DOI:
10.1039/C8QO01089G
(Research Article)
Org. Chem. Front., 2019,
6, 22-26
Asymmetric synthesis of the tetracyclic core of bufogargarizin C by an intramolecular [5 + 2] cycloaddition†
Received
10th October 2018
, Accepted 5th November 2018
First published on 6th November 2018
Abstract
A concise asymmetric synthesis of the core of bufogargarizin C, which contains a synthetically challenging 7/5/6/5-tetracyclic ring system, is reported. This approach features a unique Brønsted acid-mediated intramolecular [5 + 2] cycloaddition, which is the first example of intramolecular [5 + 2] cycloaddition of an allene moiety mediated by acid.
1. Introduction
Steroids play a significant role in synthetic organic chemistry and drug development owing to their biological importance and structural diversity.1 Bufadienolides (Fig. 1) have a steroidal structure with a 2-pyranone ring at the C17 position and are an important class of natural products. These compounds are active ingredients of toad venom and exhibit significant cardiotonic, anesthetic, and antitumor activities.2 Additionally, bufadienolides have been reported to be specific inhibitors of Na+/K+-ATPase and have attracted attention as potential targets for anticancer drugs following the recent discovery of the signaling pathways.3 In 2010, bufogargarizin A (1) – the first example of a bufadienolide with unusual alterations of rings A and B – was isolated from the venom of Bufo bufo gargarizans by Ye and co-workers.4a In 2015, bufogargarizin C (2) was isolated by Hu and co-workers.4b The structures of bufogargarizins A and C are based on a carbon skeleton with an unusual 7/5/6/5 ring system, instead of the 6/6/6/5 skeleton of common bufadienolides. Furthermore, bufogargarizins A and C contain eight or ten stereocenters with three tetra-substituted centers including an all-carbon quaternary center. These structural features pose considerable challenges to the synthesis of the compounds. However, the relative scarcity of natural sources of bufogargarizins A and C has impeded a systematic evaluation of their biological activity. Therefore, the development of an efficient method of synthesis for these complex molecules and their analogues is highly desirable.
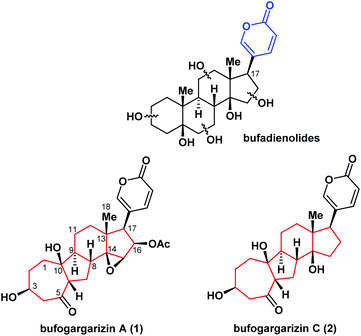 |
| Fig. 1 Bufogargarizins A and C. | |
Together with their promising pharmacological properties, the fascinating structural motifs of bufadienolides have attracted significant interest from the synthetic chemistry community.5 However, no synthetic studies or total syntheses of bufogargarizins A and C have been reported. As part of our continuing efforts toward the synthesis of biologically active natural products,6 we herein report a concise synthetic approach to the construction of the core of bufogargarizin C containing the 7/5/6/5-tetracyclic ring system, using a unique Brønsted acid-mediated intramolecular [5 + 2] cycloaddition.
Results and discussion
Retrosynthetic analysis of bufogargarizin C
Fig. 2 illustrates the retrosynthetic analysis of bufogargarizin C (2) that was used to develop the concise strategy employed in our synthesis. We anticipated that 2 could be generated from compound 3 through a series of functional group transformations, including selective reductive cleavage of the C4–O bond, installation of the δ-lactone ring at C17 (bufogargarizin C numbering), and synthesis of the tertiary alcohol at C14 via elimination and hydration (see Scheme 4 for more details). In turn, the 7/5/6/5-tetracyclic ring system of 3 would be synthesized from 4 using type I intramolecular [5 + 2] cycloaddition7,8 of the allene moiety, although it would be difficult to control the diastereoselectivity of the reaction of compound 4. Furthermore, the intramolecular [5 + 2] cycloaddition could result in the 7/6/6/5-tetracyclic ring system iso-3, making this reaction particularly challenging. Compound 4 can be synthesized from 5 by an Achmatowicz reaction.9 Finally, compound 5 could be generated from readily available furan 6, Grignard reagent 7, and commercially available 86a as the chiral pool compound10 using Stille coupling and a 1,2-addition reaction.
