DOI:
10.1039/C8RA09018A
(Paper)
RSC Adv., 2019,
9, 10927-10936
Retracted Article: Structural characterization of centipede oligopeptides and capability detection in human small cell lung carcinoma: inducing apoptosis
Received
31st October 2018
, Accepted 12th March 2019
First published on 8th April 2019
Abstract
Lung cancer is the most frequent cause of cancer deaths in the world, and smoking is considered as one of the major causes. Small cell lung carcinoma (SCLC) represents a highly malignant and particularly aggressive form, with properties of widespread metastases and poor prognosis. Herein, twenty-five Scolopendra subspinipes mutilans L. Koch Oligopeptides (SSMOs) were isolated and their structures were identified, and the anti-proliferative activity against lung cancer cell lines was evaluated. Results showed that SSMO-5 induced the production of reactive oxygen species (ROS) markedly in NCI-H446 cells. Furthermore, SSMO-5 decreased the mitochondrial membrane potential (MMP) and enhanced the mitochondria-related apoptosis. These results demonstrate that in NCI-H446 cells, the apoptotic and cytotoxic effects of SSMO-5 are mediated by the intrinsic mitochondria-mediated apoptotic pathway, which in turn causes the activation of caspases and increases Bax expression, while decreases Bcl-2 and Bcl-xL expressions and regulates the interaction of p53/MDM2. In conclusion, a ROS-mediated mitochondrial pathway plays an important role in the process of SSMO-5-induced apoptosis against SCLC.
1 Introduction
Lung cancer is a leading cause of cancer death worldwide, which is classified into two major groups, small cell lung carcinoma (SCLC) and non-small cell lung carcinoma (NSCLC).1 In contrast to NSCLC, SCLC is a highly fatal cancer with properties of wide-spread metastasis and fast resistance to chemotherapy. Despite initial sensitivity to chemotherapy, the survival rate of SCLC is still very low.2 Elderly SCLC patients and those with poor performance status are not treated with chemotherapy because of the high toxicity of chemotherapy.3 Therefore, novel methods that can improve the effect of chemotherapy for SCLC treatment are urgently required.
Pharmaceutical peptides have been recognized for years with their special biological properties of small molecular weight, endogenous targets, easy to penetrate tumor cells, significant immune response, and inhibiting tumor angiogenesis, tumor growth and metastasis, and such anti-tumor peptides have become a hot spot in cancer treatments.4 Researchers have screened dozens of anti-tumor peptides and have also proved that they can effectively inhibit the growth or occurrence of tumors. Various anti-tumor peptides have been successfully applied to improve the anticancer efficacy. For example, tyroserleutide can increase the concentration of Ca2+ in human hepatoma BEL-7402 cells, induce calcium overload and reduce the mitochondrial transmembrane potential (MTP), but have no harmful effects on normal liver cells. Tyroservaltide can induce necrosis and apoptosis of cancer cells by altering the ultrastructure and down-regulating the expression of proliferating cell nuclear antigen (PCNA). Thymopentin can promote the reconstruction of immune function, inhibit the proliferation, spread and metastasis of tumor, reduce the side effects and improve the quality of life. Furthermore, both medicinal herbs and animals provide a significant peptide resource, which can be explored for potential anticancer agents.5–7
The centipede (Scolopendra subspinipes mutilans L. Koch) is used in traditional Chinese medicine against different sorts of diseases, including cancer, apoplexy, and epilepsy.8,9 Several studies have demonstrated that water-soluble Scolopendra subspinipes mutilans L. Koch extracts induce tumor apoptosis and increase immune activity in tumor-bearing mice.10 However, to date, no work has focused on the Scolopendra subspinipes mutilans L. Koch peptide for lung cancer therapy. Herein, the Scolopendra subspinipes mutilans L. Koch peptides were isolated, their amino acid sequences were identified, and their potential anti-cancer effects against SCLC were also evaluated.
