DOI:
10.1039/C8QO01048J
(Research Article)
Org. Chem. Front., 2019,
6, 36-40
Photoinduced synthesis of allylic sulfones using potassium metabisulfite as the source of sulfur dioxide†
Received
29th September 2018
, Accepted 8th November 2018
First published on 8th November 2018
Abstract
Synthesis of allylic sulfones through a photoinduced three-component reaction of aryl/alkyl halides, potassium metabisulfite, and allylic bromides under ultraviolet irradiation at room temperature is developed. Diverse allylic sulfones are generated in moderate to good yields without the addition of any metals or photoredox catalysts. Different functional groups, including amino, ester, cyano, trifluoromethyl, and chloro are compatible under the conditions. Not only aryl halides but also alkyl halides are workable in the transformation. During this process, the natural abundant potassium metabisulfite is used as the source of sulfur dioxide. A plausible mechanism which involves the photoinduced C–X bond dissociation, sulfur dioxide fixation, and addition of the sulfonyl radical to allylic bromide is proposed.
Introduction
Allylic sulfones as important units are found in numerous biologically active compounds,1 such as antibacterial agents,1a anticancer agents,1b herbicides,1cetc. Moreover, due to the unique chemical properties of the R-SO2-moiety, allylic sulfones have been applied broadly as synthetic precursors in many useful transformations.2 Therefore, continuous interest is focused on the development of synthetic methodologies towards the synthesis of various allylic sulfones, and many reports have been reported recently.3–6 Among them, there are mainly three reaction types between sulfonyl precursors and allylic substrates, including nucleophilic addition,3 nucleophilic substitution,4 and radical addition.5 The sulfone precursors are restricted to sulfinic salts, sulfinic acids, arylsulfonylmethyl isocyanides, arylsulfonyl hydrazides or their derivatives. However, the generation of pre-installed sulfonyl compounds usually suffered from harsh reaction conditions and the odorous thiol substrates. Additionally, metal catalysts, such as Pd, Ir, Fe, etc., or additives are always needed in the transformations. Thus, it is highly desirable to develop easy and effective methods for the synthesis of allylic sulfones.
In the past decade, continuous interest has been focused on the synthesis of sulfonyl compounds through the insertion of sulfur dioxide.7–9 Among the approaches, we have discovered that arylsulfonyl radicals generated from aryldiazonium tetrafluoroborates and the sulfur dioxide surrogate DABCO·(SO2)2 or inorganic sulfites could be easily accessed under catalyst- and additive-free conditions.9 The sulfonyl radicals could be effectively utilized for the preparation of various sulfonyl-containing compounds under mild conditions. However, the drawback associated with this approach is the scope limitation, since alkyldiazonium tetrafluoroborates are extremely unstable. Thus, only arylsulfonyl compounds could be produced, and we had to look for an alternative route for the synthesis of alkylsulfonyl compounds.
In the meantime, it is well established that aryl/alkyl halides are easily available and applied broadly in transition metal-catalyzed coupling reactions. The only concern is the β-H elimination during the reaction process when alkyl halides are employed. It would be ideal if aryl/alkyl halides could be used as the source of aryl/alkyl radicals under metal-free conditions. Inspired by these results, we envisioned that sulfur dioxide could be incorporated into the transformation. Thus, aryl/alkyl sulfonyl radicals would be afforded from aryl/alkyl halides with sulfur dioxide. It would provide a general and efficient method for the generation of aryl/alkyl sulfonyl compounds through the insertion of sulfur dioxide. Additionally, compared with the transition metal-catalyzed coupling reactions, the β-H elimination would be avoided when alkyl halides are employed in the transformation.