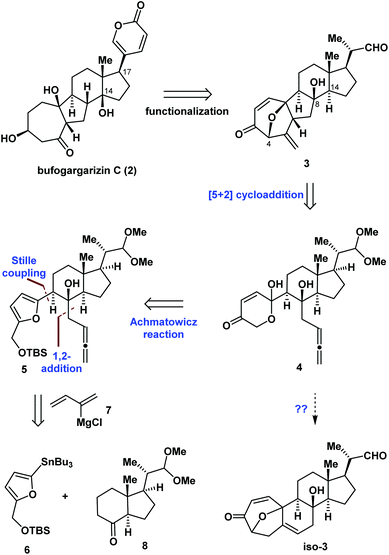 |
| Fig. 2 Retrosynthetic analysis of bufogargarizin C. | |
Asymmetric synthesis of the tetracyclic core of bufogargarizin C
Our synthesis began with the preparation of compound 10 by the previous synthetic route from starting material 86a (Scheme 1). Stille coupling of 10 and 611 catalyzed by Pd(PPh3)4 and copper(I) thiophene carboxylate (CuTc) in N-methyl-2-pyrrolidone (NMP) produced 11 in 81% yield. The 1,4-reduction of enone 11 using NaBH4 and NiCl2
12 in MeOH provided ketone 12 diastereoselectively in 80% yield. Treatment of 12 with the Grignard reagent (7)13 in THF produced compound 5 as a single product in 71% yield. Protection of the tertiary alcohol using the MOM group and removal of the TBS groups using TBAF in situ generated compound 13 in 82% yield.
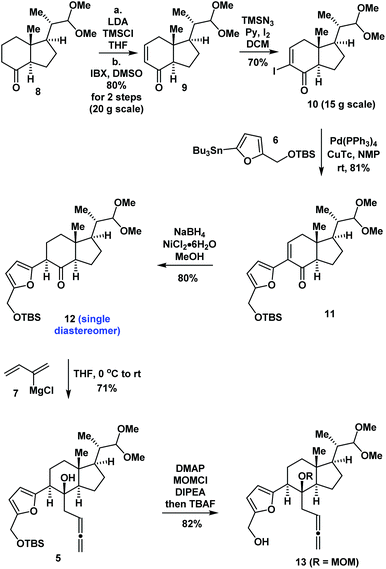 |
| Scheme 1 Synthesis of compound 13. | |
Following the successful synthesis of compound 13, we proceeded to investigate the synthesis of the tetracyclic core of bufogargarizin C using intramolecular [5 + 2] cycloaddition. Initially, oxidative rearrangement of 13 using mCPBA in DCM (Scheme 2) gave an inseparable mixture of 14a and 14b (14b/14a = 2). Allenes 14a and 14b are in dynamic equilibrium. Treatment of the mixture of 14a and 14b with acetic anhydride (Ac2O), Et3N, and 4-dimethylaminopyridine (DMAP) gave compound 15b as a single product which could not undergo intramolecular [5 + 2] cycloaddition. After testing various reaction conditions, the desired precursor of intramolecular [5 + 2] cycloaddition (15a) could not be obtained because of the steric hinderance of the anomeric hydroxyl group of 14a.
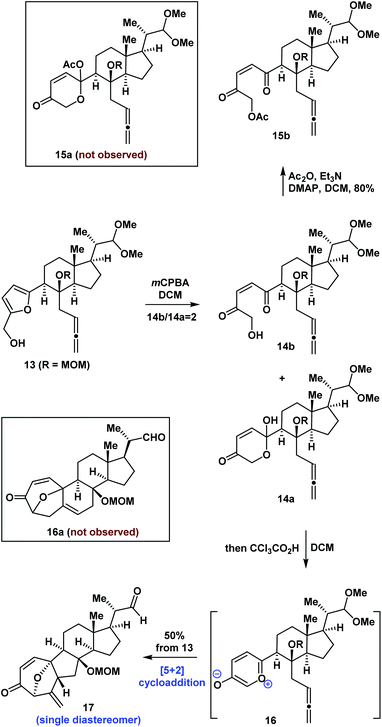 |
| Scheme 2 Synthesis of 17 by an intramolecular [5 + 2] cycloaddition reaction mediated by a Brønsted acid. | |
We proposed that the anomeric hydroxyl group of 14a would undergo elimination and enolation in the presence of acid to generate the oxidopyrylium ylide 16. Intramolecular [5 + 2] cycloaddition of 16 could then occur (Scheme 2). We therefore investigated the reaction under acidic conditions. After extensive experimentation, we identified the following protocol to be optimal: compound 13 was reacted with mCPBA in DCM for 0.5 hours, followed by the addition of CCl3CO2H. The compound underwent a regio- and diastereoselective intramolecular [5 + 2] cycloaddition and deprotection to give a 7/5/6/5-tetracyclic ring system (17) in 50% overall yield (1.0 g scale). The structure of 17 was unambiguously confirmed by X-ray crystallographic analysis of its derivative 19 (Scheme 3). Our intramolecular [5 + 2] cycloaddition therefore allowed efficient synthesis of the challenging 7/5/6/5-tetracyclic core of 17, which was achieved using a linear sequence of only 8 steps from the starting material (8).
