DOI:
10.1039/C8AY02173B
(Paper)
Anal. Methods, 2019,
11, 21-28
MWCNT based monolith for the analysis of antibiotics and pesticides in milk and honey by integrated nano-liquid chromatography-high resolution orbitrap mass spectrometry†
Received
4th October 2018
, Accepted 14th November 2018
First published on 15th November 2018
Abstract
A monolith based on MWCNTs incorporated into HPMACl was prepared for use as a stationary phase in nano-liquid chromatography. The performance of the monolith was tested at 800 nL min−1, in the separation of several compounds of environmental and pharmaceutical interest, namely antibiotics and pesticides. Good resolution was observed on the MWCNT incorporated column. Excellent separation performance was obtained in most cases. The high chromatographic separation capability and great stability observed in nano LC-UV encouraged the analyses to be carried out via HR Orbitrap MS. The antibiotics and pesticides were then injected into an integrated nano LC-HR Orbitrap MS system and they were identified by their retention time and accurate mass which were measured with product ions in full MS-all-ion fragmentation mode. High sensitivity (at the ppb level) and reproducibility were obtained. The applicability of the method with the monolith was demonstrated using milk and honey samples with the LODs ranging from 0.1 to 20 μg kg−1.
Introduction
Miniaturization in modern liquid chromatography has played an essential role in analytical separation science.1–3 Nano-LC (nano-liquid chromatography) is a miniaturized chromatography technique that is used as an analytical platform for advanced food analysis, and this technique offers unique advantages over conventional liquid chromatography such as reduced volumes of the mobile phase and an enhanced sensitivity as well as flow rate adjustment with a flow splitter.4 Nano-LC has also been used for the separation of a wide number of compounds with very low abundance in a matrix. These separations are performed with packed or monolithic capillary columns.5–7 The use of monolithic columns has risen significantly in micro/nano-scale analytical separations.8–10 Svec and Fréchet first introduced polymer-based monoliths, and since that time much effort has been made for the development of these materials.11 Over the past three decades, many monolithic columns have been prepared and applied in separation science.
In recent years, the use of nanoparticles (NPs) has been the newest approach to the preparation of monolithic columns. MWCNTs (multiwalled carbon nanotubes) are nanomaterials with sizes of 1–100 nm in more dimensions and show unique properties for many types of applications including medical and electronic.12 These NPs have a large surface area/volume ratio, and mechanical and biological qualities. Recently, the use of MWCNTs has also been adopted in the field of monolithic stationary phases, and MWCNT based monoliths have been prepared and used in reversed-phase chromatography (RPC).13–15 In the preparation of these kinds of monoliths, glycidyl methacrylate (GMA) has been used as the main monomer.16,17 The development of MWCNT based stationary phases with a new monomer, namely 3-chloro-2-hydroxypropyl methacrylate (HPMACl) to achieve multiple separation capabilities is essential for modern LC, especially with respect to nano-LC. A major drawback for the development of nano-LC based monolithic columns has been the limitations of the nano-flow instrument in terms of injection and detection tools. Considering the sensitivity of mass spectrometry, many nano-LC instruments have recently become commercially available. In this regard, the development of monolithic columns at the nano-scale (i.e. 10–100 μm i.d.) for sensitive separations with limited sample amounts (e.g., environmental, proteomics) seems to be an important strategy for selected bioanalytical applications.
The use of antibiotics (e.g. sulfonamides) is widely applied to treat bacterial infections of animals. Similarly, pesticides are widely used for crops at various stages of cultivation.18–20 Maximum residue levels (MRLs) of these compounds are in the range of ng kg−1 to few μg kg−1.5 Therefore, the development of more sensitive analytical approaches for the determination of these compounds at such low levels is of great interest. High resolution orbitrap mass spectrometry (HR Orbitrap MS) is one of the most powerful tools for targeted screening of complex matrices due to its high resolution, high sensitivity and full mass accuracy. Also, the reduction of flow rates in mass spectrometry, in particular in HR Orbitrap MS, offers an enhancement in sensitivity. Improvement in chromatographic separation is the main approach for quantitative LC-MS measurements. The use of nano-LC coupled to MS has been proven to be a better alternative to conventional LC due to faster separation, sharp peak shapes and reduction of organic solvent consumption. Nano-LC based applications with MS have been usually performed using particulate based columns.5,7,19,21–24 Nano-LC instrumentation with new column technology integrating nano-monoliths and nano-ESI spray will make accessible such a sophisticated technique to routine analysis. Up to now, the use of nano LC-MS with a monolith has been restricted to selected relevant applications.25 Considering the advantages of monoliths over their particulate counterparts, the adoption of monoliths to routine applications with nano LC-MS is needed. Therefore, in this work, as a new approach, the preparation of an HPMACl based monolithic stationary phase with incorporated MWCNTs is demonstrated. The monolith exhibited great separation capability for small compounds. In light of the higher UV chromatographic separation capability and great stability observed, parallel investigations were focused on searching for new nano-LC and nano LC-MS techniques, based on a MWCNT based monolith. Encouraged by these results, we used an integrated nano LC-HR Orbitrap MS system for the analyses of antibiotics and pesticides, employing a MWCNT based monolithic stationary phase. This monolith with a nanostructure exhibited great potential for achieving efficient analyses with a high resolution.
