Effects of geochemical conditions, surface modification, and arsenic (As) loadings on As release from As-loaded nano zero-valent iron in simulated groundwater†
Received
25th October 2018
, Accepted 20th November 2018
First published on 21st November 2018
Abstract
Arsenic (As) released from As-loaded nano zero-valent iron (nZVI) after As(V) remediation could become an environmental hazard, thus deserving in-depth investigations. This study investigated As release from As-loaded nZVI under different geochemical conditions (i.e., pH, Ca2+, and humic acid (HA) concentrations), as well as the effects of nZVI surface modification (chitosan and polyaniline) and As loadings (low and high) on As release. Alkaline condition (pH = 9) and the presence of humic acid were found to enhance As release from all the types of nZVI samples. Ca2+ had no effect on As release for all the types of nZVI at both As loadings, except for chitosan- and polyaniline-modified nZVI at high As loading when Ca2+ concentrations increased up to 16 mg L−1. At low As loading, surface modification had a limited impact on As release from As-loaded nZVI. When As loading was high, a higher percentage of As was released from pristine nZVI than those from both the types of modified nZVI. This is because pristine nZVI either formed only monodentate complexes or had a higher ratio of As(III)/As(V). Under the same geochemical conditions, As released from all the types of As-loaded nZVI at high As loadings was lower than that at low As loadings. This is due to the formation of bidentate complexes with stronger bindings and lower ratios of As(III)/As(V) for high As loadings. These findings provide a fundamental understanding regarding the environmental behaviors of As-loaded nZVI under natural conditions and the risk associated with the release of As from As-loaded nZVI.
Water impact
The fate of arsenic (As) on nZVI in groundwater has received no attention. This work has determined that low pH and low humic acid concentration, as well as surface modification and high As loading, decreased the release of As. This facilitates the assessment of potential risks and guides the selections of surface modification and nZVI dosage in relation to the released As in groundwater.
|
1. Introduction
Nano zero-valent iron (nZVI) has been widely employed for efficiently remediating As(V)-contaminated groundwater.1,2 During the remediation process, As(V) is sorbed onto nZVI, forming As-loaded nZVI, which is subsequently reduced to As(III).3,4 As(III) is more mobile5 and can be easily released from As-loaded nZVI into the environment, causing secondary pollution.6 This is a major concern for applications of nZVI that requires additional attention.
Sorption/desorption is a dynamic process,7,8 which is affected by the groundwater geochemical conditions. In groundwater, these geochemical conditions include pH, calcium, and humic substances; their effects on the interactions between nZVI and As could be significant.2,9 First, previous studies have reported the formation of bidentate complexes with stronger As–Fe bindings under acidic conditions and monodentate complexes with weaker bindings under alkaline conditions.10,11 Weaker As–Fe binding may cause As to be released more easily from As-loaded nZVI (than stronger binding). Therefore, it is suspected that the amount of As release may be affected by pH. Second, calcium (Ca2+) may also affect the As release. Ca2+ has been reported to increase As removal by promoting the formation of CaCO3 as a nucleation seed for the growth of surface corrosion products12 and even in the coprecipitation of calcium–iron–As(V).13 When loaded with As, the surface of nZVI becomes corroded and forms iron (oxy)hydroxides. If Ca2+ could still be a precursor for CaCO3 formation, the formation of iron (oxy)hydroxides might be enhanced, thereby increasing the surface adsorption sites. This increase would affect the As release. However, the effect of Ca2+ on As release from As-loaded nZVI is unclear, and therefore, needs to be investigated. Finally, humic acid (HA)—a typical representative of humic substances—is not only able to form complexes with dissolved As ions, but can also be adsorbed, thereby competing for sorption sites on the nZVI surface.14,15 As a result, the dissolved and adsorbed forms of HA may promote the desorption of As from the As-loaded nZVI and eventually affect the release of As. In the light of the above data, pH, Ca2+, and HA concentrations were selected as typical factors for representing geochemical conditions in order to investigate their impact on As release from As-loaded nZVI.
Recently, various surface modifiers, including chitosan,16,17 polyaniline (PANI),18,19 starch,20 and Tween 20 (ref. 21) have been investigated. Among them, chitosan and PANI can also enhance the reactivity of surface-modified nZVI.18,22 For example, as compared to pristine nZVI (107 mg g−1),10 both chitosan- and PANI-modified nZVI have shown higher As(V) adsorption capacities (119 mg g−1 and 227 mg g−1, respectively). Moreover, as compared to pristine nZVI, surface-modified nZVI reduced a lesser amount of As(V) to As(III).18,22 This is because the polymers that covered the nZVI surface decreased the reactive surface between nZVI and As,23 thereby affecting the amount of As released from As-loaded nZVI. Up until now, however, the effect of surface modification on this release pattern has not been investigated.
In addition, for the field-scale remediation of As(V)-contaminated groundwater, the amount of injected nZVI can be overdosed or underdosed relative to a certain level of As(V) in the groundwater. This leads to different amounts of As loaded onto the nZVI surface. Since different As loadings lead to the formation of different types of surface complexes (such as monodentate and bidentate complexes) with different binding strengths of the Fe–As bond,11 the adsorbed As would have different abilities to release from As-loaded nZVI. Therefore, it is necessary to consider the amount of adsorbed As by nZVI.
In order to provide a more fundamental understanding of the environmental behaviors of As-loaded nZVI under natural conditions, the objectives of this study were to investigate the following: (1) As release from As-loaded nZVI under different geochemical conditions (such as pH, calcium ion, and HA concentrations); (2) the effect of surface modification on As release; and (3) the effect of the amount of adsorbed As(V) on nZVI (such as low and high As loadings) on the As release. This study can facilitate the assessment of the potential risk caused by the released As from the As-loaded nZVI under different geochemical conditions and provide guidelines for the selections of surface modification and nZVI dosage with regard to the As release in groundwater.
