DOI:
10.1039/C8QO01144C
(Research Article)
Org. Chem. Front., 2019,
6, 94-98
Cu(OTf)2-mediated C(sp2)–H arylsulfonylation of enamides via the insertion of sulfur dioxide†
Received
23rd October 2018
, Accepted 21st November 2018
First published on 23rd November 2018
Abstract
An efficient and straightforward three-component reaction of enamides, aryldiazonium tetrafluoroborates, and 1,4-diazabicyclo[2.2.2]octane bis(sulfur dioxide) (DABSO) for the direct C(sp2)–H arylsulfonylation of enamides has been developed. This Cu(OTf)2-mediated SO2 insertion reaction proceeds smoothly to afford a diverse range of β-amidovinyl sulfones bearing manifold functional groups in moderate to excellent yields with high regio- and stereoselectivities.
Enamides are particularly attractive and pivotal synthetic intermediates owing to their potential ability to undergo various organic transformations for the synthesis of a plethora of natural products and pharmaceuticals.1,2 Therefore, the development of facile and highly efficient approaches for the synthesis of functionalized enamides has gathered considerable attention among the chemical community. In the past few years, plenty of breakthroughs have been achieved to develop novel synthetic strategies for the synthesis of β-functionalized enamides bearing a diverse range of functional groups through the direct C–H functionalization of the β-C(sp2)–H bond, such as arylation,3 olefination,4 trifluoromethylation,5 alkynylation,6 alkylation,7 carbonylation8 and other transformations.9 Nevertheless, the direct sulfonylation of enamides has been scarcely demonstrated. One of the early studies on the direct β-sulfonylation of enamides was established by our group through a Pd-catalyzed C(sp2)–H sulfonylation of cyclic enamides via the insertion of arylsulfonyl chlorides into a vinyl palladium intermediate (Scheme 1a, eqn (1)).10a Another elegant example was demonstrated by Yu and coworkers through an iridium-catalyzed direct arylsulfonylation of enamides with arylsulfonyl chlorides via visible-light photoredox catalysis (Scheme 1a, eqn (2)).10b Recently, a metal-free visible light-induced cross-coupling of enamides with sodium sulfinates was achieved by Zhang and coworkers [Scheme (1b)].11 Despite these breakthroughs, the existing methods for the sulfonylation of enamides are somewhat limited in terms of the finite substrate scope, the employment of expensive catalysts and the need for more than stoichiometric amounts of hazardous and polluting oxidants or additives. Thus, the development of a robust, efficient and generally applicable tool for the preparation of synthetically important β-amidovinyl sulfones with high stereoselectivity is still highly desirable.
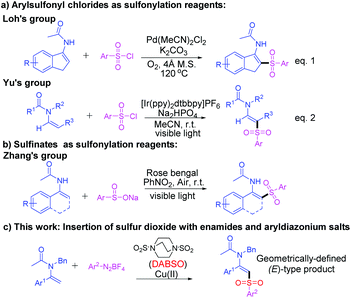 |
| Scheme 1 Sulfonylation of enamides. | |
Recently, the insertion of sulfur dioxide has gained increasing attention in the research field of the synthesis of sulfonyl derivatives.12 After the first description of 1,4-diazabicyclo[2.2.2]octane bis(sulfur dioxide) (DABSO) as demonstrated by Santos and Mello,13 and its pioneering application as a readily available surrogate of sulfur dioxide as disclosed by Willis and coworkers,14 a range of protocols using DABSO as sulfonylation reagents blossomed rapidly with several remarkable advances as established by the group of Wu,15 Tu,16 and other groups.17 Particularly, as originally reported by Wu and coworkers,18 the combination of DABSO and aryldiazonium salts has now been widely utilized as an efficient approach for the generation of arylsulfonyl radicals which could trigger ensuing radical addition to alkenes for sp2-arylsulfonylation.15g–m Nevertheless, to the best of our knowledge, the direct C(sp2)–H sulfonylation of enamides via the insertion of sulfur dioxide was undeveloped. Herein, we demonstrate a Cu(OTf)2-mediated C(sp2)–H direct sulfonylation of enamides with sulfonyl radicals generated in situ from DABSO and diazonium salts (Scheme 1c). The approach proceeds smoothly to afford geometrically defined (E)-β-amidovinyl sulfones in moderate to excellent yields with high stereoselectivity.
