DOI:
10.1039/C9NA00401G
(Communication)
Nanoscale Adv., 2019,
1, 3400-3405
One-pot synthesis of a new generation of hybrid bisphosphonate polyoxometalate gold nanoparticles as antibiofilm agents†
Received
25th June 2019
, Accepted 29th July 2019
First published on 29th July 2019
Abstract
A reduced polyoxovanadate functionalized with bisphosphonate molecules was synthesized and used to prepare in one step hybrid organic–inorganic polyoxometalate decorated gold nanoparticles. These new composites were shown to strongly inhibit P. aeruginosa and S. epidermidis biofilm growth, with the three components constituting the nanoparticles (Au0 core, vanadium and alendronate) acting synergistically.
Nowadays, the wide use of antibiotics has led to the emergence of resistant bacteria causing serious public health problems. Antibiotic resistance currently includes all known classes of natural and synthetic compounds leading to an urgent requirement for alternatives.1 Among the different resistance mechanisms developed by bacteria, some bacterial populations use cell attachment to solid substrates forming associations named biofilms.2 Particularly, bacterial biofilms are much more resistant to antibiotics than planktonic cells because of the generation of extracellular polymeric substances, which impede the penetration of antibacterial agents that reduce the growth rates of the biofilm cells, impacting the effectiveness of antibiotics capable of targeting rapidly multiplying cells.3,4 Recently, the use of antimicrobial nanomaterials has attracted great attention because of their ability to disrupt the bacterial membranes and to hinder biofilm formation.5,6 Several nanomaterials, such as silver, copper and zinc oxide nanoparticles (NPs), exhibit intrinsic antibacterial activity.1 Nevertheless, their toxicity remains problematic. In contrast, it has been evidenced that gold nanoparticles (AuNPs) are non-toxic,7–9 and in the last decade conjugates of AuNPs with antibiotics10–14 or other antibacterial agents15–19 have been shown to improve antibacterial activity against biofilms. Besides, several polyoxometalates (POMs), which can be seen as negatively charged molecular oxides incorporating metals (mainly tungsten, molybdenum and vanadium) in high oxidation states,20 have shown important antibacterial activity,21–26 and notably two examples of antibiofilm POM composites have been reported in the literature.27,28 In this context, the use of POMs as active antibacterial agents supported by AuNPs represents a promising alternative. The relevance of this approach to the elaboration of antibacterial agents is supported by the studies of Daima et al., which showed that AuNPs post-functionalized with purely inorganic commercial POMs exhibit antibacterial activity against the non-resistant E. coli bacteria.29,30 In addition, precedent studies have established that POMs containing MoV or VIV ions can be used as reducing agents for the synthesis of a large variety of POM functionalized metallic NPs. Importantly, AuNPs consisting of a metallic core stabilized by POMs in their oxidized form have been obtained in one step, at room temperature and in water.31–33 This synthetic strategy has, however, only been exploited for purely inorganic reduced POMs, while the use of reduced hybrid organic–inorganic polyoxometalates could lead to antibacterial materials combining the properties of AuNPs, POMs and biologically active organic substrates. The chemistry of POMs structured by bisphosphonate ligands (BPs) has been widely studied,34–40 and in particular several molybdenum, tungsten and vanadium complexes incorporating the alendronate (Ale) ligand [O3PC(C3H6NH3)OPO3]4− have been obtained.20,41 Interestingly, it has also been shown that alendronate itself increases antibiofilm effects when combined with bioactive glass.42 Considering inorganic POMs, it has been evidenced that compounds incorporating vanadium centers exhibit the highest antibacterial activities.19 It follows that the study of the antibacterial properties of systems combining alendronate molecules, POMs containing vanadium ions and AuNPs appears to be particularly attractive.