Experimental section
Instrumentation
Micrographs with a thin layer of gold (10 nm) were obtained by scanning electron microscopy, SEM (Zeiss, Evo-50, Germany). An X-ray diffractometer (Rigaku, Ultima-IV) was used for the characterization studies of dried monoliths. Measurements of the pore size and specific surface area of the monoliths were carried out using a Nova 2200 E (Malvern Instruments Ltd, Worcestershire, U.K.). An Ultimate 3000 Chromatography System with nano-flow (Dionex Technologies, Munich, Germany) was used for nano-LC experiments. The system includes four parts: (i) a flow manager with a flow splitter,(ii) an Ultimate 3000 pump, (iii) an Ultimate 3000 autosampler and (iv) an Ultimate 3000 RS variable wavelength detector. This system was readily coupled to an HR Orbitrap MS. Nano LC software was inserted into TraceFinder 3.2 (Thermo Scientific), which was used for the analyses of antibiotics and pesticides. Data were recorded using Xcalibur software version 2.1.0.1140 (Thermo Fisher Scientific). MS detection was performed using an Exactive Plus Orbitrap (Thermo Fisher Scientific) with a heated electrospray interface in both positive and negative modes scanning the ions in m/z 100–750. The Exactive Plus Orbitrap was calibrated with a calibration solution using an automatic syringe injector (Chemyx Inc. Fusion 100 T, USA). The calibration solution (Sigma-Aldrich) contained caffeine, Met-Arg-Phe-Ala, and Ultramark 1621. Nanospray parameters were as follows: capillary voltage, 28 V; capillary temperature, 270 °C; spray voltage, 3.2 kV; S-lens RF level, 60. The resolving power was set to 17
500. Extracted ion chromatograms were reconstructed based on ±3 ppm for identification purposes.
Materials and methods
Ethylene dimethacrylate (EDMA) (99%), 3-chloro-2-hydroxypropyl methacrylate (HPMACl) (99%), trifluoroacetic acid (TFA), 3-trimethoxysilylpropyl methacrylate (TMSPM, 98%), ACN, methanol, isopropanol and dodecanol were obtained from Sigma-Aldrich Chemical (Milwaukee, WI, USA). Multiwalled carbon nanotubes (MWCNTs) with 6–13 nm length (98% carbon basis) were purchased from Aldrich. 2,2′-Azobisisobutyronitrile (AIBN, Merck A.G.) was recrystallized from methanol before use. All antibiotics including sulfisoxazole (SXZ), sulfadiazine (SDZ), sulfapyridine (SPRY), sulfamethazine (SMZ), sulfathiazole (STZ), sulfanilamide (SNM), sulfacetamide (SCM) and sulfadoxine (SDX) were purchased from Sigma-Aldrich Chemical. Also, six pesticides including, chlorotoluron (CH), simazine (SM), metoxuron (MT), cyanazine (CY), atrazine (AT) and ropazine (PR) were also purchased from Sigma-Aldrich Chemical. Fused-silica capillaries (i.d.75 μm and o.d. 360 μm) were purchased from Polymicro Technologies (Phoenix, AZ, USA).