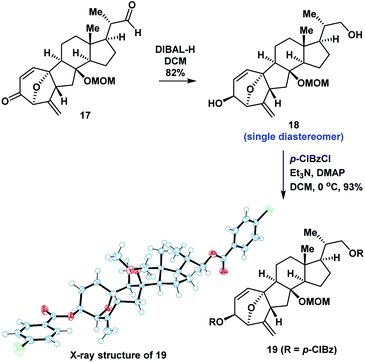 |
| Scheme 3 Synthesis of 19 and its X-ray structure. | |
To the best of our knowledge, there have been few reports of intramolecular [5 + 2] cycloadditions mediated by a Brønsted acid.7 This work provides a new approach for the generation of oxidopyrylium ylide for [5 + 2] cycloaddition besides the group elimination7 and group transfer strategies.14
Furthermore, in the presence of Crabtree's ([Ir(COD)(PCy3)(py)]PF6) and Wilkinson's (RhCl(PPh)3) catalysts (Scheme 4), compound 19 underwent regioselective hydrogenation, followed by ozonolysis to give the ketone 21 in 60% overall yield, together with by-product 20 in 8% yield which was formed via an unusual oxidative rearrangement. Thus, the 7/5/6/5-tetracyclic ring system 21 – which contained the desired functional groups including the intended stereochemistry at C3, C6, C9, C13, and C17 of bufogargarizin C (2) (highlighted in red) – was successfully synthesized. This compound (21) could be a potentially advanced intermediate for the preparation of bufogargarizin C (2) (as shown in Scheme 4).15
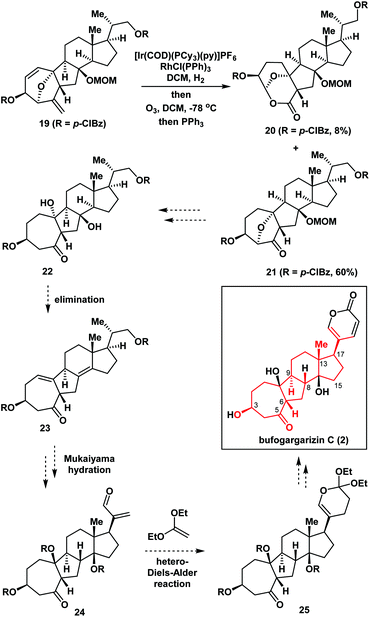 |
| Scheme 4 Synthesis of 21 and proposed functional group transformations to bufogargarizin C. | |
Conclusions
A concise asymmetric synthetic approach to construct the core of bufogargarizin C has been developed, with successful synthesis of the challenging 7/5/6/5-tetracyclic ring system confirmed. This approach features a unique Brønsted acid-mediated intramolecular [5 + 2] cycloaddition and represents the first example of acid-mediated intramolecular [5 + 2] cycloaddition of an allene moiety. This offers a new strategy to produce the reactive oxidopyrylium ylide for [5 + 2] cycloaddition besides group elimination and transfer strategies. Five of the eight stereocenters in 2 that contain the desired functional groups have been included in compound 21. Efforts to further streamline this sequence and apply it to the total synthesis of 2 are underway in our laboratory. An investigation of the biological activities of the oxa-7/5/6/5 core is also underway.
Conflicts of interest
There are no conflicts to declare.
Acknowledgements
This work was supported by the Natural Science Foundation of China (Grant no. 21472081, 21522204, and 21672095), Guangdong Science and Technology Department (2016A050503011), the Shenzhen Science and Technology Innovation Committee (Grant no. JCYJ20170412152454807 and JSGG20160301103446375), the Shenzhen Nobel Prize Scientists Laboratory Project (C17783101) and Project KQTD2016053117035204 supported by Shenzhen Peacock Plan.