2. Materials and methods
2.1. Reagents
FeCl3·6H2O, NaBH4, As2O5, NaCl, CaCl2, NaHCO3, Na2SO4, NaOH, HCl, HNO3, chitosan, acetic acid, aniline, and HA were of the analytic grade obtained from Aldrich Chemical Ltd. The stock solution of As(V) was prepared by dissolving As2O5 into 0.1 M NaOH solution, and the solution pH was adjusted to 7 ± 0.1. As(V) solutions at different concentrations were obtained by diluting the stock solution. The groundwater was artificially prepared by dissolving 0.2925 g NaCl, 0.0888 g CaCl2, 0.252 g NaHCO3, and 0.142 g Na2SO4 in 1 L of ultrapure water to mimic natural groundwater.10
2.2. nZVI synthesis and characterization
In this study, three types of nZVI (pristine nZVI and chitosan- and PANI-modified nZVI) were synthesized according to previous methods.10,18,24 Detailed synthesis procedures are elaborated in the ESI.†
The above three types of freshly prepared nZVI were characterized by using transmission electron microscopy (TEM, JEOL 2010 TEM), X-ray diffraction (XRD, PW-1830, Philips), and Fourier-transform infrared spectrometry with a transmission mode (FTIR, Vertex 70 Hyperion 1000, Bruker) for analyzing the surface morphologies and particle size, surface crystallinity, and surface functional groups and structures of nZVI. The surface charge of the three types of nZVI over the pH range of 4–10 was measured using a zeta potential analyzer (Zetaplus, LaborScience S.A.).
2.3. As release from As-loaded nZVI
Before conducting the experiment involving As release, As adsorption experiments (kinetics and isotherms) were conducted to obtain the As-loaded nZVI. Then, the kinetics of the As release was also investigated to determine the equilibrium time of the As release from the three types of As-loaded nZVI. The procedures are elaborated in the ESI.†
To investigate the effects of geochemical conditions (pH, Ca2+, and HA) on the As release, batch experiments (set no. 1 to 11; Table 1) were conducted in synthetic groundwater. Different solution pH values (5, 7, and 9) were selected as acidic, neutral, and alkaline conditions (set no. 1, 2, and 3), with Ca2+ and HA concentrations of 32 mg L−1 and 0 mg L−1, respectively. For set no. 4 to 7, various Ca2+ concentrations (0 mg L−1, 16 mg L−1, 32 mg L−1, and 64 mg L−1) were introduced to examine the Ca2+ effect on As release from the As-loaded nZVI at pH 7 and HA concentration of 0 mg L−1. The concentrations of NaCl were adjusted to maintain the ion strength as a constant (equivalent to 13.4 mM NaCl) in the solutions. Similarly, different HA concentrations (0, 5, 10, and 20 mg L−1) were introduced into set no. 8 to 11 at pH 7 and Ca2+ concentration of 32 mg L−1. The As-loaded nZVI was added in the above solutions to 41 mL vials, which were subsequently shaken in an end-over-end rotator at 26 rpm at room temperature (23 °C) for 18 h. Thereafter, the supernatants were collected from the vials by filtration and then diluted and acidified for As measurements using a graphite furnace atomic absorption spectrophotometer (GF-AAS) (Hitachi, Z-8200, Japan).25,26 HA concentrations in the supernatants were measured in terms of the dissolved organic carbon (DOC) using a TOC analyzer (Shimadzu Corporation, TOC-V CPH, Japan). All the tests were run in triplicate.
Table 1 Batch study settings and compositions of synthetic groundwater used in the batch study experiments
Batch experiments (set no.) |
pH |
CaCl2 (mg L−1) |
HA (mg L−1) |
NaCl (mg L−1) |
NaHCO3 (mg L−1) |
Na2SO4 (mg L−1) |
1 |
5 |
32 |
0 |
293 |
252 |
132 |
2 |
7 |
32 |
0 |
293 |
252 |
132 |
3 |
9 |
32 |
0 |
293 |
252 |
132 |
4 |
7 |
0 |
0 |
433 |
252 |
132 |
5 |
7 |
16 |
0 |
363 |
252 |
132 |
6 |
7 |
32 |
0 |
293 |
252 |
132 |
7 |
7 |
64 |
0 |
152 |
252 |
132 |
8 |
7 |
32 |
0 |
293 |
252 |
132 |
9 |
7 |
32 |
5 |
293 |
252 |
132 |
10 |
7 |
32 |
10 |
293 |
252 |
132 |
11 |
7 |
32 |
20 |
293 |
252 |
132 |
2.4. Characterizations of As-loaded nZVI
Before and after the As release, solids were collected by filtration from the solution and then freeze-dried in a vacuum for 48 h. Then, the freeze-dried solids were used for further analyses. FTIR spectrometry with the transmission mode (Vertex 70 Hyperion 1000, Bruker) was used to analyze the functional groups of nZVI and the formation of complexes between As and nZVI. XRD (PW-1830, Philips) was employed to analyze the crystal phase of the nZVI surface. XRD experiments were carried out using a powder X-ray diffractometer with a 2 kW rotating anode Cu-Kα X-ray source and a diffracted beam graphite monochromator. The data were collected in the angular range of 10–80° (2θ) in the continuous mode with an integrated step scan of 0.02° (2θ) and 4 s per step. X-ray photoelectron spectroscopy (XPS, PHI 5600, Physical Electronics) was employed to analyze the valence state of the surface chemical species. XPS experiments were carried out under ultrahigh vacuum conditions, so that there would be no surface oxidation during the measurements. The ratio of As(III)/As(V) on the surface of the As-loaded nZVI was calculated by XPS PEAK 41 software.
