Urban sources of synthetic musk compounds to the environment†
Received
30th July 2018
, Accepted 27th November 2018
First published on 21st December 2018
Abstract
The occurrence and potential sources of synthetic musk compounds (SMCs) in the urban and surrounding environment were investigated. We analyzed air, soils and surface waters from a wide array of land-use types and urban densities including air from wastewater treatment plants (WWTPs), indoor, urban, rural, and remote Arctic sites; surface waters from urban and rural tributaries; and effluents of three WWTPs. In air, the median sum concentration of six selected polycyclic musks (Σ6PCMs) (i.e., galaxolide, tonalide, cashmeran, celestolide, phantolide, traseolide) were the highest from WWTP on-site > indoor > urban > WWTP off-site > rural. SMCs were not found in remote Arctic air indicating low potential for long-range atmospheric transport. SMCs were not found in soils, likely because of their high volatility and fast biodegradation rate. Galaxolide (HHCB) and tonalide (AHTN) were the two most abundant SMCs in air, tributaries and WWTP effluents. Σ6PCM concentrations in air taken along urban–rural transects and in tributary water were positively correlated with population density. In WWTP on-site air, trace levels of the toxic nitro-musks, namely musk xylene and musk ketone were detected and macrocyclic musks accounted for ∼10% of the total SMCs measured. In WWTP effluents, the concentrations of Σ6PCMs were proportional to the population served. We conclude that sources of SMCs to the outdoor urban environment and hence the surrounding region, originate from releases from indoor air, and temperature-dependent volatilization from WWTPs during treatment.
Environmental significance
Synthetic musk compounds (SMCs) are high production volume substances that are widely used in everyday consumer products. Some have similar physical–chemical properties to persistent organic pollutants. Little is known about the major sources, occurrence, fate and transport of SMCs in the environment. We found that SMCs in ambient air and tributary surface water were positively correlated with population density. Their levels in the environment were influenced by local emissions from indoor air and release from wastewater treatment plants. Most SMCs have short half-lives, and thus were not found in soils and Arctic air, and are unlikely to undergo long-range atmospheric transport. However, the continuous release of SMCs in high volumes from the urban environment, notably wastewater treatment plants, could pose a chronic toxicological threat to biota in receiving waters.
|
Introduction
Synthetic musk compounds (SMCs) are semi-volatile cyclic organic chemicals that are used as fragrance materials. These compounds are widely used in many personal care products, including soaps, shampoo, detergents, and deodorants, as well as other scented products such as air fresheners and scented candles. Four main groups of SMCs are marketed in categories according to their chemical structures, namely nitro-musks (NMs), polycyclic musks (PCMs), macrocyclic musks (MCMs), and alicyclic musks (ACMs).
NMs have similar physical–chemical properties to persistent organic pollutants such as organochlorine pesticides and polychlorinated biphenyls (Table 1). For example, the octanol–water partition coefficients (log
KOW) of musk ketone (MK) and musk xylene (MX) are 4.3 and 4.9, respectively,1,2 and octanol–air partition coefficient (log
KOA) is 12 for both MK and MX.3 NMs, particularly MK and MX, have been found to be weakly estrogenic and may increase the genotoxicity of other chemicals in a species-specific fashion.4,5 NMs are known to cause toxic effects at low concentrations in aquatic and terrestrial organisms.6,7 A recent exposure-based prioritization study indicated MX is a highly waterborne persistent contaminant that may pose potential planetary boundary threats.8 MX has been banned in the European Union and Japan as it has been identified as very persistent and bioaccumulative chemical.9,10 It is prohibited for use as fragrance by the International Fragrance Association (IFRA), a self-regulatory representative body of the worldwide fragrance industry. MK is not regulated by any government agency but it is listed on the third Priority Substances List under the superseded Existing Substances Regulation (ESR) of REACH.11 MK is prohibited by IFRA if it contains more than 0.1% of MX as an impurity.12 Other NMs, including musk ambrette (MA), musk tibetene (MT) and musk moskene (MM) are prohibited by IFRA. In Canada, use of MA and MT is prohibited for use in cosmetic products.13 Canada has also placed a Significant New Activity (SNAc) order on MM based on its potential toxicity, whereby ministerial approval is required for use in volumes greater than 100 kg per year.14
Table 1 Physical–chemical properties of synthetic musk compounds (refer to Table S1 for chemical name, structure, solubility and half-life in air)
Compound (abbrev.) |
CAS number |
MW (g mol−1) |
log KOW |
log KOA |
P
L (Pa) |
HLC (Pa m3 mol−1) |
Paasivirta et al., 2002.78
US EPA., 2018. 3 EPI Suite™ version 4.11. Specific methods used: (i) solubility – WSKOW v1.42, based on EPI Suite estimated log KOW; (ii) HLC – HENRYWIN v3.20 based on bond method; (iii) log KOW: KOWWIN v1.68; (iv) sub-cooled liquid VP – MPBPWIN v1.43, based on mod-grain method; log KOA; (v) log KOA: KOAWIN.
Wong et al., 2017.79
Tas et al., 1997.2
Balk et al., 1999.80
Lee et al., 2012.81
|
Nitro-musks
|
Musk tibetene (MT) |
145-39-1 |
266 |
5.2a |
10.1b |
0.0074a |
100.8a |
8.3c |
0.0068b |
0.0295b |
0.018c |
Musk ambrette (MA) |
83-66-9 |
268 |
4.3a |
10.4b |
0.081a |
25.6a |
0.0066b |
0.0014b |
Musk moskene (MM) |
116-66-5 |
278 |
5.8a |
10.5b |
0.011a |
257.6a |
7.8c |
0.0040b |
0.0208b |
0.11c |
Musk ketone (MK) |
81-14-1 |
29 4 |
4.3a |
12b |
0.011a |
5.8a |
0.00101b |
4.87b |
0.00004d |
0.0061d |
0.336f |
Musk xylene (MX) |
81-15-2 |
297 |
3.8a |
12b |
0.035a |
41.2a |
4.5b |
0.00058b |
0.000105b |
4.9d |
0.00003d |
0.018d |
3.11f |
![[thin space (1/6-em)]](https://www.rsc.org/images/entities/char_2009.gif) |
Polycyclic musks
|
1-Methyl-alpha-ionone |
7779-30-8 |
206 |
4.8b |
6.9b |
1.6b |
24.3b |
Cashmeran (DPMI) |
33704-61-9 |
206 |
4.9a |
6.7b |
0.012a |
11.6a |
4.5b |
1.5b |
14.4b |
Iso E super (OTNE) |
54464-57-2 |
234 |
5.2b |
6.9b |
0.56b |
47.6b |
Celestolide (ADBI) |
13171-00-1 |
244 |
6.6a |
8.8b |
0.15a |
2066a |
5.9b |
0.094b |
3.22b |
Phantolide (AHMI) |
15323-35-0 |
244 |
6.7a |
8.7b |
0.096a |
786a |
5.9b |
7.5c |
0.102b |
3.22b |
0.16c |
Traseolide (ATII) |
68140-48-7 |
258 |
8.1a |
9.07b |
0.039a |
113a |
6.3b |
7.7c |
0.053b |
4.28b |
0.079c |
Galaxolide (HHCB) |
1222-05-5 |
258 |
7.3a |
8.2b |
0.038a |
51.8a |
6.3b |
0.068b |
13.4b |
5.9e |
7.8c |
0.056c |
11.3e |
|
0.073e |
|
Tonalide (AHTN) |
21145-77-7 |
258 |
7.3a |
8.0b |
0.034a |
1210a |
6.3b |
0.047b |
4.28b |
5.7e |
0.068e |
12.5e |
![[thin space (1/6-em)]](https://www.rsc.org/images/entities/char_2009.gif) |
Macrocyclic musks
|
Exaltone |
502-72-7 |
224 |
5.6b |
7.1b |
0.13b |
66b |
6.6c |
1.4c |
Muskone |
541-91-3 |
238 |
5.9b |
7.4b |
0.109b |
88b |
Exaltolide |
106-02-5 |
240 |
6.2b |
7.2b |
0.0080b |
235b |
Ambrettolide |
7779-50-2 |
252 |
5.4b |
6.3b |
0.0036b |
274b |
7.4c |
0.33c |
16-Hexadecanolide |
109-29-5 |
254 |
6.7b |
7.6b |
0.0040b |
312b |
7.4c |
0.22c |
Musk MC-4 |
54982-83-1 |
256 |
4.2b |
8.2b |
0.00033b |
0.24b |
7.8c |
0.11c |
Cervolide |
6707-60-4 |
256 |
4.9b |
8.0b |
0.0033b |
2.07b |
7.7c |
0.14c |
Ethylene brassylate |
105-95-3 |
270 |
4.7b |
8.6b |
0.00016b |
0.32b |
In the U.S., the national production volume of galaxolide (HHCB), the most widely used PCM worldwide, increased from approximately one million kg per year in 2011 to up to four million kg per year during 2012–2015.15 Such an increase may be due to increased market demand for the chemical and the shift from NM to PCMs in the U. S.16 Similar phenomenon is also observed in the European market as a result of the strict international regulation of NMs.17
Although the toxicity of PCMs is believed to be low, some evidence suggests that they may cause endocrine disruption through anti-estrogenic activity.4,18,19 PCMs can also act as hormonal agonists and antagonists in humans and wildlife.20,21 Recent studies have found that PCMs could have harmful health effect.22,23 For example, Fang et al.22 reported that tonalide (AHTN) can act as a photosensitizer to increase photo-induced oxidative damage to natural amino acid on skin surfaces. Gao et al.24 reported that indirect photochemical transformation products of AHTN in water could have up to 8 times higher bioconcentration factor than parent AHTN. Quantitative Structure Activity Relationships (QSAR)-based risk assessment indicated that HHCB could pose a risk to biota exposed through sediment, soil, water and in some cases air by Homem et al.25
These potential effects of PCMs, when combined with their propensity to bioaccumulate due to their high lipophilicity (log
KOW ∼ 4.5–7.0, Table 1), have led to concern about their long-term human and environmental health effects.25–28 As such, IFRA has restricted the use of phantolide in “leave-on” products to no more than 2%.12 Otherwise, there are no regulations in the use of other PCMs.