Herein, we report on the inhibition of Gram-negative and Gram-positive bacteria biofilm growth by such composites. A reduced polyoxovanadate (POVred) functionalized with alendronate ligands was first synthesized. Then, the ability of this reduced POVred to act as both a reducing and stabilizing agent was exploited32,43 to prepare in one step alendronate POV capped gold NPs (NPs@POV). In parallel, citrate-stabilized gold nanoparticles (CitNPs) functionalized with hybrid POVs in their reduced form were prepared for comparison of the activities of the two systems (Scheme 1). Finally the antibacterial properties of these new NPs@POV hybrid materials toward biofilm formation by Pseudomonas aeruginosa and Staphylococcus epidermidis were studied. To the best of our knowledge, this work represents the first investigation of materials associating NPs, POMs and biologically active organic substrates for antibiofilm applications.
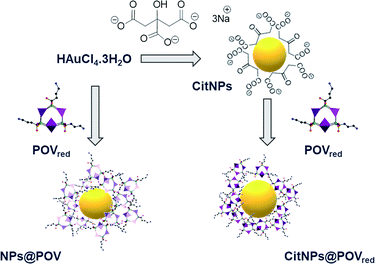 |
| Scheme 1 Schematic representation of CitNPs, CitNPs@POVred and NPs@POV synthesis. | |
Results and discussion
Polyoxovanadate and nanoparticle synthesis and characterization
First, a reduced polyoxovanadate (V3(Ale)3, also called here POVred) was synthesized by mixing sodium metavanadate and alendronic acid at pH 4.7 in water. In this synthesis, the VV ions are likely reduced by triethylamine which is added in the medium to increase the pH (see the Experimental section, ESI†). Despite numerous efforts, only low quality single-crystals were obtained. However, data collection at the synchrotron allowed solving the structure of the synthesized compound. In the structure of POVred, two independent molecules are in the asymmetric unit, which only differ by the position of the alkyl chain of one of the alendronate ligands (Fig. 1 and S1, ESI†). X-ray diffraction studies thus evidence the formation of the title complex Na3[V3O3(H2O)(O3PC(C3H6NH3)(OH)PO3)3]·10H2O (V3(Ale)3). This POVred is reminiscent of the zoledronate compound Na3[V3O3(H2O)(O3PC(C4H6N2)(OH)PO3)3]·12H2O (V3(Zol)3) previously characterized by some of us.44 In both V3(Zol)3 and V3(Ale)3, three VIV ions (bond valence sums for the vanadium ions in V3(Ale)3 = 3.80–4.05, Fig. S1, ESI†) form an isosceles triangle, with the vanadium centers being in distorted octahedral environments. Three alendronate moieties occupy the edges of the triangle, acting as tetradentate ligands by connecting two by two the vanadium ions. Each vanadium center is connected to a terminal oxygen atom (dV
O = 1.63–1.65 Å). Two of these V
O bonds (V2 = O and V3 = O, Fig. 1) point to the outside of the molecule while the third one (V1 = O) points to the inside. Finally, the coordination sphere of the V1 atom is completed by a terminal water molecule while the V2 and V3 atoms are weakly bound to the O
V1 oxygen atom (dV–O = 2.76 and 2.73 Å, respectively). Energy-dispersive X-ray (EDX) spectroscopy measurements are in agreement with a P
:
V
:
Na ratio of 6
:
3
:
3 (ESI†). This is confirmed by Inductively Coupled Plasma Atomic Emission Spectroscopy (ICP-OES), with a determined P/V ratio of 2.04. Elemental analysis further confirms the proposed formula (Experimental section, ESI†). The TGA curve (Fig. S2a, ESI†) reveals three steps that can be attributed to water removal, ligand decomposition and finally formation of inorganic oxides (final experimental weight loss 41.0%, calculated 39.0%). Finally, the infrared spectrum of POVred (Fig. S2b, ESI†) presents the characteristic bands of both the P–O/P
O groups in the 1039–1086 cm−1 range and P–O and V
O/V–O groups in the 929–999 cm−1 range.
