DOI:
10.1039/C8QM00409A
(Research Article)
Mater. Chem. Front., 2019,
3, 39-49
A colorless semi-aromatic polyimide derived from a sterically hindered bromine-substituted dianhydride exhibiting dual fluorescence and phosphorescence emission†
Received
17th August 2018
, Accepted 19th October 2018
First published on 22nd October 2018
Abstract
Colorless, transparent, and environmentally stable polymer films with large luminescence Stokes shift are in demand for down-conversion of solar UV radiation. To develop such a polymer film that exhibits phosphorescence at room-temperature with a large Stokes shift and excellent thermal stability, a semi-aromatic polyimide (DBrBP-PI) was newly synthesized using a biphenyltetracarboxylic dianhydride substituted with two bromine (Br) atoms. Thin films of DBrBP-PI were completely colorless because of effective suppression of the aggregation of the PI chains due to the steric effects of the Br-substituted dianhydride structure. The PI films exhibited bright green phosphorescence at 512 nm (Φ = 0.02) with a very large Stokes shift of 12
215 cm−1 as well as prompt blue fluorescence at around 408 nm when excited at 315 nm at 298 K. The glass transition and the 5 wt% loss temperatures of DBrBP-PI were 325 °C and 427 °C, respectively. The phosphoresce intensity of the DBrBP-PI film was significantly enhanced under vacuum (Φ = 0.22) and at low temperatures (Φ = 0.76 at 77 K) owing to suppression of triplet state quenching by atmospheric oxygen and dumping of the molecular motions of the PI chains in the solid film. The suggested material design concept shows a way towards creation of stable and efficient down-converters for UV radiation.
1. Introduction
Photoluminescent organic polymers, such as polyphenylene and polyfluorene derivatives, have been widely studied because of their potential applications for spectral converters in the fields of luminescent solar concentrators, displays, LEDs, photovoltaic devices, and crop cultivation.1–4 Down-converters are expected to convert short-wavelength light (ultraviolet (UV) or violet-blue light) to longer-wavelength light (green, yellow, red, or near-infrared (IR)) with high efficiency. Practical applications of solar down-converters have been investigated only with rare-earth or semiconductor quantum dots containing inorganic or metallic elements.1,5–7 Organic polymeric materials possess inherent advantages, e.g., low cost, light weight, flexibility, facile production, and the ability to be processed into solid thin films. However, conventional organic photoluminescent polymers consisting of π-conjugated sequences do not have sufficient thermal, mechanical, and environmental stabilities compared to their inorganic and metallic counterparts.7–18 In particular, because of their poor thermal stability, the high-temperature processes required for the fabrication of flat panel displays and solar cells are not applicable. In addition, they generally have no resistance to natural chemicals, environmental harshness, and long-term irradiation, which is also an important requirement for practical spectral converters.
Polyimides (PIs) are well known as a class of super-engineering plastics possessing outstanding thermal, chemical, and radiation stabilities originating from their rigid repeating-unit structures and strong intermolecular interactions.19,20 Therefore, they are applied in a wide range of industries, including the automotive, microelectronics, photonics, and aerospace industries. Recently, the photoluminescence properties of thin films of PIs have been studied extensively for possible applications as spectral converters.21–29 The photo-luminescence quantum yields (Φ) of conventional wholly aromatic PIs, which are synthesized from aromatic dianhydrides and aromatic diamines, are generally very low (Φ ≪ 0.01) because of the charge-transfer (CT) interactions between electron-donating diamine and electron-accepting dianhydride moieties. Thus, the photoluminescence of PIs received little attention for a long period. Hasegawa et al.21 reported that a wholly aromatic PI synthesized from pyromellitic dianhydride and bis(4-aminophenyl)ether exhibited very weak fluorescence emission in the solid film state (Φ = 9.7 × 10−7). However, we have previously reported that a series of semi-aromatic PI films, prepared from aromatic dianhydrides and alicyclic diamines, exhibited significantly enhanced fluorescence emissions owing to the suppression of the CT interaction by the weakly electron-donating alicyclic diamine.21–28 For example, a semi-aromatic PI film prepared from 1,4-bis(3,4-dicarboxyphenoxy)benzene dianhydride and 4,4′-diaminocyclohexylmethane (HQDEA/DCHM) exhibited strong blue fluorescence with a high Φ value of 0.11.23 This much higher Φ value compared to those of conventional PIs is attributable not only to effective suppression of the CT interaction, but also to the enhancement of the oscillator strengths of the local π–π* transitions derived from the π-conjugated dianhydride moiety. Although the photoluminescence properties of PI films have been improved dramatically on the basis of the above-mentioned molecular design, their Stokes shifts (ν), the energy gaps between the excitation and emission wavelengths, are insufficient for wavelength conversion applications. In the case of the commercialized single-crystal Si-type solar cell, their spectral sensitivity covers the visible and the near-IR region (400–1100 nm) and the highest efficiency is attained between 700 and 1000 nm.6 Therefore, in addition to a high Φ value, a significantly enlarged Stokes shift that enables the conversion of UV light (∼380 nm) to longer-wavelength light (>500 nm) is strongly demanded for practical spectral down-converters.30–34
The enhancement and control of the Stokes shifts of organic compounds have been attempted a number of ways, including the application of excited-state intramolecular proton transfer (ESIPT),24,26,29 charge transfer,35,36 excited complexes,37 and triplet states (phosphorescence).38
Room-temperature phosphorescence (RTP) of organic molecules has recently attracted increasing attention owing to potential applications based on extraordinarily large Stokes shifts (>10
000 cm−1) as well as ultra-long luminescence lifetimes (10−6 to 10 s).39–41 In general, organic molecules rarely exhibit RTP because of low intersystem crossing (ISC) efficiency and vigorous molecular motions accompanying non-radiative deactivations during the ultra-long lifetime of the excited triplet state. Therefore, phosphorescence of organic molecules has been observed only under cryogenic conditions or in rigid matrices such as crystalline compounds or host–guest systems, in which molecular motions are effectively suppressed.42–53 It has been known for many years that organic molecules substituted with heavy halogens (e.g., Br, I) inherently exhibit RTP.39,54 The spin–orbital coupling constants of heavy halogen atoms are much larger than those of light atoms such as C, N, and O, and this leads to a high possibility of ISC and efficient RTP.41 Recently, phosphorescence of heavy-halogen-containing organic molecules has attracted much interest again for the purpose of controlling emission colours and Stokes shifts.55–58 Because heavy-halogen-containing organic molecules have many advantages such as facile synthesis with low cost, low toxicity, and low environmental load, many researchers have attempted to apply them to practical materials including those used in bio-imaging and temperature/oxygen sensing.55–58 The RTP of such heavy-halogen-containing molecules can be realized by incorporating them with single crystals, co-crystals, or host–guest systems.59–68 These methods require high-quality crystals or the selection of suitable host matrices that are compatible with guest molecules. On the other hand, it has also been reported that organic polymers bearing heavy-halogen-containing phosphors in side groups or end groups exhibit phosphorescence. Tian et al.69 reported that polyacrylamide derivatives with bromobenzaldehyde, bromonaphthalene, and bromonaphthalic anhydride structures as the side groups exhibit green to red phosphorescence when suspended in N,N-dimethylformamide (DMF)/H2O and in the solid powdery state; this is attributed to the suppression of the molecular motions of the phosphors via interchain hydrogen bonding at the acrylamide moiety. Fraser et al.70–73 reported that polylactic acid with iodo-substituted difluoroboron dibenzoylmethane as the end groups exhibit tunable phosphorescence properties in response to the molecular weight of the main chain. In addition, owing to their biocompatibility and photo-stability, nanoparticles made of these polymers have been applied for hypoxia bio-imaging by using the fluorescence/phosphorescence intensity ratio. Although various organic polymers can exhibit RTP, their thermal and environmental stabilities are still insufficient, and most of them exhibit significant coloration; thus, they cannot be used for practical solar spectral converters.