Our investigation began with the reaction of 1a with 2a for the screening of the optimal reaction conditions (for details see ESI, Tables S1–S4†), and it was found that the best result was obtained in the presence of 1.2 eq. of Cu(OTf)2 and 1.5 eq. of DABSO using a solvent mixture (DCE/iPrOH = 5/1) at 50 °C (see ESI, Table S4,† entry 9). With the optimal reaction conditions in hand, we started to investigate the reaction scope with respect to aryldiazonium tetrafluoroborates 2a–t. The results are summarized in Table 1.
Table 1 Substrate scope of aryldiazonium tetrafluoroborates 2a–ta,b,c
Reaction conditions: Enamide 1a (0.3 mmol), diazonium salts 2a–t (0.45 mmol, 1.5 equiv.), DABSO (0.45 mmol, 1.5 equiv.), and Cu(OTf)2 (0.36 mmol, 1.2 equiv.) in a mixed solvent (DCE/iPrOH = 5/1, 1.5 mL) at 50 °C for 12 h under nitrogen.
Isolated yields.
The ratios of E/Z shown in parentheses were determined from the 1H NMR spectra at the end of the corresponding reactions.
|
|
Generally, the benzenediazonium tetrafluoroborates bearing electron-donating groups reacted with 1a and DABSO smoothly to give the desired products 3aa–3ah in moderate to excellent yields (50–88%). The configuration of 3aa was further confirmed by X-ray crystallography.19 Notably, manifold functional groups including –OH, –CN, –NO2, –CO2Et, –COMe, and –COPh were well tolerated under the standard conditions to give the desired products 3af, 3am–3aq in moderate to good yields. Substrates bearing halogen atoms (–F, –Cl, –Br, and –I) also gave 3ai–3al in good yields, which made them amenable for further functionalization through cross-coupling reactions. In addition, the more bulky substrates 2h, 2i and 2k could also undergo the transformation to produce the desired products 3ah, 3ai and 3ak in 70%, 48% and 72% yields, respectively. Moreover, aryl group Ar2 may also be an α-naphthyl or a β-naphthyl group, generating the target products 3ar and 3as in 73% and 69% yields, respectively. As for the scope of Ar2, heterocyclic skeletons such as thiophene were also tolerated in this transformation to afford the desired product 3at in 26% yield.
Encouraged by the above results, we turned our attention to the substrate scope of enamides. The results are listed in Table 2. It was found that enamides 1b–j bearing diverse substituents with various electronic peculiarities (electron-donating or electron-withdrawing) and steric effects (ortho-, meta-, or para-) on the aryl groups could react well with diazonium salt 2a and DABSO under the optimal conditions, delivering the geometrically defined desired products 3ba–ja with excellent yields and stereoselectivities (E-configured) (85–95%). Fortunately, when the Ar1 group is β-naphthyl, the reaction could proceed well to afford the product 3ka in 93% yield. In addition, when an enamide bearing a heterocyclic 2-thienyl moiety N-benzyl-N-(1-(thiophen-2-yl)vinyl)acetamide (1l) was applied to the reaction mixture, the desired product 3la was also successfully obtained with considerable yield and stereoselectivity (E/Z = 84
:
16) (81% yield). It should be noted that 3ma could also be synthesized in 87% yield when the scale of the reaction was expanded to 0.5 mmol.
Table 2 Substrate scope of enamides 1b–ma,b,c
Reaction conditions: Enamides 1b–m (0.3 mmol), diazonium salt 2a (0.45 mmol, 1.5 equiv.), DABSO (0.45 mmol, 1.5 equiv.), and Cu(OTf)2 (0.36 mmol, 1.2 equiv.) in a mixed solvent (DCE/iPrOH = 5/1, 1.5 mL) at 50 °C for 12 h under nitrogen.
Isolated yields.
The ratios of E/Z shown in parentheses were determined from the 1H NMR spectra at the end of the corresponding reactions.
|
|
In order to further exhibit the practicality and synthetic utility of this arylsulfonylation, we carried out the following reactions. Firstly, a gram scale reaction of 1a with 2a and DABSO proceeded smoothly to furnish 3aa in 83% yield (Scheme 2a). Subsequently, further transformations of arylsulfonylation products were explored (Scheme 2b). A palladium-catalyzed intramolecular Heck coupling reaction of 3ma proceeded smoothly, which generated the synthetically and pharmaceutically important isoquinoline product 5 in 64% yield. In addition, hydrolysis of 3aa in the presence of concentrated hydrochloric acid could produce 1-phenyl-2-(phenylsulfonyl)ethan-1-one 6 in 95% yield.