It was reported that aryl/alkyl radicals could be produced from the corresponding aryl/alkyl halides through photoinduced C–X bond dissociation under ultraviolet irradiation.10,11 Since aryl/alkyl halides are easily available, the route to aryl/alkyl sulfonyl radicals from the corresponding aryl/alkyl halides with the insertion of sulfur dioxide would be attractive and promising. Inspired by these results, we conceived that the construction of allylic sulfones through aryl–X or alkyl–X bond dissociation under ultraviolet irradiation with the insertion of sulfur dioxide would be feasible (Scheme 1). We hypothesized that allylic sulfones 3 could be prepared through a metal-free reaction of aryl/alkyl halides 1, sulfur dioxide, and allylic bromides 2. We assumed that under ultraviolet irradiation, the C–X bond of aryl/alkyl halide 1 would be dissociated to provide the corresponding aryl/alkyl radical. The aryl/alkyl radical would be trapped by sulfur dioxide, giving rise to aryl/alkyl sulfonyl radical A. Subsequently, allylic bromide 2 would be involved to capture the aryl/alkyl sulfonyl radical A, leading to radical intermediate B. Further release of the bromine radical would afford the corresponding allylic sulfone 3. On the basis of this hypothesis, we therefore started to explore the practicability of this proposed route.
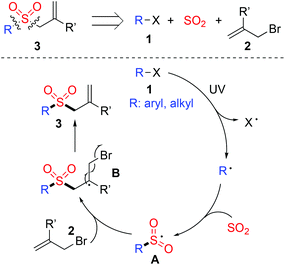 |
| Scheme 1 A proposed radical strategy for the synthesis of allylic sulfones. | |
Results and discussion
Since inorganic sulfites are abundant in nature, potassium metabisulfite was selected as the source of sulfur dioxide.12 Initially, a three-component reaction of 1-iodo-4-methylbenzene 1a, potassium metabisulfite, and 3-bromo-2-methylprop-1-ene 2a was carried out as a model to explore the optimal reaction parameters under ultraviolet irradiation. The results are summarized in Table 1. At the beginning, the reaction was performed in toluene at room temperature under ultraviolet irradiation overnight. To our delight, the expected 1-methyl-4-((2-methylallyl)sulfonyl)benzene 3a was obtained in 48% yield (Table 1, entry 1). The yield was dramatically decreased to 26% when toluene was replaced by 1,4-dioxane (Table 1, entry 2). The result could not be improved when the solvent was changed to acetonitrile or 1,2-dichloroethane (Table 1, entries 3 and 4). Gratifyingly, the yield was increased to 58% by using 3.0 equivalents of potassium metabisulfite (Table 1, entry 5). We assumed that the presence of potassium metabisulfite would act as a reductant as well during the reaction process, which assisted the conversion of the iodine radical and bromine radical to the corresponding anions. Similar results were provided with the further examination of other reductants instead of potassium metabisulfite (Table 1, entries 6–9). Investigations were continued by screening different ratios of the substrates. An inferior result was observed when the ratio of substrates 1a/2a/K2S2O5 was tuned into 1
:
2
:
3 (Table 1, entry 10). Increasing the amount of 1-iodo-4-methylbenzene 1a (2.5 or 3.0 equiv.) could give rise to a higher yield (Table 1, entries 11 and 12). Additionally, the sulfur dioxide surrogate of DABCO·(SO2)2 was used as a replacement for potassium metabisulfite in the reaction of 1-iodo-4-methylbenzene 1a with 3-bromo-2-methylprop-1-ene 2a under ultraviolet irradiation in toluene. The reaction worked efficiently as well, leading to the corresponding product in 70% yield. Since potassium metabisulfite is cheaper and more convenient, we envisioned that using potassium metabisulfite as the source of sulfur dioxide would be more attractive and interesting.
Table 1 Initial studies for the reaction of 1-iodo-4-methylbenzene 1a, K2S2O5, and 3-bromo-2-methylprop-1-ene 2aa

|
Entry |
Additive |
Solvent |
K2S2O5 (equiv.) |
Yieldb (%) |
Reaction conditions: 1-Iodo-4-methylbenzene 1a (0.8 mmol), 3-bromo-2-methylprop-1-ene 2a (0.4 mmol), K2S2O5 (0.8 mmol), additive (0.4 mmol), solvent (4.0 mL), N2, overnight.