MCMs are more easily degraded than NMs and PCMs.29 The production volume of MCMs is less than PCMs, but they are becoming more available because of advances in synthesis technology. Hence, increased use of MCMs is expected. ACMs are the fourth generation of odorant musks, but their current use in personal care products is very limited.
Most studies on SMCs have focused on NMs and PCMs with fewer conducted on MCMs.1,30,31 NMs and PCMs have been reported in indoor dust,1,32–34 indoor air,32,35,36 outdoor air,37–42 biota;43–48 surface waters;37,41,49–51 sea water,37 sediments,52 wastewater treatment plants (WWTPs);53–55 drinking water,56 human adipose tissue,45,57,58 breast milk,31,59–61 and serum.59,62
The potential for SMCs to undergo long-range transport is debatable. Villa et al.63 reported that AHTN and HHCB were chemicals with high persistence and low long-range transport potential based on their overall persistence of 245–250 days, a characteristic travel distance of 150 km and a very low transfer efficiency. Given the short half-lives of most SMCs (e.g. PCMs and MCMs) on the order of hours due to photodegradation, long-range transport is not anticipated.64 HHCB and AHTN were not found in polar bears in Alaska45 but HHCB, cashmeran (DPMI), traseolide (ATII), and MT were detected in polar bears in Greenland.65
Melymuk et al.42 estimated that the main loadings of PCMs (i.e. sum of HHCB and AHTN) to Lake Ontario were from waste water treatment plant (WWTP) effluent which accounted for 83% of total loadings, with minor contributions from atmospheric deposition (10%) and tributary discharge into the lake (7%). McDonough et al.41 reported that in Lake Ontario and Lake Erie, HHCB underwent net volatilization from water to air but the flux was variable for AHTN. The authors suggested that volatilization could be responsible for the loss of 64 to 213 kg PCMs per year from the lakes.
Harrad and Diamond66 proposed a conceptual model for source-to-receptor transport of chemicals contained within a stock or inventory of materials and products (Fig. S1†). For chemicals used in everyday commodities, this inventory is held mainly indoors, which is the first environment into which the chemicals migrate. From indoors, chemicals migrate outdoors into the urban environment. Persistent chemicals will be exported from the urban area mainly by atmospheric movement and a smaller, but significant fraction will find its way to urban soils and surface waters. Very persistent chemicals will be transferred through terrestrial and aquatic food webs from which the chemicals may return to the urban area in foods that we eat. Although most SMCs are not persistent due to their ability to degrade via photolysis, their continual use and release has rendered them “pseudo-persistent” in densely populated locations.
Our main goal is to use the conceptual framework of Harrad and Diamond66 to interpret measured concentrations of SMCs from potential urban sources, to receptor regions in rural or remote areas. This analysis includes data from air (i) indoors in offices and homes, (ii) outdoors along urban-rural transects, (iii) outdoors at an urban site in downtown Toronto, (iv) immediately adjacent to and at a distance from WWTPs, (v) outdoors at a rural site on the shore of Lake Ontario, and (vi) outdoors at a remote site in the Canadian High Arctic. We also consider SMCs in soils, final effluents from wastewater treatment plants (WWTPs), and surface waters of tributaries in Ontario, Canada. Our secondary goal was to establish baseline environmental concentrations of SMCs in different media in Canada.
Methods
Air and soil samples
Sampling Strategy.
Fig. 1 shows a map of our sampling locations. Air samples were collected using a combination of active and passive air sampling techniques. Here we give a brief description of the sampling locations and analytical method. Details can be found in the references cited and in ESI.†
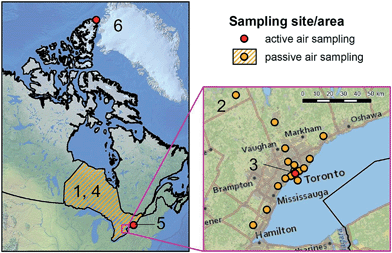 |
| Fig. 1 Map of air sampling sites. (1) Indoor passive air sampling in 20 homes and offices in Ontario; (2) passive air sampling sites on 3 urban-rural transects in Ontario; (3) urban active air sampling in city of Toronto; (4) eight WWTP in Ontario by passive air sampling; (5) rural active air sampling on the shore of Lake Ontario; (6) remote active air sampling in the Canadian High Arctic, alert. Passive samplers are indicated by orange dots and active sampling sites by red dots. | |
(i) Indoor air from homes and offices was sampled using polyurethane foam (PUF) disk-type passive samplers (PUF-PASs) (n = 20). Samplers were deployed for 30 days from August to September 2006. Approximately 25 m3 of air were collected for each sample, assuming a sampling rate of 0.7 m3 per day. Further details about each sampling site and methods are described in Zhang et al.67 (ii) Outdoor air and soils along three urban-rural transects that extended east, west, and north from downtown Greater Toronto Area (GTA). Air samples were collected using PUF-PAS deployed at 19 sites exposed for 3 months each, for over four seasonal periods during 2007–2008. Each sample represented ∼250 m3 of air, assuming a sampling rate of 2.69 m3 per day. The sampling rate was determined based on a 125 day calibration study specifically designed for SMCs.68 Details about the passive sampling sites are reported by Melymuk et al.69 Surface soils (n = 19) were collected at each passive air sampling site concurrent with initial deployment of sampler (October 2007). (iii) Outdoor air from an urban site, city of Toronto (n = 32) was obtained using a high volume active air sampler (HV-AAS). Glass fibre filter (GFF) and PUF plug samples were collected for particle- and gas-phases, respectively, every 12 days during 2007–2008. Each sample represented ∼450 m3 of air.70
Archived air extracts provided by Environment and Climate Change Canada (ECCC) were also analyzed: (iv) WWTPs (n = 32), where air samples were obtained from 8 plants during the summer (August to November 2013) and winter (January to March 2014). Sorbent-impregnated polyurethane foam (SIP) disk-type PAS were deployed “on-site” (i.e. above the aeration tank or adjacent to the lagoon) and “off-site” (i.e. ∼100–150 m away from the active area on the premises of the WWTP). Details of the sampling sites and methods are presented by Shoeib et al.71 A sampling rate of 4 m3 per day derived for poly- and perfluroalkyl substances (PFASs) and organophosphate esters by SIP-PAS was used.71–73 On average each sample represented ∼ 280 m3 of air. (v) A rural site located at the nearshore of Lake Ontario (n = 32). The air samples were collected in 2010 using an HV-AAS, with GFF and PUF plug as sorbents. About ∼340 m3 of air were collected for each sample.74(vi) A remote site in the Canadian High Arctic (n = 21), where the air samples were obtained at Alert, Nunavut, Canada, during 2009–2010 using an HV-AAS with GFF, and PUF/XAD/PUF sandwich as sorbents. Each sample represented 2000 m3 of air. Description of sampling method is given in Wong et al.75
Extraction and clean-up.
Air samples from (i) indoor and (ii) urban-rural transects, and (iii) the urban sites, and soil samples were analyzed at the University of Toronto (UT). Details of the extraction and clean-up methods have been previously reported.68 In brief, PUFs and filters were extracted by a Dionex ASE350 (Accelerated Solvent Extraction System) with dichloromethane (DCM). All samples were spiked 50 ng of d10-fluoranthene prior to extraction. Samples were cleaned using a 1 g silica solid-phase extraction (SPE) cartridge (Varian, Canada) with 25 ml of 50
:
50 DCM/hexane.
Each soil sample (∼25 g) was combined with anhydrous sodium sulphate, then placed in a pre-cleaned cellulose thimble, and extracted for 18 hours in DCM in a Soxhlet apparatus. d10-Fluoranthene (50 ng) was added to the thimble prior to extraction, and 2 g of activated copper was added to the solvent collection flask for sulfur removal. The soils underwent the same clean-up and analytical procedure as the air samples described above.
The air samples from the (iv) WWTPs (SIP-PAS), (v) rural (Lake Ontario) and (vi) remote (Arctic) site were analysed at ECCC. The WWTP air samples were extracted by a Dionex ASE350 using petroleum ether/acetone (83/17, v/v). The extracts did not undergo clean-up procedures. Details of the analytical procedure have been reported.71 The rural air samples were extracted using a Soxhlet apparatus with hexane, dried with anhydrous sodium sulfate, and cleaned-up using a florisil column. The analytical procedure has been previously published.74 The remote air samples from the Canadian Arctic were extracted by Dionex ASE350 with hexane. The extracts did not undergo clean-up procedures.75
Tributary surface waters and wastewater treatment plant effluent
Sampling strategy.
Tributary surface water samples were collected in 2008 from 10 sites across the GTA that reflected a range of urban densities and land use types, and encompassed the 6 major watersheds in the area (Fig. 2). We sampled during 7 events of which 5 and 2 were taken under high and base flow conditions, respectively. At each sampling event, one 19 L sample was taken using a remotely triggered ISCO 6712 pump into pre-cleaned stainless steel canisters, after which it was stored at 4 °C until analysis.