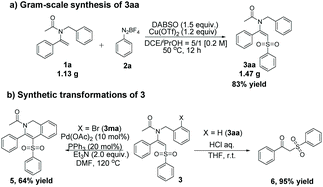 |
| Scheme 2 Demonstrating the practicality of the arylsulfonylation reaction of enamides. | |
Several control experiments were also carried out to shed more light on the reaction mechanism (Scheme 3). Initially, the radical scavenger 2,2,6,6-tetramethyl-1-piperidinyloxy (TEMPO) could inhibit the transformation so that the product yield decreased dramatically when an increasing amount of TEMPO was added (Scheme 3, eqn (a1)). The arylsulfonyl radical species could also be trapped when ethene-1,1-diyldibenzene 7 was added to the model reaction under standard conditions to give 8 accordingly (Scheme 3, eqn (a2)). Both of those observations suggested that the reaction went through a plausible radical process. Notably, 3ba and 8 could not be observed in the absence of Cu(OTf)2, which showed that Cu(OTf)2 might play a crucial role in activating the double bond to promote the transformation (Scheme 3, eqn (a2)). Moreover, when 2.0 equivalents of TEMPO were added, the desired product 3aa could still be obtained in 45% NMR yield, indicating that the enamides might be chelated with Cu(II) to generate a highly reactive species which could intercept the sulfonyl radical rapidly to afford the desired product (Scheme 3, eqn (a1)). The poison reaction of the copper source under the standard conditions with 1,4-diaza[2.2.2]bicyclooctane (DABCO) was carried out and the reaction was inhibited, which further proved the essential role of Cu(OTf)2 (Scheme 3, eqn (b)). Additionally, an intermolecular competition reaction between 1a and dideuterated 1a-d2 was carried out, calculating a kinetic isotope effect (KIE) KH/KD = 0.79 (Scheme 3, eqn (c)), showing that the C–H bond cleavage might not be involved in the rate-determining step.
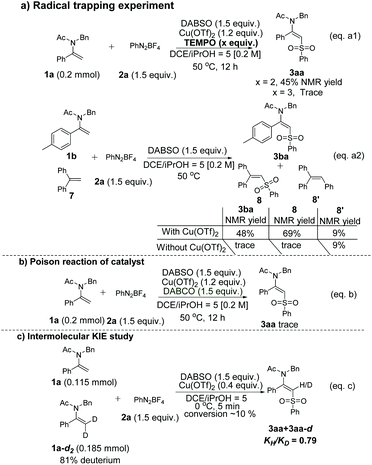 |
| Scheme 3 Control experiments. | |
On the basis of the above results and previous literature reports,15b,20 a plausible mechanism is proposed as shown in Scheme 4. Initially, Cu(II) coordinates with enamide 1 to give an active complex A (Scheme 3, eqn (a2)). Then, the arylsulfonyl radical,12 which is produced in situ by the reaction of diazonium salt 2 and DABSO, is intercepted by complex A rapidly to give a radical species B. Subsequently, a single electron transfer (SET) process between B and DABCO˙+ generates a cationic intermediate C, and sequential complexation of Cu(II) and DABCO and deprotonation of cation D forms the final product 3 (Scheme 3, eqn (b)).
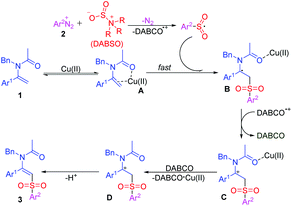 |
| Scheme 4 Proposed mechanism. | |
In conclusion, we have developed an efficient Cu(OTf)2-mediated three component C(sp2)–H arylsulfonylation of enamides with aryldiazonium tetrafluoroborates and DABCO˙(SO2)2. The reaction provides a novel, straightforward and robust approach to prepare synthetically valuable vinyl sulfones with excellent yields and high stereoselectivities. Due to simple operation and the easy availability of the starting materials, this method will be useful in organic synthesis. Further studies for the C(sp2)–H functionalization of enamides are ongoing in our laboratory.
Conflicts of interest
There are no conflicts to declare.
Acknowledgements
We gratefully acknowledge the financial support from Nanjing Tech University (Start-up Grant No. 39837137, 39837101 and 3827401739), and National Natural Science Foundation of China (21372210, 21672198, and 21801129), the State Key Program of National Natural Science Foundation of China (21432009), Natural science research projects in Jiangsu higher education institutions (18KJB150018) for generous financial support.