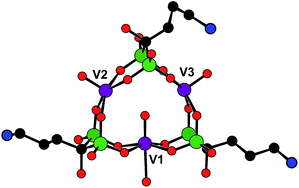 |
| Fig. 1 Ball-and-stick representation of one of the molecules present in the structure of the V3(Ale)3 complex. Purple sphere = V, green spheres = P, black spheres = C, red spheres = O, blue spheres = N; H atoms are omitted for clarity. | |
POVred was then used to prepare in one step functionalized polyoxovanadate decorated gold nanoparticles. While reduced POVs have already been considered for the one pot preparation of metallic nanoparticles,45,46 it rapidly appeared that the nanoparticle synthesis protocol with the reported hybrid POVred needed to be tailored. In order to prevent the POVred denaturation, the synthesis was carried out by adding an aqueous solution of HAuCl4 basified to pH = 6 to an aqueous solution of POVred at pH = 6. Indeed, the IR spectrum of the powder obtained by dissolution in water (pH 6) of POVred followed by the addition of ethanol is similar to that of POVred (Fig. S2b, ESI†), strongly suggesting that the title complex is stable in this medium. Additionally, an excess of POM was used (γ = [HAuCl4]/[POM] = 0.3) to ensure the total reduction of the AuIII ions (see the ESI† for details). The colour change of the solution from turquoise to pink was indicative of the formation of the NPs@POV (POV being an oxidized form of the POVred) gold nanoparticles, as confirmed by the presence of a surface plasmon resonance (SPR) band at 531 nm in the UV-spectra (Fig. 2a). In parallel, the CitNPs synthesized following the Turkevich method47 and the POVred functionalized citrate nanoparticles (CitNPs@POVred), prepared according to the procedure previously described48 for the grafting of Keggin polyoxotungstates, both show the expected narrow band centered at 520 nm characteristic of a particle size of ca. 15 nm (Fig. 2a). Note that the shift in the position of the SPR bands between the NPs@POV and CitNPs@POVred is characteristic of the different sizes of the nanoparticles.
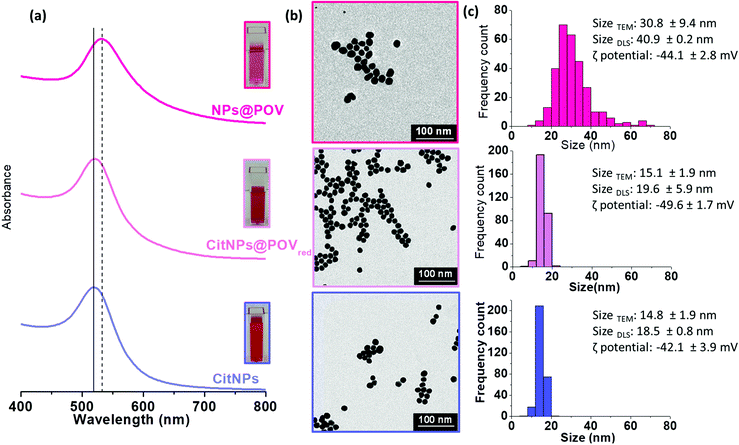 |
| Fig. 2 Characterization of CitNPs, CitNPs@POVred and NPs@POV. UV-vis spectra and colloidal suspension pictures (inset) (a), TEM images (b) and particle size distribution histograms and dynamic light scattering size and zeta potential values (c). | |
The size and dispersion of NPs@POV, CitNPs@POVred and CitNPs were determined from Transmission Electron Microscopy (TEM) (Fig. 2b and S3, ESI†) and Dynamic Light Scattering (DLS) analyses (Fig. 2c). TEM images revealed particle sizes of 30.8 ± 9.4, 15.1 ± 1.9 and 14.8 ± 1.9 nm for NPs@POV, CitNPs@POVred, and CitNPs, respectively. As expected, the hydrodynamic diameter measured by DLS is larger for the three different batches of particles as it takes into account the presence of hydration layers, but also of POM layers for the two decorated NPs, not visible on TEM images. Besides, the zeta potential of CitNPs@POVred (−49.6 ± 1.7 mV) is lower than the zeta potential of pristine CitNPs (−42.1 ± 3.9 mV), which can be due to the adsorption at the surface of the NPs of the triply negatively charged POV. The zeta potential of NPs@POV (−44.1 ± 2.8 mV) is slightly less negative than for CitNPs@POVred. This can be attributed to the oxidation of the VIV centers during the formation of the NPs@POV. Attenuated Total Reflectance Fourier Transform Infrared spectroscopy (ATR-FTIR) has also confirmed the presence of POVs at the surface of both NPs@POV and CitNPs@POVred, with the V–O vibrations related to the POV together with the P–O vibrations and NH3 deformations related to the alendronate ligand being observed in the IR spectra (Fig. S4 and Table S1, ESI†). ICP-OES allowed confirming the presence of V and P in stoichiometric proportions compared to the initial POV, with a P/V ratio equal to 2.0 ± 0.1 for NPs@POV and 1.9 ± 0.1 for CitNPs@POVred. Moreover, taking into account the Au/V ratio determined by ICP and the size of the NPs, the number of POV molecules per nm2 was estimated to be 38 ± 8 for the spherical 30.8 nm NPs@POV and 23 ± 5 for the 15.1 nm CitNPs@POVred (see the ESI† for details). We can note that the coverages are too high to correspond to a monolayer, which would lead to a maximum of 6 POV per nm2 (see the ESI† for details). Therefore, the ICP data clearly corroborate the presence of POV multilayers as previously discussed on the basis of DLS measurements.
