield.21 Rapid access to a new class of unsymmetrically substituted tetrabenzocarbazole is notable, and should contribute to the exploration of new compounds for organic electronics application.
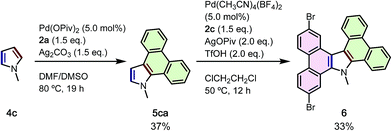 |
| Scheme 4 Sequential APEX reactions of N-methylpyrrole (4c) for the synthesis of unsymmetrically substituted tetrabenzocarbazole 6. | |
The current APEX reaction also provided a facile route to polycyclic aromatic compounds containing both nitrogen and sulfur (N–S-PACs) (Scheme 5). N-Methylindole (1a) coupled with 3,3′-diiodo-2,2′-bibenzothiophene (7) to give di(benzothieno)carbazole 8 in 32%. To our delight, the reaction of N-methylpyrrole (4c) with diiodo-2,2′-bibenzothiophene 7 afforded a double APEX product, tetra(benzothieno)carbazole 9, in 15% yield. While the yields were low, the generation of these novel N–S-PAC structures, which are highly interesting from the viewpoint of optoelectronic properties yet otherwise difficult to synthesize by conventional organic reactions, is notable.
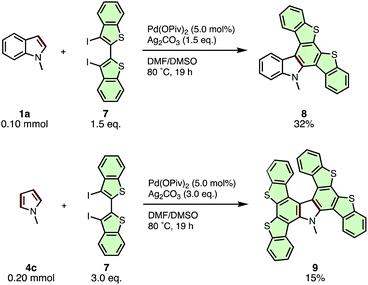 |
| Scheme 5 APEX reactions of N-methylindole (1a) and N-methylpyrrole (4c) with 3,3′-diiodo-2,2′-bibenzothiophene (7) for the synthesis of N–S-PACs. | |
The structural and electronic properties of 8 and 9 were elucidated via X-ray crystallography, UV-vis/photoluminescence spectroscopy, and DFT/TD-DFT calculations at the B3LYP/6-31G(d) level of theory (Fig. 2). Single crystal X-ray structures (Fig. 2a, b, S2 and S3†) reveal that compound 8 adopts a relatively flattened structure in the solid state (Fig. 2a), while compound 9 possesses a twisted structure owing to the embedded heterohelicene moiety. DFT calculations for 8 (Fig. 2c) reveal delocalization of the HOMO (−5.23 eV) over the entire molecule, while the LUMO (−1.49 eV) localizes on a benzothienocarbazole wing. On the other hand, the HOMO and LUMO of 9 are delocalized over entire molecule, and thus the energy level of LUMO (−1.72 eV) is slightly lower than that of 8. The UV-vis absorption spectra of 8 and 9 in CH2Cl2 show that both compounds have broad absorption bands between 300 and 450 nm (Fig. 2e). Absorption maxima were found at 294, 317, 339, 357, 381 and 399 nm in 8, and the corresponding peaks were also found in 9 at 305, 332, 348, 393 and 412 nm. The TD-DFT calculations revealed that the longest-wavenumber absorptions in 8 and 9 (399 and 412 nm) are attributed to the allowed HOMO–LUMO transitions (see ESI† for details). The fluorescence spectra of 8 and 9 in CH2Cl2 display broad emissions with emission maxima of 427 and 437 nm, respectively (Fig. 2e).
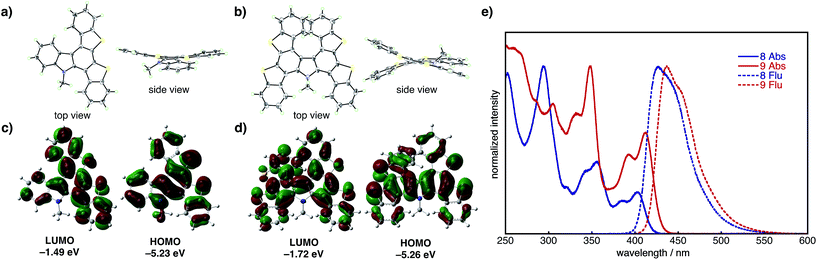 |
| Fig. 2 Top and side views of the X-ray crystal structures of (a) 8 and (b) 9. Thermal ellipsoids are drawn at 50% probability. Pictorial Frontier molecular orbitals and energy levels of (c) 8 and (d) 9 calculated using the B3LYP/6-31G(d) level of theory. (e) Normalized UV-vis absorption and fluorescence spectra of 8 and 9 in CH2Cl2 at rt. | |
Conclusions
In summary, we have developed a novel palladium-catalyzed APEX reaction to enable the annulative π-extension of indoles/pyrroles with diiodobiaryls. Use of the Pd(OPiv)2/Ag2CO3 catalytic system in a mixed DMF/DMSO solvent allows the preparation of a diverse range of N-PACs in a single step, including several previously unsynthesized structures. Rapid access to exotic scaffolds such as complex, unsymmetrically substituted tetrabenzocarbazoles and extended N-heteroarenes featuring multiple helicene moieties is a particular highlight of the present APEX protocol. Developed APEX methodology also has great potential for the efficient and rapid synthesis of planar and non-planar π-extended N-PACs such as π-extended azacorannulenes, aza-buckybowls and pyrrolopyrroles which are regarded as one of promising materials for optoelectronics.22 Further investigations into the reaction mechanism and applications of this APEX method towards the synthesis of larger π-extended heteroaromatics are currently underway.