Notes and references
- For reviews of practicability of enamides, see:
(a) R. Matsubara and S. Kobayashi, Acc. Chem. Res., 2008, 41, 292 CrossRef CAS;
(b) D. R. Carbery, Org. Biomol. Chem., 2008, 6, 3455 RSC;
(c) T. C. Nugent and M. El-Shazly, Adv. Synth. Catal., 2010, 352, 753 CrossRef CAS;
(d) J.-H. Xie, S.-F. Zhu and Q.-L. Zhou, Chem. Rev., 2011, 111, 1713 CrossRef CAS;
(e) K. Gopalaiah and H. B. Kagan, Chem. Rev., 2011, 111, 4599 CrossRef CAS;
(f) K. Wang, F.-D. Hu, Y. Zhang and J.-B. Wang, Sci. China: Chem., 2015, 58, 1252 CrossRef CAS;
(g) T. Courant, G. Dagousset and G. Masson, Synthesis, 2015, 47, 1799 CrossRef CAS.
-
(a) Y. Sumiki, K. Isono, J. Nagatsu and T. Takauchi, J. Antibiot., Ser. A., 1960, 13, 416 CAS;
(b) I. G. Macara, G. C. McLeod and K. Kustin, Biochem. J., 1979, 181, 457 CrossRef CAS;
(c) R. C. Bruening, E. M. Oltz, J. Furukawa, K. Nakanishi and K. Kustin, J. Am. Chem. Soc., 1985, 107, 5298 CrossRef CAS;
(d) K. Nagaoka, M. Matsumoto, J. Oono, K. Yokoi, S. Ishizeki and T. Nakashima, J. Antibiot., 1986, 39, 1527 CrossRef CAS;
(e) D. Davyt, W. Entz, R. Fernandez, R. Mariezcurrena, A. W. Mombrú, J. Saldaña, L. Domínguez, J. Coll and E. Manta, J. Nat. Prod., 1998, 61, 1560 CrossRef CAS;
(f) L. Yet, Chem. Rev., 2003, 103, 4283 CrossRef CAS;
(g) T. Kuranaga, Y. Sesoko and M. Inoue, Nat. Prod. Rep., 2014, 31, 514 RSC.
-
(a) M. Prakash, S. Muthusamy and V. Kesavan, J. Org. Chem., 2014, 79, 7836 CrossRef CAS;
(b) F. Bartoccini, D. M. Cannas, F. Fini and G. Piersanti, Org. Lett., 2016, 18, 2762 CrossRef CAS;
(c) N. Gigant, L. Chausset-Boissarie, M.-C. Belhomme, T. Poisson, X. Pannecoucke and I. Gillaizeau, Org. Lett., 2013, 15, 278 CrossRef CAS;
(d) H. Zhou, W.-J. Chung, Y.-H. Xu and T.-P. Loh, Chem. Commun., 2009, 3472 RSC;
(e) H. Zhou, Y.-H. Xu, W.-J. Chung and T.-P. Loh, Angew. Chem., Int. Ed., 2009, 48, 5355 CrossRef CAS;
(f) S. Pankajakshan, Y.-H. Xu, J.-K. Cheng, M.-T. Low and T.-P. Loh, Angew. Chem., Int. Ed., 2012, 51, 5701 CrossRef CAS;
(g) M. Wang, X. Zhang, Y.-X. Zhuang, Y.-H. Xu and T.-P. Loh, J. Am. Chem. Soc., 2015, 137, 1341 CrossRef CAS.
-
(a) T. Besset, N. Kuhl, F. W. Patureau and F. Glorius, Chem. – Eur. J., 2011, 17, 7167 CrossRef CAS;
(b) Y.-H. Xu, Y.-K. Chok and T.-P. Loh, Chem. Sci., 2011, 2, 1822 RSC.
-
(a) H. Wang, Y.-Z. Cheng and S.-Y. Yu, Sci. China: Chem., 2016, 59, 195 CrossRef CAS;
(b) R. Rey-Rodriguez, P. Retailleau, P. Bonnet and I. Gillaizeau, Chem. – Eur. J., 2015, 21, 3572 CrossRef CAS;
(c) V. Krishnamurti, S. B. Munoz, X. Ispizua-Rodriguez, J. Vickerman, T. Mathew and G. K. S. Prakash, Chem. Commun., 2018, 54, 10574 RSC;
(d) C. Feng and T.-P. Loh, Chem. Sci., 2012, 3, 3458 RSC.