Preparation and incorporation of the MWCNTs based monolith
The polymerization procedure and incorporation of MWCNTs were similar to the procedure described by Aydoğan3 with a few modifications. The preparation of the monolithic column including the coating involved the following steps: a 75 μm i.d. capillary was washed successively with 0.2 M NaOH for 4 h and water for 20 min. Then, this capillary was modified by first filling with TMSPM (50%,v/v) in toluene, then plugging it with GC septa and finally the reaction was allowed to take place at 70 °C for 18 h. A standard polymerization mixture including HPMACl (16.4 wt%) and EDMA (22.4 wt%) was prepared in an appropriate porogenic solvent composed of isopropanol (30.6, wt%) and dodecanol (30.6, wt%). A specific amount of 0.1 wt% AIBN (with respect to the total volume) was added to the solution. Then, the solution thus obtained was introduced into the column and heated at 60 °C for 12 h. The column was washed with ACN and water, and dried with nitrogen. This monolith without nanotubes is referred to as the “blank monolith”. The same polymerization mixture with a specific amount of MWCNTs was vortexed for 10 min, sonicated for 30 min and then purged with nitrogen gas for 5 min at 25 °C to remove oxygen. The final polymeric solution was filled into a silanized 10 cm × 75 μm id capillary. Thereafter, both ends of the capillary were sealed and it was placed in a water bath for 12 h at 60 °C. The resulting monolithic column was carefully washed with a mixture of ACN and water (75/25%,v/v) for 2 h. In order to examine the effect of MWCNTs on the separation, these two columns were used for further studies described in this research article.
Chromatography conditions
The mobile phase was prepared by mixing water with an appropriate volume of ACN. The antibiotics and pesticides were dissolved in a mixture containing 70% ACN
:
30% water (v/v) and diluted to a suitable concentration. Isocratic elution was used for the separations. The mobile phase consisted of 75% ACN
:
15% MeOH
:
10% H2O with 3–5% (v/v) formic acid. This mobile phase was used for both nano LC-UV and nano LC-HROrbitrap MS. The injection volume was 1 μL and the flow rate was 800 nL min−1. Before the nano-LC experiment, the monolithic column was equilibrated for 10 min. The temperature of the monolithic column was kept at 20 °C. Milk samples were purchased from different local markets. The samples were filtered through a 0.10 μm membrane. A Captiva ND cartridge was used for the extraction.
Results and discussion
Preparation and characterization of MWCNT based monolithic columns
In our recent studies, we introduced different types of HPMACl based monoliths for use in nano-LC.3,4 Although various GMA based MWCNT stationary phases are available for HPLC and μLC, there has not been any report on the preparation of HPMACl based MWCNT monolithic stationary phases. The preparation of this monolith might play an important role in investigating nanotube effects on chromatographic separations. Therefore, a new monolith preparation was attempted. The monolith described in this study was optimized according to the procedure reported in our previous article.3 With the extensive experience gained in the design of HPMACl monoliths in our previous studies, the porogenic solvent system and MWCNT content were carefully evaluated. To incorporate MWCNTs into the HPMACl monolith, dispersion of nanotubes in the polymerization mixture was necessary. The solvent mixture of cyclohexanol and dodecanol was used in order to disperse MWCNTs.13 However, sufficient solubility could not be achieved using this porogenic solvent. In order to find the appropriate solvent mixture, several solvents such as 1-propanol/dodecanol, isopropanol/dodecanol, butanol/decanol and hexanol/dodecanol were tried to get an appropriate homogeneous solution. When the mixture of isopropanol/dodecanol was added to the polymerization mixture and MWCNTs, the homogeneous mixture remained dispersed for several weeks. Considering the best solubility for a long time, the porogenic solvent isopropanol/dodecanol was selected as the solvent system in order to achieve the suitable incorporation of MWCNTs.
The MWCNT content was optimized according to the research reported in recent articles.3,26 The combined total weight was kept constant in order to maintain the same ratio of the monomer (HPMACl) to the cross-linker (EDMA) that is essential to prepare a suitable MWCNT based monolith. The effect of nanotube content in the polymerization mixture on the extent of polymerization was examined. The back pressure and permeability of the columns with different nanotube contents (e.g., 3.7, 5.1, 6.1 wt%) were investigated. The highest degree of polymerization was achieved when 6.1 wt% was employed. With lower content of nanotubes in the polymerization mixture the permeability slightly decreased when compared to the blank monolith. The obtained results may indicate that the degree of macroporosity increased with increasing nanotube content (i.e., 3.7, 5.1, 6.1, wt%). On the other hand, further increase of nanotube content resulted in columns with high pressure, leading to blockage of the flow through macropores and/or destabilization of the monolith structure. The best MWCNT content was found to be 5.1 wt%. The structural morphology of the monoliths was observed by SEM. Comparative