Isolated yield based on 3-bromo-2-methylprop-1-ene 2a.
The reaction was performed with 1-iodo-4-methylbenzene 1a (0.4 mmol) and 3-bromo-2-methylprop-1-ene 2a (0.8 mmol).
In the presence of 1-iodo-4-methylbenzene 1a (1.2 mmol).
In the presence of 1-iodo-4-methylbenzene 1a (1.0 mmol).
|
1 |
— |
Toluene |
2.0 |
48 |
2 |
— |
1,4-Dioxane |
2.0 |
26 |
3 |
— |
MeCN |
2.0 |
46 |
4 |
— |
DCE |
2.0 |
46 |
5 |
— |
Toluene |
3.0 |
58 |
6 |
1,4-Cyclohexadiene |
Toluene |
2.0 |
57 |
7 |
GAMMA-terpinene |
Toluene |
2.0 |
52 |
8 |
Ph(Me)2SiH |
Toluene |
2.0 |
58 |
9 |
K2SO3 |
Toluene |
2.0 |
56 |
10c |
— |
Toluene |
3.0 |
38 |
11d |
— |
Toluene |
3.0 |
73 |
12e |
— |
Toluene |
3.0 |
72 |
With the above-mentioned optimal conditions in hand, the scope of the radical coupling reaction of alkyl/aryl halides 1, potassium metabisulfite and allylic bromides 2 was then evaluated. Various alkyl/aryl halides 1 reacted well with potassium metabisulfite and allylic bromides 2, leading to the desired allylic sulfones in moderate to good yields (Table 2). Aryl halides with different groups on the aromatic ring were compatible in this transformation. For example, the cyano-substituted and ester-substituted products 3g and 3h were obtained in 56% and 75% yields, respectively. The amino- and trifluoromethyl-substituted product 3m was generated in 46% yield. However, aryl chloride was inert under the standard conditions, giving rise to the chloro-substituted allylic sulfones 3d in 67% yield. Notably, the reactions of both 1° and 2° alkyl iodides proceeded effectively to afford the corresponding products (3n–p). Subsequently, the reactions of several allylic bromides, including 3-bromoprop-1-ene, methyl 2-(bromomethyl)acrylate and (3-bromoprop-1-en-2-yl)benzene with different aryl iodides attached with electron-donating groups or electron-withdrawing groups were investigated. The conversion occurred well to produce the corresponding products.
Table 2 Scope exploration for the reaction of aryl/alkyl iodide 1, K2S2O5, and allylic bromide 2a
Isolated yield based on 3-bromo-2-methylprop-1-ene 2.
|
|
Additionally, 4-methoxyphenyl bromide 4 was employed in the reaction of potassium metabisulfite with 3-bromo-2-methylprop-1-ene 2a under the above-mentioned conditions (Scheme 2). This reaction proceeded smoothly as well, giving rise to the desired product 3i in 50% yield.
 |
| Scheme 2 Reaction of 4-methoxyphenyl bromide 4 and potassium metabisulfite with 3-bromo-2-methylprop-1-ene 2a. | |
As mentioned above, the C-central radical would be generated through C–X bond dissociation under ultraviolet irradiation. We postulated that the reaction would proceed through a radical process with the insertion of sulfur dioxide. Therefore, 3.0 equivalents of 2,2,6,6-tetramethylpiperidine-1-oxyl (TEMPO) was added to the reaction of 1-iodo-4-methylbenzene 1a, potassium metabisulfite, and 3-bromo-2-methylprop-1-ene 2a under optimal conditions. As expected, the transformation was completely inhibited (Scheme 3, eqn (a)). Additionally, the cyclized product 6 was obtained in 57% yield when 1-(allyloxy)-2-iodobenzene 5 was used in the reaction of potassium metabisulfite, and 3-bromo-2-methylprop-1-ene 2a (Scheme 3, eqn (b)). This result also confirmed that a radical process would be involved.