Most recently, our group reported that semi-aromatic PIs containing a Br or an iodine (I) atom in the dianhydride moiety exhibit large-Stokes-shifted RTP (ν = ∼10
000 cm−1) while maintaining the thermal stability of PI (5% weight loss temperature T5d > 370 °C).28 Such new main-chain-type phosphorescent PIs can be used for thin films without the preparation of high-quality crystals and selection of suitable matrices. However, thin films of these PIs exhibit a broad absorption peak in the visible region, leading to yellowish coloration, owing to the PI chain aggregations in the film caused by π–π stacking between the dianhydride moieties of the PI chains. Because conventional solar cells inherently have sensitivity in the entire visible region, such visible absorption should be avoided, i.e., colorless and transparent PI films with enhanced phosphorescence are strongly demanded.
Herein, we report a highly colorless and transparent PI film exhibiting dual luminescence originating from fluorescence and phosphorescence emission at room temperature (298 K) prepared from a Br-substituted biphenyl tetracarboxylic dianhydride and an alicyclic diamine (DBrBP-PI; Chart 1b). We also synthesized an unsubstituted PI (BP-PI; Chart 1a) to clarify the effects of Br substitution on the luminescence properties. The mechanism and properties of the dual luminescence have been investigated by means of time-resolved emission and steady-state measurements. Furthermore, to clarify the effects of atmospheric oxygen and molecular motions of PI chains, we examined the steady-state photoluminescence properties under vacuum and at low temperatures.
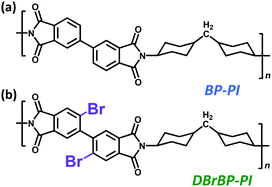 |
| Chart 1 Structures of (a) BP-PI and (b) DBrBP-PI. | |
2. Experimental
2.1. Materials
3,4,3′,4′-Biphenyltetracarboxylic dianhydride (BPDA; compound 1) for the synthesis of DBrBP-PI was provided by Ube Industries Ltd and used as received. For the synthesis of BP-PI, BPDA was used after drying at 180 °C for 10 h under reduced pressure and subsequent sublimation at 280 °C. 4,4′-Diaminocyclohexylmethane (DCHM), purchased from Tokyo Kasei Kogyo Co., Ltd, was used after recrystallization from n-hexane and sublimation at 70 °C under reduced pressure. N,O-Bis(trimethylsilyl)trifluoroacetamide (BSTFA, 99+%) and N,N-dimethylacetamide (DMAc, anhydrous), purchased from Aldrich, were used as received.
2.2. Synthesis
The synthetic scheme of DBrBP-PI and the preparation procedure of the PI film are shown in Scheme 1. Detailed synthetic procedures and characterization data are summarized in the ESI.† 2,2′-Dibromo-4,4′,5,5′-biphenyltetracarboxylic acid (3) was synthesized through the direct bromination of the sodium salt of biphenyltetracarboxylic acid (2), prepared via the hydrolysis of BPDA, and subsequent acidification with hydrochloric acid.74 2,2′-Dibromo-4,4′,5,5′-biphenyltetracarboxylic dianhydride (4) was synthesized by heating compound 3 at 180 °C under reduced pressure, and purified through subsequent sublimation at 240 °C. DBrBP-PI was synthesized using an in situ silylation method reported by Oishi et al.75,76 The precursor of the PI, poly(amic acid) silyl ester (PASE), was prepared by mixing DCHM (alicyclic diamine), BSTFA (silylation agent), and compound 4 in anhydrous DMAc under a N2 atmosphere. The resulting viscous polymer solution was spin-coated onto a fused silica (amorphous SiO2) substrate, and then soft-baked at 70 °C for 1 h; this was followed by thermal imidization at 220 °C for 1.5 h under a N2 flow. The film thickness was estimated to be 4.4 μm.
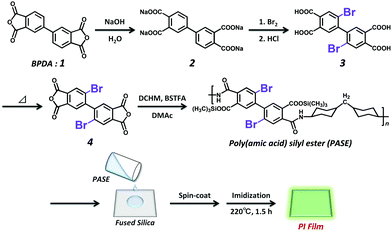 |
| Scheme 1 Synthetic scheme of DBrBP-PI and preparation procedure of PI film. | |
A BP-PI film was prepared in a similar manner to the preparation of the DBrBP-PI film via the reaction of DCHM and BPDA. The film thickness was estimated to be 12.4 μm.
All compounds except for the PIs were characterized using 1H nuclear magnetic resonance (NMR) spectroscopy (Fig. S1 and S2 in the ESI†), elemental analysis, and mass spectrometry, and by measuring the melting points. 13C NMR spectra of each compound could not be measured owing to their poor solubility.
2.3. Measurements
UV-visible (UV-vis) absorption and photoluminescence excitation/emission spectra of the PI films coated on the substrate were measured with a V-760 spectrophotometer (JASCO, Japan) and F-7100 fluorescence spectrometer (Hitachi Hi-Tech, Japan) equipped with an R928 photomultiplier tube (HAMAMATSU, Japan), respectively. Photoluminescence spectra under vacuum conditions were measured using a custom-made vacuum chamber (Akada Industry Co., Ltd, Japan) installed in the same fluorescence spectrometer. Spectra were measured after pumping the chamber out for 30 min using a vacuum pump (PFEIFFER XtraDry 150-2, Germany). Photoluminescence spectra at low temperatures were measured by mounting the samples on a temperature controller (CoolSpek UV USP-203-B, Unisoku Co., Ltd, Japan) installed in the same fluorescence spectrometer. Samples were cooled by circulating liquid nitrogen in the chamber. Photoluminescence quantum efficiencies (Φ) were measured through another method by using a calibrated integrating sphere (HAMAMATSU C9920) connected to a multichannel analyser (HAMAMATSU C7473) via an optical fibre link. Low-temperature Φ values were estimated using the same method, in which samples were immersed in liquid nitrogen in a Dewar vessel made of quartz. The photoluminescence decay kinetics of the PI films were investigated using a fluorescence lifetime spectrometer (HAMAMATSU, Quantaurus-Tau). Decay kinetics under vacuum conditions were also investigated with the same equipment.
Picosecond time-resolved photoluminescence spectra were measured using a streak camera (HAMAMATSU C5680). A femtosecond Yb:KGW oscillator (Light Conversion Ltd, Lithuania) was employed. The oscillator produced 80 fs, 1030 nm light pulses at a repetition rate of 76 MHz, which were frequency tripled to 343 nm (HIRO harmonics generator, Light Conversion Ltd), attenuated, and focused into a ∼100 μm spot on the sample, resulting in an average excitation power of approximately 1 mW mm−2. The maximum time resolution of the entire system was approximately 3.0 ps.
Thermogravimetric analysis (TGA) was conducted using a Shimadzu DTG-60 analyser with a heating rate of 5 °C min−1 under a N2 flow. Thermomechanical analysis (TMA) was conducted using a Shimadzu TMA-60 with a fixed load of 3.0 g and a heating rate of 2 °C min−1 under a N2 flow. Fourier transform infrared (FT-IR) spectra were measured using an Avatar-320 (Thermo-Fisher, USA) spectrometer equipped with a Thunder-dome attenuated total reflection (ATR) attachment (incident angle of 45°). The IRE (internal reflection element) prism was made of a germanium crystal with a refractive index of 4.0. The film thicknesses of the PI films were measured with a probe-pin-type surface profilometer (DEKTAK III, ULVAC Inc., Japan).