-
(a) A. C. Shaikh, D. R. Shinde and N. T. Patil, Org. Lett., 2016, 18, 1056 CrossRef CAS;
(b) C. Feng, D.-M. Feng and T.-P. Loh, Chem. Commun., 2014, 50, 9865 RSC;
(c) Y.-H. Xu, Q.-C. Zhang, T. He, F.-F. Meng and T.-P. Loh, Adv. Synth. Catal., 2014, 356, 1539 CrossRef CAS.
-
(a) B. Maji, S. Lakhdar and H. Mayr, Chem. – Eur. J., 2012, 18, 5732 CrossRef CAS;
(b) W.-L. Yu, W. Zhang, Y. Liu, Z.-X. Liu and Y.-H. Zhang, Org. Chem. Front., 2017, 4, 77 RSC;
(c) H.-M. Liu, W. Lu, C.-P. Luo and L. Yang, Tetrahedron Lett., 2016, 57, 4243 CrossRef CAS;
(d) R. Ding, Z.-D. Huang, Z.-L. Liu, T.-X. Wang, Y.-H. Xu and T.-P. Loh, Chem. Commun., 2016, 52, 5617 RSC.
-
(a) M. Chen, Z.-H. Ren, Y.-Y. Wang and Z.-H. Guan, Angew. Chem., Int. Ed., 2013, 52, 14196 CrossRef CAS;
(b) H. Wang, L. N. Guo and X. H. Duan, Org. Lett., 2012, 14, 4358 CrossRef CAS;
(c) S. Sharma, I. A. Khan and A. K. Saxena, Adv. Synth. Catal., 2013, 355, 673 CrossRef CAS;
(d) Z. Xiong, D.-D. Liang and S. Luo, Org. Chem. Front., 2017, 4, 1103 RSC;
(e) Z. Zhang, C.-J. Zhu, M. Miao, J.-L. Han, T. Ju, L. Song, J.-H. Ye, J. Li and D.-G. Yu, Chin. J. Chem., 2018, 36, 430 CrossRef CAS;
(f) R. Ding, Q.-C. Zhang, Y.-H. Xu and T.-P. Loh, Chem. Commun., 2014, 50, 11661 RSC.
-
(a) A. Madin, C. J. O'Donnell, T. Oh, D. W. Old, L. E. Overman and M. J. Sharp, Angew. Chem., Int. Ed., 1999, 38, 2934 CrossRef CAS;
(b) W. G. Earley, J. E. Jacobsen, A. Madin, G. P. Meier, C. J. O'Donnell, T. Oh, D. W. Old, L. E. Overman and M. J. Sharp, J. Am. Chem. Soc., 2005, 127, 18046 CrossRef CAS;
(c) W.-L. Yu, J. Chen, K. Gao, Z.-X. Liu and Y.-H. Zhang, Org. Lett., 2014, 16, 4870 CrossRef CAS;
(d) K. D. Hesp, R. G. Bergman and J. A. Ellman, J. Am. Chem. Soc., 2011, 133, 11430 CrossRef CAS;
(e) Y.-H. Li, K. Cheng, X.-X. Lu and J. Sun, Adv. Synth. Catal., 2010, 352, 1876 CrossRef CAS;
(f) L. Yang, Q. Wen, F.-H. Xiao and G.-J. Deng, Org. Biomol. Chem., 2014, 12, 9519 RSC;
(g) Y. Liu, Z.-X. Liu, Y.-H. Zhang and C.-H. Xiong, Adv. Synth. Catal., 2018, 360, 3492 CrossRef CAS;
(h) S. B. Munoz, V. Krishnamurti, P. Barrio, T. Mathew and G. K. S. Prakash, Org. Lett., 2018, 20, 1042 CrossRef CAS.
-
(a) Y.-H. Xu, M. Wang, P. Lu and T.-P. Loh, Tetrahedron, 2013, 69, 4403 CrossRef CAS;
(b) H. Jiang, X.-J. Chen, Y. Zhang and S.-Y. Yu, Adv. Synth. Catal., 2013, 355, 809 CrossRef CAS.
- D.-L. Sun and R.-H. Zhang, Org. Chem. Front., 2018, 5, 92 RSC.