Inhibition of Gram-negative and Gram-positive bacteria biofilm growth by CitNPs, CitNPs@POVred and NPs@POV
The optical density method (OD620 nm) based on crystal violet staining was used to investigate the effect of CitNPs, CitNPs@POVred and NPs@POV on P. aeruginosa ATCC® 27853™ and S. epidermidis CIP 105 777 biofilm formation (see the ESI† for details).49 These Gram-negative and Gram-positive bacteria, respectively, represent an excellent model for biofilm growth inhibition studies as they have been shown to severely develop on medical device surfaces, causing serious nosocomial infections.50–53 The biofilm formation was examined over periods of 24 and 48 hours (Fig. 3). The antibacterial activities of POVred and alendronate at concentrations higher than those established for the functionalized NPs were also determined as references for the two different strains.
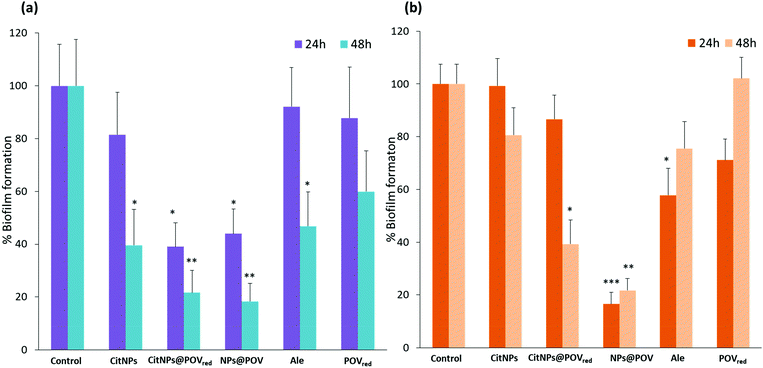 |
| Fig. 3 The effect of CitNPs (1.3 nM), CitNPs@POVred (1.3 nM), NPs@POV (1.0 nM), alendronate (540 μM), and POVred (180 μM) on biofilm formation by (a) P. aeruginosa and (b) S. epidermidis after incubation for 24 and 48 h in 96-well plates. The values of the experimental groups were compared with the control group. Statistical significance was analyzed by Students’ t-test to detect the presence of statistically significant differences (***p < 0.001, **p < 0.01, *p < 0.05). For CitNPs, CitNPs@POVred and NPs@POV the numbers in brackets correspond to the concentration of gold nanoparticles. | |
For P. aeruginosa, the bacterial growth was strongly inhibited after 24 hours of treatment by both NPs@POV and CitNPs@POVred, with a decrease of biofilm formation of ca. 60% compared to the control experiment. Noticeably, the biofilm growth inhibition is not significant for neither the unfunctionalized CitNPs nor the POVred complex, when utilized separately, highlighting a synergistic effect between the AuNPs and the POV. These results suggest that the gold nanoparticles could act as carriers to improve the penetration of the POV into the bacteria. This trend is confirmed after 48 hours of treatment, with a decrease of the biofilm formation of ca. 80% for NPs@POV and CitNPs@POVred. Noticeably, after 48 h of treatment with alendronate and CitNPs, an antibacterial activity is observed as biofilm growth inhibition was around 60%. However this antibacterial effect remains lower than the one observed for POV decorated nanoparticles, confirming the synergistic effect.