Conflicts of interest
There are no conflicts to declare.
Acknowledgements
This work was supported by the ERATO program from JST (JPMJER1302 to K. I.), the JSPS KAKENHI Grant Numbers (JP26810057, JP16H00907, JP17K19155 and JP18H02019 to H. I.), the SUMITOMO Foundation (141495 to H. I.) and the DAIKO Foundation (H. I.). We also thank Dr Yasutomo Segawa and Mr Kenta Kato for assistance of X-ray crystallographic analysis and Dr David R. Levine for proofreading. The computations were performed using the Research Center for Computational Science, Okazaki, Japan. ITbM is supported by the World Premier International Research Center Initiative (WPI), Japan.
Notes and references
-
(a) H.-J. Knölker and K. R. Reddy, Chem. Rev., 2002, 102, 4303–4427 CrossRef;
(b) M. Somei and F. Yamada, Nat. Prod. Rep., 2003, 20, 216–242 RSC;
(c) S. Deslandes, S. Chassaing and E. Delfourne, Mar. Drugs, 2009, 7, 754–786 CrossRef PubMed;
(d) A. W. Schmidt, K. R. Reddy and H.-J. Knölker, Chem. Rev., 2012, 112, 3193–3328 CrossRef PubMed;
(e) N. Blouin and M. Leclerc, Acc. Chem. Res., 2008, 41, 1110–1119 CrossRef PubMed;
(f) P. M. Beaujuge and J. R. Reynolds, Chem. Rev., 2010, 110, 268–320 CrossRef PubMed;
(g) C. Wang, H. Dong, W. Hu, Y. Liu and D. Zhu, Chem. Rev., 2012, 112, 2208–2267 CrossRef PubMed;
(h) M. Bashir, A. Bano, A. S. Ijaz and B. A. Chaudhary, Molecules, 2015, 20, 13496–13517 CrossRef PubMed;
(i) S. M. Thomas, A. Purmal, M. Pollastri and K. Mensa-Wilmot, Sci. Rep., 2016, 6, 32083 CrossRef PubMed;
(j) H. Li, F. Zhe-Ling, W. Yi-Tao and L. Li-Gen, Chin. J. Nat. Med., 2017, 15, 881–888 CrossRef PubMed;
(k) K. Karon and M. Lapkowski, J. Solid State Electrochem., 2015, 19, 2601–2610 CrossRef.
-
(a) Z. Chen, B. Wang, J. Zhang, W. Yu, Z. Liu and Y. Zhang, Org. Chem. Front., 2015, 2, 1107–1295 RSC;
(b) G. Song, F. Wang and X. Li, Chem. Soc. Rev., 2012, 41, 3651–3678 RSC;
(c) J. Yuan, C. Liu and A. Lei, Chem. Commun., 2015, 51, 1394–1409 RSC;
(d) M. Zhang, Y. Zhang, X. Jie, H. Zhao, G. Li and W. Su, Org. Chem. Front., 2014, 1, 843–895 RSC;
(e) J. Bariwal and E. Van der Eycken, Chem. Soc. Rev., 2013, 42, 9283–9303 RSC;
(f) M.-L. Louillat and F. W. Patureau, Chem. Soc. Rev., 2014, 43, 901–910 RSC;
(g) V. S. Thirunavukkarasu, S. I. Kozhushkov and L. Ackermann, Chem. Commun., 2014, 50, 29–39 RSC;
(h) P. Thansandote and M. Lautens, Chem.–Eur. J., 2009, 15, 5874–5883 CrossRef PubMed.