- For reviews of the insertion of sulfur dioxide, see:
(a) P. Vogel, M. Turks, L. Bouchez, D. Marković, A. Varela-Alvarez and J. A. Sordo, Acc. Chem. Res., 2007, 40, 931 CrossRef CAS;
(b)
D.-Q. Zheng and J. Wu, Sulfur Dioxide Insertion Reactions for Organic Synthesis, Nature Springer, Berlin, 2017 CrossRef;
(c) G. Qiu, K.-D. Zhou, L. Gao and J. Wu, Org. Chem. Front., 2018, 5, 691 RSC;
(d) G. Liu, C.-B. Fan and J. Wu, Org. Biomol. Chem., 2015, 13, 1592 RSC;
(e) P. Bisseret and N. Blanchard, Org. Biomol. Chem., 2013, 11, 5393 RSC;
(f) A. S. Deeming, E. J. Emmett, C. S. Richards-Taylor and M. C. Willis, Synthesis, 2014, 46, 2701 CrossRef;
(g) E. J. Emmett and M. C. Willis, Asian J. Org. Chem., 2015, 4, 602 CrossRef CAS;
(h) K. Hofman, N.-W. Liu and G. Manolikakes, Chem. – Eur. J., 2018, 24, 11852 CrossRef CAS PubMed;
(i) K. Sutal and M. Turks, Chem. Heterocycl. Compd., 2018, 54, 584 CrossRef;
(j) G. Qiu, L.-F. Lai, J. Cheng and J. Wu, Chem. Commun., 2018, 54, 10405 RSC;
(k) M.-H. Huang, W.-J. Hao and B. Jiang, Chem. – Asian J., 2018, 13, 2958 CrossRef CAS;
(l) M.-H. Huang, W.-J. Hao, G.-G. Li, S.-J. Tu and B. Jiang, Chem. Commun., 2018, 54, 10791 RSC;
(m) G. Qiu, K.-D. Zhou and J. Wu, Chem. Commun., 2018, 54, 12561 RSC.
- P. S. Santos and M. T. S. Mello, J. Mol. Struct., 1988, 178, 121 CrossRef CAS.
- For recent selected examples, see:
(a) B. Nguyen, E. J. Emmett and M. C. Willis, J. Am. Chem. Soc., 2010, 132, 16372 CrossRef CAS;
(b) Y.-D. Chen and M. C. Willis, Chem. Sci., 2017, 8, 3249 RSC;
(c) A. T. Davies, J. M. Curto, S. W. Bagley and M. C. Willis, Chem. Sci., 2017, 8, 1233 RSC;
(d) Y.-D. Chen, P. R. D. Murray, A. T. Davies and M. C. Willis, J. Am. Chem. Soc., 2018, 140, 8781 CrossRef CAS PubMed;
(e) V. Vedovato, E. P. A. Talbot and M. C. Willis, Org. Lett., 2018, 20, 5493 CrossRef CAS.
- For recent selected examples of C(sp2)–H bond sulfonylation of arenes and heteroarenes, see:
(a) K.-D. Zhou, J. Zhang, L.-F. Lai, J. Cheng, J.-T. Sun and J. Wu, Chem. Commun., 2018, 54, 7459 RSC;
(b) H.-G. Xia, Y.-Y. An, X.-C. Zeng and J. Wu, Org. Chem. Front., 2018, 5, 366 RSC;
(c) T. Liu, W. Zhou and J. Wu, Org. Lett., 2017, 19, 6638 CrossRef CAS;
(d) H.-G. Xia, Y.-Y. An, X.-C. Zeng and J. Wu, Chem. Commun., 2017, 53, 12548 RSC;
(e) S.-Q. Ye, H.-H. Wang, Q. Xiao, Q.-P. Ding and J. Wu, Adv. Synth. Catal., 2014, 356, 3225 CrossRef CAS;
(f) K.-D. Zhou, M. Chen, L.-Q. Yao and J. Wu, Org. Chem. Front., 2018, 5, 371 RSC. For recent selected examples of C(sp2)–sulfonylation of alkenes, see:
(g) R. Mao, Z. Yuan, R. Zhang, Y. Ding, X. Fan and J. Wu, Org. Chem. Front., 2016, 3, 1498 RSC;
(h) X.-F. Wang, Y.-W. Li, G. Qiu and J. Wu, Org. Chem. Front., 2018, 5, 2555 RSC;