images for the columns can be seen in Fig. 1a1, a2, b1 and b2. As can be seen, both the phase ratio and surface area were increased as compared with the blank monolith (Fig. 1b1 with 2500× magnification and Fig. 1b2 with 10000× magnification). The morphology of a monolith tightly coated onto the column could be seen. It is worth noting that increasing the nanotube content in the polymerization mixture under the same conditions produced a dense polymeric layer surface with nanotubes. Based on visual evaluation, there is clearly a difference between the blank monolith (Fig. 1a1) and the nanotube incorporated monolith (Fig. 1b2). This also documents that larger MWCNTs form a thicker and denser layer on the pore surface. The observed dense nanotube layer is a proof that shows an enhancement in the surface area. The nanotube layer thus obtained also allowed the mobile phase to contact a large surface area of the monolith. The results also showed the variation in the morphology of the nanotube layer (Fig. 1a2 and b2). With a higher content of nanotubes in the polymerization mixture (e.g., 6.1 wt%), the permeability of the column was too low for rinsing. This showed that increasing the nanotube content may block the pores of the monolith. The blank HPMACl-EDMA monolith and its counterparts containing 3.7and 5.1 wt% MWCNTs were first prepared in tubes and used for surface area measurements. Nitrogen sorption studies revealed a surface area of 53 m2 g−1 for the blank monolith and 234 and 414 m2 g−1 for MWCNT counterparts, respectively. To compare the monolith incorporated with MWCNTs with the blank monolith, the monoliths were further studied by XRD (see Fig. 1a3 and b3). No increase in the peak intensity at 20–40° (2θ) was observed (see Fig. 1a3) for the blank monolith. However, there was an increase in the peak intensity at 20–40° (2θ) for the nanotube incorporated monolith. This result agreed with that reported in recent studies.27,28Fig. 1b3 shows that MWCNTs are well incorporated within the monolithic structure. The stability of the monolith with nanotubes was tested by measuring the back-pressure values under the same conditions daily. The monolith showed a linear relationship (R2 > 0.9996) between the flow rate and the resulting backpressure. The obtained result shows the good stability of the monolithic column. The incorporation of MWCNTs into the blank monolith afforded an increased efficiency of 48
000 plates per m. The relative standard deviations (RSDs) of the column were evaluated using the retention factor of benzene as a test compound using the mobile phase consisting of ACN/H2O 80/20 (v/v) in an isocratic mode. Run-to-run, day-to-day and column-to-column reproducibility values were less than 2.2%, 3.3%, and 3.6% (n = 5), respectively. The low RSD% values for the reproducibility indicated that this strategy is a reliable method for monolith fabrication. The obtained results also showed that the columns could be prepared and used with satisfactory reproducibility.
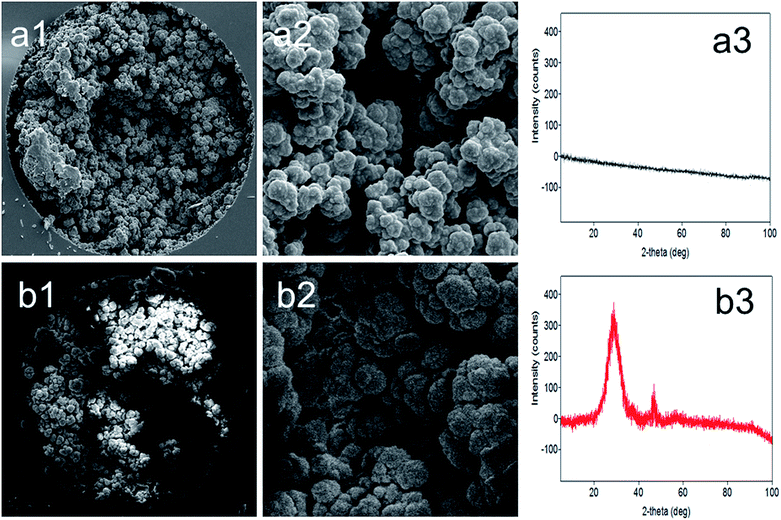 |
| Fig. 1 SEM microphotographs of the monolithic columns prepared with nanotubes (b1 with mag 2500× and b2 with mag 10000×) and without nanotubes (a1 and a2) and X-ray diffraction results prepared without nanotubes (a3) and with nanotubes (b3). | |
Adjusting mobile phase composition for the baseline separation of antibiotics and pesticides in nano LC-UV
Monolithic capillary columns operated in nano-LC have broad application potentials in sample analysis.23,25 Further adoption of such columns (i.e. monolithic columns) in sample analysis of different nature seems to be an essential strategy for nano-LC. In this regard, the MWCNT based monolith under investigation was examined for the separation of antibiotics and pesticides. First, the separation of eight antibiotics including SXZ, SDZ, SPRY, SMZ, STZ, SNM, SNC and SDX was attempted. A mobile phase containing 75% ACN
:
15% MeOH
:
10% H2O (v/v) was initially tested for the baseline separation of antibiotics. While the blank monolith was not useful for separations in the RPC mode, the MWCNT based monolith exhibited great separation ability towards these compounds. However, the resolution still needed an improvement, which was achieved by exploiting the differences in their pKa values. To achieve an improved separation, differences in their pKa values were exploited. In this regard, formic acid was added to the mobile phase at 5% (v/v), which was composed of 75% ACN
:
15% MeOH
:
10% H2O, and a flow rate of 800 nL min−1 was used for the separation. It was found that this mobile phase increased both the solubility and resolution of the compounds. Fig. 2 illustrates the separation of the compounds using the blank monolith (Fig. 2a) and the MWCNT incorporated monolith (Fig. 2b). As can be seen, the retention time and resolution of the antibiotics improved greatly. In contrast to the monolith without nanotubes, the nanotube counterpart yielded an increased efficiency amounting to 46
000 plates per m for the antibiotics. This confirms that the MWCNT based monolith provided high hydrophobicity originating from the incorporated nanotubes, thus enabling good separation and resolution of the antibiotic compounds. In other words, the improvement in separation represents a proof of the usefulness of the incorporation of nanotubes in enhancing both retention and selectivity.