3. Results and discussion
3.1. Characteristics of nZVI without As loading
TEM images show the particle morphologies of the three types of nZVI. The particles exhibited spherical shapes with a mean size of 48 nm for pristine nZVI (Fig. 1a), 81 nm for chitosan-modified nZVI (Fig. 1b), and 124 nm for PANI-modified nZVI (Fig. 1c). The EDS elemental mapping images show the existence of elements such as C and N on the surface of the modified nZVI, indicating that nZVI was successfully modified by chitosan and PANI. This is also supported by the FTIR analysis (Fig. 1d), which shows that the bonds ascribed to chitosan (C–O, C–N, and N–H bonds) and PANI (benzenoid–NH, N–H bond, and C–N bond) are detected for chitosan- and PANI-modified nZVI. The pH of the zero point charge, pHzpc, for pristine and chitosan- and PANI-modified nZVI are 7.6, 4.9, and 5.5, respectively (Fig. 1e). This is because as compared to pristine nZVI, both the types of modified nZVI surfaces are more negatively charged due to the presence of negatively charged groups (e.g., hydroxyl, quinonoid, and benzenoid rings). Therefore, the pHzpc values for both the types of modified nZVI are lower than that of pristine nZVI. The XRD analysis (Fig. 1f) shows that all the types of nZVI are mainly composed of Fe0, confirming the successful synthesis of nZVI.
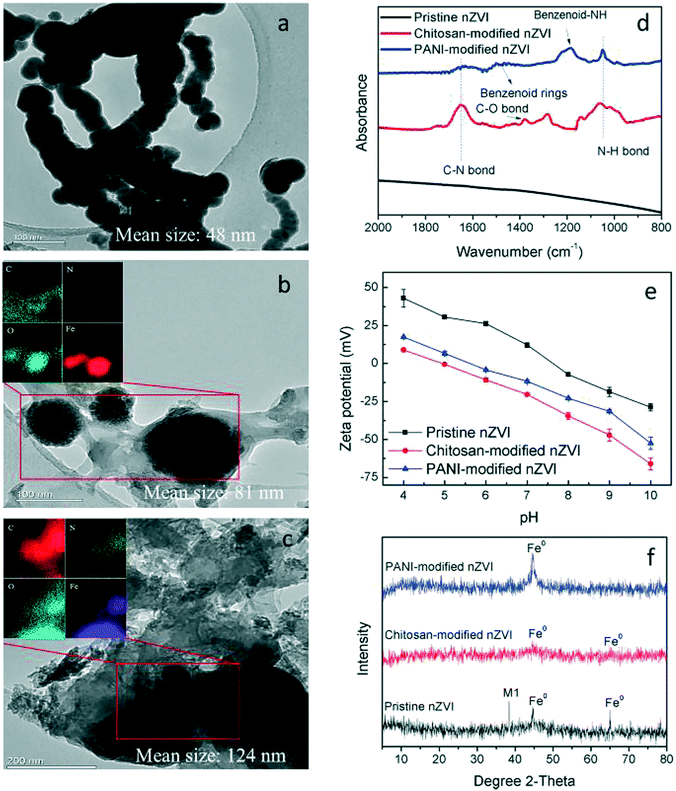 |
| Fig. 1 TEM images of (a) pristine nZVI, (b) chitosan-modified nZVI, and (c) PANI-modified nZVI; FITR spectra (d), zeta potential measurements (e), and XRD patterns (f) of the three types of fresh nZVI. Inset shows the EDS elemental mapping images, revealing the elemental information in the selected areas of the chitosan- and PANI-modified nZVI. The marked peaks in the XRD patterns are referred to as maghemite (M1) and nZVI (Fe0). | |
3.2. Effects of geochemical conditions on As release
3.2.1. pH.
As the pH increases from 5 to 7, As release from the three types of nZVI at low As loading shows only marginal changes, but increases when the pH increases to 9 (Fig. 2a). This increase can be related to the interactions between As and nZVI at different pH values. For all the types of nZVI samples at low As loading, the peaks of the monodentate complexes were found at pH = 5 and 7, but were not found at pH = 9 (Fig. 3). Previous studies have suggested the formation of stronger As–Fe bidentate complexes at pH = 5 and the formation of weaker monodentate complexes at pH = 9.10,27 Furthermore, a previous study has reported the mechanisms for the formation of complexes (namely, monodentate and bidentate) via ligand exchange reactions between As and goethite,28 and these complexation reactions were favored by single coordinated –OH groups between As oxyanions and goethite.29 Here, the dissociation constants for As oxyanions are as follows: pKa1 = 2.3 (H2AsO4−), pKa2 = 6.8 (HAsO42−), and pKa3 = 11.6 (AsO43−).30 This indicates that between the pH values of 5 and 7, As exists as HAsO42−; when pH increases to 9, As exists mainly as AsO43−. Increasing the pH results in the hydrolysis of As oxyanions, which subsequently influences the complexation process by affecting the coordinated –OH group of the As species. Based on this, at pH = 5 and 7, the coordinated –OH group of HAsO42− could form monodentate complexes. As the pH increases to 9, AsO43− cannot form complexes because of the absence of an –OH group, leading to the absence of peaks at pH = 9. Consequently, the binding strength between the adsorbed As and nZVI is weaker, yielding higher percentages of As release at pH = 9 than those at pH = 5 and 7.