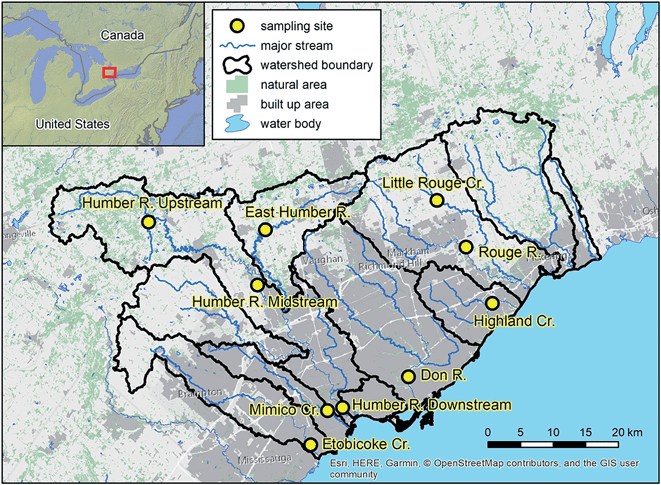 |
| Fig. 2 Map of tributary surface water sampling locations. | |
Effluents of three WWTPs in the GTA were collected in 2008. The samples were obtained after the final treatment stage at each plant, immediately prior to being released into the receiving waters. Each 8 L sample was collected over the course of 24 h from each site using a remotely triggered ISCO 6712 pump into pre-cleaned amber glass bottles, which were immediately retrieved and stored at 4 °C until analysis. Ten samples were collected from each site over the same time period as the tributary surface water samples. Further details are provided by Melymuk et al.42
Extraction and clean-up.
SMCs were extracted from the water and wastewater samples by filtering them through a pre-cleaned GFF (142 mm diameter, 1.2 μm nominal pore size) followed by a XAD-4 sorbent resin column (30 mL bed volume). Both the XAD column and filter were then spiked with the internal standard (d10-fluoranthene) and extracted on a Dionex ASE300 using acetone/hexane (1
:
1, v/v). The GFF and XAD extracts were then combined and dehydrated on a sodium sulfate column. Extracts were then purified as per the passive air samples that were taken at the urban-rural transects. Procedures are reported by Melymuk et al.42
Instrumental analysis
Table 1 lists the target SMCs along with their physical–chemical properties including partition coefficients of octanol–water (KOW), octanol–air (KOA), Henry's law constant (HLC), and sub-cooled liquid vapour pressure (PL). The structures, chemical name, CAS no., supplier, % purity of standard used, solubility and half-live in air due to hydroxyl radical (OH) reaction are given in Table S1.† The indoor air, urban-rural transect air, urban air, soils, tributary surface waters and WWTP effluents were analysed at UT for 6 PCMs, namely cashmeran (DPMI), galaxolide (HHCB), tonalide (AHTN), phanolide (AHMI), celestolide (ADBI), traseolide (ATII). Analysis was achieved by gas chromatography-mass spectrometry analysis (GC/MS), using an Agilent 6890N gas chromatograph coupled to an Agilent 5975 Inert Mass Selective Detector (MSD) in electron impact (EI) mode. GC/MS operation parameters are presented in S1.† The target SMCs were quantified against d10-fluoranthene which was added prior to extraction. d10-p-Terphenyl was added prior to instrumental analysis and it was used to quantify the recovery of d10-fluoranthene.
The archived air samples from the WWTPs, rural and remote Arctic sites were analyzed at ECCC for 21 musks, including the 6 PCMs analyzed at UT. Samples were analyzed using an Agilent 7000C triple quadrupole MS connected to a 7890B GC, operated in multiple reaction mode (MRM) under EI conditions. The monitoring transitions for each target chemical are presented in Table S2.† Details about the operation parameters of the instrumental analysis are given in S1.† The SMCs were quantified against mirex, which was added prior to instrumental analysis.
Quality assurance and quality control
Each of the air sampling techniques that were used has been tested for many groups of persistent organic pollutants (POPs) such as polychlorinated biphenyls (PCBs), organochlorine pesticides and polybrominated diphenyl ethers (PBDEs).76,77 Since PCMs have similar physical and chemical properties as POPs (e.g. log
KOA ∼ 8), we believe that these collection techniques were suitable for PCMs.
The validity of the analytical method for SMCs in air, tributary surface water and WWTP effluents analyzed by UT was assessed through spikes of native musks to PUF-PAS (n = 5) and XAD resins used for tributary surface water samples (n = 5). These spiked samples underwent the same analytical procedures as the air and water samples. Recoveries of native musks were 87% and 111%, respectively, for PUF-PAS and XAD. Mean recoveries for d10-fluoranthene was ∼80%. Further information about the QA/QC is given by Melymuk et al.42
The validity of the method for SMCs in air analyzed by ECCC was evaluated by external spike experiments to assess the recovery of 21 native SMCs on PUF-plugs (n = 3) and filters (n = 3). The spike samples were extracted by Dionex ASE350 with hexane and did not undergo clean-up. Recoveries of the 21 native SMCs ranged from 66–93% for the filters and 82–153% for the PUFs (Table S3†), which was considered satisfactory. The mean recoveries and standard deviations of individual native SMC spiked to each matrix is presented in Table S3.†
All sample and blank extracts were stored in amber GC vials, sealed with Teflon tape and stored in a freezer at −10 °C. Calibration standards were stored in a separate freezer to prevent contamination of the sample extracts. Most samples were analyzed for SMCs within two years of sample collection. These included the indoor passive air samples, passive air and soil samples collected along the 3 urban-rural transects, urban active air samples, tributary surface water samples and wastewater treatment plant effluent samples. The archived air samples from the eight WWTPs in Ontario, rural site on the shore of Lake Ontario and remote site in the Canadian High Arctic were analyzed within 7 years of sample collection. To reduce blank contamination, all analysts were encouraged to reduce the use of any SMC-containing products. No SMC-containing products were allowed into the laboratories where the analyses were carried out. Trace amounts of SMCs were found in most blanks. The mean ± standard deviation (sd) of blank values for air and water are given in Table S5.† All data were blank corrected and non-detects were replaced with 2/3 instrumental detection limit (IDL) when performing statistical calculations. Values that were below 3 × sd of the mean blank were considered non-detect. IDL and blank values are given in Tables S4 and S5,† respectively. Statistical analysis was performed using Microsoft Excel 2010 and OriginPro 2017. We report total air concentrations as the sum of chemicals found in the filters and PUFs for samples which were collected by HV-AAS. We used medians and ranges to describe chemical concentrations in the discussion. As the air and water data were not normally distributed, Mann–Whitney test was used to assess the significant difference between datasets.
Readers are advised to interpret the data with caution in light of possible compromising factors including potential contamination and degradation of the less recalcitrant SMCs, such as the macrocyclic musk compounds. Particular care should be exercised in consideration of the archived air extracts from the eight WWTPs in Ontario, rural site on the shore of Lake Ontario and remote site in the Canadian High Arctic because of the extended extract storage time.
Results and discussion
Indoor air
Air concentrations of individual indoor sample were given in Table S6.† The sum of six polycyclic musks, (Σ6PCMs), i.e., DPMI, ADBI, AHMI, ATII, HHCB, AHTN, in indoor air (n = 20) ranged from 0.42–200 ng m−3, with a median concentration of 8.6 ng m−3. AHMI was found in 80% of the samples and the other 5 PCMs were detected in 100% of the samples. Median concentrations of Σ6PCMs in homes (n = 10, 12 ng m−3) were 3 times greater than those in offices (n = 10, 3.8 ng m−3) but not significantly different (p > 0.05). We found concentrations of DPMI in two homes (162 and 152 ng m−3) and one office (79 ng m−3) that were up to 5 orders of magnitude greater than those at other indoor locations (range 0.009–0.37 ng m−3). PCMs in indoor air sorted in order of highest to lowest median concentration (ng m−3) were: HHCB (4.5) > AHTN (2.1) > ADBI (0.15) > DPMI (0.090) > ATII (0.083) > AHMI (0.030). Excluding the three indoor environments that had high levels of DPMI, indoor air (n = 17) was dominated by HHCB (60%) and AHTN (29%) (Fig. S2†).
The overall range of HHCB and AHTN in our indoor air were respectively, 0.30–18 ng m3, and 0.09–17 ng m−3. These levels were much lower than those previously reported by Regueiro et al.36 in homes from Spain, in which HHCB and AHTN, respectively, were in the range of 140–1130 ng m−3 and 21–77 ng m−3; and those from Fromme et al.32 in German kindergardens, in which HHCB and AHTN have mean concentrations of 120 ng m−3 and 47 ng m−3, respectively. Sofuoglu et al. reported HHCB and AHTN range from 145–270 ng m−3 and 41–58 ng m−3, respectively in classroom and sports center in Turkey. The wide-range in concentrations of SMCs in indoor air may be due to the diverse application and amount of SMC-containing consumer products, area of use, and type of building ventilation system.
Outdoor air and soils
Urban-rural transects in Toronto.
Air concentrations along the three transects (total 19 sites) are presented in Table S7.† Detection frequencies of ADBI, AHMI, ATII, HHCB, and AHTN ranged from 78–93%. DPMI was not found in any of the samples. The concentration of Σ6PCMs (DPMI, ADBI, AHMI, ATII, HHCB, AHTN) along the North transect ranged from 0.002–4.6 ng m−3, the west transect ranged from 0.014–3.8 ng m−3 and the east transect 0.31–0.46 ng m−3. HHCB was the most abundant PCM detected with a median concentration, of 0.99 ng m−3, followed by AHTN, with a median concentration of 0.22 ng m−3. The median percent contribution of HHCB and AHTN to Σ6PCMs was 77% and 19% respectively.
Air concentrations of Σ6PCMs along the urban-rural transect were positively correlated with population density (Fig. 3).69 In general, the median Σ6PCMs was ∼3 times higher at the 3 most urban sites, which were located at 0 km, 1 km, and 5 km from the city centre, than at the 3 most rural sites, which were located 40 km, 60 km and 80 km from the city centre. Our results are consistent with those of McDonough et al.41 who found significant correlations between population density and Σ5PCMs (ADBI, AHMI, ATII, HHCB, AHTN) in both air and water. We hypothesize that PCM sources supporting this gradient are the collective emissions from indoor air via building ventilation and from WWTPs during the treatment process. The gradient is also accentuated by the relatively short atmospheric half-lives of the PCMs, which preclude significant atmospheric transport. Half-lives of these 6 PCMs in air (t1/2,AIR) due to reaction with the hydroxyl radical range from 2.4 to 33 h (Table S1†).