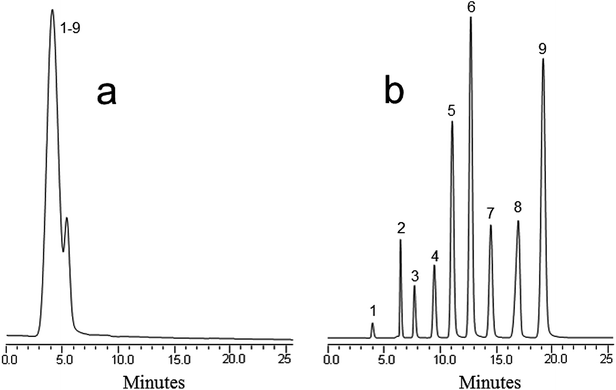 |
| Fig. 2 Chromatograms of antibiotics obtained by nano LC-UV on the monolithic capillary column (a) without MWCNTs and (b) with incorporated MWCNTs. Conditions: column dimensions, 10 cm × 75 μm i.d.; pump pressure, 6 MPa; concentration, 0.01 mg mL−1; detection wavelength, 270 nm; flow rate, 800 nL min−1; mobile phases: in (a), 75% ACN : 15% MeOH : 10% H2O; in (b)75% ACN : 15% MeOH : 10% H2O at 5% FA. Order of peaks (1), thiourea; (2), SCM; (3), SNM; (4), SDZ; (5), SPRY; (6), SDX; (7), STZ; (8), SMZ; (9), SXZ. | |
The use of pesticides is becoming widespread as a result of increasing agricultural productivity. There is a small tolerance policy according to the European Union (Regulation, 2008) (i.e. tolerance policy of up to 1 ppb in agricultural matrices). Despite the small amount, it may cause unexpected hazardous intoxication. Therefore, the development of a sensitive analysis for pesticides is essential. In this respect, the obtained monolith was further applied to the separation of six pesticides, including CH, SM, MT, CY, AT and PR. A mobile phase composition consisting of 50% ACN
:
50% H2O was used for the separation according to the literature.29 The optimal mobile phase conditions with respect to hydrophobic interactions were obtained using a slightly lower ACN content. The best separation was obtained at 60% ACN (v/v) in the mobile phase (Fig. 3a). However, the baseline separation still could not be achieved. When a formic acid solution at 3% (v/v) was added to the mobile phase, the six pesticides eluted between 6 and 14 min with baseline resolution at a flow rate of 800 nL min−1 (Fig. 3b). It is worth noting that the separation mechanism involved the hydrophobic interactions between nanotubes and the pesticide analyte under the optimum RPC conditions. The obtained results demonstrated the successful separation ability of the MWCNT based monolith.