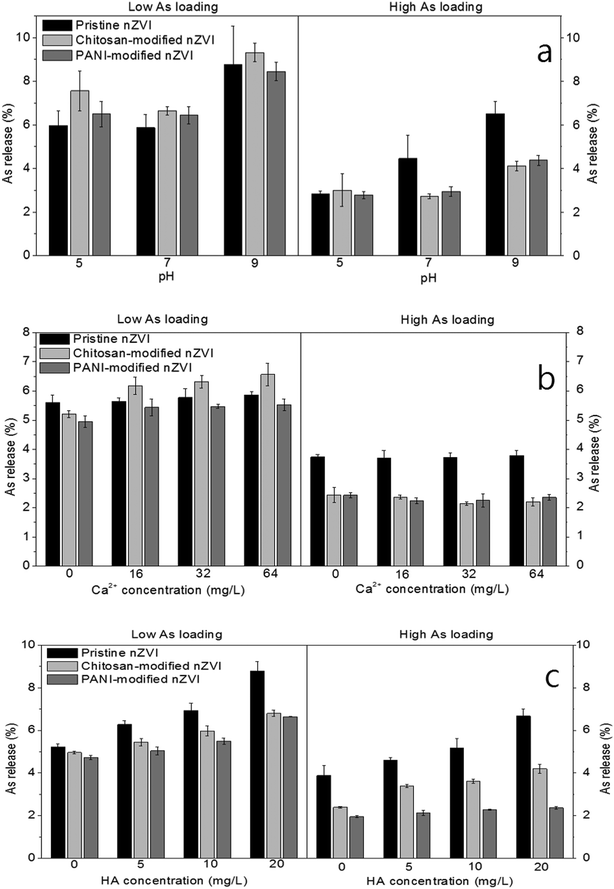 |
| Fig. 2 Influence of (a) pH, (b) Ca2+, and (c) HA on the As release (%) from the three types of As-loaded nZVI at low and high As loadings. | |
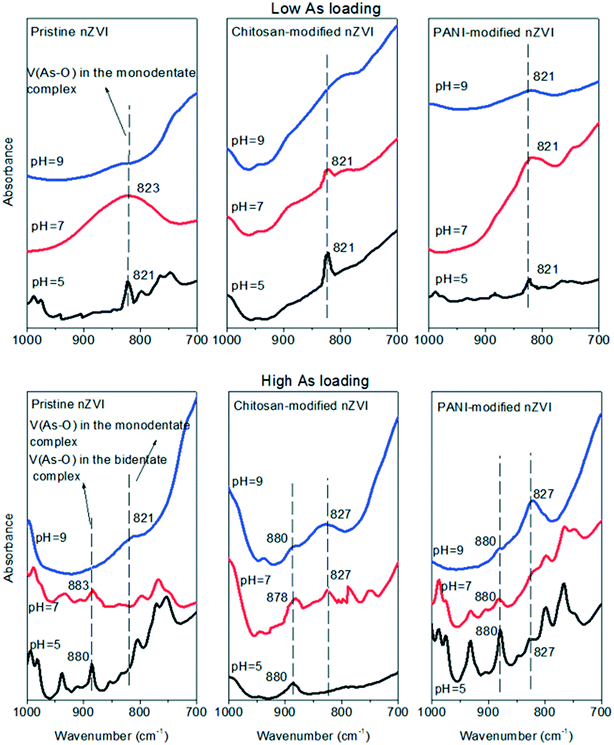 |
| Fig. 3 FTIR spectra of As-loaded pristine, chitosan-modified, and PANI-modified nZVI at low and high As loadings in synthetic groundwater with different pH values (5, 7, and 9). | |
At high As loading, an increase in the As release from pristine nZVI can also be found as the pH increased from 5 to 9 (Fig. 2a). This is because bidentate complexes were detected at both pH = 5 and 7 (Fig. 3); however, their peak intensities were weakened as the pH increased from 5 to 7, leading to a higher percentage of As release at pH = 7 (Fig. 2a). At pH = 9, only monodentate complexes were formed for pristine nZVI (Fig. 3); therefore, the percentage of As release was higher than that at pH = 5 and 7. For both the types of modified nZVI, bidentate complexes were detected at pH = 5 and 7, with the peak intensities showing insignificant changes. This accounts for the insignificant change in the As release when the pH increases from 5 to 7. Although bidentate complexes were detected at pH = 9, their peak intensities were weakened as compared to those at pH = 5 and 7 (Fig. 3), leading to a higher percentage of As release. Therefore, the alkaline condition promotes the As release from the three types of As-loaded nZVI at both low and high As loadings.
3.2.2. Ca2+.
Fig. 2b shows the percentages of As release from the three types of nZVI at low and high As loadings as a function of Ca2+ concentration. At low As loading, Ca2+ has an insignificant influence on As release from pristine nZVI, but has a discernible effect on As release from both the types of modified nZVI. In the absence of Ca2+, the percentages of As release from both the types of modified nZVI were lower than those in the presence of Ca2+. As the Ca2+ concentration increased from 0 to 16 mg L−1, the percentage of As release from both the types of modified nZVI increased, but showed insignificant changes when the Ca2+ concentration increased from 16 mg L−1 to 64 mg L−1. At high As loading, Ca2+ has an insignificant influence on the As release from all the types of nZVI (Fig. 2b).