Notes and references
- For representative reviews, see:
(a)
L. F. Fieser and M. Fieser, Steroide, Verlag Chemie, Weinheim, 1961 Search PubMed;
(b) J. F. Biellmann, Chem. Rev., 2003, 103, 2019 CrossRef CAS;
(c) N. S. Sarma, M. S. Krishna, S. G. Pasha, T. S. P. Rao, Y. Venkateswarlu and P. S. Parameswaran, Chem. Rev., 2009, 109, 2803 CrossRef CAS;
(d) J. R. Hanson, Nat. Prod. Rep., 2010, 27, 887 RSC;
(e) J. A. R. Salvador, J. F. S. Carvalho, M. A. C. Neves, S. M. Silvestre, A. J. Leitão, M. M. C. Silva and M. L. Sá e Melo, Nat. Prod. Rep., 2013, 30, 324 RSC;
(f) R. Bansal and P. C. Acharya, Chem. Rev., 2014, 114, 6986 CrossRef CAS. Cortistatin A represents the most prominent recent example. For isolation, see:
(g) S. Aoki, Y. Watanabe, M. Sanagawa, A. Setiawan, N. Kotoku and M. Kobayashi, J. Am. Chem. Soc., 2006, 128, 3148 CrossRef CAS.
-
(a) P. S. Steyn and F. R. Van Heerdeen, Nat. Prod. Rep., 1998, 15, 397 RSC;
(b) W. Schoner, Eur. J. Biochem., 2002, 269, 2440 CrossRef CAS.
-
(a) I. Prassas and E. P. Diamandis, Nat. Rev. Drug Discovery, 2008, 7, 926 CrossRef CAS;
(b) T. Mijatovic, L. Ingrassia, V. Facchini and R. Kiss, Expert Opin. Ther. Targets, 2008, 12, 1403 CrossRef CAS.
-
(a) H. Y. Tian, L. Wang, X. Q. Zhang, Y. Wang, D. M. Zhang, R. W. Jiang, Z. Liu, Y. L. Li and W. C. Ye, Chem. – Eur. J., 2010, 16, 10989 CrossRef CAS PubMed;
(b) H. Chen, Y. H. Meng, D. A. Guo, X. Liu, J. H. Liu and L. H. Hu, Fitoterapia, 2015, 104, 1 CrossRef CAS.
- Synthesis of bufadienolides and related cardenolides, for reviews, see:
(a) B. Heasley, Chem. – Eur. J., 2012, 18, 3092 CrossRef CAS;
(b) M. Michalak, K. Michalak and J. Wicha, Nat. Prod. Rep., 2017, 34, 361 RSC. For selected examples, see:
(c) A. R. Daniewski, M. M. Kabat, M. Masnyk, J. Wicha and W. Wojciechowska, J. Org. Chem., 1988, 53, 4855 CrossRef CAS;
(d) W. Deng, M. S. Jensen, L. E. Overman, P. V. Rucker and J. P. Vionnet, J. Org. Chem., 1996, 61, 6760 CrossRef CAS;
(e) G. Stork, F. West, H. Y. Lee, R. C. A. Isaacs and S. Manabe, J. Am. Chem. Soc., 1996, 118, 10660 CrossRef CAS;
(f) M. Honma and M. Nakada, Tetrahedron Lett., 2007, 48, 1541 CrossRef CAS;
(g) H. Zhang, M. S. Reddy, S. Phoenix and P. Deslongchamps, Angew. Chem., Int. Ed., 2008, 47, 1272 CrossRef CAS;
(h) M. E. Jung and D. Yoo, Org. Lett., 2011, 13, 2698 CrossRef CAS;
(i) H. Renata, Q. Zhou and P. S. Baran, Science, 2013, 339, 59 CrossRef CAS;
(j) K. Mukai, D. Urabe, S. Kasuya, N. Aoki and M. Inoue, Angew. Chem., Int. Ed., 2013, 52, 5300 CrossRef CAS;
(k) H. Renata, Q. Zhou, G. Dunstl, J. Felding, R. R. Merchant, C. H. Yeh and P. S. Baran, J. Am. Chem. Soc., 2015, 137, 1330 CrossRef CAS;
(l) K. Mukai, S. Kasuya, Y. Nakagawa, D. Urabe and M. Inoue, Chem. Sci., 2015, 6, 3383 RSC.
-
(a) J. Liu, J. Wu, J. H. Fan, X. Yan, G. Mei and C. C. Li, J. Am. Chem. Soc., 2018, 140, 5365 CrossRef CAS;
(b) M. J. Cheng, J. Q. Cao, X. Y. Yang, L. P. Zhong, L. J. Hu, X. Lu, B. L. Hou, Y. J. Hu, Y. Wang, X. F. You, L. Wang, W. C. Ye and C. C. Li, Chem. Sci., 2018, 9, 1488 RSC;
(c) B. Chen, X. Liu, Y. J. Hu, D. Zhang, L. Deng, J. Lu, L. Min, W. C. Ye and C. C. Li, Chem. Sci., 2017, 8, 4961 RSC;
(d) C. Qiao, W. Zhang, J. C. Han and C. C. Li, Org. Lett., 2016, 18, 4932 CrossRef CAS;
(e) J. C. Han, F. Li and C. C. Li, J. Am. Chem. Soc., 2014, 136, 13610 CrossRef CAS;
(f) H. Wei, C. Qiao, G. Liu, Z. Yang and C. C. Li, Angew. Chem., Int. Ed., 2013, 52, 620 CrossRef CAS PubMed.