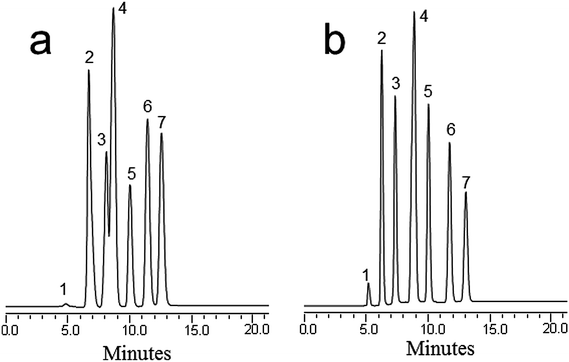 |
| Fig. 3 Chromatograms of pesticides obtained by nano LC-UV on the monolithic capillary column with incorporated MWCNTs. Conditions: column dimensions, 10 cm × 75 μm i.d.; pump pressure, 6 MPa; concentration; 0.01 mgmL−1, detection wavelength, 270 nm; flow rate, 800 nL min−1; mobile phases: in (a) 60% ACN : 40% H2O; in (b) 60% ACN : 40% H2O at 3% FA. Order of peaks: (1), thiourea; (2), MT; (3), SM; (4), CY; (5), CH; (6), AT; (7), PR. | |
Analysis of antibiotics and pesticides using the MWCNT based column with nano LC-HR oOrbitrap MS
Miniaturized separation techniques are a sound option to improve the sensitivity using MS with electrospray detection.30 The analyses of antibiotics and pesticides were rarely performed using the nano LC-MS technique with packed columns.5,31–33 Nano LC-MS with new column technology (e.g., MWCNT incorporated monoliths) integrating nano-monolith and nano-ESI spray could make accessible such a sophisticated technique to routine analysis. In this respect, considering the higher UV chromatographic separation capability, the results obtained encouraged the analyses of antibiotics and pesticides using nano LC-HR Orbitrap MS with the novel monolith. The UV results were also promising for the future development of the nano LC-HR Orbitrap MS method for the analyses of antibiotics and pesticides on the MWCNT based monolith. This can also become a crucial point in sensitive applications, with the use of the monolith in small volume analysis. Therefore, the MWCNT based monolith was applied to the analysis of antibiotics and pesticides by using nano LC-HR Orbitrap MS at concentration levels from 0.005 to 50 ppm. All analyses were performed in triplicate for each analyte. The samples were first injected into the monolith one by one in order to compare the retention time values between UV and HR Orbitrap MS. The retention time and theoretical values for the eight antibiotics and six pesticides are given (see ESI Tables S1 and S2†).
When MS was used, the peaks showed a distorted shape due to the low data rate (see Fig. 4b). This can be explained by the fact that the ionization efficiency may not reach 100% under the operational conditions, as it may get dispersed in charged nanodroplets. This may also show that the ESI ionization in the HR Orbitrap MS may cause a decrease in sensitivity for polar compounds (e.g. sulfamethazine). The results indicated that the retention times of all samples were almost constant under identical chromatographic conditions. Nevertheless, nano LC-HR Orbitrap MS with the MWCNT-based monolith showed high efficiency and sensitivity towards antibiotics and pesticides. Full-MS AIF scan mode allowed for the screening of the compounds. The nano LC-HR Orbitrap MS spectra of sulfapyridine are given in Fig. S1.† As can be seen here, high resolution was observed and the mass error was less than 2 ppm, which was acceptable for the screening. The total ion chromatograms in the case of pesticides are shown in Fig. S2.† A very high sensitivity was observed for the pesticides. By increasing the flow rate, it is possible to reduce the overall analysis time with only a small deterioration in the MWCNT based monolith efficiency. The sensitivity is superior to that reported in previously published papers with packed/monolithic columns.34,35 All these results demonstrated that the MWCNT based monolith has potential applications in the screening of antibiotics and pesticides using an integrated nano LC-HR Orbitrap MS analytical system. The system with the monolith exhibited easy access to routine analysis. The obtained results were also a proof which shows that the nano LC-HR Orbitrap MS system with the novel monolith introduced in this research report may provide the opportunity to analyze several classes of compounds.
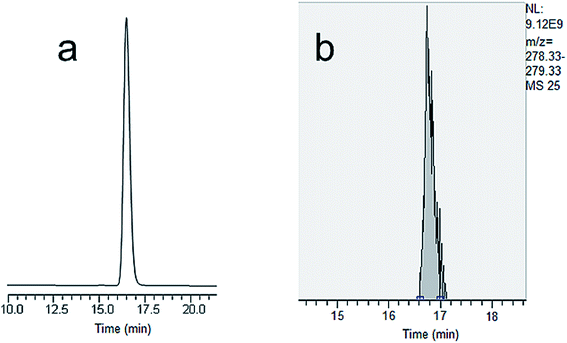 |
| Fig. 4 (a) Nano LC-UV chromatogram of sulfamethazine. (b) Extracted ion chromatogram of sulfamethazine using nano LC-HR Orbitrap MS. | |
Method validation
European commission (EU) required the control of residues in samples and consequently the analytical method should be based on analytical spectrometry detection (Commission Regulation, 1999). The validation was performed with the antibiotics because quantitative determination in milk samples could be achieved. Linearity, LOD and LOQ were applied to generate a calibration curve in the concentration range of 0.1 to 25 μg kg−1 of the antibiotics. (0.1, 0.5, 1.0, 5.0, 10, and 25 ppb). %RSD values were calculated using three different concentrations of spiked milk samples (0.25, 2.5, and 25 ppb). These values allowed us to estimate the precision of the method. The method validation parameters for the antibiotics are given in Table S3.† The obtained linear relationships (R2) were higher than 0.997 for all the compounds. The matrix extraction was evaluated using different ratios of ACN
:
H2O. This was optimized as 60
:
40 v/v. The use of nanospray ionization with the matrix matched calibration curves with appropriate dilutions provided negligible matrix effects. Matrix effects were almost removed for the antibiotics. This was a proof that shows the superior performance for the proposed method. The extraction recoveries ranged from 77 to 89%. The intraday/interday precisions for the samples were in the range of 0.5–11% for all the antibiotics. The LOQ was 0.21 μg kg−1 while the LOD was established at 0.1 μg kg−1 for the compounds.