To investigate the possible influence of the particle size on the antibacterial activity, the inhibitory effects of citrate gold particles functionalized with POVred (CitNPs@POVred) with two different sizes (15 and 47 nm) were investigated on P. aeruginosa. The synthesis and characterization of the 47 nm diameter AuNPs are described in the ESI (see the Experimental section and Fig. S5†). The antibacterial activities of the 15 and 47 nm NPs were found to be similar (Fig. S6†). Therefore, we can assume that the size of the nanoparticles cannot be regarded as a determining factor influencing the antibacterial activity.
Focusing now on S. epidermidis, Fig. 3b, it was observed that only the NPs@POV induce a significant decrease of the biofilm formation starting after 24 h of treatment, and, very interestingly, this effect is very strong (>80%). The high antibacterial activity of the NPs@POV compared to the absence of activity of the CitNPs@POVred against S. epidermidis can be tentatively attributed to the oxidation state of the vanadium centers (VIV in CitNPs@POVred and VV in NPs@POV). Finally, we can also note that the antibiofilm activity of the NPs@POV against S. epidermidis remains unchanged after an additional 24 h.
The effect on the biofilm growth of the NPs@POV concentration in the 0.1–1 nM range was also studied for both strains (Fig. S7, ESI†). For a concentration of 0.1 nM, a decrease of the P. aeruginosa biofilm formation of 50% after 24 h of treatment was observed, with no further evolution after 24 h. At 0.2 nM, a decrease of 60% after 24 h and 80% after 48 h was determined, indicating that a 0.2 nM concentration of NPs@POV is sufficient to obtain a strong antibacterial effect against this strain. Regarding S. epidermidis, a decrease of the biofilm formation of 40 and 70% upon 24 and 48 h of treatment, respectively, was observed for a concentration of 0.6 nM. The maximum antibacterial effect was found for a concentration of 1 nM, with ca. 80% inhibition of the biofilm formation after 24 h.
In order to observe possible changes of the morphology of bacteria, scanning electron microscopy (SEM-FEG) micrographs of the P. aeruginosa and S. epidermidis were recorded after 24 h in the presence and in the absence of NPs@POV (Fig. S8, ESI†). We used NPs@POV as an example as these hybrids show a good antibacterial activity against both bacterial strains.
The SEM images of the two bacterial strains without treatment showed intact bacterial membranes with P. aeruginosa exhibiting a rod like shape (Fig. S8a, ESI†) and S. epidermidis exhibiting a spherical shape (Fig. S8c, ESI†). In contrast, the cell envelopes of P. aeruginosa and S. epidermidis treated with NPs@POV are damaged and appear pierced causing the leakage of the cytoplasmic content and the collapse of the bacteria (Fig. S8b and d, ESI†). From these results, it could be concluded that NPs@POV seriously affect the bacterial membrane integrity causing bacterial death. The exact antibacterial mechanism is, however, not well elucidated and will be investigated in future studies.