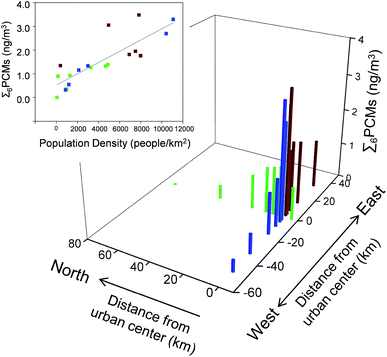 |
| Fig. 3 Sum of 6 PCMs (Σ6PCMs) along the urban-rural transects and correlation with population density. Urban centre is indicated as 0 km on the x and y scales. Red bar indicates the east transect, blue bar indicates the west transect and green bar indicates the north transect. Readers are referred to the web version of this article for interpretation of the references to colour. | |
In soils, none of the PCMs were found at detectable levels (i.e. in excess of the levels found in the laboratory blanks). The low levels of PCMs in soils were expected given the relatively high volatility (vapour pressure ranged from 0.034 to 1.6 Pa (ref. 3)) and fast biodegradation rate.82,83 Therefore, despite high usage of PCMs in urban areas, urban soils are not considered to be a reservoir of PCMs in the environment.
Urban site at downtown Toronto.
The total gas- and particle-phase concentrations of PCMs in Toronto urban air (n = 32) are given in Table S8.† The median concentration of Σ6PCMs (DPMI, ADBI, AHMI, ATII, HHCB, AHTN) was 1.5 ng m−3, ranging from 0.033–4.4 ng m−3 or within 2 orders of magnitude at the same site. DPMI was not found in any of the samples at this urban site. Median concentrations of HHCB and AHTN were 1.2 and 0.26 ng m−3 respectively. The median percent contribution of HHCB and AHTN to Σ6PCMs was 80% and 16%, respectively (Fig. S2†).
Wastewater treatment plants in Ontario.
Eleven SMCs (MX, MK, DPMI, ADBI, AHMI, HHCB, AHTN, 16-hexadecanolide, musk M4, cervolide, ethylene brassylate) were found in the air from 8 WWTPs in Ontario. Data are given in Table S9.† The median concentration of Σ11SMCs in on-site air (WWTP-On) (mean of winter and summer) was 25 ng m−3 (range 0.36–166 ng m−3), and off-site air (WWTP-Off) was 0.38 ng m−3 (range 0.19–35 ng m−3). Thus, concentrations dropped by ∼100 times within 100–150 m of the source. It is also interesting to note that the WWTP-On concentrations were similar to those measured indoors, as discussed below.
Again, HHCB and AHTN were the dominant musks in all samples at both on-and off-site locations and seasons (Fig. S2†). In the WWTP-On air, HHCB ranged from 0.22–115 ng m−3 and AHTN ranged from 0.11–45 ng m−3. In the WWTP-Off air, HHCB ranged from 0.091–22 ng m−3 and AHTN ranged from 0.049–11 ng m−3. Nitro-musks (MK, MX) were found in WWTP-On air with concentration ranges of MK of <0.0017–0.23 ng m−3 and MX of <0.0028–0.21 ng m−3. MK and MX were mostly non-detectable in WWTP-Off air (MK < 0.0017–0.104 ng m−3; MX 0.0028–0.095 ng m−3). Several MCMs (i.e. 16-hexadecanolide, musk MC-4, cervolide and ethylene brassylate) were found in the WWTP air at low concentrations. The sum of these four MCMs ranged from 0.052–20 ng m−3 for WWTP-On air and 0.053–1.9 ng m−3 for the WWTP-Off air. Fig. S2† shows the composition of SMCs in the WWTP-On air. HHCB, AHTN and cervolide accounted for 60%, 23% and 5.3% of the sum of SMCs. It is noted that overall PCMs and MCMs respectively accounted for 92% and 7% of the Σ11SMCs found in the WWTP-On air. Our results agree with those of Upadhyay et al.55 who reported that air from aeration basins of WWTPs in Arizona, U. S. was dominated by HHCB and AHTN. Our data support their contention that direct emissions of PCM from aeration tanks contributes to the environmental occurrence of SMCs.
We analyzed the correlation between the Σ11SMCs from the WWTP-On air and selected operational parameters of the specific WWTP71 (Fig. 4). Σ11SMCs in WWTP-On air were positively correlated with population served (p < 0.001, r = 0.77) and influent water flow rate (p < 0.001, r = 0.80, but were negatively correlated with retention time (p < 0.001, r = −0.91) which may reflect greater removal of musks in the wastewater with extended time allowed for microbial activity in the aeration tank/lagoon.
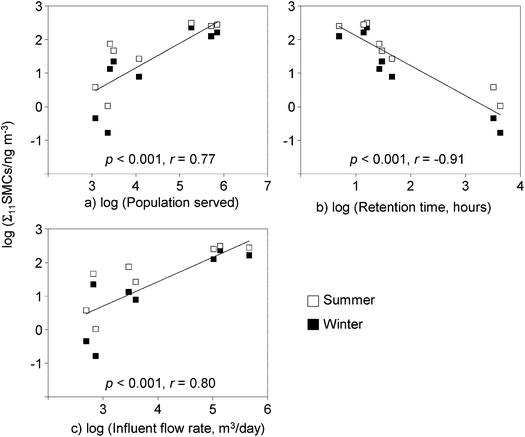 |
| Fig. 4 Correlation between Σ11SMCs in WWTP-On air (ng m−3) and selected operational parameters, (a) population served, (b) hydraulic, retention time and (c) influent water flow rate. | |
Rural site in the Great Lakes Basin and remote site in the Arctic.
Only HHCB and AHTN were detected in the gas-phase extracts from the rural site in the Great Lakes Basin (n = 32) (Table S10†). No SMCs were detected in the particle-phase. The median concentration (range) for HHCB was 0.031 (<0.00032–0.072) ng m−3 and for AHTN was 0.028 (<0.00024–0.038) ng m−3. A similar proportion of HHCB (54%) and AHTN (46%) was found (Fig. S2†). At the remote site in the Arctic (n = 21), SMCs were not detected in any samples, except in one sample in which we found HHCB, musk M4 and ethylene brassylate. It is suspected that this sample had been contaminated during sample collection or analysis.
Overview of SMCs in air
Fig. 5 shows the Σ6PCMs (DPMI, ADBI, AHMI, ATII, HHCB, AHTN) found in air from the WWTPs (on- and off-sites), Indoor, Urban and Rural sites (left panel) and the urban-rural transects (right panel). Median Σ6PCMs of the 5 site types sorted in the order of highest to lowest concentrations were: WWTP-On > indoor > urban > WWTP-Off > rural. PCM concentrations in WWTP-On and indoor air were not significantly different, but both of them were significantly higher than all other sites, including the urban air, WWTP-Off and rural air. PCM concentration in rural air was significantly lower than all other sites. Σ6PCMs in passive air samples from sites that were closer to the urban center is within the range of those collected by active air sampling at the urban site of Toronto. Σ6PCMs in passive air samples from sites that were further away from the urban center (>40 km) were similar to those obtained in rural site (Fig. 5, right panel). Our results imply that WWTPs and indoor environment are likely significant sources of SMCs to outdoor air, supporting the hypothesis of Harrad and Diamond (2006).66
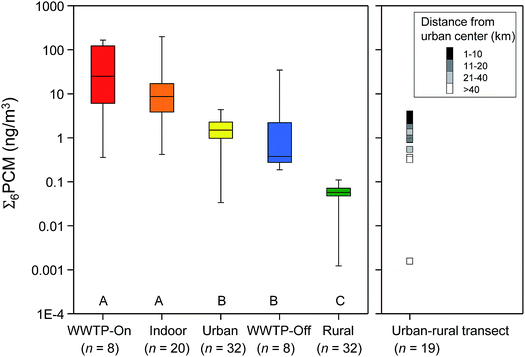 |
| Fig. 5 The sum of 6 PCMs (Σ6PCMs) found in air from: the on-site locations of the WWTPs (WWTP-On), indoor, urban, the off-site locations of the WWTPs (WWTP-Off) and rural site. n is the number of samples. The mid-line represents the median, whiskers are the maximum and minimum and the box represents 25th and 75th percentile of the data. WWTP-On refers to air taken above the aeration tank or adjacent to the lagoon. WWTP-Off refers to air ∼100 m away from the premises of the WWTP. The mean of summer and winter data were used to summarize the levels in WWTP-On and WWTP-Off air. The urban and rural sites were represented by downtown Toronto and nearshore of Lake Ontario, respectively. Data for the urban-rural transect represent the mean of four sampling periods. Compounds sharing the same letter are not statistically different (p > 0.05). | |
We plotted literature concentrations of SMCs according to the nature of the sampling sites in Fig. 6 along with results from our study. The overall concentration gradients found for literature data were consistent with our results, with the highest SMC concentration found in indoor air, followed by urban, rural and remote air. The literature indoor air concentrations of the selected chemicals (HHCB, AHTN, ADBI, AHMI an MX) were higher than our WWTP-On and indoor air. In general, literature air concentrations of SMCs from the urban sites were in the same range as those in our urban air. The HHCB and AHTN levels in our rural site were in the lower range of those in the literature rural sites. Data about the nitro-musks in the literature were scarce and their levels were variable among the sampling sites.