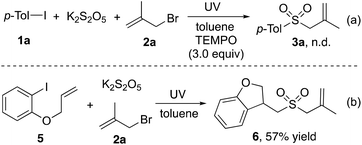 |
| Scheme 3 Investigation of the mechanism. | |
Conclusions
In conclusion, we have reported the synthesis of allylic sulfones through a photoinduced three-component reaction of aryl/alkyl halides, potassium metabisulfite, and allylic bromides under ultraviolet irradiation at room temperature. Diverse allylic sulfones are generated in moderate to good yields without the addition of any metals or photoredox catalysts. Different functional groups including amino, ester, cyano, trifluoromethyl, and chloro are compatible under the conditions. Not only aryl halides but also alkyl halides are workable in the transformation. During this process, the natural abundant potassium metabisulfite is used as the source of sulfur dioxide. A plausible mechanism which involves the photoinduced C–X bond dissociation, sulfur dioxide fixation, and addition of the sulfonyl radical to allylic bromide is proposed.
Conflicts of interest
There are no conflicts to declare.
Acknowledgements
Financial support from the National Natural Science Foundation of China (No. 21672037 and 21871053) and the China Postdoctoral Science Foundation (2018M630396) is gratefully acknowledged.
Notes and references
-
(a) F. Reck, F. Zhou, M. Girardot, G. Kern, C. J. Eyermann, N. J. Hales, R. R. Ramsay and M. B. Gravestock, J. Med. Chem., 2005, 48, 499 CrossRef CAS;
(b)
N. Neamati, G. W. Kabalka, B. Venkataiah and R. Dayam, US patent0203224, 2007 Search PubMed;
(c)
M. C. Gershengorn, S. Neumann, C. J. Thomas, H. Jaeschke, S. Moore, G. Krause, B. Raaka, R. Paschke and G. Kleinau, US patent0203716, 2009 Search PubMed;
(d)
M. Muehlebach, W. Lutz;J. Wenger;J. Finney and C. J. Mathews and D. Fawke, WO patent, 110308, 2008 Search PubMed;
(e) H. G. F. Richter, P. Angehrn, C. Hubschwerlen, M. Kania, M. G. P. Page, J.-L. Specklin and F. K. Winkler, J. Med. Chem., 1996, 39, 3712 CrossRef CAS;
(f) C. Pabba, B. T. Gregg, D. B. Kitchen, Z. Jia Chen and A. Judkins, Bioorg. Med. Chem. Lett., 2011, 21, 324 CrossRef CAS.
-
(a)
N. S. Simpkins, Sulfones in Organic Synthesis, Pergamon Press, New York, 1993 Search PubMed;
(b) A. EI-Awa, M. N. Noshi, X. M. du Jourdin and P. L. Fuchs, Chem. Rev., 2009, 109, 2315 CrossRef;
(c) A. Hassner, E. Ghera, T. Yechezkel, V. Kleiman, T. Balasubramanian and D. Ostercamp, Pure Appl. Chem., 2000, 72, 1671 CAS.