2.4. Quantum chemical calculations
The density functional theory (DFT) with the three-parameter Becke-style hybrid functional (B3LYP)77–79 and the 6-311G(d,p) basis set functions was adopted for the geometry optimizations of the model compounds of PIs in the ground state. The time-dependent (TD) DFT with the CAM-B3LYP80 functional and the 6-311++G(d,p) basis set functions was used to calculate the molecular orbitals (MOs), vertical one-electron excitation wavelengths, and oscillator strengths (f) for the optimized geometries. A method to generate calculated absorption spectra of organic compounds was reported elsewhere.81–83 All of the calculations were performed using the Gaussian 09 rev. C.0184 program package installed at the Global Scientific Information and Computing Centre (GSIC), Tokyo Institute of Technology.
3. Results and discussion
3.1. Characterization
The ATR FT-IR spectra of BP-PI and DBrBP-PI films prepared in this study are shown in Fig. S3 in the ESI.† Both PIs showed peaks at around 1360, 1710, and 1770 cm−1, which are assignable to the in-plane stretching of imide C–N bonds and the asymmetric and symmetric stretching of C
O bonds in the imide rings, respectively, clearly indicating the completion of the thermal imidization of the precursor compounds.
The TGA and TMA curves obtained for the BP-PI and DBrBP-PI films are shown in Fig. S4 and S5 in the ESI.† Both PIs demonstrated good thermal stability with high Tg values (BP-PI: 355 °C, DBrBP-PI: 325 °C) and without significant weight loss below 400 °C (decomposition temperatures with 5% weight loss, T5d: BP-PI: 433 °C, DBrBP-PI: 427 °C); this arises from the rigid chemical structures and strong intermolecular interactions of these PIs.
3.2. Optical absorption spectra
Fig. 1a shows the UV-vis absorption spectra of the BP-PI and DBrBP-PI films. The spectra are normalized by the film thicknesses. The absorption band of DBrBP-PI was in a shorter-wavelength region than that of BP-PI. A similar hypochromic shift was also observed comparing absorption spectra of solutions of low-molecular-weight model compounds BP-PI (BP-MC) and DBrBP-PI (DBrBP-MC), which have the same chemical structure as the repeating unit of the corresponding PIs (see Fig. S6 in the ESI†). The geometries of the model compounds in the ground state calculated by the DFT method revealed that DBrBP-MC has a much larger biphenyl dihedral angle of 89° than that of BP-MC (40°) because of the steric hindrances effect of the attached Br atoms85 (Fig. 1b). This implies that DBrBP-MC has a much lower degree of π-conjugation in the biphenyl dianhydride moiety than BP-MC, which is in accordance with the hypochromic shift of the absorption band. Based on these facts, identical hypochromic absorption shift observed for DBrBP-PI should be also attributed to the steric hindrances effect of the attached Br atoms.
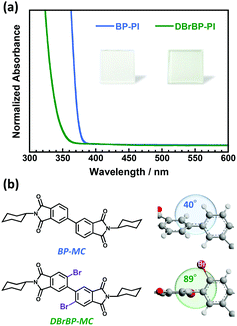 |
| Fig. 1 (a) UV-vis absorption spectra of BP-PI and DBrBP-PI films, and (b) biphenyl dihedral angles of the optimized structures of the model compounds (BP-MC and DBrBP-MC) obtained from DFT calculations. Spectra were normalized by their film thicknesses. | |
No absorption band attributable to the aggregates of the PI chains was observed; this should be because of the intermolecular steric effect of the twisted biphenyl dianhydride structure, which can suppress the formation of aggregates even in the solid film. Both of the PI films exhibited absorption bands only in the UV region (<400 nm), thus, they are perfectly colorless and transparent (see the photos in Fig. 1a).
3.3. Steady-state and time-resolved photoluminescence
Fig. 2a shows the photoluminescence excitation and emission spectra, and Fig. 2b shows the photoluminescence decay curves of the BP-PI film measured at 298 K in ambient air and under vacuum conditions. The BP-PI film showed excitation and emission peaks at 367 and 406 nm, respectively, with a relatively small ν of 2617 cm−1 (see Table 1).23 This small Stokes shift clearly indicates that the emission of BP-PI is attributable to fluorescence from an excited singlet state with limited conformational or electronic changes in the excited state. The photoluminescence measured at 420 nm decayed non-exponentially with the longest delay component of 6.3 ns under atmospheric conditions. This decay behaviour did not change even under vacuum conditions, which proves the typical fluorescent nature of the BP-PI film.
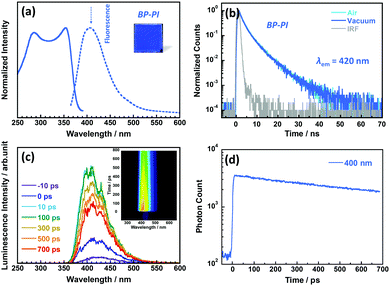 |
| Fig. 2 Photoluminescence properties of the BP-PI film. (a) Emission spectrum measured under excitation at 367 nm and excitation spectrum measured by monitoring the emission intensity at 406 nm. (b) Photoluminescence decay curves at 420 nm under atmospheric and vacuum conditions. The instrument response function (IRF) is shown in gray. (c) Picosecond time-resolved fluorescence spectra at different times measured by using the streak camera under excitation at 343 nm, and (d) fluorescence decay at 400 nm. | |
Table 1 Photoluminescence properties of BP-PI and DBrBP-PI films measured at 298 K in ambient air. Excitation/emission spectra (λex, λem) along with the Stokes shifts (ν) and photoluminescence quantum yields (Φ)
PI |
λ
ex (nm) |
λ
em (nm) |
ν (cm−1) |
Φ
|
Components of fluorescence emission.
Components of phosphorescence emission.
|
BP-PI |
367 |
406 |
2617 |
0.220 |
DBrBP-PI |
338 |
408a |
5076 |
0.002 |
315 |
512b |
12 215 |
0.020 |
The ultrafast time-resolved fluorescence spectra of the BP-PI film investigated by using the streak camera showed a single emission component at around 400 nm with a slow decay (Fig. 2c and d). It showed no significant changes of the spectrum with time, which indicates no fast processes in the excited state, and also shows that the film is remarkably homogeneous. Therefore, the spectral diffusion, often observed in molecular solids, is insignificant for BP-PI.
Fig. 3a shows the photoluminescence excitation and emission spectra, and Fig. 3b shows the photoluminescence decay curves of the DBrBP-PI film measured at 298 K in ambient air and under vacuum conditions. In contrast to BP-PI, the emission spectrum of the DBrBP-PI film consists of two broad components, which can be quantified by fitting using two Gaussian broadening functions (see Fig. S7 in the ESI†). The spectrum is dominated by the prominent emission at 512 nm with a very large Stokes shift (ν = 12
215 cm−1) when excited at 315 nm at 298 K. This emission can be attributed to phosphorescence from an excited triplet state induced by enhanced ISC originating from the heavy atom effect due to Br atoms in the dianhydride moiety. Interestingly, the photoluminescence at 520 nm lasted for more than 1 ms even under atmospheric conditions (Fig. 3b). This long lifetime could be attributable to the rigid skeletal structure of the PI with restricted internal rotation at the biphenyl moiety, which potentially suppresses the local molecular motions inducing non-radiative decay of triplet excitons. In addition, the photoluminescence lifetime was significantly increased under vacuum conditions (Fig. 3b), which indicates that the emission at 512 nm observed in the steady-state spectrum is highly sensitive to atmospheric oxygen, and this fact confirms the attribution of the large-Stokes-shifted emission to the excited triplet state.28 These facts indicate that the introduction of Br atoms to the biphenyltetracarboxylic dianhydride moiety endows the PI with colorlessness with high transparency and large-Stokes-shifted phosphorescence properties, which is unusual not only for polyimides but also for the main-chain-type polymers. Thus, DBrBP-PI is a promising candidate for spectral down-converting materials. An additional emission band was observed for DBrBP-PI at shorter wavelengths around 408 nm (Fig. 3a). This emission is attributable to prompt fluorescence from the excited singlet state without ISC because the band position is very close to the fluorescence peak of BP-PI. The photoluminescence excitation/emission spectra of this band are shown in Fig. S8 in the ESI.† The relatively small Stokes shift (5076 cm−1), observed when excited at 338 nm at 298 K, also supports this view. Consequently the colorless and transparent DBrBP-PI film exhibits dual, fluorescence and prominent phosphorescence emission at room temperature.