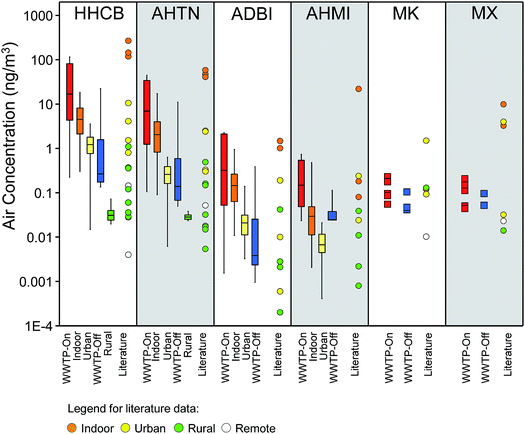 |
| Fig. 6 Air concentrations of SMCs measured in this study and the literature. The literature data are given in Table S11.† Data from this study is shown as whisker–box plot. Refer to Fig. 5 for definitions of the whiskers and box. Data for MK and MX from this study are shown as individual data because only few data was available. Reference for the indoor air (orange dot) – Fromme et al.,32 Sofuoglu et al.,35 urban air (yellow dot) – Peck et al.,38,39 Ramírez et al.,84 McDonough et al.,41 rural air (green dot) – Peck et al.,38,39 Xie et al.37 McDonough et al.;41 remote air (white dot) – Xie et al.,37 Kallenborn et al.85 | |
Temperature dependence and seasonal trend of SMCs
The temperature dependence of selected SMCs was investigated in air from the urban and rural sites obtained by HV-AAS every 12 days (Fig. 7). We observed a negative correlation (p < 0.05) between the natural log gas-phase concentrations (ln
C) of HHCB and AHTN and the inverse temperature at both these sites and ATII at the urban site. Air concentrations were generally higher during the warm season than the cold season, consistent with greater volatilization from sources (e.g., WWTP) and/or the effect of greater releases of indoor air during warm weather when ventilation increases. Peck and Hornbuckle39 also found a strong negative relationship between HHCB and AHTN in outdoor air in Iowa and inverse temperature. We observed that Σ11SMCs in SIP-PAS-derived air concentrations from the WWTP-On and WWTP-Off site taken during the summer were greater than those in the winter which is consistent with greater temperature-dependent volatilization (Fig. S3†). At WWTP-On, the median concentration of Σ11SMCs in summer was 41 vs. winter 7.1 ng m−3, at WWTP-Off, the level of Σ11SMCs in summer was 0.84 vs. winter was 0.20 ng m−3. No significant correlation was observed between concentrations of ADBI and AHMI and temperature and the reason is unclear.
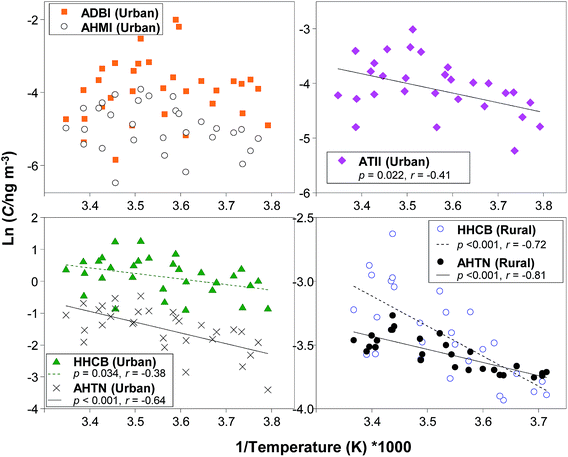 |
| Fig. 7 Correlations between gas-phase air concentrations of selected PCMs (ln (C/ng m−3)) and inverse temperature (T, K−1) at urban and rural sites. Linear regression statistics are shown for significant correlations. r represents the Pearson's correlation coefficient. Air concentrations were obtained from a HV-AAS. | |
HHCB/AHTN ratio was calculated to investigate potential sources. Here, the ratio of HHCB/AHTN was calculated when both compounds were above the detection limit in the same sample. The ratio should decrease with distance from the source because HHCB (t1/2,AIR = 0.22 d (ref. 64)) has a shorter atmospheric lifetime than AHTN ((t1/2,AIR = 0.60 d (ref. 3)). The median (range) of HHCB/AHTN ratios were as follows: WWTP-On: 2.6 (1.5–6.8); indoor: 2.3 (0.88–4.0); urban: 4.9 (0.98–7.8); WWTP-Off: 2.2 (0.71–7.0); and rural: 1.2 (0.82–1.9). The HHCB/AHTN ratio at the urban site agrees well with those reported in the nearshore air samples from Toronto by McDonough et al.41 of 5 ± 1. However, it is odd that the ratio for indoor air, the presumed source of these compounds that are not influenced by atmospheric loss processes was lower than for urban air. HHCB/AHTN ratio of 0.9–1.7 in air in the North Sea and 0.1–0.4 in air in the Arctic was reported by Xie et al.37 In a urban site in Germany, ratio of HHCB/AHTN was 3.5, which was similar to our HHCB/AHTN ratio at the urban site. We note that the ratio of HHCB/AHTN is influenced by the difference in volatility of the two chemicals. However, the extreme uncertainty in the Henry's law constant of AHTN, which ranged from 4.28–1210 Pa m3 mol−1, makes the interpretation difficult (Table 1).
Our results suggested that the occurrence of PCMs in ambient air is due to local emissions from indoor air ventilation, as well as volatilization from WWTP during the treatment process. We did not observe any evidence of regional or long-range transport of the PCMs or other SMCs. This is likely because most SMCs are short-lived chemicals (Table S1†).
Surface waters of tributaries in GTA and wastewater plant effluents
Concentrations of PCMs in the tributary surface waters are given in Table S12,† and are summarized in Fig. 8 and S4.† A strong urban-rural trend was evident with tributary concentrations 1–4 orders of magnitude higher at the urban (n = 5) than at the rural sites (n = 5). Specifically, the median Σ6PCM concentrations of the seven sampling events ranged from 0.016 ng L−1 at the rural site, Humber River Upstream, to 100 ng L−1 at the urban site, Don River. In general, median concentrations at the urban sites (Σ6PCM range 3.9–100 ng L−1) exceeded those at the rural sites (range 0.016–2.7 ng L−1). These values were less than those reported elsewhere e.g. the Elbe River in Germany at 184 ng L−1 Σ5PCM,86 the Glatt River in Switzerland at 214 ng L−1 Σ5PCM,58 and the Ruhr River in Germany at 600 ng L−1 Σ5PCM.87
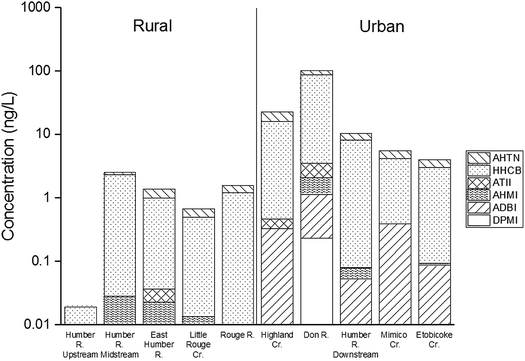 |
| Fig. 8 Median concentrations of PCMs (ng L−1) in surface waters of tributaries in rural and urban sites of the Greater Toronto Area (GTA). | |
HHCB and AHTN dominated SMCs in the tributary surface water, which respectively accounted for 74% (48–87%) and 19% (9–27%) of Σ6PCMs. Other PCMs contributed <2% of Σ6PCMs. The median concentrations of PCMs in the urban sites ranged from 2.9–83 ng L−1 for HHCB and 0.99–15 ng L−1 for AHTN, which generally exceeded those in Lake Ontario and Lake Erie for HHCB and AHTN of non-detect to 1.6 ng L−1 and 0.014 to 0.16 ng L−1, respectively.41
Several sources likely contribute to PCMs in urban surface waters: (1) entry via storm water, (2) direct discharge into tributaries due to illegal sanitary sewer cross connections, and (3) WWTP discharges. The first explanation (1) involves PCM scavenged from air by precipitation and wash-off of atmospherically deposited PCMs to films on impervious surfaces.88 By far the highest concentration seen at one urban site (Don River) was likely due to releases from an upstream WWTP.
Concentrations of PCMs in WWTP effluent are given in Table S13.† The median Σ6PCMs concentrations in WWTP effluents were one order of magnitude higher than those in the tributary surface water (Table 2). Within WWTPs, the highest Σ6PCM concentration was in Plant 2 (median 3300 ng L−1; 1600–11500 ng L−1), which is the largest of the three WWTPs, serving a population of approximately 1,524,000 as well as numerous commercial and some industrial facilities. HHCB and AHTN were the most abundant SMCs found in WWTP effluents, as reported previously.53
Table 2 Σ6PCMs (i.e., DPMI, ADBI, AHMI, ATII, HHCB, AHTN) in effluents of WWTPs
|
Plant 1 (n = 4) |
Plant 2 (n = 6) |
Plant 3 (n = 7) |
Population served |
450 000 |
1 524 000 |
651 000 |
![[thin space (1/6-em)]](https://www.rsc.org/images/entities/char_2009.gif) |
Σ
6
PCMs (ng L
−1
)
|
Median |
2100 |
3300 |
1800 |
Standard deviation |
430 |
3900 |
2500 |
Minimum |
1700 |
1600 |
860 |
Maximum |
2600 |
11 500 |
8200 |
Median concentrations of HHCB in the effluents of the three plants ranged from 1000–1800 ng L−1 and for AHTN ranged from 140–270 ng L−1, which were comparable with effluents from other WWTPs in Canada89,90 but much higher than one WWTP located in Peterborough, Canada.82 These variations were likely due to differences in inputs within the sewer-sheds, facility capacities, and retention times, in addition to differences in contributions from storm water to WWTP.
The half-life of HHCB in experimental lake water due to photolysis is about 25 times longer than for AHTN (109 vs. 4 h) and hence a higher HHCB/AHTN may indicate an aged source.91 The HHCB/AHTN ratios in the urban tributary surface waters (2.6–5.9) were not significantly different from those at the rural sites (1.2–4), with one exception for Humber River Midstream that showed a high ratio of 23, consistent with aging. In the WWTP effluents, the HHCB/AHTN ranged from 7.2–8.3, which was higher than those in the tributary surface waters but similar to those reported in water from Lake Ontario, with ratio of 6–9 measured in 2005 (ref. 92) and 7–12 measured in 2012.41 In water of the North Sea, the median (range) ratio of HHCB/AHTN of 2.0 (0.8–5.0) was reported,37 which is much lower than the ratio in our WWTP effluents.