2 Materials and methods
2.1 Materials
The Scolopendra subspinipes mutilans L. Koch were acquired from the Ertiantang pharmacy (Guangzhou, China) and identified by Professor Zhou (Jinan University, Guangzhou, China). All the cell lines were obtained from the Cell Bank of the Chinese Academy of Sciences (Shanghai, China). CCD19 cells were grown in DMEM medium (Life Technologies, Grand Island, NY, USA). The reagents PI and JC-1 were obtained from Sigma Chemical Co. (St. Louis, MO, USA). The PierceTM BCA Protein Assay Kit was obtained from Thermo Fisher Scientific (Rockford, IL, USA). MTT, TUNEL Apoptosis Detection Kit, dithiothreitol (DTT), Nuclear and Cytoplasmic Extraction Kit, RIPA buffer and RNase were obtained from Beyotime (Shanghai, China). Phosphatase inhibitor cocktail tablets and protease inhibitor cocktail tablets were supplied by Roche (Mannheim, Germany). All the antibodies were obtained from Cell Signaling Technology (CST, Beverly, MA, USA). All the other chemicals were obtained from Sigma or Adamas and used without further purification.
2.2 Cell culture
All the cell lines were obtained from the Cell Bank of the Chinese Academy of Sciences (Shanghai, China). The CCD19 is a human lung fibroblast cell line, and the cells were cultured in 90% high glucose DMEM with 10% fetal bovine serum (FBS). The NCI-H446 is a human lung fibroblast cell line and the cells were cultured in RPMI-1640 with 10% FBS. The A549 is a human pulmonary alveolar epithelial cell line and the NCI-H1299 is a human non-small cell lung cancer cell line. Both the A549 and NCI-H1299 cells were cultured in DMEM with 10% FBS. All the culture media were added with penicillin (100 U mL−1), and streptomycin (100 mg mL−1). The cells were incubated at 37 °C in a humidified atmosphere with 5% CO2.
2.3 Isolation and identification of the SSMOs
2.3.1 Preparation of crude protein. All extraction and separation procedures were carried out at 4 °C. Scolopendra subspinipes mutilans L. Koch was minced to a homogenate and defatted according to a previously described method and with some improvements.11 The homogenate and iso-propanol were mixed in a ratio of 1
:
4 (w/v) and stirred for 4 h. The iso-propanol was replaced every 1 h. The supernatant was removed, and the sediment was lyophilized and stored at −20 °C.The defatted precipitate (100 g) was dissolved (5%, w/v) in 0.2 M phosphate buffer solution (PBS, pH 7.0); then, a KQ-250B ultrasonic cleaner (Shanghai, China) with straight probe and continuous pulse was used to ultrasound for 1 h. After centrifugation (8000 × g, 40 min), the supernatant was collected as total protein and fractionated by salting-out with increasing concentrations of ammonium sulfate, and the resulting supernatant was lyophilized and stored at −20 °C for further analysis.
2.3.2 Fractionation of SSMOs by ultrafiltration. The proteins were fractionated using ultrafiltration with 1 kDa molecular weight (MW) cut off membranes (Millipore, Hangzhou, China) for the lab scale. Two peptide fractions, named SSMO-A (MW < 1 kDa) and SSMO-B (MW > 1 kDa), were collected and lyophilized.
Hydrophobic chromatography. The SSMO-A was dissolved in 1.2 M NH4Cl prepared with 30 mM phosphate buffer (pH 8.0) and loaded onto a Phenyl Sepharose CL-4B hydrophobic chromatography column (3.0 cm × 60 cm) which had previously been equilibrated with the above buffer. A stepwise elution was performed with decreasing concentrations of NH4Cl (1.2, 0.6 and 0 M) dissolved in 30 mM phosphate buffer (pH 8.0) at a flow rate of 2.0 mL min−1. Each fraction was collected at a volume of 50 mL and was monitored at 280 nm. Fractions were then lyophilized and anticancer activity was detected.Herein, the anticancer activity of the lyophilized part was detected in A549 and NCI-H446 cells and the method was set as follows: the MTT (3-(4,5-dimethylthiazol-2-yl)2,5-diphenyl tetrazolium bromide) assay was used to evaluate cell viability. After treatment with the lyophilized part (0.1 mg mL−1) for 48 h, the cells were washed with PBS. Then, MTT (0.5 mg mL−1) was added to each well and the mixture was incubated at 37 °C for 0.5 h, and the absorbance of each well was detected at 550 nm with a microplate reader (Bio-Tek, USA).