- For nucleophilic addition for allylic sulfones, see:
(a) G. W. Kabalka, B. Venkataiah and G. Dong, Tetrahedron Lett., 2003, 44, 4673 CrossRef CAS;
(b) S. Chandrasekhar, B. Saritha, V. Jagadeshwar, C. Narsihmulu, D. Vijay, G. D. Sarma and B. Jagadeesh, Tetrahedron Lett., 2006, 47, 2981 CrossRef CAS;
(c) V. Garima, P. Srivastava, L. Dhar and S. Yadav, Tetrahedron Lett., 2011, 52, 4622 CrossRef CAS;
(d) X.-Q. Chu, H. Meng, X.-P. Xu and S.-J. Ji, Chem. – Eur. J., 2015, 21, 11359 CrossRef CAS;
(e) B. M. Trost, M. J. Krische, R. Radinov and G. Zanoni, J. Am. Chem. Soc., 1996, 118, 6297 CrossRef CAS;
(f) L. Jiang, T.-G. Li, J.-F. Zhou, Y.-M. Chuan, H.-L. Li and M.-L. Yuan, Molecules, 2015, 20, 8213 CrossRef CAS;
(g) C.-R. Liu, M.-B. Li, D.-J. Cheng, C.-F. Yang and S.-K. Tian, Org. Lett., 2009, 11, 2543 CrossRef CAS;
(h) M. Ueda and J. F. Hartwig, Org. Lett., 2010, 12, 92 CrossRef CAS;
(i) L. Rajender Reddy, B. Hu, M. Prashad and K. Prasad, Angew. Chem., Int. Ed., 2009, 48, 172 CrossRef;
(j) E. Jacobsen, M. K. Chavda, K. M. Zikpi, S. L. Waggoner, D. J. Passini, J. A. Wolfe, R. Larson, C. Beckley, C. G. Hamaker and S. R. Hitchcock, Tetrahedron Lett., 2017, 58, 3073 CrossRef CAS.
- For nucleophilic substitution for allylic sulfones, see:
(a) B. M. Trost and N. R. Schmuff, J. Am. Chem. Soc., 1985, 107, 396 CrossRef CAS;
(b) M. Jegelka and B. Plietker, Chem. – Eur. J., 2011, 17, 10417 CrossRef CAS;
(c) X.-S. Wu, Y. Chen, M.-B. Li, M.-G. Zhou and S.-K. Tian, J. Am. Chem. Soc., 2012, 134, 14694 CrossRef CAS;
(d) S. Chandrasekhar, V. Jagadeshwar, B. Saritha and C. Narsihmulu, J. Org. Chem., 2005, 70, 6506 CrossRef CAS;
(e) F.-X. Felpin and Y. Landais, J. Org. Chem., 2005, 70, 6441 CrossRef CAS PubMed;
(f) H.-H. Li, D.-J. Dong, Y.-H. Jin and S.-K. Tian, J. Org. Chem., 2009, 74, 9501 CrossRef CAS;
(g) M. Jegelka and B. Plietker, Org. Lett., 2009, 11, 3462 CrossRef CAS;
(h) M. Billamboz, F. Mangin, N. Drillaud, C. Chevrin-Villette, E. Banaszak-Léonard and C. Len, J. Org. Chem., 2014, 79, 493 CrossRef CAS;
(i) X.-T. Ma, R.-H. Dai, J. Zhang, Y. Gu and S.-K. Tian, Adv. Synth. Catal., 2014, 356, 2984 CrossRef CAS;
(j) T.-T. Wang, F.-X. Wang, F.-L. Yang and S.-K. Tian, Chem. Commun., 2014, 50, 3802 RSC;
(k) M.-Y. Chang, H.-Y. Chen and H.-S. Wang, J. Org. Chem., 2017, 82, 10601 CrossRef CAS.
- For radical addition for allylic sulfones, see:
(a) X. Lei, L. Zheng, C. Zhang, X. Shi and Y. Chen, J. Org. Chem., 2018, 83, 1772 CrossRef CAS;
(b) X. Li, X. Xu and Y. Tang, Org. Biomol. Chem., 2013, 11, 1739 RSC;
(c) G. Zhang, L. Zhang, H. Yi, Y. Luo, X. Qi, C.-H. Tung, L.-Z. Wu and A. Lei, Chem. Commun., 2016, 52, 10407 RSC;
(d) R. Mao, Z. Yuan, R. Zhang, Y. Ding, X. Fan and J. Wu, Org. Chem. Front., 2016, 3, 1498 RSC;
(e) S. Parisotto, G. Garreffa, C. Canepa, E. Diana, F. Pellegrino, E. Priola, C. Prandi, V. Maurino and A. Deagostino, ChemPhotoChem, 2017, 1, 56 CrossRef CAS;
(f) X. Li, X. Xu and C. Zhou, Chem. Commun., 2012, 48, 12240 RSC;
(g) L. Kadari, R. K. Palakodety and L. P. Yallapragada, Org. Lett., 2017, 19, 2580 CrossRef CAS.