The effect of Ca2+ on the interactions of As and nZVI was determined by FTIR analysis. From Fig. 4, it is evident that at low As loading, the formation of monodentate complexes of As and pristine nZVI can be observed, regardless of the Ca2+ concentration. The peak intensities did not change even if the Ca2+ concentration increased, supporting the insignificant changes in the As release from pristine nZVI at different Ca2+ concentrations. For chitosan- and PANI-modified nZVI, the peaks of monodentate complexes were also found in the absence of Ca2+. When Ca2+ was added, however, the peaks of the monodentate complexes of chitosan-modified nZVI were absent and the peak intensities of PANI-modified nZVI were weakened. This suggests that the presence of Ca2+ suppressed the formation of complexes for surface-modified nZVI, and hence, induced higher percentages of As release. Previous studies have shown that Ca2+ can be adsorbed and form complexes with surface sites (coordinated O(H) groups),31 inhibiting the formation of complexes of the adsorbed As- and surface-modified nZVI. Since both the types of modified nZVI were more negatively charged, more Ca2+ can be adsorbed by complexation with surface sites. Therefore, as compared to the absence of Ca2+, adding Ca2+ would inhibit or weaken the formation of complexes and then enhance As release from both the types of modified nZVI. As the Ca2+ concentration increased from 16 mg L−1 to 64 mg L−1, the peaks of the monodentate complexes of chitosan-modified nZVI were not detected, indicating insignificant differences in the As release at different Ca2+ concentrations. Although the peaks of monodentate complexes were found for PANI-modified nZVI, regardless of the Ca2+ concentration, their intensities showed insignificant changes as the Ca2+ concentration increased from 16 mg L−1 to 64 mg L−1. This suggests that Ca2+ concentration has a limited effect on the formation of monodentate complexes for PANI-modified nZVI. For all the types of nZVI at high As loadings, however, the peaks of both monodentate and bidentate complexes were detected, and their peak intensities did not change (Fig. 4), thereby inducing no changes in the percentages of the As release when the Ca2+ concentration increased from 0 mg L−1 to 64 mg L−1.
 |
| Fig. 4 FTIR spectra of As-loaded pristine nZVI, As-loaded chitosan-modified nZVI, and As-loaded PANI-modified nZVI at low and high As loadings in synthetic groundwater with different Ca2+ concentrations. | |
Moreover, our previous study has found that Ca2+ can be adsorbed onto hydrous ferric oxide, forming CaCO3 as a nucleation precursor for the growth of iron (hydr)oxide particles.32 This surface coverage could also coprecipitate As,33 which increases the As removal efficiency, subsequently impeding As release from As-loaded nZVI. In the present study, the XRD analyses show that CaCO3 and the coprecipitate of calcium iron arsenate were not detected for all the types of As-loaded nZVI (Fig. S4†). This indicates that after As loading, Ca2+ cannot be a precursor for CaCO3 formation. Then, the formation of iron (oxy)hydroxides and the adsorption sites did not increase. This result is consistent with the results of the effect of Ca2+ concentration on As release as well as the FTIR analyses.
3.2.3. HA.
The results of HA effect on As release from the three types of nZVI at low and high As loadings are shown in Fig. 2c. Evidently, the percentages of As release from the three types of nZVI at both As loadings increase as the concentration of HA increases from 0 mg L−1 to 20 mg L−1. A previous study has shown that HA can be easily adsorbed by nZVI and can compete with As(III) and As(V) for the occupation of the sorption sites.15 In the present study, the concentration of HA as DOC in solution after As release was measured, and the results are shown in Fig. 5. It is observed that HA was adsorbed onto three types of As-loaded nZVI at both As loadings. As the HA concentration increases from 0 mg L−1 to 20 mg L−1, the amount of adsorbed HA onto all the types of nZVI increases (Fig. 5). Therefore, As release was enhanced at higher HA concentrations.
 |
| Fig. 5 Amount of adsorbed HA on the three types of As-loaded nZVI at low and high As loadings at various HA concentrations. | |
To further understand how the adsorbed HA affects As release, the interactions of As and nZVI in the absence or presence of HA (20 mg L−1) were measured by FTIR analysis. As shown in Fig. 6, at both loadings, monodentate and bidentate complexes were detected at both HA concentrations of 0 and 20 mg L−1, but their peak intensities were weakened when the HA concentration increased. This suggested that an increased number of complexes of the adsorbed As and nZVI were formed at lower HA concentration, and the adsorbed HA (Fig. 5) suppressed the formation of the complexes of As and nZVI. Therefore, the adsorbed As could less easily release from As-loaded nZVI when the HA concentration is lower.
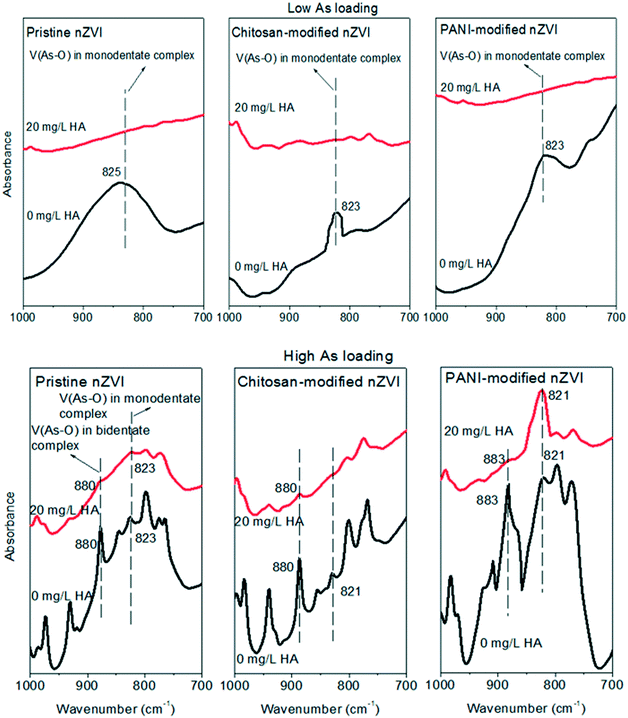 |
| Fig. 6 FTIR spectra of the three types of As-loaded nZVI at low and high As loadings in synthetic groundwater with HA concentrations of 0 mg L−1 and 20 mg L−1. | |
3.3. Effect of surface modification on As release
At the same pH, the percentages of As release from the three types of nZVI at low As loadings were comparable, which is similar to that for all the types of nZVI at high As loadings for pH = 5 (Fig. 2a). This is because under the same conditions, all the types of nZVI samples formed the same type of complexes (at pH = 5 and 7), i.e., monodentate complexes at low As loadings or bidentate complexes at high As loadings; complexes (at pH = 9) were not formed when the As loading was low. At pH = 7 and 9, the percentages of As release from pristine nZVI at high As loading were higher than both the types of modified nZVI (Fig. 2a). Since bidentate complexes were detected for three types of nZVI at high As loading, at pH = 7, the distribution of As speciation, which also influences the As–Fe binding strength, plays a role in As release. At pH = 7, the ratio of As(III)/As(V) of pristine nZVI was higher than those of both the types of modified nZVI at high As loadings, yielding a higher percentage of As release from As-loaded pristine nZVI (Table 2). At pH = 9, only monodentate complexes were detected for As-loaded pristine nZVI, while bidentate complexes were detected for both the types of modified nZVI (Fig. 3, high As loading). Moreover, the percentages of As release from both the types of modified nZVI were comparable at pH = 7 and 9 (Fig. 2a). This is because at high loadings, they formed the same type of complexes, i.e., bidentate complexes at pH = 7 and both monodentate and bidentate complexes at pH = 9 (Fig. 3).