Conclusions
In conclusion, we have used a new reduced polyoxovanadate V3(Ale)3 incorporating the biologically relevant alendronate ligand to prepare in one-pot, in water, at room temperature and in the absence of any additional surfactant the hybrid functionalized gold nanoparticles NPs@POV. The optimized synthetic protocol produced size-controlled monodisperse stable NPs. The POVred thus acts as a reducing agent but also as capping agent to stabilise the nanoparticles. The nanocomposites were thoroughly characterized by TGA, ATR-FTIR, UV-Vis, DLS, TEM, EDX and elemental analysis experiments. The high antibiofilm activity of NPs@POV towards both the Gram-negative and Gram-positive resistant bacteria was evidenced. Importantly, comparative studies strongly suggest that the three components constituting the NPs@POV (Au0 core, vanadium and alendronate) act synergistically. The activity of POVred functionalized citrate gold nanoparticles, CitNPs@POVred, was also studied, allowing us to conclude that the oxidation state of the vanadium centers constituting the POM may play a significant role in the inhibition of Gram-positive bacteria biofilm growth. This work, which to the best of our knowledge represents the first investigation of POM-functionalized NPs for antibiofilm applications, opens the way to a new generation of antibiofilm agents. We are currently investigating the influence of the nature of the polyoxometalate on the biological properties of such nanocomposites. Also, an advantage of alendronate is that it can be easily functionalized via its amino group. We are thus also presently working on the elaboration and the investigation of the inhibition of biofilm growth activity of antibiotic/alendronate/polyoxometalate NP systems.
Conflicts of interest
There are no conflicts to declare.
Acknowledgements
This work was supported by the Ministère de l’Enseignement Supérieur et de la Recherche, the CNRS, the Université de Versailles Saint Quentin en Yvelines and a public grant overseen by the French National Research Agency (ANR) as part of the “Investissements d’Avenir” program no. ANR-11-IDEX-0003-02 and CHARMMMAT ANR-11-LABX-0039.
Notes and references
- P. V. Baptista, M. P. McCusker, A. Carvalho, D. A. Ferreira, N. M. Mohan, M. Martins and A. R. Fernandes, Front. Microbiol., 2018, 9, 1–26 CrossRef PubMed.
- L. Hall-Stoodley, J. W. Costerton and P. Stoodley, Nat. Rev. Microbiol., 2004, 2, 95–108 CrossRef CAS PubMed.
- D. Davies, Nat. Rev. Drug Discovery, 2003, 2, 114–122 CrossRef CAS PubMed.
- P. S. Stewart and J. W. Costerton, Lancet, 2001, 358, 135–138 CrossRef CAS.
- G. R. Rudramurthy, M. K. Swamy, U. R. Sinniah and A. Ghasemzadeh, Molecules, 2016, 21, 1–30 CrossRef.
- C. W. Chen, C. Y. Hsu, S. M. Lai, W. J. Syu, T. Y. Wang and P. S. Lai, Adv. Drug Delivery Rev., 2014, 78, 88–104 CrossRef CAS PubMed.
- J. Conde, M. Larguinho, A. Cordeiro, L. R. Raposo, P. M. Costa, S. Santos, M. S. Diniz, A. R. Fernandes and P. V. Baptista, Nanotoxicology, 2014, 8, 521–532 CrossRef CAS PubMed.
- X. Li, S. M. Robinson, A. Gupta, K. Saha, Z. Jiang, D. F. Moyano, A. Sahar, M. A. Riley and V. M. Rotello, ACS Nano, 2014, 8, 10682–10686 CrossRef CAS PubMed.
- U. Rajchakit and V. Sarojini, Bioconjugate Chem., 2017, 28, 2673–2686 CrossRef CAS.
- Y. Zhao and X. Jiang, Nanoscale, 2013, 5, 8340–8350 RSC.
- M. Rai, A. P. Ingle, R. Pandit, P. Paralikar, I. Gupta, M. V. Chaud and C. A. dos Santos, Int. J. Pharm., 2017, 532, 139–148 CrossRef CAS.
- A. N. Brown, K. Smith, T. A. Samuels, J. Lu, S. O. Obare and M. E. Scott, Appl. Environ. Microbiol., 2012, 78, 2768–2774 CrossRef CAS PubMed.
- T. Roshmi, K. R. Soumya, M. Jyothis and E. K. Radhakrishnan, Gold Bull., 2015, 48, 63–71 CrossRef CAS.
- H. Mu, J. Tang, Q. Liu, C. Sun, T. Wang and J. Duan, Sci. Rep., 2016, 6, 1–9 CrossRef.
- G. Vinoj, R. Pati, A. Sonawane and B. Vaseeharan, Antimicrob. Agents Chemother., 2015, 59, 763–771 CrossRef PubMed.