-
(a) J. Yamaguchi, A. D. Yamaguchi and K. Itami, Angew. Chem., Int. Ed., 2012, 51, 8960–9009 CrossRef PubMed;
(b) C. S. Yeung and V. M. Dong, Chem. Rev., 2011, 111, 1215–1292 CrossRef PubMed;
(c) G. P. McGlacken and L. M. Bateman, Chem. Soc. Rev., 2009, 38, 2447–2464 RSC;
(d) L. Ackermann, R. Vicente and A. R. Kapdi, Angew. Chem., Int. Ed., 2009, 48, 9792–9826 CrossRef PubMed;
(e) Y. Segawa, T. Maekawa and K. Itami, Angew. Chem., Int. Ed., 2015, 54, 66–81 CrossRef PubMed.
-
(a)
T. Eicher, S. Hauptmann and A. Speicher, Five-Membered Heterocycles, in The Chemistry of Heterocycles: Structure, Reactions, Syntheses, and Applications, Wiley-VCH Verlag GmbH & Co. KGaA, Weinheim, 2nd edn, 2003, ch. 5.1–5.21, pp. 52–121 CrossRef;
(b) S.-M. T. Toguem, I. Knepper, P. Ehlers, T. T. Dang, T. Patonay and P. Langer, Adv. Synth. Catal., 2012, 354, 1819–1826 CrossRef.
- For a review on APEX reactions, see: H. Ito, K. Ozaki and K. Itami, Angew. Chem., Int. Ed., 2017, 56, 11144–11164 CrossRef PubMed.
-
(a) K. Ozaki, K. Kawasumi, M. Shibata, H. Ito and K. Itami, Nat. Commun., 2015, 6, 6251 CrossRef PubMed;
(b) K. Ozaki, K. Murai, W. Matsuoka, K. Kawasumi, H. Ito and K. Itami, Angew. Chem., Int. Ed., 2017, 56, 1361–1364 CrossRef PubMed;
(c) Y. Yano, H. Ito, Y. Segawa and K. Itami, Synlett, 2016, 27, 2081–2084 CrossRef;
(d) K. Kato, Y. Segawa and K. Itami, Can. J. Chem., 2017, 95, 329–333 CrossRef.
-
(a) K. Ozaki, H. Zhang, H. Ito, A. Lei and K. Itami, Chem. Sci., 2013, 4, 3416–3420 RSC;
(b) K. Ozaki, W. Matsuoka, H. Ito and K. Itami, Org. Lett., 2017, 19, 1930–1933 CrossRef PubMed;
(c) W. Matsuoka, H. Ito and K. Itami, Angew. Chem., Int. Ed., 2017, 56, 12224–12228 CrossRef PubMed.
-
(a) B. M. Trost, Science, 1991, 254, 1471–1477 CrossRef PubMed;
(b) B. M. Trost, Angew. Chem., Int. Ed., 1995, 34, 259–281 CrossRef.
-
(a) M. Yamashita, H. Horiguchi, K. Hirano, T. Satoh and M. Miura, J. Org. Chem., 2009, 74, 7481–7488 CrossRef PubMed;
(b) Y. Matsuda, S. Naoe, S. Oishi, N. Fujii and H. Ohno, Chem.–Eur. J., 2015, 21, 1463–1467 CrossRef PubMed.
-
(a) T. Guo, Q. Jiang, F. Huang, J. Chen and Z. Yu, Org. Chem. Front., 2014, 1, 707–711 RSC;
(b) A. K. Verma, A. K. Danodia, R. K. Saunthwal, M. Patel and D. Choudhary, Org. Lett., 2015, 17, 3658–3661 CrossRef PubMed;
(c) J. K. Laha and N. Dayal, Org. Lett., 2015, 17, 4742–4745 CrossRef PubMed;
(d) K. Lin, Y. Jian, P. Zhao, C.-S. Zhao, W.-D. Pan and S. Liu, Org. Chem. Front., 2018, 5, 590–594 RSC;
(e) R. K. Saunthwal, K. M. Saini, M. Patel and A. K. Verma, Tetrahedron, 2017, 73, 2415–2431 CrossRef.
- N. Thies, C. G. Hrib and E. Haak, Chem.–Eur. J., 2012, 18, 6302–6308 CrossRef PubMed.
-
(a) S. G. Dawande, V. Kanchupalli, J. Kalepu, H. Chennamsetti, B. S. Lad and S. Katukojvala, Angew. Chem., Int. Ed., 2014, 53, 4076–4080 CrossRef PubMed;
(b) K. S. Rathore, M. Harode and S. Katukojvala, Org. Biomol. Chem., 2014, 12, 8641–8645 RSC;
(c) J.-Q. Wu, Z. Yang, S.-S. Zhang, C.-Y. Jiang, Q. Li, Z.-S. Huang and H. Wang, ACS Catal., 2015, 5, 6453–6457 CrossRef.
- S. Paria and O. Reiser, Adv. Synth. Catal., 2014, 356, 557–562 CrossRef.