Conclusions
We investigated the occurrence and potential sources of SMCs in the urban environment in Canada. Highest concentrations of SMCs were found in indoor air, and WWTP On-site air, and the levels of which were not significantly different. SMCs in ambient air and tributary surface water were positively correlated with population density. These concentrations varied by ∼3-fold and ∼20-fold between urban and rural sites for air and water, respectively. Our results indicated that WWTP and indoor environment are likely main sources of SMCs to the environment. SMCs were not found in remote Arctic air indicating low potential for long-range atmospheric transport. SMCs were also not found in soils, consistent with their high rates of biodegradation and relatively high volatility. In all media, the PCMs particularly, AHTN and HHCB dominated the composition of SMCs. WWTP On-site air, trace levels of the toxic nitro-musk, namely MX and MK were detected despite having been phased out since the late 1990's. MCMs accounted for ∼10% of the total SMCs measured. Although the long-range transport potential of these chemicals is low, the continuous release of SMCs in high volumes from the urban environment, notably wastewater treatment plants, could pose a chronic toxicological threat to biota in receiving waters. We noted that there are factors that may compromise the interpretation of our results. Such factors include the various sampling techniques, analytical approaches and sample extract storage and archiving conditions. However, our initial screening of SMCs in a wide-array of environmental media provided valuable baseline information for planning future research specifically built for these chemicals. Finally, we recognize that experimental physical–chemical properties of SMCs are scarce. Large discrepancies in the physical–chemical property values of SMCs were found in the literature (Tables 1 and S1†). Future studies focusing on the physical-chemical and toxicological properties of SMCs and their degradation products are recommended to improve our understanding of their environmental fate and risk to ecological and human health.
Conflicts of interest
There are no conflicts to declare.
Acknowledgements
Funding was provided by The Chemicals Management Plan through Environment and Climate Change Canada, The Great Lakes Atmospheric Deposition Fund, Ontario Ministry of the Environment, Conservations and Parks, Toronto and Region Conversation Authority, and the Natural Sciences and Engineering Research Council of Canada (NSERC), and the Czech Ministry of Education, Youth and Sports (LO2014). We thank Orrin Carson for air sample collection; Dave Supper for water and wastewater sample collection; Rob Brennan and Sheng Yang for water sample processing. Martin Shigeishi and plant operators provided access to City of Toronto wastewater treatment plants for sampling, and Matthew Caschera provided WWTP flow information. We thank Liisa Jantunen for comments and Ondřej Sáńka for map figures.
References
- H. Nakata, M. Hinosaka and H. Yanagimoto, Macrocyclic-, polycyclic-, and nitro musks in cosmetics, household commodities and indoor dusts collected from Japan: Implications for their human exposure, Ecotoxicol. Environ. Saf., 2015, 111, 248–255 CrossRef CAS PubMed.
- J. W. Tas, F. Balk, R. A. Ford and E. J. van de Plasschem, Environmental risk assessment of musk ketone and musk xylene in the Netherlands in accordance with the EU-TGD, Chemosphere, 1997, 35, 2973–3002 CrossRef CAS PubMed.
-
USEPA, Estimation Programs Interface Suite™ for Microsoft® Windows, v 4.11, United States Environmental Protection Agency, Washington, DC, USA, 2017 Search PubMed.
- T. Luckenbach and D. Epel, Nitromusk and polycyclic musk compounds as long-term inhibitors of cellular xenobiotic defense systems mediated by multidrug transporters, Environ. Health Perspect., 2005, 113, 17–24 CrossRef CAS PubMed.
- K. M. Taylor, M. Weisskopf and J. Shine, Human exposure to nitro musks and the evaluation of their potential toxicity: An overview, Environ. Health, 2014, 13, 14 CrossRef PubMed.
-
ECB, European Union Risk Assessment Report: 4′-tert-butyl-2′,6′-dimethyl-3′,5′-dinitroacetophenone (musk ketone), European Chemicals Bureau, Bilthoven, The Netherlands, http://echa.europa.eu/documents/10162/c870f3b6-a674-4589-9565-c7a771b5fb5d, accessed October 27, 2017 Search PubMed.
-
ECB, European Union Risk Assessment Report: 5-tert-butyl-2,4,6-trinitro-m-xylene (musk xylene), European Chemicals Bureau, Bilthoven, The Netherlands, http://echa.europa.eu/documents/10162/dc1a179e-699e-44c2-b4ad-371b9b89efab, accessed October 27, 2017 Search PubMed.
- E. Rappas-Chrysovitsinos, A. Sobet and M. MacLeod, Screening-level exposure-based prioritization to identify potential POPs, vPvBs and planetary boundary threats among Arctic contaminants, Emerg. Contam., 2017, 3, 85–94 CrossRef.
-
NITE, Chemical Risk Information Platform (CHRIP), National Institute of Technology and Evaluation, Tokyo, Japan, http://www.safe.nite.go.jp/english/sougou/view/SystemTop_en.faces, accessed October 27, 2017 Search PubMed.
-
European Commission, 2009. Cosmetics Regulation (EC) No 1223/2009 of the European Parliament and of the Council, Official Journal of the European Union, http://eur-lex.europa.eu/LexUriServ/LexUriServ.do?uri=OJ:L:2009:342:0059:0209:en:PDF, accessed April 22, 2018 Search PubMed.
-
ECHA, Information from the Existing Substances Regulation, European Chemicals Agency, Helsinki, Finland, http://echa.europa.eu/information-on-chemicals/information-from-existing-substances-regulation, accessed October 27, 2017 Search PubMed.
-
IFRA, International Fragrance Association – Standards Library, http://www.ifraorg.org/en-us/standards-library#.WfNIu8Zrypo, accessed October 27, 2017.
-
Health Canada, List of Ingredients that are Prohibited for Use in Cosmetic Products, https://www.canada.ca/en/health-canada/services/consumer-product-safety/cosmetics/cosmetic-ingredient-hotlist-prohibited-restricted-ingredients/hotlist.html, accessed October 27, 2017 Search PubMed.
-
Government of Canada, Managing substances in the environment, https://pollution-waste.canada.ca/substances-search/Substance/DisplaySubstanceDetails?Id=116-66-5, accessed October 27, 2017 Search PubMed.
-
USEPA, ChemView, https://chemview.epa.gov/chemview, accessed July 18, 2018 Search PubMed.
- USEPA, TSCA Work Plan Chemical Risk Assessment: HHCB, 1,3,4,6,7,8-Hexahydro-4,6,6,7,8,8-hexamethylcyclopenta-γ-2-benzopyran, CASRN: 1222-05-5. EPA Document#746-R1-4001, 2014.
-
OSPAR, OSPAR Background Document on Musk Xylene and Other Musks, Hazardous Substances Series, 2004 Search PubMed.
- D. B. D. Simmons, V. L. Marlatt, V. L. Trudeau, J. P. Sherry and C. D. Metcalfe, Interaction of Galaxolide® with the human and trout estrogen receptor-α, Sci. Total Environ., 2010, 408, 6158–6164 CrossRef CAS PubMed.
- R. H. M. M. Schreurs, J. Legler, E. Artola-Garicano, T. L. Sinnige, P. H. Lanser, W. Seinen and B. Van Der Burg,
In vitro and in vivo antiestrogenic effects of polycyclic musks in zebrafish, Environ. Sci. Technol., 2004, 38, 997–1002 CrossRef CAS PubMed.
- R. J. Witorsch and J. A. Thomas, Personal care products and endocrine disruption: a critical review of the literature, Crit. Rev. Toxicol., 2010, 40, 1–30 CrossRef CAS PubMed.
- B. van der Burg, R. Schreurs, S. van der Linden, W. Seinen, A. Brouwer and E. Sonneveld, Endocrine effects of polycyclic musks: do we smell a rat?, Int. J. Androl., 2008, 31, 188–193 CrossRef CAS PubMed.
- H. Fang, Y. Gao, H. Wang, H. Yin, G. Li and T. An, Photo-induced oxidative damage to dissolved free amino acids by the photosensitizer polycyclic musk tonalide: Transformation kinetics and mechanisms, Water Res., 2017, 115, 339–346 CrossRef CAS PubMed.
- Y. Gao, G. Li, S. Ma and T. An, Research progress and challenge of synthetic musks: from personal care, environment pollution to human health, Progr. Chem., 2017, 29, 1082–1092 Search PubMed.
- Y. Gao, Y. Ji, G. Li, B. Mai and T. An, Bioaccumulation and ecotoxicity increase during indirect photochemical transformation of polycyclic musk tonalide: a modeling study, Water Res., 2016, 105, 47–55 CrossRef CAS PubMed.
- V. Homem, J. A. Silva, N. Ratola, L. Santos and A. Alves, Prioritisation approach to score and rank synthetic musk compounds for environmental risk assessment, J. Chem. Technol. Biotechnol., 2015, 90, 1619–1630 CrossRef CAS.
- D. Salvito, T. Luckenbach and D. Epel, Synthetic musk compounds and effects on human health?, Environ. Health Perspect., 2005, 113, A802–A804 CrossRef PubMed.
- F. Balk and R. A. Ford, Environmental risk assessment for the polycyclic musks, AHTN and HHCB. II. Effect assessment and risk characterisation, Toxicol. Lett., 1999, 111, 81–94 CrossRef CAS PubMed.
- K. Kannan, J. L. Reiner, S. H. Yun, E. E. Perrotta, L. Tao, B. Johnson-Restrepo and B. D. Rodan, Polycyclic musk compounds in higher trophic level aquatic organisms and humans from the United States, Chemosphere, 2005, 61, 693–700 CrossRef CAS PubMed.