Table 2 Calculated As(III)/As(V) ratios on the surface of the three types of nZVI before and after As release (determined by XPS analysis) at pH = 7, 32 mg L−1 Ca2+, and 0 mg L−1 HA
As loadings |
Before As release |
After As release |
Low |
High |
Low |
High |
Pristine nZVI |
3.65 : 1 |
0.44 : 1 |
1.51 : 1 |
0.25 : 1 |
Chitosan-modified nZVI |
1.18 : 1 |
0.27 : 1 |
0.26 : 1 |
0.11 : 1 |
PANI-modified nZVI |
1.11 : 1 |
0.22 : 1 |
0.25 : 1 |
0.09 : 1 |
In addition, from Fig. 2b, it is evident that as the Ca2+ concentration increases from 16 mg L−1 to 64 mg L−1, the percentages of As release from pristine and PANI-modified nZVI at low As loadings become comparable, and they are always lower than that of chitosan-modified nZVI at the same Ca2+ concentrations. This is due to the formation of monodentate complexes that were detected for pristine and PANI-modified nZVI, but no complexes were detected for chitosan-modified nZVI at low As loadings (Fig. 4). At high As loadings, the percentages of As release from pristine nZVI were constantly higher than both the types of modified nZVI at the same Ca2+ concentrations. This can be related to the distribution of As speciation. Table 2 shows that at high As loadings, pristine nZVI exhibits a larger decrease in the As(III)/As(V) ratio than both the types of modified nZVI before and after As release. The ratio of As(III)/As(V) for pristine nZVI decreased from 0.44
:
1 to 0.25
:
1, while that for chitosan-modified nZVI decreased from 0.27
:
1 to 0.11
:
1 and that for PANI-modified nZVI decreased from 0.22
:
1 to 0.09
:
1. Therefore, a higher decrease in the As(III)/As(V) ratio leads to a higher percentage of As release.
At various HA concentrations, the percentages of As release from pristine As-loaded nZVI at both the As loadings were constantly higher than those from the two types of modified nZVI (Fig. 2c). Kim et al. (2014) suggested that pristine nZVI surface exhibited more sorption sites toward HA than polymer-modified nZVI.34 In the present study, more HA was adsorbed onto pristine nZVI than that onto both the types of modified nZVI at both As loadings (Fig. 5). Since the adsorbed HA competes with As for the sorption sites,14 the percentages of As release from pristine nZVI were larger than those from both the types of modified nZVI (as shown in Fig. 2c). It is also observed that at both the As loadings, the percentage of As release from As-loaded chitosan-modified nZVI was marginally higher than that from As-loaded PANI-modified nZVI, irrespective of the HA concentration. Since the amount of HA adsorbed onto both the types of modified nZVI at both the As loadings were comparable (Fig. 5) and the peaks of the bidentate complexes were found for both the types of modified nZVI at the same level of HA (Fig. 6), this difference can be ascribed to the distribution of As speciation (shown in Table 2). After As release, As-loaded chitosan-modified nZVI experienced a slightly higher decrease in the As(III)/As(V) ratios at both the As loadings than those in PANI-modified nZVI. Given that both the types of modified nZVI were loaded with the same amount of As at either low or high As loadings, the higher decrease in the As(III)/As(V) ratio causes a larger percentage in As release. Therefore, at both As loadings, chitosan-modified nZVI released more As than PANI-modified nZVI.
3.4. Effect of As loadings on As release
As compared to As release at high As loadings, the percentages of As release from the three types of As-loaded nZVI at low As loading were much higher at the same pH (Fig. 2a). This is due to the formation of different types of complexes at different As loadings. From Fig. 3, it is evident that at low As loadings, monodentate complexes were formed for all the types of As-loaded nZVI at pH = 5 and 7, and no monodentate complexes were formed at pH = 9. While at high As loadings, bidentate complexes were formed at all the pH values, except for pristine nZVI in which only monodentate complexes were formed at pH = 9 (Fig. 3). At different Ca2+ and HA concentrations, the percentages of As release from the three types of nZVI at low As loadings were also higher than those at high As loadings (Fig. 2b and c). From Fig. 3, 4 and 6, it can be observed that either none or only monodentate complexes were detected at low As loadings, while both monodentate and bidentate complexes were detected at high As loadings. Therefore, adsorbed As can be much easily released from As-loaded nZVI at low As loadings, leading to a higher percentage of As release.
This result can also be supported by the distribution of As speciation on nZVI surfaces. Table 2 lists a higher ratio of As(III)/As(V) at low As loadings than that at high As loadings for all the types of nZVI, indicating that more As(V) was reduced to As(III). Since nZVI doses at both low and high As loadings were the same (150 mg L−1), the normalized amount of Fe0 (nZVI dose/amount of adsorbed As) available to reduce As(V) to As(III) was higher at low As loadings than that at high As loadings. Therefore, at low As loadings, monodentate complexes and a higher ratio of As(III)/As(V) lead to a higher percentage of As release from As-loaded nZVI.