- Y. Wu, X. Peng, B. Luo, F. Wu, B. Liu, F. Song, P. Huang and S. Wen, Org. Biomol. Chem., 2014, 12, 9777–9780 RSC.
-
(a) C. Qin and W. Lu, J. Org. Chem., 2008, 73, 7424–7427 CrossRef PubMed;
(b) A. J. Hickman and M. S. Sanford, ACS Catal., 2011, 1, 170–174 CrossRef;
(c) K. D. Collins, R. Honeker, S. Vásquez-Céspedes, D.-T. D. Tang and F. Glorius, Chem. Sci., 2015, 6, 1816–1824 RSC;
(d) H. Kawai, Y. Kobayashi, S. Oi and Y. Inoue, Chem. Commun., 2008, 1464–1466 RSC;
(e) K. Funaki, H. Kawai, T. Sato and S. Oi, Chem. Lett., 2011, 40, 1050–1052 CrossRef.
-
(a) N. Lebrasseur and I. Larrosa, J. Am. Chem. Soc., 2008, 130, 2926–2927 CrossRef PubMed;
(b) D. J. Liston, Y. J. Lee, W. R. Scheidt and C. A. Reed, J. Am. Chem. Soc., 1989, 111, 6643–6648 CrossRef;
(c) V. G. Albano, M. Di Serio, M. Monari, I. Orabona, A. Panunzi and F. Ruffo, Inorg. Chem., 2002, 41, 2672–2677 CrossRef PubMed.
-
(a) M. Shibata, H. Ito and K. Itami, J. Am. Chem. Soc., 2018, 140, 2196–2205 CrossRef PubMed;
(b) A. Nova, G. Ujaque, F. Maseras, A. Lledós and P. Espinet, J. Am. Chem. Soc., 2006, 128, 14571–14578 CrossRef PubMed . Also see ref. 6a and 16.
- For the Pd-catalyzed intramolecular C–H/C–I coupling of related compounds, see:
(a) C.-W. Li, C.-I. Wang, H.-Y. Liao, R. Chaudhuri and R.-S. Liu, J. Org. Chem., 2007, 72, 9203–9207 CrossRef PubMed;
(b) R. Chaudhuri, M.-Y. Hsu, C.-W. Li, C.-I. Wang, C.-J. Chen, C. K. Lai, L.-Y. Chen, S.-H. Liu, C.-C. Wu and R.-S. Liu, Org. Lett., 2008, 10, 3053–3056 CrossRef PubMed;
(c) T.-A. Chen and R.-S. Liu, Org. Lett., 2011, 13, 4644–4647 CrossRef PubMed.
- Changing the reaction temperature or the amount of diiodobiphenyl did not change this result (see ESI† for details).
-
(a) G. B. Jones and J. E. Mathews, Tetrahedron, 1997, 53, 14599–14614 CrossRef;
(b)
S. Y. Hyun, S. O. Jung and H. J. Oh, KR Pat. 20150121626A, 2015.
- In this reaction, the previously developed APEX conditions using Pd(CH3CN)4(BF4)2/AgOPiv/TfOH gave the best result in terms of yield of 6. On the other hand, the present APEX conditions did not give 6.
-
(a) S. Ito, Y. Tokimaru and K. Nozaki, Angew. Chem., Int. Ed., 2015, 54, 7256–7260 CrossRef PubMed;
(b) H. Yokoi, Y. Hiraoka, D. Sakamaki, S. Seki and H. Shinokubo, Nat. Commun., 2015, 6, 8215 CrossRef PubMed;
(c) Y. Tokimaru, S. Ito and K. Nozaki, Angew. Chem., Int. Ed., 2018, 57, 9818–9822 CrossRef PubMed;
(d) M. Krzeszewski, T. Kodama, E. M. Espinoza, V. Vullev, T. Kubo and D. T. Gryko, Chem.–Eur. J., 2016, 22, 16478–16488 CrossRef PubMed;
(e) S. Mishra, M. Krzeszewski, C. A. Pignedoli, P. Ruffieux, R. Fasel and D. T. Gryko, Nat. Commun., 2018, 9, 1714 CrossRef PubMed;
(f) M. Krzeszewski, M. Gryko and D. T. Gryko, Acc. Chem. Res., 2017, 50, 2334–2345 CrossRef PubMed.
Footnote |
† Electronic supplementary information (ESI) available: Syntheses, NMR, UV-vis-nearIR absorption, CV and crystallographic table. CCDC 1848311 (3ad), 1848309 (8), 1848310 (9). For ESI and crystallographic data in CIF or other electronic format see DOI: 10.1039/c8sc02802h |
|
This journal is © The Royal Society of Chemistry 2018 |
Click here to see how this site uses Cookies. View our privacy policy here.