-
K. Bester, Personal Care Compounds in the Environment, VCH-Wiley, Weinheim, 2007 Search PubMed.
- H. Wang, J. Zhang, F. Gao, Y. Yang, H. Duan, Y. Wu, J.-D. Berset and B. Shao, Simultaneous analysis of synthetic musks and triclosan in human breast milk by gas chromatography tandem mass spectrometry, J. Chromatogr. B: Anal. Technol. Biomed. Life Sci., 2011, 879, 1861–1869 CrossRef CAS PubMed.
- M. Schlumpf, K. Kypke, M. Wittassek, J. Angerer, H. Mascher, D. Mascher, C. Vökt, M. Birchler and W. Lichtensteiger, Exposure patterns of UV filters, fragrances, parabens, phthalates, organochlor pesticides, PBDEs, and PCBs in human milk: correlation of UV filters with use of cosmetics, Chemosphere, 2010, 81, 1171–1183 CrossRef CAS PubMed.
- H. Fromme, T. Lahrz, M. Piloty, H. Gebhart, A. Oddoy and H. Rüden, Occurrence of phthalates and musk fragrances in indoor air and dust from apartments and kindergartens in Berlin (Germany), Indoor Air, 2004, 14, 188–195 CrossRef CAS PubMed.
- Y. Lu, T. Yuan, S. H. Yun, W. Wang and K. Kannan, Occurrence of synthetic musks in indoor dust from China and implications for human exposure, Arch. Environ. Contam. Toxicol., 2011, 60, 182–189 CrossRef CAS PubMed.
- J. Regueiro, M. Llompart, C. Garcia-Jares and R. Cela, Development of a high-throughput method for the determination of organochlorinated compounds, nitromusks and pyrethroid insecticides in indoor dust, J. Chromatogr. A, 2007, 1174, 112–124 CrossRef CAS PubMed.
- A. Sofuoglu, N. Kiymet, P. Kavcar and S. C. Sofuoglu, Polycyclic and nitro musks in indoor air: a primary school classroom and a women's sport center, Indoor Air, 2010, 20, 515–522 CrossRef CAS PubMed.
- J. Regueiro, C. Garcia-Jares, M. Llompart, J. P. Lamas and R. Cela, Development of a method based on sorbent trapping followed by solid-phase microextraction for the determination of synthetic musks in indoor air, J. Chromatogr. A, 2009, 1216, 2805–2815 CrossRef CAS PubMed.
- Z. Xie, R. Ebinghaus, C. Temme, O. Heemken and W. Ruck, Air-sea exchange fluxes of synthetic polycyclic musks in the North Sea and the Arctic, Environ. Sci. Technol., 2007, 41, 5654–5659 CrossRef CAS PubMed.
- A. M. Peck and K. C. Hornbuckle, Synthetic musk fragrances in Lake Michigan, Environ. Sci. Technol., 2004, 38, 367–372 CrossRef CAS PubMed.
- A. M. Peck and K. C. Hornbuckle, Synthetic musk fragrances in urban and rural air of Iowa and the Great Lakes, Atmos. Environ., 2006, 40, 6101–6111 CrossRef CAS.
- I. Weinberg, A. Dreyer and R. Ebinghaus, Waste water treatment plants as sources of polyfluorinated compounds, polybrominated diphenyl ethers and musk fragrances to ambient air, Environ. Pollut., 2011, 159, 125–132 CrossRef CAS PubMed.
- C. A. McDonough, P. A. Helm, D. Muir, G. Puggioni and R. Lohmann, Polycyclic musks in the air and water of the lower Great Lakes: spatial distribution and volatilization from surface waters, Environ. Sci. Technol., 2016, 50, 11575–11583 CrossRef CAS PubMed.
- L. Melymuk, M. Robson, S. A. Csiszar, P. A. Helm, G. Kaltenecker, S. Backus, L. Bradley, B. Gilbert, P. Blanchard, L. Jantunen and M. L. Diamond, From the city to the lake: loadings of PCBs, PBDEs, PAHs and PCMs from Toronto to Lake Ontario, Environ. Sci. Technol., 2014, 48, 3732–3741 CrossRef CAS PubMed.
- H. Nakata, H. Sasaki, A. Takemura, M. Yoshioka, S. Tanabe and K. Kannan, Bioaccumulation, temporal trend, and geographical distribution of synthetic musks in the marine environment, Environ. Sci. Technol., 2007, 41, 2216–2222 CrossRef CAS PubMed.
- H. Nakata, R. I. Shinohara, Y. Nakazawa, T. Isobe, A. Sudaryanto, A. Subramanian, S. Tanabe, M. P. Zakaria, G. J. Zheng, P. K. S. Lam, E. Y. Kim, B. Y. Min, S. U. We, P. H. Viet, T. S. Tana, M. Prudente, D. Frank, G. Lauenstein and K. Kannan, Asia-Pacific mussel watch for emerging pollutants: distribution of synthetic musks and benzotriazole UV stabilizers in Asian and US coastal waters, Mar. Pollut. Bull., 2012, 64, 2211–2218 CrossRef CAS PubMed.
- K. Kannan, J. L. Reiner, H. Y. Se, E. E. Perrotta, L. Tao, B. Johnson-Restrepo and B. D. Rodan, Polycyclic musk compounds in higher trophic level aquatic organisms and humans from the United States, Chemosphere, 2005, 61, 693–700 CrossRef CAS PubMed.
- A. Schiavone, K. Kannan, Y. Horii, S. Focardi and S. Corsolini, Occurrence of brominated flame retardants, polycyclic musks, and chlorinated naphthalenes in seal blubber from Antarctica: comparison to organochlorines, Mar. Pollut. Bull., 2009, 58, 1415–1419 CrossRef CAS PubMed.
- R. Kallenborn, R. Gatermann, T. Nygård, J. Knutzen and M. Schlabach, Synthetic musks in Norwegian marine fish samples collected in the vicinity of densely populated areas, Fresenius Environ. Bull., 2001, 10, 832–842 CAS.
- H. Rüdel, W. Böhmer and C. Schröter-Kermani, Retrospective monitoring of synthetic musk compounds in aquatic biota from German rivers and coastal areas, J. Environ. Monit., 2006, 8, 812–823 RSC.
- K. Bester, N. Hüffmeyer, E. Schaub and J. Klasmeier, Surface water concentrations of the fragrance compound OTNE in Germany – a comparison between data from measurements and models, Chemosphere, 2008, 73, 1366–1372 CrossRef CAS PubMed.
- I. S. Lee, U. J. Kim, J. E. Oh, M. Choi and D. W. Hwang, Comprehensive monitoring of synthetic musk compounds from freshwater to coastal environments in Korea: with consideration of ecological concerns and bioaccumulation, Sci. Total Environ., 2014, 470–471, 1502–1508 CrossRef CAS PubMed.
- S. Villa, L. Assi, A. Ippolito, P. Bonfanti and A. Finizio, First evidences of the occurrence of polycyclic synthetic musk fragrances in surface water systems in Italy: spatial and temporal trends in the Molgora River (Lombardia Region, Northern Italy), Sci. Total Environ., 2012, 416, 137–141 CrossRef CAS PubMed.
- A. M. Peck, E. K. Linebaugh and K. C. Hornbuckle, Synthetic musk fragrances in Lake Erie and Lake Ontario sediment cores, Environ. Sci. Technol., 2006, 40, 5629–5635 CrossRef CAS PubMed.
- V. Homem, J. A. Silva, N. Ratola, L. Santos and A. Alves, Long lasting perfume: a review of synthetic musks in WWTPs, J. Environ. Manage., 2015, 149, 168–192 CrossRef PubMed.
- Y. Horii, J. L. Reiner, B. G. Loganathan, K. Senthil Kumar, K. Sajwan and K. Kannan, Occurrence and fate of polycyclic musks in wastewater treatment plants in Kentucky and Georgia, USA, Chemosphere, 2007, 68, 2011–2020 CrossRef CAS PubMed.
- N. Upadhyay, Q. Sun, J. O. Allen, P. Westerhoff and P. Herckes, Synthetic musk emissions from wastewater aeration basins, Water Res., 2011, 45, 1071–1078 CrossRef CAS PubMed.
- W. D. Wombacher and K. C. Hornbuckle, Synthetic musk fragrances in a conventional drinking water treatment plant with lime softening, J. Environ. Eng., 2009, 135, 1192–1198 CrossRef CAS PubMed.
- A. Schiavone, K. Kannan, Y. Horii, S. Focardi and S. Corsolini, Polybrominated diphenyl ethers, polychlorinated naphthalenes and polycyclic musks in human fat from Italy: comparison to polychlorinated biphenyls and organochlorine pesticides, Environ. Pollut., 2010, 158, 599–606 CrossRef CAS PubMed.
- S. Müller, P. Schmid and C. Schlatter, Occurrence of nitro and non-nitro benzenoid musk compounds in human adipose tissue, Chemosphere, 1996, 33, 17–28 CrossRef.
- C. S. Kang, J. H. Lee, S. K. Kim, K. T. Lee, J. S. Lee, P. S. Park, S. H. Yun, K. Kannan, Y. W. Yoo, J. Y. Ha and S. W. Lee, Polybrominated diphenyl ethers and synthetic musks in umbilical cord serum, maternal serum, and breast milk from Seoul, South Korea, Chemosphere, 2010, 80, 116–122 CrossRef CAS PubMed.
- S. Lignell, P. O. Darnerud, M. Aune, S. Cnattingius, J. Hajslova, L. Setkova and A. Glynn, Temporal trends of synthetic musk compounds in mother's milk and associations with personal use of perfumed products, Environ. Sci. Technol., 2008, 42, 6743–6748 CrossRef CAS PubMed.
- S. Lee, S. Kim, J. Park, H. J. Kim, J. Jae Lee, G. Choi, S. Choi, S. Young Kim, K. Choi and H. B. Moon, Synthetic musk compounds and benzotriazole ultraviolet stabilizers in breast milk: occurrence, time-course variation and infant health risk, Environ. Res., 2015, 140, 466–473 CrossRef CAS PubMed.