- For review and selected applications of [5 + 2] cycloaddition reaction in natural product synthesis, see:
(a) X. Liu, Y. J. Hu, J. H. Fan, J. Zhao, S. P. Li and C. C. Li, Org. Chem. Front., 2018, 5, 1217 RSC;
(b) L. P. Bejcek and R. P. Murelli, Tetrahedron, 2018, 74, 2501 CrossRef CAS;
(c) K. E. O. Ylijoki and J. M. Stryker, Chem. Rev., 2013, 113, 2244 CrossRef CAS;
(d) P. A. Wender, K. D. Rice and M. E. Schnute, J. Am. Chem. Soc., 1997, 119, 7897 CrossRef CAS;
(e) P. A. Roethle, P. T. Hernandez and D. Trauner, Org. Lett., 2006, 8, 5901 CrossRef CAS;
(f) M. Zhang, N. Liu and W. Tang, J. Am. Chem. Soc., 2013, 135, 12434 CrossRef CAS;
(g) S. Kruger and T. Gaich, Angew. Chem., Int. Ed., 2015, 54, 315 CrossRef;
(h) C. He, J. Hu, Y. Wu and H. Ding, J. Am. Chem. Soc., 2017, 139, 6098 CrossRef CAS.
- For the type II intramolecular [5 + 2] cycloaddition reactions developed by our group, see:
(a) G. Mei, X. Liu, C. Qiao, W. Chen and C. C. Li, Angew. Chem., Int. Ed., 2015, 54, 1754 CrossRef CAS;
(b) X. Liu, J. Liu, J. Zhao, S. Li and C. C. Li, Org. Lett., 2017, 19, 2742 CrossRef CAS. For its application in natural product total synthesis, see ref. 6a.
- O. Achmatowicz Jr., P. Bukowski, B. Szechner, Z. Zwierzchowska and A. Zamojski, Tetrahedron, 1971, 27, 1973 CrossRef.
- For a review about the chiral pool in the total synthesis, see: Z. G. Brill, M. L. Condakes, C. P. Ting and T. J. Maimone, Chem. Rev., 2017, 117, 11753 CrossRef CAS.
- For synthesis of compound 6, see: J. Sandosham and K. Undheim, Acta Chem. Scand., 1994, 48, 279 CrossRef.
- D. Dhawan and S. K. Grover, Synth. Commun., 1992, 22, 2405 CrossRef CAS.
-
(a) S. Nunomoto and Y. Yamashita, J. Org. Chem., 1979, 44, 4788 CrossRef CAS;
(b) T. A. Bradford, A. D. Payne, A. C. Willis, M. N. Paddon-Row and M. S. Sherburn, Org. Lett., 2007, 9, 4861 CrossRef CAS.
-
(a) P. A. Wender and J. L. Mascareñas, J. Org. Chem., 1991, 56, 6267 CrossRef CAS;
(b) P. A. Wender and J. L. Mascareñas, Tetrahedron Lett., 1992, 33, 2115 CrossRef CAS;
(c) G. Mei, H. Yuan, Y. Gu, W. Chen, L. W. Chung and C. C. Li, Angew. Chem., Int. Ed., 2014, 53, 11051 CrossRef CAS.
- For Mukaiyama hydration, see:
(a) T. Mukaiyama, S. Isayama, S. Inoki, K. Kato, T. Yamada and T. Takai, Chem. Lett., 1989, 18, 449 CrossRef;
(b) S. W. M. Crossley, C. Obradors, R. M. Martinez and R. A. Shenvi, Chem. Rev., 2016, 116, 8912 CrossRef CAS. For hetero-Diels-Alder reaction, see:
(c) S. Leconte, G. Dujardin and E. Brown, Eur. J. Org. Chem., 2000, 639 CrossRef CAS;
(d) G. Blond, M. Gulea and V. Mamane, Curr. Org. Chem., 2016, 20, 2161 CrossRef CAS.
Footnotes |
† Electronic supplementary information (ESI) available. CCDC 1867814. For ESI and crystallographic data in CIF or other electronic format see DOI: 10.1039/c8qo01089g |
‡ These authors contributed equally to this work. |
|
This journal is © the Partner Organisations 2019 |
Click here to see how this site uses Cookies. View our privacy policy here.