- Q. Yu, J. Li, Y. Zhang, Y. Wang, L. Liu and M. Li, Sci. Rep., 2016, 6, 1–14 CrossRef.
- M. Ramasamy, J. H. Lee and J. Lee, Colloids Surf., B, 2017, 160, 639–648 CrossRef CAS PubMed.
- X. Zhao, Y. Jia, J. Li, R. Dong, J. Zhang, C. Ma, H. Wang, Y. Rui and X. Jiang, ACS Appl. Mater. Interfaces, 2018, 10, 29398–29406 CrossRef CAS PubMed.
- Y. Feng, W. Chen, Y. Jia, Y. Tian, Y. Zhao, F. Long, Y. Rui and X. Jiang, Nanoscale, 2016, 8, 13223–13227 RSC.
- A. Dolbecq, E. Dumas, C. R. Mayer and P. Mialane, Chem. Rev., 2010, 110, 6009–6048 CrossRef CAS PubMed.
- A. Bijelic, M. Aureliano and A. Rompel, Chem. Commun., 2018, 54, 1153–1169 RSC.
- N. I. Gumerova, E. Al-Sayed, L. Krivosudský, H. Čipčić-Paljetak, D. Verbanac and A. Rompel, Front. Chem., 2018, 6, 1–9 CrossRef PubMed.
- T. Yamase, J. Mater. Chem., 2005, 15, 4773 RSC.
- A. Kubo, L. Kremer, S. Herrmann, S. G. Mitchell, O. M. Bondarenko, A. Kahru and C. Streb, ChemPlusChem, 2017, 10130, 867–871 CrossRef.
- P. Yang, B. S. Bassil, Z. Lin, A. Haider, G. Alfaro-Espinoza, M. S. Ullrich, C. Silvestru and U. Kortz, Chem.–Eur. J., 2015, 21, 15600–15606 CrossRef CAS PubMed.
- P. Yang, Z. Lin, G. Alfaro-Espinoza, M. S. Ullrich, C. I. Raţ, C. Silvestru and U. Kortz, Inorg. Chem., 2016, 55, 251–258 CrossRef CAS PubMed.
- A. Misra, I. Franco Castillo, D. P. Müller, C. González, S. Eyssautier-Chuine, A. Ziegler, J. M. de la Fuente, S. G. Mitchell and C. Streb, Angew. Chem., Int. Ed., 2018, 57, 14926–14931 CrossRef CAS PubMed.
- R. H. Piva, M. C. Rocha, D. H. Piva, H. Imasato, I. Malavazi and U. P. Rodrigues-Filho, ACS Appl. Mater. Interfaces, 2018, 10, 30963–30972 CrossRef CAS.
- H. K. Daima, P. R. Selvakannan, A. E. Kandjani, R. Shukla, S. K. Bhargavaa and V. Bansal, Nanoscale, 2014, 6, 758–765 RSC.
- H. K. Daima, P. R. Selvakannan, R. Shukla, S. K. Bhargava and V. Bansal, PLoS One, 2013, 8, 1–14 CrossRef PubMed.
- A. Dolbecq, J. D. Compain, P. Mialane, J. Marrot, F. Sécheresse, B. Keita, L. R. B. Holzle, F. Miserque and L. Nadjo, Chem.–Eur. J., 2009, 15, 733–741 CrossRef CAS PubMed.
- G. Zhang, B. Keita, R. N. Biboum, F. Miserque, P. Berthet, A. Dolbecq, P. Mialane, L. Catala and L. Nadjo, J. Mater. Chem., 2009, 19, 8639–8644 RSC.
- U. Jameel, M. Zhu, X. Chen and Z. Tong, J. Mater. Sci., 2016, 51, 2181–2198 CrossRef CAS.
- R. Ban, X. Sun, J. Wang, P. Ma, C. Zhang, J. Niu and J. Wang, Dalton Trans., 2017, 46, 5856–5863 RSC.
- L. Yang, Z. Zhou, J. Wang and J. Niu, Cryst. Growth Des., 2013, 4, 2540–2547 CrossRef.