- Z. Hu, Y. Shi, H. Niu, Y. Cai, G. Jiang and Y. Wu, Occurrence of synthetic musk fragrances in human blood from 11 cities in China, Environ. Toxicol. Chem., 2010, 29, 1877–1882 CrossRef CAS PubMed.
- S. Villa, M. Vighi and A. Finizio, Theoretical and experimental evidences of medium range atmospheric transport processes of polycyclic musk fragrances, Sci. Total Environ., 2014, 481, 27–34 CrossRef CAS PubMed.
- S. M. Aschmann, J. Arey, R. Atkinson and S. L. Simonich, Atmospheric lifetimes and fates of selected fragrance materials and volatile model compounds, Environ. Sci. Technol., 2001, 35, 3595–3600 CrossRef CAS PubMed.
-
K. Vorkamp, M. Dam, F. Riget, P. Fauser, R. Bossi and A. B. Hansen, Screening of “new” contaminants in the marine environment of Greenland and the Faroe Islands, National Environmental Research Institute, Denmark, NERI Technical Report No. 525, 2004 Search PubMed.
- S. Harrad and M. L. Diamond, Exposure to polybrominated diphenyl ethers (PBDEs) and polychlorinated diphenyls (PCBs): current and future scenarios, Atmos. Environ., 2006, 40, 1187–1188 CrossRef CAS.
- X. Zhang, M. L. Diamond, M. Robson and S. Harrad, Sources, emissions, and fate of polybrominated diphenyl ethers and polychlorinated biphenyls indoors in Toronto, Canada, Environ. Sci. Technol., 2011, 45, 3268–3274 CrossRef CAS PubMed.
- L. Melymuk, M. Robson, P. A. Helm and M. L. Diamond, Evaluation of passive air sampler calibrations: Selection of sampling rates and implications for the measurement of persistent organic pollutants in air, Atmos.
Environ., 2011, 45, 1867–1875 CrossRef CAS.
- L. Melymuk, M. Robson, P. A. Helm and M. L. Diamond, Application of land use regression to identify sources and assess spatial variation in urban SVOC concentrations, Environ. Sci. Technol., 2013, 47, 1887–1895 CrossRef CAS PubMed.
- L. Melymuk, M. Robson, P. A. Helm and M. L. Diamond, PCBs, PBDEs, and PAHs in Toronto air: spatial and seasonal trends and implications for contaminant transport, Sci. Total Environ., 2012, 429, 272–280 CrossRef CAS PubMed.
- M. Shoeib, J. Schuster, C. Rauert, K. Su, S. A. Smyth and T. Harner, Emission of poly and perfluoroalkyl substances, UV-filters and siloxanes to air from wastewater treatment plants, Environ. Pollut., 2016, 218, 595–604 CrossRef CAS PubMed.
- L. Ahrens, T. Harner, M. Shoeib, M. Koblizkova and E. J. Reiner, Characterization of two passive air samplers for per- and polyfluoroalkyl substances, Environ. Sci. Technol., 2013, 47, 14024–14033 CrossRef CAS PubMed.
- A. Abdollahi, A. Eng, L. M. Jantunen, L. Ahrens, M. Shoeib, J. M. Parnis and T. Harner, Characterization of polyurethane foam (PUF) and sorbent impregnated PUF (SIP) disk passive air samplers for measuring organophosphate flame retardants, Chemosphere, 2017, 167, 212–219 CrossRef CAS PubMed.
- C. Shunthirasingham, A. Gawor, H. Hung, K. A. Brice, K. Su, N. Alexandrou, H. Dryfhout-Clark, S. Backus, E. Sverko, C. Shin, R. Park and R. Noronha, Atmospheric concentrations and loadings of organochlorine pesticides and polychlorinated biphenyls in the Canadian Great Lakes Basin (GLB): spatial and temporal analysis (1992–2012), Environ. Pollut., 2016, 217, 124–133 CrossRef CAS PubMed.
- F. Wong, M. Shoeib, A. Katsoyiannis, S. Eckhardt, A. Stohl, P. Bohlin-Nizzetto, H. Li, P. Fellin, Y. Su and H. Hung, Assessing temporal trends and source regions of per- and polyfluoroalkyl substances (PFASs) in air under the Arctic Monitoring and Assessment Programme (AMAP), Atmos. Environ., 2018, 172, 65–73 CrossRef CAS.
- S. Genualdi, S. C. Lee, M. Shoeib, A. Gawor, L. Ahrens and T. Harner, Global pilot study of legacy and emerging persistent organic pollutants using sorbent-impregnated polyurethane foam disk passive air samplers, Environ. Sci. Technol., 2010, 44, 5534–5539 CrossRef CAS PubMed.
- S. J. Hayward, T. Gouin and F. Wania, Comparison of Four Active and Passive Sampling Techniques for Pesticides in Air, Environ. Sci. Technol., 2010, 44, 3410–3416 CrossRef CAS PubMed.
- J. Paasivirta, S. Sinkkonen, A. L. Rantalainen, D. Broman and Y. Zebühr, Temperature dependent properties of environmentally important synthetic musks, Environ. Sci. Pollut. Res., 2002, 9, 345–355 CrossRef CAS PubMed.
-
F. Wong, C. Shunthirasingham, N. Alexandrou, A. Pajda, J. Okeme, T. Rodgers, L. M. Jantunen and H. Hung, Atmospheric concentrations of synthetic musks in Canada, 37th International Symposium on Halogenated Persistent Organic Pollutants (POPs) – DIOXIN, Vancouver, Canada, August 20–25, 2017, http://www.dioxin2017.org/uploadfiles/others/9938_0515081222.pdf Search PubMed.
- F. Balk and R. A. Ford, Environmental risk assessment for the polycyclic musks AHTN and HHCB in the EU. I. Fate and exposure assessment, Toxicol. Lett., 1999, 111, 57–79 CrossRef CAS PubMed.
- H. Lee, H. J. Kim and J. H. Kwon, Determination of Henry's law constant using diffusion in air and water boundary layers, J. Chem. Eng. Data, 2012, 57, 3296–3302 CrossRef CAS.
- J. J. Yang and C. D. Metcalfe, Fate of synthetic musks in a domestic wastewater treatment plant and in an agricultural field amended with biosolids, Sci. Total Environ., 2006, 363, 149–165 CrossRef CAS PubMed.
- A. M. DiFrancesco, P. C. Chiu, L. J. Standley, H. E. Allen and D. T. Salvito, Dissipation of fragrance materials in sludge-amended
soils, Environ. Sci. Technol., 2004, 38, 194–201 CrossRef CAS PubMed.
- N. Ramírez, R. M. Marcé and F. Borrull, Development of a thermal desorption-gas chromatography-mass spectrometry method for determining personal care products in air, J. Chromatogr. A, 2010, 1217, 4430–4438 CrossRef PubMed.
- R. Kallenborn, R. Gatermann, S. Planting, G. G. Rimkus, M. Lund, M. Schlabach and I. C. Burkow, Gas chromatographic determination of synthetic musk compounds in Norwegian air samples, J. Chromatogr. A, 1999, 846, 295–306 CrossRef CAS.
- M. Winkler, G. Kopf, C. Hauptvogel and T. Neu, Fate of artificial musk fragrances associated with suspended particulate matter (SPM) from the River Elbe (Germany) in comparison to other organic contaminants, Chemosphere, 1998, 37, 1139–1156 CrossRef CAS.
- H. D. Eschke, H. J. Dibowski and J. Traud, Unter-suchungen zum Vorkommen polycyclischer Moschus-Duft-stoffe in verschiedenen Umwelkompartimenten, 2. Mitteilung: Befunde in Oberflachen-, Abwassern und Fis-chen sowie in Waschmitteln und Kosmetika, UWSF-Z, Umweltchem. Okotoxikol, 1994, 7, 131–138 CrossRef.
-
M. L. Diamond and L. Thidobeaux, Handbook of Mass Transfer Coefficients, ed. L. Thibodeaux and D. Mackay, Taylor & Francis, 2010 Search PubMed.
- L. Lishman, S. A. Smyth, K. Sarafin, S. Kleywegt, J. Toito, T. Peart, B. Lee, M. Servos, M. Beland and P. Seto, Occurrence and reductions of pharmaceuticals and personal care products and estrogens by municipal wastewater treatment plants in Ontario, Canada, Sci. Total Environ., 2006, 367, 544–558 CrossRef CAS PubMed.
- S. A. Smyth, L. A. Lishman, E. A. McBean, S. Kleywegt, J. J. Yang, M. L. Svoboda, S. Ormonde, V. Pileggi, H. B. Lee and P. Seto, Polycyclic and nitro musks in Canadian municipal wastewater: occurrence and removal in wastewater treatment, Water Qual. Res. J. Can., 2007, 42, 138–152 CrossRef CAS.
- I. J. Buerge, H. R. Buser, M. D. Müller and T. Poiger, Behavior of the polycyclic musks HHCB and AHTN in lakes, two potential anthropogenic markers for domestic wastewater in surface waters, Environ. Sci. Technol., 2003, 37, 5636–5644 CrossRef CAS PubMed.
- J. A. Andresen, D. Muir, D. Ueno, C. Darling, N. Theobald and K. Bester, Emerging pollutants in the North Sea in comparison to Lake Ontario, Canada, data, Environ. Toxicol. Chem., 2007, 26, 1081–1089 CrossRef CAS PubMed.
Footnotes |
† Electronic supplementary information (ESI) available. See DOI: 10.1039/c8em00341f |
‡ Department of Earth Sciences, University of Toronto, Toronto, Ontario, M5S 3B1, Canada. |
§ Department of Chemical Engineering & Applied Chemistry, University of Toronto, Toronto, Ontario, M5S 3E5, Canada. |
|
This journal is © The Royal Society of Chemistry 2019 |
Click here to see how this site uses Cookies. View our privacy policy here.