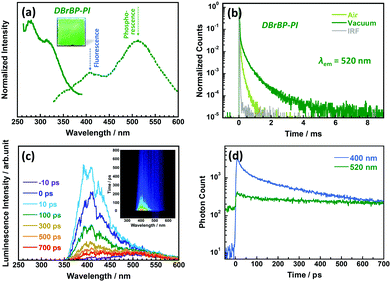 |
| Fig. 3 Photoluminescence properties of the DBrBP-PI film. (a) Emission spectrum measured under excitation at 315 nm and excitation spectrum measured by monitoring emission intensity at 512 nm. (b) Photoluminescence decay curves at 520 nm under atmospheric and vacuum conditions. The instrument response function (IRF) is shown in gray. (c) Picosecond time-resolved photoluminescence spectra at different times and (d) photoluminescence decays at 400 nm and 520 nm measured by the streak camera under excitation at 343 nm. | |
Unlike in the steady-state spectrum, the time-resolved spectrum of DBrBP-PI showed a much stronger fluorescence emission component at around 400 nm (Fig. 3c and d) and only weak phosphorescence spectrum at around 500 nm. The fluorescence decays nonexponentially with the fast decay component of tens of ps during the initial ∼100 ps and the longest time constant of 3 ns. The decay dynamics is identical both under atmospheric and vacuum conditions (see Fig. S9 in the ESI†), which confirms that this emission originates from an oxygen-insensitive excited singlet state. Note that the longest fluorescence decay component at 408 nm of DBrBP-PI (3 ns) was shorter than that of BP-PI (6.3 ns), which should be due to the additional decay pathway of the singlet exciton via the efficient ISC.70 We do not see any significant changes of the fluorescence spectrum during this time, which rules out any conformational changes in the polymer chain. Most likely, the difference in local conformations causes distribution of internal conversion or ISC rates.
As seen in Fig. 3a and Fig. S8 (ESI†), a fluorescent peak of DBrBP-PI was observed at the same position (408 nm) irrespective of the excitation wavelengths (315 and 338 nm). In addition, the peak in Fig. S8 (ESI†) is more intense than in Fig. 3a. Fig. 1a showed that the wavelength of 315 nm is located in the intense π–π* absorption band, whereas that of 338 nm is close to the absorption edge. Fig. S10 in the ESI† shows the calculated absorption spectra of BP-MC and DBrBP-MC, and Fig. S11 in the ESI† displays the calculated one-electron transitions of DBrBP-MC corresponding to the excitations at 315 nm and 336 nm, respectively, where the spatial distributions of MOs with the largest contributions to these transitions are depicted. A small absorption peak of DBrBP-MC appearing at 320 nm is attributable to a π–π* transition having a charge transfer (CT) character between HOMO and LUMO. In the case that the biphenyl dihedral angle is rotated from the optimized geometry (89°) to a slightly planar structure (120°), this peak is shifted to 335 nm with enhancement of its intensity. On the other hand, a stronger peak appearing at 297 nm is attributable to a typical π–π* transition with a larger oscillator strength. These facts strongly suggest that the excitation at 315 nm generates plural locally excited (LE) singlet states at higher energies (Sn) which are readily followed by efficient ISC and internal conversion (IC) to the triplet state (T1). This energy path conduces the intense phosphorescence at 512 nm. On the other hand, PI chains having more planar conformations can be excited at longer wavelengths (typically at 338 nm), and the generated excited state preferentially emits fluorescence rather than phosphorescence. Table S1 in the ESI† shows the experimental fluorescent decay constants (τ) of the DBrBP-PI film excited at different wavelengths. The average time constant (τav) increased with increasing the excitation wavelengths, which indicates that more efficient ISC was induced by excitation at shorter wavelengths. These results accord well with the TD-DFT calculations and the spectral difference between Fig. 3a and Fig. S8 (ESI†).
Moreover, detailed analysis of the kinetics of the growth of the phosphorescence spectrum could give an answer to this question, however its lifetime was too long to investigate the dynamics. The weak phosphorescence emission at around 500 nm was observed for DBrBP-PI in the entire time range (Fig. 3c). Because of the long phosphorescence lifetime, this emission did not decay completely during the 13 ns time period between excitation pulses, resulting in the residual component at negative times. Therefore, the initial increasing process of the phosphorescence intensity, which corresponds to the rate of ISC, could not be observed clearly.
We also measured steady-state emission spectra under vacuum conditions (Fig. 4). The emission intensity and spectral shape of BP-PI did not show significant changes under vacuum (Fig. 4a), which indicates the insensitivity of the excited singlet state to oxygen. In contrast, the emission intensity at around 510 nm of DBrBP-PI was significantly enhanced under vacuum (Fig. 4b). This result indicates that this emission was effectively quenched by the triplet–triplet energy transfer to oxygen under atmospheric conditions, which is typical phosphorescence behavior.70 In the case of the amorphous PI films prepared in this study, atmospheric oxygen exists not only at the surface, but also in the interior free volume of the film. Note that the fluorescence band at around 408 nm showed no significant change under vacuum, which coincides well with the lifetime measurement (Fig. S9, ESI†). Since the DBrBP-PI film exhibited oxygen-sensitive phosphorescence as well as oxygen-insensitive fluorescence at the same time, this material could be applicable to oxygen sensors based on the fluorescence/phosphorescence intensity ratio.70
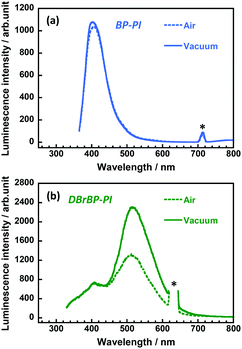 |
| Fig. 4 Photoluminescence emission spectra of (a) BP-PI and (b) DBrBP-PI films under atmospheric and vacuum conditions. Excitation wavelengths were 367 nm for BP-PI and 315 nm for DBrBP-PI. Asterisks indicate second order diffraction of excitation light. | |
3.4. Photoluminescence at low temperatures
In the case of solid amorphous films of semi-aromatic PIs, PI chains undergo local molecular motions at timescales similar to the lifetime of triplet excitons. We have determined the nuclear magnetic spin–lattice relaxation times in the rotating frame (T1ρ) for the hydrogen and fluorine atoms of an amorphous fluorine-containing PI to be 9.2 and 1.8 ms, respectively at 298 K, by using 19F–1H cross-polarization solid-state NMR spectroscopy.86 This experiment indicated that local motions in the order of milliseconds exist extensively in the main chains of such PIs even at temperatures much lower than the glass transition (Tg > 300 °C). The phosphorescence properties could be significantly affected by this molecular motion. To verify this view, we conducted photoluminescence measurements at lower temperatures (Fig. 5). The luminescence intensity of BP-PI increased by 5–6 times at low temperatures, and the Φ value at liquid nitrogen temperature (77 K) was estimated to be 0.51, which is 2.3 times larger than the value at 298 K (Φ = 0.22). Because of the nature of the fluorescence of BP-PI, which has a short photoluminescence lifetime, the fluorescence intensity did not increase significantly at low temperatures because of the low sensitivity to the molecular motions. In contrast, the luminescence intensity of DBrBP-PI at low temperatures was significantly higher than that at 298 K by ca. 45 times. The Φ value at 77 K was estimated to be 0.76, which was 38 times as large as the value at 298 K (Φ = 0.02) and exceeded that of BP-PI at 77 K (Φ = 0.51). This Φ value is close to that of benzophenone dissolved in an organic solvent at 77 K (Φ = ∼0.9), in which the probability of ISC is almost 100%.41 Because of the long lifetime of the phosphorescence of DBrBP-PI, the photoluminescence is significantly influenced by the various types of molecular motions. Note that the fluorescence intensity of DBrBP-PI increased by only three times even at low temperatures; this is because of the short fluorescence lifetime of this emission (Fig. S7 in the ESI†). Since the DBrBP-PI film exhibits intense phosphorescence at low temperatures as well as under deoxygenated conditions, it could be promising for use in space, for example, as a spectral down-converter for space solar cells, since the lowest temperature in the mesosphere (altitude of ca. 80 km from the ground) is around −90 °C.