(i) J. Zhang, K.-D. Zhou and J. Wu, Org. Chem. Front., 2018, 5, 813 RSC;
(j) F.-S. He, X.-F. Cen, S.-Y. Yang, J. Zhang, H.-G. Xia and J. Wu, Org. Chem. Front., 2018, 5, 2437 RSC;
(k) J. Zhang, F. Zhang, L.-F. Lai, J. Cheng, J.-T. Sun and J. Wu, Chem. Commun., 2018, 54, 3891 RSC;
(l) F.-S. He, Y.-Q. Wu, J. Zhang, H.-G. Xia and J. Wu, Org. Chem. Front., 2018, 5, 2940 RSC;
(m) T. Liu, Y.-W. Li, L.-F. Lai, J. Cheng, J.-T. Sun and J. Wu, Org. Lett., 2018, 20, 3605 CrossRef CAS. For recent selected examples of C(sp3)–H bond sulfonylation from sulfur dioxide, see:
(n) Y. Li, R. Mao and J. Wu, Org. Lett., 2017, 19, 4472 CrossRef CAS;
(o) X.-X. Gong, J.-H. Chen, L.-F. Lai, J. Cheng, J.-T. Sun and J. Wu, Chem. Commun., 2018, 54, 11172 RSC. For a recent example on the arylsulfonylation of N-chloroamines, see:
(p) F. Zhang, D.-Q. Zheng, L.-F. Lai, J. Cheng, J.-T. Sun and J. Wu, Org. Lett., 2018, 20, 1167 CrossRef CAS.
-
(a) F. Liu, J.-Y. Wang, P. Zhou, G. Li, W.-J. Hao, S.-J. Tu and B. Jiang, Angew. Chem., Int. Ed., 2017, 56, 15570 CrossRef CAS;
(b) Z.-J. Shen, Y.-N. Wu, C.-L. He, L. He, W.-J. Hao, A.-F. Wang, S.-J. Tu and B. Jiang, Chem. Commun., 2018, 54, 445 RSC.
-
(a) D.-S. Yang, P.-F. Sun, W. Wei, F.-J. Liu, H. Zhang and H. Wang, Chem. – Eur. J., 2018, 24, 4423 CrossRef CAS;
(b) N.-W. Liu, S. Liang and G. Manolikakes, Adv. Synth. Catal., 2017, 359, 1308 CrossRef CAS;
(c) N. Wolff, J. Char, X. Frogneux and T. Cantat, Angew. Chem., Int. Ed., 2017, 56, 5616 CrossRef;
(d) H. Konishi, H. Tanaka and K. Manabe, Org. Lett., 2017, 19, 1578 CrossRef CAS;
(e) D.-L. Sun, K. Yin and R.-H. Zhang, Chem. Commun., 2018, 54, 1335 RSC;
(f) H.-B. Zhu, Y.-J. Shen, Q.-Y. Deng, C.-Y. Huang and T. Tu, Chem. – Asian J., 2017, 12, 706 CrossRef CAS;
(g) J. Sheng, Y.-W. Li and G. Qiu, Org. Chem. Front., 2017, 4, 95 RSC;
(h) K. Wang, G.-D. Wang, G.-Y. Duan and C.-C. Xia, RSC Adv., 2017, 7, 51313 RSC;
(i) H. Wang, S. Sun and J. Cheng, Org. Lett., 2017, 19, 5844 CrossRef CAS;
(j) Y. Wang, L.-L. Deng, J. Zhou, X.-C. Wang, H.-B. Mei, J.-L. Han and Y. Pan, Adv. Synth. Catal., 2018, 360, 1060 CrossRef CAS;
(k) H.-P. Wang, B.-B. Wang, S. Sun and J. Cheng, Org. Chem. Front., 2018, 5, 2547 RSC.
- D. Zheng, Y. An, Z. Li and J. Wu, Angew. Chem., Int. Ed., 2014, 53, 2451 CrossRef CAS.
- CCDC 1862564† contains the crystallographic data for this paper.
- D.-Q. Zheng, J.-Y. Yu and J. Wu, Angew. Chem., Int. Ed., 2016, 55, 11925 CrossRef CAS.
Footnote |
† Electronic supplementary information (ESI) available. CCDC 1862564. For ESI and crystallographic data in CIF or other electronic format see DOI: 10.1039/c8qo01144c |
|
This journal is © the Partner Organisations 2019 |
Click here to see how this site uses Cookies. View our privacy policy here.