4. Conclusions
The fate of As-loaded nZVI in groundwater should attract increased attention due to the possible secondary pollution from the released As. This study has determined that geochemical conditions (pH, Ca2+, and HA), surface modification, and As loading affect As release from As-loaded nZVI. The results enable the assessment of the potential risk caused by the released As from As-loaded nZVI under different geochemical conditions. For the remediation of As-contaminated groundwater, injected nZVI is recommended to be modified by polymers so that the ability of the adsorbed As to release will be weakened, and an appropriate dosage of nZVI injected into the As-contaminated groundwater zone (for producing higher As loadings) may be preferable in order to reduce the risk of As release.
Conflicts of interest
There are no conflicts to declare.
Acknowledgements
This research was supported by the National Natural Science Foundation of China (NSFC) and the Research Grants Council (RGC) of Hong Kong Joint Research Scheme (N_HKUST603/16).
References
- W. Liu, S. Tian, X. Zhao, W. Xie, Y. Gong and D. Zhao, Application of Stabilized Nanoparticles for In Situ Remediation of Metal-Contaminated Soil and Groundwater: a Critical Review, Curr. Pollut. Rep., 2015, 1, 280–291 CrossRef.
- Y. Zou, X. Wang, A. Khan, P. Wang, Y. Liu, A. Alsaedi, T. Hayat and X. Wang, Environmental Remediation and Application of Nanoscale Zero-Valent Iron and Its Composites for the Removal of Heavy Metal Ions: A Review, Environ. Sci. Technol., 2016, 50, 7290–7304 CrossRef CAS PubMed.
- X. Guan, Y. Sun, H. Qin, J. Li, I. M. Lo, D. He and H. Dong, The limitations of applying zero-valent iron technology in contaminants sequestration and the corresponding countermeasures: the development in zero-valent iron technology in the last two decades (1994-2014), Water Res., 2015, 75, 224–248 CrossRef CAS PubMed.
- L. Ling and W. X. Zhang, Visualizing Arsenate Reactions and Encapsulation in a Single Zero-Valent Iron Nanoparticle, Environ. Sci. Technol., 2017, 51, 2288–2294 CrossRef CAS PubMed.
- Y. Mamindy-Pajany, C. Hurel, N. Marmier and M. Roméo, Arsenic(V) adsorption from aqueous solution onto goethite, hematite, magnetite and zero-valent iron: effects of pH, concentration and reversibility, Desalination, 2011, 281, 93–99 CrossRef CAS.
- Z. Yu, L. Hu and I. M. Lo, Transport of the arsenic (As)-loaded nano zero-valent iron in groundwater-saturated sand columns: Roles of surface modification and As loading, Chemosphere, 2018, 216, 428–436 CrossRef PubMed.
- Z. Yu, C. Zhang, Z. Zheng, L. Hu, X. Li, Z. Yang, C. Ma and G. Zeng, Enhancing phosphate adsorption capacity of SDS-based magnetite by surface modification of citric acid, Appl. Surf. Sci., 2017, 403, 413–425 CrossRef CAS.
- C. Zhang, Z. Yu, G. Zeng, B. Huang, H. Dong, J. Huang, Z. Yang, J. Wei, L. Hu and Q. Zhang, Phase transformation of crystalline iron oxides and their adsorption abilities for Pb and Cd, Chem. Eng. J., 2016, 284, 247–259 CrossRef CAS.
- X. Zhao, W. Liu, Z. Cai, B. Han, T. Qian and D. Zhao, An overview of preparation and applications of stabilized zero-valent iron nanoparticles for soil and groundwater remediation, Water Res., 2016, 100, 245–266 CrossRef CAS PubMed.
- H. Dong, X. Guan and I. M. Lo, Fate of As(V)-treated nano zero-valent iron: determination of arsenic desorption potential under varying environmental conditions by phosphate extraction, Water Res., 2012, 46, 4071–4080 CrossRef CAS PubMed.
- X. Peng, B. Xi, Y. Zhao, Q. Shi, X. Meng, X. Mao, Y. Jiang, Z. Ma, W. Tan, H. Liu and B. Gong, Effect of Arsenic on the Formation and Adsorption Property of Ferric Hydroxide Precipitates in ZVI Treatment, Environ. Sci. Technol., 2017, 51, 10100–10108 CrossRef CAS PubMed.
- F. Tang, J. Xin, T. Zheng, X. Zheng, X. Yang and O. Kolditz, Individual and combined effects of humic acid, bicarbonate and calcium on TCE removal kinetics, aging behavior and electron efficiency of mZVI particles, Chem. Eng. J., 2017, 324, 324–335 CrossRef CAS.
- Y. Jia and G. P. Demopoulos, Coprecipitation of arsenate with iron(III) in aqueous sulfate media: effect of time, lime as base and co-ions on arsenic retention, Water Res., 2008, 42, 661–668 CrossRef CAS PubMed.
- B. Hu, G. Chen, C. Jin, J. Hu, C. Huang, J. Sheng, G. Sheng, J. Ma and Y. Huang, Macroscopic and spectroscopic studies of the enhanced scavenging of Cr(VI) and Se(VI) from water by titanate nanotube anchored nanoscale zero-valent iron, J. Hazard. Mater., 2017, 336, 214–221 CrossRef CAS PubMed.
- Y. Xie, H. Dong, G. Zeng, L. Zhang, Y. Cheng, K. Hou, Z. Jiang, C. Zhang and J. Deng, The comparison of Se(IV) and Se(VI) sequestration by nanoscale zero-valent iron in aqueous solutions: The roles of solution chemistry, J. Hazard. Mater., 2017, 338, 306–312 CrossRef CAS PubMed.