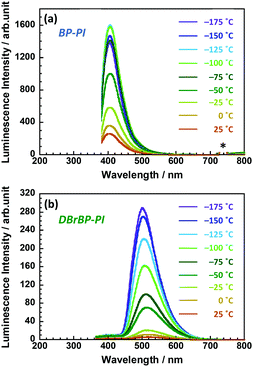 |
| Fig. 5 Photoluminescence emission spectra of (a) BP-PI and (b) DBrBP-PI films at low temperatures. Spectra were measured using excitation wavelengths of 367 nm for BP-PI and 315 nm for DBrBP-PI. The asterisk indicates second order diffraction of excitation light. | |
4. Conclusions
A novel colorless and transparent semi-aromatic PI with a Br-substituted biphenyl-tetracarboxylic dianhydride moiety (DBrBP-PI) was newly designed and synthesized, and its photoluminescence properties were extensively investigated by comparing them with those of an unsubstituted PI (BP-PI). Both the BP-PI and DBrBP-PI films exhibited significantly high thermal stabilities, as indicated by their high T5d values of 433 and 427 °C and high Tg values of 355 and 325 °C, respectively. The thin film of DBrBP-PI exhibited complete colorlessness with high transparency owing to the effective suppression of aggregate formation due to the steric effect of the twisted Br-substituted biphenyl structure. The DBrBP-PI film exhibited very-large-Stokes-shifted phosphorescence (12
215 cm−1) with a long lifetime (0.2 ms) even under atmospheric conditions because of the enhanced ISC arising from the heavy atom effect of the Br atoms as well as the rigid skeletal structure of the PI that effectively suppressed local molecular motions. To our best knowledge, this is the first report of a main-chain-type fully aromatic polymer with room temperature phosphorescence exhibiting both high colorlessness and a very large Stokes shift. An additional emission band was observed at shorter wavelengths around 408 nm, which is attributable to prompt fluorescence from the excited singlet state. Time-resolved photoluminescence spectrum measurements using a streak camera revealed very fast singlet-exciton decay for DBrBP-PI due to the rapid ISC, which was followed by relatively slow phosphorescence emission. The phosphorescence of the DBrBP-PI film was significantly enhanced under vacuum because of the suppression of the triplet–triplet energy transfer to atmospheric oxygen at the surface and in the interior of the PI film. In addition, the phosphorescence intensity increased dramatically at lower temperatures, at which the local molecular motions in the PI film were effectively suppressed, and the Φ value was estimated to be 0.76 at 77 K, which was 38 times as large as that at 298 K. Even though the Φ at 298 K (0.02) is not high enough, this could be enhanced by effective suppression of the local molecular motions. This new type of PI material exhibiting dual fluorescence and phosphorescence emission and high thermal stability can be used as a lightweight and flexible light emitting thin film, applicable to spectral converters in various fields especially in cosmic space.
Conflicts of interest
There are no conflicts to declare.
Acknowledgements
This work was partly supported by Grants-in-Aid for Scientific Research, the Japan Society for the Promotion of Science (25288096, 15K13782, and 17H03112) and the Bilateral Joint Japan-Lithuanian Research Project by Japan Society for the Promotion of Science and Lithuanian Research Council (LJB-17-007). The authors wish to thank Ms Mayuko Nara and Mr Eisuke Fujiwara at Tokyo Institute of Technology for their help in analysing photoluminescence data.
References
- W. G. J. H. M. van Sark, A. Meijerink, R. E. I. Schropp, J. A. M. van Roosmalen and E. H. Lysen, Enhancing solar cell efficiency by using spectral converters, Sol. Energy Mater. Sol. Cells, 2005, 87, 395–409 CrossRef CAS.
- W. G. J. H. M. van Sark, K. W. J. Barnham, L. H. Slooff, A. J. Chatten, A. Büchtemann, A. Meyer, S. J. McCormack, R. Koole, D. J. Farrell, R. Bose, E. E. Bende, A. R. Burgers, T. Budel, J. Quilitz, M. Kennedy, T. Meyer, C. D. M. Donegá, A. Meijerink and D. Vanmaekelbergh, Luminescent Solar Concentrators - A review of recent results, Opt. Express, 2008, 16, 21773 CrossRef CAS.
- R. Reisfeld, New developments in luminescence for solar energy utilization, Opt. Mater., 2010, 32, 850–856 CrossRef CAS.
- M. G. Debije and P. P. C. Verbunt, Thirty Years of Luminescent Solar Concentrator Research: Solar Energy for the Built Environment, Adv. Energy Mater., 2012, 2, 12–35 CrossRef CAS.
- F. Purcell-Milton and Y. K. Gun’ko, Quantum dots for Luminescent Solar Concentrators, J. Mater. Chem., 2012, 22, 16687–16697 RSC.
- X. Huang, S. Han, W. Huang and X. Liu, Enhancing solar cell efficiency: the search for luminescent materials as spectral converters, Chem. Soc. Rev., 2013, 42, 173–201 RSC.
- L. R. Bradshaw, K. E. Knowles, S. McDowall and D. R. Gamelin, Nanocrystals for luminescent solar concentrators, Nano Lett., 2015, 15, 1315–1323 CrossRef CAS.
- F. I. Wu, R. Dodda, K. Jakka, J. H. Huang, C. S. Hsu and C. F. Shu, Enhancing the thermal and spectral stabilities of polyfluorene-based blue-light-emitting materials by incorporating pendent spiro-cycloalkyl groups, Polymer, 2004, 45, 4257–4263 CrossRef CAS.
- Q. Peng, Z. Y. Lu, Y. Huang, M. G. Xie, S. H. Han, J. B. Peng and Y. Cao, Synthesis and characterization of new red-emitting polyfluorene derivatives containing electron-deficient 2-pyran-4-ylidene-malononitrile moieties, Macromolecules, 2004, 37, 260–266 CrossRef CAS.
- D. Vak, J. Jo, J. Ghim, C. Chun, B. Lim, A. J. Heeger and D. Y. Kim, Synthesis and characterization of spiro-triphenylamine configured polyfluorene derivatives with improved hole injection, Macromolecules, 2006, 39, 6433–6439 CrossRef CAS.