Real sample application
Two milk samples (MS1 and MS2) from different local markets were analyzed. It was shown that no detectable antibiotics were found in the samples (n = 5). In addition, two honey samples (HS1 and HS2) obtained from different local markets were analyzed. The residues present in the honey samples were identified according to the same method. It was found that HS1 and HS2 showed detectable levels of sulfamethazine antibiotic (between 0.34 and 2.05ppb) although below MRLs in all cases. No deterioration of the monolithic column efficiency with more than 100 injections of real samples was observed.
The obtained results showed that the method with the novel monolith was highly sensitive. The developed method with the novel monolithic column was successfully applied for the determination of antibiotics in milk samples.
Conclusions
A novel MWCNT based monolith was successfully prepared and used as a stationary phase for nano LC-UV and nano LC-HR Orbitrap MS. The prepared column was investigated for the analyses of eight antibiotics and six pesticides. The MWCNT entrapped monolith exhibited high sensitivity and selectivity. The produced monolith yielded an efficiency up to 46
000 plates per m for the retained antibiotics. The carbon nanotubes significantly affected the separation performance of the monolith. The separation and analyses were conducted under hydrophobic interaction conditions. Based on the high efficiency in UV-based chromatographic separation, the monolith was used in an integrated nano LC-HR Orbitrap MS system. The use of monolith based nano-LC in combination with HR Orbitrap MS exhibited low limits of detection at the ppb level. This result was due to the use of the nanoscale monolith (75 μm i.d.), which led to increased ionization efficiency that originated from nanospray ionization. Monolithic reproducibility and parameters such as retention time and peak area were also evaluated with satisfactory results. The use of low flow rate (i.e. 800 nL min−1) was also another remarkable advantage related to solvent consumption. The sensitivity of the proposed method with the novel monolithic column was satisfactory. Monolith based nano LC-HR Orbitrap MS could be applied to routine residue analysis with high sensitivity (at the ppb scale) and reproducibility.
Conflicts of interest
All authors declare that we have no conflict of interest.
References
- H. Shimizu, K. Morikawa, Y. Liu, A. Smirnova, K. Mawatari and T. Kitamori, Analyst, 2016, 141, 6068–6072 RSC.
- J. Hernández-Borges, Z. Aturki, A. Rocco and S. Fanali, J. Sep. Sci., 2007, 30, 1589–1610 CrossRef.
- C. Aydoğan, Anal. Bioanal. Chem., 2016, 408, 8457–8466 CrossRef.
- C. Aydoğan, J. Chromatogr. A, 2015, 1392, 62–68 CrossRef.
- J. Alcántara-Durán, D. Moreno-González, B. Gilbert-López, A. Molina-Díaz and J. F. García-Reyes, Food Chem., 2018, 245, 29–38 CrossRef PubMed.
- Y. R. Wu, H. Y. Liu, S. L. Lin and M. R. Fuh, Talanta, 2018, 176, 293–298 CrossRef CAS.
- A. Berlioz-Barbier, A. Buleté, A. Fildier, J. Garric and E. Vulliet, Chemosphere, 2018, 196, 347–353 CrossRef CAS PubMed.
- C. Aydoğan, F. Yılmaz and A. Denizli, J. Sep. Sci., 2013, 36, 1685–1692 CrossRef.
- F. Svec and Y. Lv, Anal. Chem., 2015, 87, 250–273 CrossRef CAS PubMed.
- T. Hong, X. Yang, Y. Xu and Y. Ji, Anal. Chim. Acta, 2016, 931, 1–24 CrossRef CAS PubMed.