- T. Yoadsomsuay, N. Grisdanurak and C. H. Liao, Influence of chitosan on modified nanoscale zero-valent iron for arsenate removal, Desalin. Water Treat., 2016, 57, 17861–17869 CrossRef CAS.
- L. Sun, L. Zhang, C. Liang, Z. Yuan, Y. Zhang, W. Xu, J. Zhang and Y. Chen, Chitosan modified Fe0 nanowires in porous anodic alumina and their application for the removal of hexavalent chromium from water, J. Mater. Chem., 2011, 21, 5877–5880 RSC.
- M. Bhaumik, C. Noubactep, V. K. Gupta, R. I. McCrindle and A. Maity, Polyaniline/Fe0 composite nanofibers: An excellent adsorbent for the removal of arsenic from aqueous solutions, Chem. Eng. J., 2015, 271, 135–146 CrossRef CAS.
- M. Bhaumik, R. I. McCrindle and A. Maity, Enhanced adsorptive degradation of Congo red in aqueous solutions using polyaniline/Fe0 composite nanofibers, Chem. Eng. J., 2015, 260, 716–729 CrossRef CAS.
- H. Dong and I. M. Lo, Influence of calcium ions on the colloidal stability of surface-modified nano zero-valent iron in the absence or presence of humic acid, Water Res., 2013, 47, 2489–2496 CrossRef CAS PubMed.
- H. Dong and I. M. Lo, Influence of humic acid on the colloidal stability of surface-modified nano zero-valent iron, Water Res., 2013, 47, 419–427 CrossRef CAS PubMed.
- N. Horzum, M. M. Demir, M. Nairat and T. Shahwan, Chitosan fiber-supported zero-valent iron nanoparticles as a novel sorbent for sequestration of inorganic arsenic, RSC Adv., 2013, 3, 7828 RSC.
- J. Liu, A. Liu and W. X. Zhang, The influence of polyelectrolyte modification on nanoscale zero-valent iron (nZVI): Aggregation, sedimentation, and reactivity with Ni(II) in water, Chem. Eng. J., 2016, 303, 268–274 CrossRef CAS.
- A. Gupta, M. Yunus and N. Sankararamakrishnan, Zerovalent iron encapsulated chitosan nanospheres-A novel adsorbent for the removal of total inorganic Arsenic from aqueous systems, Chemosphere, 2012, 86, 150–155 CrossRef CAS PubMed.
- L. Tang, H. Feng, J. Tang, G. Zeng, Y. Deng, J. Wang, Y. Liu and Y. Zhou, Treatment of arsenic in acid wastewater and river sediment by Fe@Fe2O3 nanobunches: The effect of environmental conditions and reaction mechanism, Water Res., 2017, 117, 175–186 CrossRef CAS PubMed.
- H. Feng, L. Tang, J. Tang, G. Zeng, H. Dong, Y. Deng, L. Wang, Y. Liu, X. Ren and Y. Zhou, Cu-Doped Fe@Fe2O3 core-shell nanoparticle shifted oxygen reduction pathway for high-efficiency arsenic removal in smelting wastewater, Environ. Sci.: Nano, 2018, 5, 1595–1607 RSC.
- S. Bhowmick, S. Chakraborty, P. Mondal, W. Van Renterghem, S. Van den Berghe, G. Roman-Ross, D. Chatterjee and M. Iglesias, Montmorillonite-supported nanoscale zero-valent iron for removal of arsenic from aqueous solution: Kinetics and mechanism, Chem. Eng. J., 2014, 243, 14–23 CrossRef CAS.
- L. Ling and W. X. Zhang, Sequestration of Arsenate in Zero-Valent Iron Nanoparticles: Visualization of Intraparticle Reactions at Angstrom Resolution, Environ. Sci. Technol. Lett., 2014, 1, 305–309 CrossRef CAS.
- S. Fendorf, M. J. Eick, P. Grossl and D. L, Sparks, Arsenate and chromate retention mechanisms on goethite. 1. Surface structure, Environ. Sci. Technol., 1997, 31, 315–320 CrossRef CAS.
- C. Wu, J. Tu, W. Liu, J. Zhang, S. Chu, G. Lu, Z. Lin and Z. Dang, The double influence mechanism of pH on arsenic removal by nano zero valent iron: electrostatic interactions and the corrosion of Fe0, Environ. Sci.: Nano, 2017, 4, 1544–1552 RSC.
- V. Tanboonchuy, N. Grisdanurak and C. H. Liao, Background species effect on aqueous arsenic removal by nano zero-valent iron using fractional factorial design, J. Hazard. Mater., 2012, 205–206, 40–46 CrossRef CAS.
- M. S. Mak, P. Rao and I. M. Lo, Effects of hardness and alkalinity on the removal of arsenic(V) from humic acid-deficient and humic acid-rich groundwater by zero-valent iron, Water Res., 2009, 43, 4296–4304 CrossRef CAS.
- X. H. Guan, J. Wang and C. C. Chusuei, Removal of arsenic from water using granular ferric hydroxide: macroscopic and microscopic studies, J. Hazard. Mater., 2008, 156, 178–185 CrossRef CAS.
- H. S. Kim, J. Y. Ahn, C. Kim, S. Lee and I. Hwang, Effect of anions and humic acid on the performance of nanoscale zero-valent iron particles coated with polyacrylic acid, Chemosphere, 2014, 113, 93–100 CrossRef CAS.
Footnote |
† Electronic supplementary information (ESI) available. See DOI: 10.1039/c8ew00757h |
|
This journal is © The Royal Society of Chemistry 2019 |
Click here to see how this site uses Cookies. View our privacy policy here.