The SSMO-A-3 fraction had the strongest anti-proliferative activity against A549 and NCI-H446 cells, and further isolation was performed by anion-exchange chromatography with SSMO-A-3.
2.3.3 Anion-exchange chromatography of SSMO-A-3. The SSMO-A-3 solution (3 mL, 50.4 mg mL−1) was injected into a DEAE-52 cellulose (Yuanju, Shanghai, China) anion-exchange column (1.6 × 70 cm) pre-equilibrated with deionized water and was stepwise eluted with 500 mL distilled water, 0.1, 0.4, and 0.8 M NaCl solutions at a flow rate of 2.0 mL min−1. Each eluted fraction (50 mL) was collected and detected at 280 nm. Ten fractions (A-SSMO-3-1 to A-SSMO-3-10) were lyophilized and anticancer activity was detected. The SSMO-A-3-2 fraction had the strongest anti-proliferative activity against A549 and NCI-H446 cells, and further isolation was performed by gel filtration chromatography with SSMO-A-3-2.
2.3.4 Gel filtration chromatography of SSMO-A-3-2. The SSMO-A-3-2 solution (3 mL, 18.3 mg mL−1) was fractionated on a Sephadex G-25 (Sigma-Aldrich, Shanghai, China) column (3.0 × 150 cm) at a flow rate of 1.5 mL min−1. Each elute (10 mL) was collected and monitored at 280 nm, and six fractions (SSMO-A-3-2-1 to SSMO-A-3-2-6) were collected and lyophilized, and the anticancer activity was detected. The SSMO-A-3-2-5 fraction had the strongest anti-proliferative activity against A549 and NCI-H446 cells, and further isolation was performed by reversed phase high-performance liquid chromatography (RP-HPLC) with SSMO-A-3-2-5.
2.3.5 Isolation of peptides from SSMO-A-3-2-5 by RP-HPLC. SSMO-A-3-2-5 was finally separated by RP-HPLC (Agilent 1200 HPLC) on a Zorbax, SB C-18 column (4.6 × 250 mm, 5 μm). The elution solvent system was composed of water–trifluoroacetic acid (solvent A; 100
:
0.1, v/v) and acetonitrile–trifluoroacetic acid (solvent B; 100
:
0.1, v/v). The peptide was separated using gradient elution from 30% to 75% of solvent B for 50 min at a flow rate of 1.0 mL min−1. The detection wavelength was set at 280 nm and the column temperature was 20 °C; the peptides were isolated and lyophilized.
2.3.6 Amino acid sequence analysis and molecular mass determination by HPLC-ESI-MS. Prior to the HPLC-ESI-MS analysis, the lyophilized mixture was rehydrated with 1.0 mL of Milli-Q water. Before being used, the water was boiled for 5 min and then cooled to 4 °C. The rehydrated solution was stored at −20 °C until analysis.HPLC-ESI-MS was carried out on a SCIEX X500R Q-TOF mass spectrometer (Framingham, U.S.A.). The MS conditions were as follows: ESI-MS analysis was performed using a SCIEX X500R Q-TOF mass spectrometer equipped with an ESI source. The mass range was set at m/z 100–1000. The Q-TOF MS data were acquired in the positive mode, and the conditions of MS analysis were as follows: CAD gas flow-rate, 7 L min−1; drying gas temperature, 550 °C; ion spray voltage, 5500 V; declustering potential, 80 V. Software generated data file: SCIEX OS 1.0.