- R. Tang, Z. Tan, Y. Li and F. Xi, Synthesis of new conjugated polyfluorene derivatives bearing triphenylamine moiety through a vinylene bridge and their stable blue electroluminescence, Chem. Mater., 2006, 18, 1053–1061 CrossRef CAS.
- C. H. Yang, C. J. Bhongale, C. H. Chou, S. H. Yang, C. N. Lo, T. M. Chen and C. S. Hsu, Synthesis and light emitting properties of sulfide-containing polyfluorenes and their nanocomposites with CdSe nanocrystals: a simple process to suppress keto-defect, Polymer, 2007, 48, 116–128 CrossRef CAS.
- J. Liu, J. Zou, W. Yang, H. Wu, C. Li, B. Zhang, J. Peng and Y. Cao, Highly efficient and spectrally stable blue-light-emitting polyfluorenes containing a dibenzothiophene-S,S-dioxide unit, Chem. Mater., 2008, 20, 4499–4506 CrossRef CAS.
- V. N. Bliznyuk, S. A. Carter, J. C. Scott, G. Klärner, R. D. Miller and D. C. Miller, Electrical and photoinduced degradation of polyfluorene based films and light-emitting devices, Macromolecules, 1999, 32, 361–369 CrossRef CAS.
- S. I. Hintschich, C. Rothe, S. Sinha, A. P. Monkman, P. Scandiucci de Freitas and U. Scherf, Population and decay of keto states in conjugated polymers, J. Chem. Phys., 2003, 119, 12017–12022 CrossRef CAS.
- A. Kobayashi, H. Takahashi, K. Moriguchi, Y. Matsuura, K. Matsukawa and H. Naito, Optical properties of photo-oxidized polyfluorene thin films, Synth. Met., 2003, 135–136, 287–288 CrossRef CAS.
- F. B. Dias, M. Knaapila, A. P. Monkman and H. D. Burrows, Fast and slow time regimes of fluorescence quenching in conjugated polyfluorene-fluorenone random copolymers: the role of exciton hopping and dexter transfer along the polymer backbone, Macromolecules, 2006, 39, 1598–1606 CrossRef CAS.
- L. Liu, S. Tang, M. Liu, Z. Xie, W. Zhang, P. Lu, M. Hanif and Y. Ma, Photodegradation of polyfluorene and fluorene oligomers with alkyl and aromatic disubstitutions, J. Phys. Chem. B, 2006, 110, 13734–13740 CrossRef CAS.
- C. E. Sroog, Polyimides, J. Polym. Sci., Part D: Macromol. Rev., 1976, 11, 161–208 CAS.
- D. Creed, C. E. Hoyle, J. W. Jordan, C. A. Pandey, R. Nagarajan, S. Pankasem, A. M. Peeler and P. Subramanian, Charge transfer states in the photophysics and photochemistry of polyimides and related phthalimides, Macromol. Symp., 1997, 116, 1–13 CrossRef CAS.
-
M. Hasegawa and K. Horie, Photophysics, photochemistry, and optical properties of polyimides, Pergamon, 2001, vol. 26 Search PubMed.
- S. Matsuda, Y. Urano, J. Park, C. Ha and S. Ando, Preparation and Characterization of Organic Electroluminescent Devices Using Fluorescent Polyimides as a Light-Emitting Layer, J. Photopolym. Sci. Technol., 2004, 17, 241–246 CrossRef CAS.
- J. Wakita, H. Sekino, K. Sakai, Y. Urano and S. Ando, Molecular Design, Synthesis, and Properties of Highly Fluorescent Polyimides, J. Phys. Chem. B, 2009, 113, 15212–15224 CrossRef CAS PubMed.
- J. Wakita, S. Inoue, N. Kawanishi and S. Ando, Excited-state intramolecular proton transfer in imide compounds and its application to control the emission colors of highly fluorescent polyimides, Macromolecules, 2010, 43, 3594–3605 CrossRef CAS.
- K. Takizawa, J. Wakita, K. Sekiguchi and S. Ando, Variations in aggregation structures and fluorescence properties of a semialiphatic fluorinated polyimide induced by very high pressure, Macromolecules, 2012, 45, 4764–4771 CrossRef CAS.
- K. Kanosue, R. Augulis, D. Peckus, R. Karpicz, T. Tamulevičius, S. Tamulevičius, V. Gulbinas and S. Ando, Polyimide and Imide Compound Exhibiting Bright Red Fluorescence with Very Large Stokes Shifts via Excited-State Intramolecular Proton Transfer II. Ultrafast Proton Transfer Dynamics in the Excited State, Macromolecules, 2016, 49, 1848–1857 CrossRef CAS.
- K. Kanosue and S. Ando, Fluorescence emissions of imide compounds and end-capped polyimides enhanced by intramolecular double hydrogen bonds, Phys. Chem. Chem. Phys., 2015, 17, 30659–30669 RSC.
- K. Kanosue and S. Ando, Polyimides with Heavy Halogens Exhibiting Room-Temperature Phosphorescence with Very Large Stokes Shifts, ACS Macro Lett., 2016, 5, 1301–1305 CrossRef CAS.
- J. E. Kwon and S. Y. Park, Advanced organic optoelectronic materials: harnessing excited-state intramolecular proton transfer (ESIPT) process, Adv. Mater., 2011, 23, 3615–3642 CrossRef CAS.
- B. S. Richards, Enhancing the performance of silicon solar cells via the application of passive luminescence conversion layers, Sol. Energy Mater. Sol. Cells, 2006, 90, 2329–2337 CrossRef CAS.
- M. J. Currie, J. K. Mapel, T. D. Heidel, S. Goffri and M. A. Baldo, High-efficiency organic solar concentrators for photovoltaics, Science, 2008, 321, 226–228 CrossRef CAS.
- P. F. Scudo, L. Abbondanza, R. Fusco and L. Caccianotti, Spectral converters and luminescent solar concentrators, Sol. Energy Mater. Sol. Cells, 2010, 94, 1241–1246 CrossRef CAS.
- W. G. J. H. M. van Sark, Luminescent solar concentrators – a low cost photovoltaics alternative, Renewable Energy, 2013, 49, 207–210 CrossRef CAS.
- J. Yoon, L. Li, A. V. Semichaevsky, J. H. Ryu, H. T. Johnson, R. G. Nuzzo and J. A. Rogers, Flexible concentrator photovoltaics based on microscale silicon solar cells embedded in luminescent waveguides, Nat. Commun., 2011, 2, 343 CrossRef.
- X. Peng, F. Song, E. Lu, Y. Wang, W. Zhou, J. Fan and Y. Gao, Heptamethine cyanine dyes with a large stokes shift and strong fluorescence: a paradigm for excited-state intramolecular charge transfer, J. Am. Chem. Soc., 2005, 127, 4170–4171 CrossRef CAS.
- G. Haberhauer, R. Gleiter and C. Burkhart, Planarized Intramolecular Charge Transfer: A Concept for Fluorophores with both Large Stokes Shifts and High Fluorescence Quantum Yields, Chem. – Eur. J., 2016, 22, 971–978 CrossRef CAS.
- J. Duhamel, New insights in the study of pyrene excimer fluorescence to characterize macromolecules and their supramolecular assemblies in solution, Langmuir, 2012, 28, 6527–6538 CrossRef CAS.
- Y. Chi and P. T. Chou, Transition-metal phosphors with cyclometalating ligands: fundamentals and applications, Chem. Soc. Rev., 2010, 39, 638–655 RSC.
- S. K. Lower and M. A. El-Sayed, The triplet state and molecular electronic processes in organic molecules, Chem. Rev., 1966, 66, 199–241 CrossRef CAS.