- For other types for allylic sulfones, see:
(a) S. Kamjio, M. Al-Masum and Y. Yamanoto, Tetrahedron Lett., 1998, 39, 691 CrossRef;
(b) H. M. Sheldrake and T. W. Wallace, Tetrahedron Lett., 2007, 48, 4407 CrossRef CAS;
(c) P.-X. Zhou, Y.-Y. Ye, L.-B. Zhao, J.-Y. Hou, X. Kang, D.-Q. Chen, Q. Tang, J.-Y. Zhang, Q.-X. Huang, L. Zheng, J.-W. Ma, P.-F. Xu and Y.-M. Liang, Chem. – Eur. J., 2014, 20, 16093 CrossRef CAS;
(d) K. Xu, V. Khakyzadeh, T. Bury and B. Breit, J. Am. Chem. Soc., 2014, 136, 16124 CrossRef CAS;
(e) A. B. Pritzius and B. Breit, Angew. Chem., Int. Ed., 2015, 54, 3121 CrossRef CAS;
(f) J. Li, G. Qin, Y. Liu and H. Huang, Org. Chem. Front., 2016, 3, 259 RSC;
(g) C.-J. Lu, H. Chen, D.-K. Chen, H. Wang, Z.-P. Yang, J. Gao and H. Jin, Org. Biomol. Chem., 2016, 14, 10833 RSC;
(h) J. E. Gómez, W. Guo and A. W. Kleij, Org. Lett., 2016, 18, 6042 CrossRef;
(i) J. Liao, W. Guo, Z. Zhang, X. Tang, W. Wu and H. Jiang, J. Org. Chem., 2016, 81, 1304 CrossRef CAS;
(j) V. Khakyzadeh, Y.-H. Wang and B. Breit, Chem. Commun., 2017, 53, 4966 RSC;
(k) Y. Hou, Q. Shen, L. Zhu, Y. Han, Y. Zhao, M. Qin and P. Gong, RSC Adv., 2017, 7, 50372 RSC;
(l) P.-X. Zhou, Y. Zhang, C. Ge, Y.-M. Liang and C. Li, J. Org. Chem., 2018, 83, 4762 CrossRef CAS PubMed;
(m) C. Liu and Q. Wang, Angew. Chem., Int. Ed., 2018, 57, 4727 CrossRef CAS PubMed;
(n) F. Xiao, C. Liu, D. Wang, H. Huang and G.-J. Deng, Green Chem., 2018, 20, 973 RSC;
(o) A. Stikute, J. Luginina and M. Turks, Tetrahedron Lett., 2017, 58, 2727 CrossRef CAS;
(p) X. Liu, X. Chen and J. T. Mohr, Org. Lett., 2015, 17, 3572 CrossRef CAS;
(q) C. Kokotos, E. Voutyritsa and I. Triandafillidi, Synthesis, 2016, 49, 917 CrossRef.