2.4 MTT assay
After the treatment with SSMOs for 48 h, the cells were washed with PBS. Then, MTT (0.5 mg mL−1) (St. Louis, MO, USA) was added to each well and the mixture was incubated at 37 °C for half an hour. Later, the absorbance of each well was detected at 550 nm with a microplate reader (Bio-Tek, USA).12
2.5 Cell apoptosis assay
NCI-H446 cells were treated with SSMO-5 at 3.84, 19.2 and 96.0 μM for 48 h; then, the apoptosis was detected by Annexin V and PI staining according to the manufacturer's protocol (Beyotime, Shanghai, China). The cells were suspended in 100 μL Annexin V binding buffer and 5 μL Annexin V and PI were added to the samples. After 30 min of incubation at r.t, the flow cytometric analysis was performed (BD FACS Calibur, Franklin Lakes, CA, USA).13 All the tests were repeated at least 3 times.
2.6 Cell cycle analysis
The cell cycle distribution was also detected in NCI-H446 cells and analyzed by PI (propidium iodide) Beyotime (Shanghai, China) staining with flow cytometry. NCI-H446 cells were exposed to SSMO-5 at 3.84, 19.2 and 96.0 μM for 48 h. After that, the cells were harvested and fixed in 70% ethanol and stored at −20 °C for 12 h. Then, the cells were washed with PBS again and stained with PI and analyzed by flow cytometry (BD FACS Calibur, Franklin Lakes, CA, USA).14
2.7 Determination of ROS generation
The levels of ROS generation were observed by the fluorogenic probe, 2′,7′-dichlorodihydrofluorescein (H2DCFDA) Beyotime (Shanghai, China). Cells were plated at a density of 2 × 105 and exposed to SSMO-5 at 3.84, 19.2 and 96.0 μM for 48 h, and at 19.2 μM for 0, 2, 4, 8, 12, 24 and 48 h. The cells were stained with H2DCFDA for half an hour at 37 °C, and then, fluorescence intensity was detected.15
2.8 Determination of mitochondrial membrane potential
Cells (2 × 105 per well) were plated in 6-well culture dishes, and treated with SSMO-5 at 3.84, 19.2 and 96.0 μM for 48 h, and at 19.2 μM for 0, 2, 4, 8, 12, 24 and 48 h. After washing with PBS, the cells were stained for half an hour with JC-1 Beyotime (Shanghai, China) at 37 °C. Afterwards, the stained cells were analyzed with flow cytometry (BD FACS Calibur, Franklin Lakes, CA, USA).16
2.9 Western blot analysis
Cells were plated in 6-well culture dishes, grown to confluence, and treated with SSMO-5 for 48 h. After incubation, the cells were washed with ice-cold PBS, scraped, pelleted and lysed in a radioimmunoprecipitation assay (RIPA) buffer (protease inhibitor cocktail and phosphatase inhibitor) (Mannheim, Germany). After incubation for 1 h on ice, the cell lysates were centrifuged at 3000 g for half an hour at 4 °C.
The lysate protein concentrations were detected by a BCA protein assay kit (Rockford, IL, USA), and the lysates were adjusted with a lysis buffer. Proteins were resolved on a 10–15% SDS-PAGE and transferred to immobilon polyvinyldifluoride (PVDF) membranes. The blots were blocked with a blocking buffer for 10 min at r.t and then probed with antibodies for 1 h at room temperature. Then, the blots were incubated with a peroxidase-conjugated donkey anti-rabbit secondary antibody (1
:
3000 dilutions) for 1 h at room temperature.
2.10 Statistics
Data analysis was performed with Graphpad Prism 5 (San Diego, USA). The statistical comparisons between the control and treatments were performed by the Student's t-test. All the experiments were performed at least three times. Data are presented as mean ± SD. p < 0.05 is considered statistically significant.