- R. J. Hurtubise, Phosphorimetry: new developments include solid-surface, micelle-stabilized, and solution-sensitized room-temperature phosphorescence, Anal. Chem., 1983, 55, 669A–680A CrossRef CAS.
-
N. J. Turro, V. Ramamurthy and J. C. Scaiano, Modern Molecular Photochemistry of Organic Molecules, University science books, Sausalito, California, 2012, vol. 88 Search PubMed.
- C. S. Bilen, N. Harrison and D. J. Morantz, Unusual room temperature afterglow in some crystalline organic compounds, Nature, 1978, 271, 235–237 CrossRef CAS.
- W. Z. Yuan, X. Y. Shen, H. Zhao, J. W. Y. Lam, L. Tang, P. Lu, C. Wang, Y. Liu, Z. Wang, Q. Zheng, J. Z. Sun, Y. Ma and B. Z. Tang, Crystallization-Induced Phosphorescence of Pure Organic Luminogens at Room Temperature, J. Phys. Chem. C, 2010, 114, 6090–6099 CrossRef CAS.
- G. P. Yong, Y. M. Zhang, W. L. She and Y. Z. Li, Stacking-induced white-light and blue-light phosphorescence from purely organic radical materials, J. Mater. Chem., 2011, 21, 18520–18522 RSC.
- G. Yong, W. She and Y. Zhang, Room-temperature phosphorescence in solution and in solid state from purely organic dyes, Dyes Pigm., 2012, 95, 161–167 CrossRef CAS.
- L. F. Vieira Ferreira, M. R. Vieira Ferreira, A. S. Oliveira and J. C. Moreira, Potentialities of diffuse reflectance laser-induced techniques in solid phase: a comparative study of benzophenone inclusion within p-tert-butylcalixarenes, silicalite and microcrystalline cellulose, J. Photochem. Photobiol., A, 2002, 153, 11–18 CrossRef CAS.
- M. C. Castex, C. Olivero, G. Pichler, D. Adès and A. Siove, Fluorescence, room temperature phosphorescence and photodegradation of carbazole compounds in irradiated poly(methyl methacrylate) matrices, Synth. Met., 2006, 156, 699–704 CrossRef CAS.
- R. Gahlaut, H. C. Joshi, N. K. Joshi, N. Pandey, P. Arora, R. Rautela, K. Suyal and S. Pant, Luminescence characteristics and room temperature phosphorescence of naphthoic acids in polymers, J. Lumin., 2013, 138, 122–128 CrossRef CAS.
- G. P. Yong, B. Zhang, Y. M. Zhang and G. S. Li, Excitation-light-responsive phosphorescent color changes in a β-cyclodextrin inclusion complex, J. Mater. Chem., 2012, 22, 13481–13483 RSC.
- G. Yong, X. Zhang and W. She, Phosphorescence enhancement of organic dyes by forming β-cyclodextrin inclusion complexes: color tunable emissive materials, Dyes Pigm., 2013, 97, 65–70 CrossRef CAS.
- H. A. Al-Attar and A. P. Monkman, Room-temperature phosphorescence from films of isolated water-soluble conjugated polymers in hydrogen-bonded matrices, Adv. Funct. Mater., 2012, 22, 3824–3832 CrossRef CAS.
- S. Hirata, K. Totani, J. Zhang, T. Yamashita, H. Kaji, S. R. Marder, T. Watanabe and C. Adachi, Efficient persistent room temperature phosphorescence in organic amorphous materials under ambient conditions, Adv. Funct. Mater., 2013, 23, 3386–3397 CrossRef CAS.
- S. Hirata, K. Totani, H. Kaji, M. Vacha, T. Watanabe and C. Adachi, Reversible thermal recording media using time-dependent persistent room temperature phosphorescence, Adv. Opt. Mater., 2013, 1, 438–442 CrossRef.
- D. S. Mcclure, Triplet-singlet transitions in organic molecules. Lifetime measurements of the triplet state, J. Chem. Phys., 1949, 17, 905–913 CrossRef CAS.
- J. Xu, A. Takai, Y. Kobayashi and M. Takeuchi, Phosphorescence from a pure organic fluorene derivative in solution at room temperature, Chem. Commun., 2013, 49, 8447–8449 RSC.
- B. Ventura, A. Bertocco, D. Braga, L. Catalano, S. D’Agostino, F. Grepioni and P. Taddei, Luminescence properties of 1,8-naphthalimide derivatives in solution, in their crystals, and in co-crystals: toward room-temperature phosphorescence from organic materials, J. Phys. Chem. C, 2014, 118, 18646–18658 CrossRef CAS.
- S. Mukherjee and P. Thilagar, Recent advances in purely organic phosphorescent materials, Chem. Commun., 2015, 51, 10988–11003 RSC.
- G. D. Gutierrez, G. T. Sazama, T. Wu, M. A. Baldo and T. M. Swager, Red Phosphorescence from Benzo[2,1,3]thiadiazoles at Room Temperature, J. Org. Chem., 2016, 81, 4789–4796 CrossRef CAS.
- S. d’Agostino, F. Grepioni, D. Braga and B. Ventura, Tipping the balance with the aid of stoichiometry: room temperature phosphorescence versus fluorescence in organic cocrystals, Cryst. Growth Des., 2015, 15, 2039–2045 CrossRef.
- H. Wang, R. X. Hu, X. Pang, H. Y. Gao and W. J. Jin, The phosphorescent co-crystals of 1,4-diiodotetrafluorobenzene and bent 3-ring-N-heterocyclic hydrocarbons by C-I⋯N and C-I⋯π halogen bonds, CrystEngComm, 2014, 16, 7942–7948 RSC.
- H. Shi, Z. An, P. Z. Li, J. Yin, G. Xing, T. He, H. Chen, J. Wang, H. Sun, W. Huang and Y. Zhao, Enhancing Organic Phosphorescence by Manipulating Heavy-Atom Interaction, Cryst. Growth Des., 2016, 16, 808–813 CrossRef CAS.
- O. Bolton, K. Lee, H. J. Kim, K. Y. Lin and J. Kim, Activating efficient phosphorescence from purely organic materials by crystal design, Nat. Chem., 2011, 3, 205–210 CrossRef CAS.
- D. Lee, O. Bolton, B. C. Kim, J. H. Youk, S. Takayama and J. Kim, Room temperature phosphorescence of metal-free organic materials in amorphous polymer matrices, J. Am. Chem. Soc., 2013, 135, 6325–6329 CrossRef CAS PubMed.
-
M. S. Kwon, D. Lee, S. Seo, J. Jung and J. Kim, Tailoring Intermolecular Interactions for Efficient Room-Temperature Phosphorescence from Purely Organic Materials in Amorphous Polymer Matrices, Angew. Chem., Int. Ed., 2014, 53, 11177–11181 Search PubMed.
- O. Bolton, D. Lee, J. Jung and J. Kim, Tuning the photophysical properties of metal-free room temperature organic phosphors via compositional variations in bromobenzaldehyde/dibromobenzene mixed crystals, Chem. Mater., 2014, 26, 6644–6649 CrossRef CAS.
- M. S. Kwon, Y. Yu, C. Coburn, A. W. Phillips, K. Chung, A. Shanker, J. Jung, G. Kim, K. Pipe, S. R. Forrest, J. H. Youk, J. Gierschner and J. Kim, Suppressing molecular motions for enhanced roomerature phosphorescence of metal-free organic materials, Nat. Commun., 2015, 6, 8947 CrossRef.