- F. Svec and J. M. J. Fréchet, Anal. Chem., 1992, 64, 820–822 CrossRef CAS.
- A. Eatemadi, H. Daraee, H. Karimkhanloo, M. Kouhi, N. Zarghami, A. Akbarzadeh, M. Abasi, Y. Hanifehpour and S. W. Joo, Nanoscale Res. Lett., 2014, 9, 393 CrossRef.
- N. Ganewatta and Z. El Rassi, Analyst, 2018, 143, 270–279 RSC.
- E. Mayadunne and Z. El Rassi, Talanta, 2014, 129, 565–574 CrossRef CAS PubMed.
- S. D. Chambers, F. Svec and J. M. J. Fréchet, J. Chromatogr. A, 2011, 1218, 2546–2552 CrossRef CAS PubMed.
- C. Miao, R. Bai, S. Xu, T. Hong and Y. Ji, J. Chromatogr. A, 2017, 1487, 227–234 CrossRef CAS PubMed.
- M. Zhang and H. Qiu, TrAC, Trends Anal. Chem., 2015, 65, 107–121 CrossRef CAS.
- A. Uclés Moreno, S. Herrera López, B. Reichert, A. Lozano Fernández, M. D. Hernando Guil and A. R. Fernández-Alba, Anal. Chem., 2015, 87, 1018–1025 CrossRef PubMed.
- A. Cappiello, G. Famiglini, F. Mangani and A. Siviero, Anal. Chim. Acta, 2003, 493, 125–136 CrossRef CAS.
- M. Luz Gómez-Pérez, R. Romero-González, V. José Luis Martínez and A. GarridoFrenich, Talanta, 2015, 131, 1–7 CrossRef PubMed.
- D. Moreno-González, P. Pérez-Ortega, B. Gilbert-López, A. Molina-Díaz, J. F. García-Reyes and A. R. Fernández-Alba, J. Chromatogr. A, 2017, 1512, 78–87 CrossRef PubMed.
- T. Vehus, K. E. Seterdal, S. Krauss, E. Lundanes and S. R. Wilson, Future Sci. OA, 2016, 2, 2 Search PubMed.
- C. Fanali, L. Dugo, P. Dugo and L. Mondello, TrAC, Trends Anal. Chem., 2013, 52, 226–238 CrossRef CAS.
- J. Šesták, D. Moravcová and V. Kahle, J. Chromatogr. A, 2015, 1421, 2–17 CrossRef.
- S. R. Wilson, T. Vehus, H. S. Berg and E. Lundanes, Bioanalysis, 2015, 7(14), 1799–1815 CrossRef CAS.
- C. Aydoğan and Z. El Rassi, J. Chromatogr. A, 2016, 1445, 55–61 CrossRef.
- D. Reznik, C. H. Olk, D. A. Neumann and J. R. D. Copley, Phys. Rev. B, 1995, 52, 116 CrossRef CAS.
- A. Bensghaïer, Z. Salmi, B. Le Droumaguet, A. Mekki, A. A. Mohamed, M. Beji and M. M. Chehimi, Surf. Interface Anal., 2016, 48, 509–513 CrossRef.
- Y. Gou and J. Pawliszyn, Anal. Chem., 2000, 72, 2774–2779 CrossRef CAS.
- G. Desmet and S. Eeltink, Anal. Chem., 2013, 85, 543–556 CrossRef CAS.
- M. Asensio-Ramos, G. D'Orazio, J. Hernandez-Borges, A. Rocco and S. Fanali, Anal. Bioanal. Chem., 2011, 400, 1113–1123 CrossRef CAS.
- K. Y. Zhu, K. W. Leung, A. K. L. Ting, Z. C. F. Wong, W. Y. Y. Ng, R. C. Y. Choi, T. T. X. Dong, T. Wang, D. T. W. Lau and K. W. K. Tsim, Anal. Bioanal. Chem., 2012, 402, 2805–2812 CrossRef CAS.
- M. F. Mirabelli, J. C. Wolf and R. Zenobi, Anal. Bioanal. Chem., 2016, 408, 3425–3434 CrossRef CAS.
- A. Cappiello, G. Famiglini, P. Palma and F. Mangani, Anal. Chem., 2002, 74, 3547–3554 CrossRef CAS.
- H. Y. Liu, S. L. Lin and M. R. Fuh, Talanta, 2016, 150, 233–239 CrossRef CAS.
Footnote |
† Electronic supplementary information (ESI) available. See DOI: 10.1039/c8ay02173b |
|
This journal is © The Royal Society of Chemistry 2019 |
Click here to see how this site uses Cookies. View our privacy policy here.