- A. Banerjee, F. S. Raad, N. Vankova, B. S. Bassil, T. Heine and U. Kortz, Inorg. Chem., 2011, 50, 11667–11675 CrossRef CAS PubMed.
- U. Kortz, G. B. Jameson and M. T. Pope, J. Am. Chem. Soc., 1994, 116, 2659–2660 CrossRef CAS.
- A. Banerjee, B. S. Bassil, G. V. Röschenthaler and U. Kortz, Chem. Soc. Rev., 2012, 41, 7590–7604 RSC.
- A. Saad, W. Zhu, G. Rousseau, P. Mialane, J. Marrot, M. Haouas, F. Taulelle, R. Dessapt, H. Serier-Brault, E. Rivière, T. Kubo, E. Oldfield and A. Dolbecq, Chem.–Eur. J., 2015, 21, 10537–10547 CrossRef CAS.
- C. Peloux, P. Mialane and A. Dolbecq, Angew. Chem., Int. Ed., 2002, 542, 2808–2810 CrossRef.
- J. D. Compain, P. Mialane, J. Marrot, F. Sécheresse, W. Zhu, E. Oldfield and A. Dolbecq, Chem.–Eur. J., 2010, 16, 13741–13748 CrossRef CAS PubMed.
- A. K. Hiltunen, M. E. Skogman, K. Rosenqvist, H. Juvonen, P. Ihalainen, J. Peltonen, A. Juppo and A. Fallarero, Int. J. Pharm., 2016, 501, 211–220 CrossRef CAS PubMed.
- Y. Wang and I. A. Weinstock, Chem. Soc. Rev., 2012, 41, 7479–7496 RSC.
- H. El Moll, W. Zhu, E. Oldfield, L. M. Rodriguez-Albelo, P. Mialane, J. Marrot, N. Vila, I. M. Mbomekallé, E. Rivière, C. Duboc and A. Dolbecq, Inorg. Chem., 2012, 51, 7921–7931 CrossRef CAS PubMed.
- Y. Y. Bao, L. H. Bi and L. X. Wu, J. Solid State Chem., 2011, 184, 546–556 CrossRef CAS.
- R. Liu, S. Li, X. Yu, G. Zhang, S. Zhang, J. Yao, B. Keita, L. Nadjo and L. Zhi, Small, 2012, 8, 1398–1406 CrossRef CAS PubMed.
- J. Tukevich, P. C. Stevenson and J. Hillier, Discuss. Faraday Soc., 1951, 11, 55–75 RSC.
- Y. Wang, A. Neyman, E. Arkhangelsky, V. Gitis, L. Meshi and I. A. Weinstock, J. Am. Chem. Soc., 2009, 131, 17412–17422 CrossRef CAS PubMed.
- H. Mu, A. Zhang, L. Zhang, H. Niu and J. Duan, Food Control, 2014, 38, 215–220 CrossRef CAS.
- K. Niemirowicz, I. Swiecicka, A. Z. Wilczewska, I. Misztalewska, B. Kalska-Szostko, K. Bienias, R. Bucki and H. Car, Int. J. Nanomed., 2014, 9, 2217–2224 CrossRef PubMed.
- M. Klausen, A. Heydorn, P. Ragas, L. Lambertsen, A. Aaes-Jørgensen, S. Molin and T. Tolker-Nielsen, Mol. Microbiol., 2003, 48, 1511–1524 CrossRef CAS PubMed.
- F. Barbier and M. Wolff, Med. Sci., 2010, 26, 960–968 Search PubMed.
- M. Otto, Nat. Rev. Microbiol., 2009, 7, 555–567 CrossRef CAS PubMed.
Footnotes |
† Electronic supplementary information (ESI) available: Experimental details, TGA and IR spectra, the effect of concentration on biofilm formation. See DOI: 10.1039/c9na00401g |
‡ These authors contributed equally to this work. |
|
This journal is © The Royal Society of Chemistry 2019 |
Click here to see how this site uses Cookies. View our privacy policy here.