- D. Lee, X. Ma, J. Jung, E. J. Jeong, H. Hashemi, A. Bregman, J. Kieffer and J. Kim, The effects of extended conjugation length of purely organic phosphors on their phosphorescence emission properties, Phys. Chem. Chem. Phys., 2015, 17, 19096–19103 RSC.
- D. Lee, J. Jung, D. Bilby, M. S. Kwon, J. Yun and J. Kim, A novel optical ozone sensor based on purely organic phosphor, ACS Appl. Mater. Interfaces, 2015, 7, 2993–2997 CrossRef CAS PubMed.
- H. Chen, X. Yao, X. Ma and H. Tian, Amorphous, Efficient, Room-Temperature Phosphorescent Metal-Free Polymers and Their Applications as Encryption Ink, Adv. Opt. Mater., 2016, 4, 1397–1401 CrossRef CAS.
- G. Zhang, G. M. Palmer, M. W. Dewhirst and C. L. Fraser, A dual-emissive-materials design concept enables tumour hypoxia imaging, Nat. Mater., 2009, 8, 747–751 CrossRef CAS PubMed.
- W. A. Morris, T. Liu and C. L. Fraser, Mechanochromic luminescence of halide-substituted difluoroboron β-diketonate dyes, J. Mater. Chem. C, 2015, 3, 352–363 RSC.
- C. A. DeRosa, C. Kerr, Z. Fan, M. Kolpaczynska, A. S. Mathew, R. E. Evans, G. Zhang and C. L. Fraser, Tailoring Oxygen Sensitivity with Halide Substitution in Difluoroboron Dibenzoylmethane Polylactide Materials, ACS Appl. Mater. Interfaces, 2015, 7, 23633–23643 CrossRef CAS PubMed.
- C. A. DeRosa, J. Samonina-Kosicka, Z. Fan, H. C. Hendargo, D. H. Weitzel, G. M. Palmer and C. L. Fraser, Oxygen sensing difluoroboron dinaphthoylmethane polylactide, Macromolecules, 2015, 48, 2967–2977 CrossRef CAS PubMed.
- F. W. Harris, S. H. Lin, F. Li and S. Z. D. Cheng, Organo-soluble polyimides: synthesis and polymerization of 2,2′-disubstituted-4,4′,5,5′-biphenyltetracarboxylic dianhydrides, Polymer, 1996, 37, 5049–5057 CrossRef CAS.
- Y. Oishi, W. Miura, H. Hirahara and K. Mori, Synthesis and Properties of Aliphatic-Aromatic Polyimides from N-Silylated Hexamethylenediamine, J. Photopolym. Sci. Technol., 1999, 217–220 CrossRef CAS.
- Y. Watanabe, Y. Sakai, M. Ueda, Y. Oishi and K. Mori, Synthesis of Wholly Alicyclic Polyimides from N-Silylated Alicyclic Diamines, Chem. Lett., 2000, 450–451 CrossRef CAS.
- C. Lee, W. Yang and R. G. Parr, Development of the Colle-Salvetti correlation-energy formula into a functional of the electron density, Phys. Rev. B: Condens. Matter Mater. Phys., 1988, 37, 785–789 CrossRef CAS.
- A. D. Becke, Density-functional thermochemistry. III. The role of exact exchange, J. Chem. Phys., 1993, 98, 5648–5652 CrossRef CAS.
- P. J. Stephens, F. J. Devlin, C. F. Chabalowski and M. J. Frisch, Ab Initio calculation of vibrational absorption and circular dichroism spectra using density functional force fields, J. Phys. Chem., 1994, 98, 11623–11627 CrossRef CAS.
- T. Yanai, D. P. Tew and N. C. Handy, A new hybrid exchange-correlation functional using the Coulomb-attenuating method (CAM-B3LYP), Chem. Phys. Lett., 2004, 393, 51–57 CrossRef CAS.
- S. Ando, T. Fujigaya and M. Ueda, Density Functional Theory Calculations of Photoabsorption Spectra of Organic Molecules in the Vacuum Ultraviolet Region, Jpn. J. Appl. Phys., 2002, 41, L105–L108 CrossRef CAS.
- S. Ando, T. Fujigaya and M. Ueda, DFT Calculations of Photoabsorption Spectra in the VUV Region for Design of Photoresist Materials for 157 nm Lithography, J. Photopolym. Sci. Technol., 2002, 15, 559–568 CrossRef CAS.
- S. Ando and M. Ueda, DFT Calculations of Photoabsorption Spectra for Alicyclic and Heterocyclic Compounds in the VUV Region, J. Photopolym. Sci. Technol., 2003, 16, 537–544 CrossRef CAS.
-
M. J. Frisch, G. W. Trucks, H. B. Schlegel, G. E. Scuseria, M. A. Robb, J. R. Cheeseman, G. Scalmani, V. Barone, B. Mennucci, G. A. Petersson, H. Nakatsuji, M. Caricato, X. Li, H. P. Hratchian, A. F. Izmaylov, J. Bloino, G. Zheng, J. L. Sonnenberg, M. Hada, M. Ehara, K. Toyota, R. Fukuda, J. Hasegawa, M. Ishida, T. Nakajima, Y. Honda, O. Kitao, H. Nakai, T. Vreven, J. A. Montgomery Jr., J. E. Peralta, F. Ogliaro, M. Bearpark, J. J. Heyd, E. Brothers, K. N. Kudin, V. N. Staroverov, R. Kobayashi, J. Normand, K. Raghavachari, A. Rendell, J. C. Burant, S. S. Iyengar, J. Tomasi, M. Cossi, N. Rega, J. M. Millam, M. Klene, J. E. Knox, J. B. Cross, V. Bakken, C. Adamo, J. Jaramillo, R. Gomperts, R. E. Stratmann, O. Yazyev, A. J. Austin, R. Cammi, C. Pomelli, J. W. Ochterski, R. L. Martin, K. Morokuma, V. G. Zakrzewski, G. A. Voth, P. Salvador, J. J. Dannenberg, S. Dapprich, A. D. Daniels, Ö. Farkas, J. B. Foresman, J. V. Ortiz, J. Cioslowski and D. J. Fox, Gaussian Inc., Wallingford CT, 2009.
- Z. Zhang, Y. S. Wu, K. C. Tang, C. L. Chen, J. W. Ho, J. Su, H. Tian and P. T. Chou, Excited-State Conformational/Electronic Responses of Saddle-Shaped N, N ′-Disubstituted-Dihydrodibenzo[a, c]phenazines: Wide-Tuning Emission from Red to Deep Blue and White Light Combination, J. Am. Chem. Soc., 2015, 137, 8509–8520 CrossRef CAS PubMed.
- S. Ando, R. K. Harris and S. A. Reinsberg, Analysis of Cross-Polarization Dynamics between Two Abundant Nuclei, 19F and 1H, Based on Spin Thermodynamics Theory, J. Magn. Reson., 1999, 141, 91–103 CrossRef CAS PubMed.
Footnote |
† Electronic supplementary information (ESI) available: Synthesis of compounds with 1H NMR spectra; ATR FT-IR spectra, TGA curves, and TMA curves of the BP-PI and DBrBP-PI films; UV-vis absorption spectra of BP-MC and DBrBP-MC in CHCl3; spectral fitting of the emission spectrum of DBrBP-PI; excitation/emission spectra of DBrBP-PI; photoluminescence decay curve of the DBrBP-PI film; calculated absorption spectra of model compounds; calculated one-electron transitions of DBrBP-PI; fluorescence decay time of DBrBP-PI with different excitation wavelengths. See DOI: 10.1039/c8qm00409a |
|
This journal is © the Partner Organisations 2019 |
Click here to see how this site uses Cookies. View our privacy policy here.