3 Results and discussion
3.1 Isolation and identification of oligopeptides
We have successfully isolated fifteen oligopeptides from Scolopendra subspinipes mutilans L. Koch. Their structures were identified by HPLC-ESI-MS analysis. The peptides were usually protonated under ESI-MS/MS conditions, and fragmentations mostly occurred at the amide bonds, because it is difficult to break the chemical bonds of the side chains at a low energy. Therefore, the b and y ions were the main fragment ions when the collision energy was <200 eV.17 SSMO-5 was analyzed by HPLC-ESI-MS for molecular mass determination and peptide characterization. The molecular mass of the peptide was determined to be 617.3226 Da. The ion fragment m/z 413.2359 was regarded as the b3 ion, and m/z 395.2183 was regarded as the [b3-H2O + H]+ ion. While, m/z 266.1615 was regarded as the b2 ion, and m/z 248.1532 was regarded as the [b2-H2O + H]+ ion. The m/z 129.1018 was the typical fragment [Lys-H2O + H]+ ion, and m/z 110.0706 was the [Lys-2H2O + H]+ ion. On the basis of this, we concluded that the sequence of the peptide was HKFW. The rest of the SSMOs were also identified, and after the analysis by MS/MS spectra processing with BioTools database, the amino acid sequences of the twenty-five oligopeptides were determined (listed in Table 1).
Table 1 Amino acid sequences and cell growth inhibition of SSMOs against cancer cell linesa
SSMOs |
Amino acid sequences |
IC50 (μM) |
NCI-H446 |
NCI-H1299 |
A549 |
IC50 values are shown as mean ± standard error of the mean (SD) from at least three independent experiments. |
SSMO-1 |
GE |
>120 |
>120 |
>120 |
SSMO-2 |
GH |
>120 |
>120 |
>120 |
SSMO-3 |
KL |
116 ± 11.2 |
92.3 ± 8.63 |
>120 |
SSMO-4 |
GAEG |
>120 |
>120 |
>120 |
SSMO-5 |
HKFW |
19.2 ± 1.51 |
20.3 ± 1.68 |
27.4 ± 2.23 |
SSMO-6 |
DKHEFS |
30.5 ± 3.38 |
35.2 ± 4.61 |
40.5 ± 3.90 |
SSMO-7 |
LWEHSH |
51.3 ± 4.26 |
62.3 ± 6.29 |
85.4 ± 7.93 |
SSMO-8 |
FSTHGG |
>120 |
116 ± 11.7 |
>120 |
SSMO-9 |
SHGEWL |
>120 |
>120 |
>120 |
SSMO-10 |
FSHTYV |
56.2 ± 4.15 |
48.2 ± 3.38 |
56.2 ± 6.03 |
SSMO-11 |
KYRFLL |
36.5 ± 3.51 |
37.4 ± 2.58 |
44.2 ± 3.54 |
SSMO-12 |
KLHLHS |
71.4 + 7.04 |
66.0 + 5.61 |
101 + 11.6 |
SSMO-13 |
HKFW |
55.6 ± 3.94 |
108 ± 9.82 |
113 ± 12.7 |
SSMO-14 |
FWEH |
96.2 ± 8.54 |
110 ± 9.91 |
98.2 ± 9.03 |
SSMO-15 |
GEGVFGH |
50.2 ± 6.17 |
61.7 ± 7.04 |
82.1 ± 7.55 |
SSMO-16 |
FEHSG |
>120 |
81.2 ± 7.40 |
103 ± 11.2 |
SSMO-17 |
HESGWL |
36.6 ± 4.23 |
72.3 ± 6.37 |
61.3 ± 6.32 |
SSMO-18 |
KSHEFG |
>120 |
104 ± 10.2 |
>120 |
SSMO-19 |
KYAVHS |
104 ± 9.77 |
>120 |
107 ± 11.7 |
SSMO-20 |
DRHSFL |
61.5 ± 4.97 |
>120 |
101 ± 11.1 |
SSMO-21 |
LYEHGSW |
>120 |
41.4 ± 3.73 |
>120 |
SSMO-22 |
TYKRHS |
>120 |
108 ± 10.1 |
>120 |
SSMO-23 |
DSHTS |
26.4 ± 2.40 |
39.2 ± 2.57 |
41.8 ± 3.31 |
SSMO-24 |
TKFSYE |
50.2 ± 6.17 |
112 ± 12.0 |
62.1 ± 5.59 |
SSMO-25 |
KESHLF |
>120 |
81.2 ± 7.40 |
102 ± 8.92 |
3.2 SSMOs inhibited the growth of cancer cells
After the treatment of SSMOs, the MTT assay was used to detect the anti-proliferative activity of SSMOs against human lung cancer cells. The NCI-H446, NCI-H1299 and A549 cells were treated with different concentrations of SSMOs for 48 h, and the results are listed in Table 1.