- For reviews, see:
(a) G. Qiu, K. Zhou, L. Gao and J. Wu, Org. Chem. Front., 2018, 5, 691 RSC;
(b) K. Hofman, N. Liu and G. Manolikakes, Chem. – Eur. J., 2018, 24, 11852 CrossRef CAS;
(c)
D. Zheng and J. Wu, Sulfur Dioxide Insertion Reactions for Organic Synthesis, Nature Springer, Berlin, 2017 CrossRef;
(d) G. Liu, C. Fan and J. Wu, Org. Biomol. Chem., 2015, 13, 1592 RSC;
(e) E. J. Emmett and M. C. Willis, Asian J. Org. Chem., 2015, 4, 602 CrossRef CAS;
(f) A. S. Deeming, E. J. Emmett, C. S. Richards-Taylor and M. C. Willis, Synthesis, 2014, 46, 2701 CrossRef;
(g) P. Bisseret and N. Blanchard, Org. Biomol. Chem., 2013, 11, 5393 RSC;
(h) G. Qiu, L. Lai, J. Cheng and J. Wu, Chem. Commun., 2018, 54, 10405 RSC;
(i) G. Qiu, K. Zhou and J. Wu, Chem. Commun., 2018, 54, 12561 RSC.
- For recent selected examples, see:
(a) Y. Wang, L. Deng, J. Zhou, X. Wang, H. Mei, J. Han and Y. Pan, Adv. Synth. Catal., 2018, 360, 1060 CrossRef CAS;
(b) Y. Wang, L. Deng, Y. Deng and J. Han, J. Org. Chem., 2018, 83, 4674 CrossRef CAS;
(c) T. Wang, F. Wang, J. Shen, T. Pang, Y. Ren, B. Wu and X. Zhang, Tetrahedron Lett., 2018, 59, 1183 CrossRef CAS;
(d) D. Sun, K. Yin and R. Zhang, Chem. Commun., 2018, 54, 1335 RSC;
(e) Z.-J. Shen, Y.-N. Wu, C.-L. He, L. He, W.-J. Hao, A.-F. Wang, S.-J. Tu and B. Jiang, Chem. Commun., 2018, 54, 445 RSC;
(f) V. Vedovato, E. P. A. Talbot and M. C. Willis, Org. Lett., 2018, 20, 5493 CrossRef CAS PubMed;
(g) D. Yang, P. Sun, W. Wei, F. Liu, H. Zhang and H. Wang, Chem. – Eur. J., 2018, 24, 4423 CrossRef CAS;
(h) H. T. Dang, V. T. Nguyen, V. D. Nguyen, H. D. Arman and O. V. Larionov, Org. Biomol. Chem., 2018, 16, 3605 RSC;
(i) M. Wang, B.-C. Tang, J.-G. Wang, J.-C. Xiang, A.-Y. Guan, P.-P. Huang, W.-Y. Guo, Y.-D. Wu and A.-X. Wu, Chem. Commun., 2018, 54, 7641 RSC;
(j) H. Wang, B. Wang, S. Sun and J. Cheng, Org. Chem. Front., 2018, 5, 2547 RSC;
(k) Y. Chen, P. R. D. Murray, A. T. Davies and M. C. Willis, J. Am. Chem. Soc., 2018, 140, 8781 CrossRef CAS;
(l) X. Gong, J. Chen, X. Li, W. Xie and J. Wu, Chem. – Asian J., 2018, 13, 2543 CrossRef CAS;
(m) Z. Chen, N.-W. Liu, M. Bolte, H. Rena and G. Manolikakes, Green Chem., 2018, 20, 3059 RSC.
- For selected examples, see:
(a) D. Zheng, Y. An, Z. Li and J. Wu, Angew. Chem., Int. Ed., 2014, 53, 2451 CrossRef CAS;
(b) D. Zheng, J. Yu and J. Wu, Angew. Chem., Int. Ed., 2016, 55, 11925 CrossRef CAS;
(c) H. Xia, Y. An, X. Zeng and J. Wu, Org. Chem. Front., 2018, 5, 366 RSC;
(d) K. Zhou, M. Chen, L. Yao and J. Wu, Org. Chem. Front., 2018, 5, 371 RSC;
(e) X. Wang, Y. Li, G. Qiu and J. Wu, Org. Chem. Front., 2018, 5, 2555 RSC;
(f) H. Xia, Y. An, X. Zeng and J. Wu, Chem. Commun., 2017, 53, 12548 RSC;
(g) K. Zhou, J. Zhang, L. Lai, J. Cheng, J. Sun and J. Wu, Chem. Commun., 2018, 54, 7459 RSC;
(h) J. Zhang, F. Zhang, L. Lai, J. Cheng, J. Sun and J. Wu, Chem. Commun., 2018, 54, 3891 RSC;
(i) J. Zhang, K. Zhou and J. Wu, Org. Chem. Front., 2018, 5, 813 RSC;
(j) F.-S. He, X. Cen, S. Yang, J. Zhang, H. Xia and J. Wu, Org. Chem. Front., 2018, 5, 2437 RSC.
- For review, see: G. Qiu, Y. Li and J. Wu, Org. Chem. Front., 2016, 3, 1011 RSC.
- For selected examples, see:
(a) P. Kamal Walia, M. Kumar and V. Bhalla, ACS Omega, 2018, 3, 1983 CrossRef;
(b) X. Yang, W. Liu, L. Li, W. Wei and C.-J. Li, Chem. – Eur. J., 2016, 22, 15252 CrossRef CAS PubMed;
(c) W. Liu, L. Li and C.-J. Li, Nat. Commun., 2015, 6, 6526 CrossRef CAS;
(d) L. Li, W. Liu, H. Zeng, X. Mu, G. Cosa, Z. Mi and C.-J. Li, J. Am. Chem. Soc., 2015, 137, 8328 CrossRef CAS;
(e) Y. Li, D. Zheng, Z. Li and J. Wu, Org. Chem. Front., 2016, 3, 574 RSC;
(f) X. Gong, Y. Ding, X. Fan and J. Wu, Adv. Synth. Catal., 2017, 359, 2999 CrossRef CAS.
- For selected examples by using inorganic sulfites as the source of sulfur dioxide, see:
(a) S. Ye and J. Wu, Chem. Commun., 2012, 48, 10037 RSC;
(b) M. Wang, S. Chen and X. Jiang, Org. Lett., 2017, 19, 4916 CrossRef CAS;
(c) M. W. Johnson, S. W. Bagley, N. P. Mankad, R. G. Bergman, V. Mascitti and F. D. Toste, Angew. Chem. Int. Ed., 2014, 53, 4404 CrossRef CAS PubMed;
(d) Y. Wang, B. Du, W. Sha, J. Han and Y. Pan, Org. Chem. Front., 2017, 4, 1313 RSC;
(e) J. Zhang, Y. An and J. Wu, Chem. – Eur. J., 2017, 23, 9477 CrossRef CAS;
(f) A. Shavnya, S. B. Coffey, A. C. Smith and V. Mascitti, Org. Lett., 2013, 15, 6226 CrossRef CAS;
(g) A. Shavnya, K. D. Hesp, V. Mascitti and A. C. Smith, Angew. Chem., Int. Ed., 2015, 54, 13571 CrossRef CAS;
(h) H. Konishi, H. Tanaka and K. Manabe, Org. Lett., 2017, 19, 1578 CrossRef CAS;
(i) X. Gong, J. Chen, L. Lai, J. Cheng, J. Sun and J. Wu, Chem. Commun., 2018, 54, 11172 RSC;
(j) N.-W. Liu, S. Liang and G. Manolikakes, Adv. Synth. Catal., 2017, 359, 1308 CrossRef CAS;
(k) H. Zhu, Y. Shen, Q. Deng, J. Chen and T. Tu, Chem. Commun., 2017, 53, 12473 RSC.
Footnote |
† Electronic supplementary information (ESI) available. See DOI: 10.1039/c8qo01048j |
|
This journal is © the Partner Organisations 2019 |
Click here to see how this site uses Cookies. View our privacy policy here.