From the results, we know that most of the SSMOs displayed a significant anti-proliferative activity, and SSMO-5 showed excellent anti-proliferative effects against the cancer cell lines, the IC50 to NCI-H446 was 19.2 ± 1.51 μM. Further study showed that SSMO-5 induced NCI-H446 cell death in a dose-dependent manner. The current evidence supports that the SSMO-5 is probably a promising anticancer agent. Therefore, the cytotoxicity against normal cell line from healthy tissues must be evaluated. Herein, the inhibition against the normal cell line was detected. The testing method was the same as that applied to cancer cell line. It was found that the concentration of SSMO-5 required to induce cytotoxicity against human lung fibroblast CCD19 is much higher compared to that required for the cancer cell lines. Comparing the IC50 values at the same incubation time (48 h), the cytotoxicity against CCD19 cells was 25.6 times lesser than that against NCI-H446 cancer cells. Definitely, SSMO-5 significantly showed an attenuated cytotoxicity effect against normal cells.
3.3 SSMO-5-induced apoptosis in NCI-H446 cells
Apoptosis, the process of programmed cell death, is an important therapy target, and the affected cells are different from the normal cells with certain distinct morphological features, such as cytoplasmic shrinkage, membrane blebbing and chromatin condensation.18 Since SSMO-5 was indicated to have the greatest enhancing effect against NCI-H446 (Table 1), together with the fact that small cell lung carcinoma (SCLC) represents a highly malignant and particularly aggressive form, with properties of widespread metastases and poor prognosis, the development of novel and effective therapies for this devastating disease is urgently needed. This is the reason why we chose the NCI-H446 cell line to explore the mechanisms involved in the prevention of the cancer cell growth by SSMO-5 (Fig. 1).
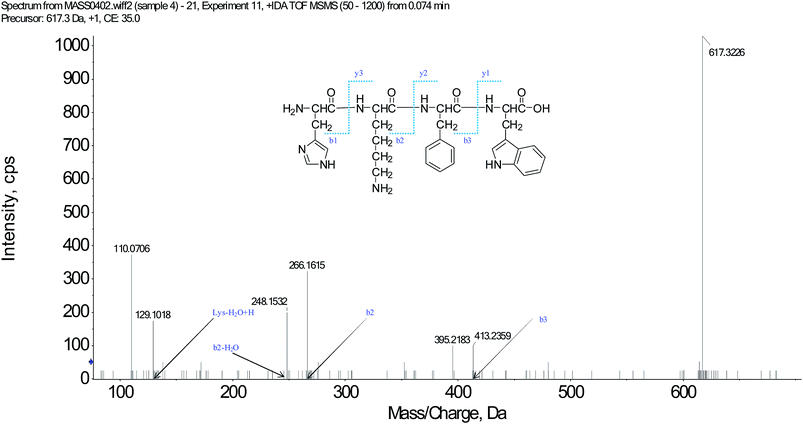 |
| Fig. 1 Structure and MS/MS spectra of SSMO-5 (m/z = 617.3226). | |
In order to investigate whether SSMO-5 can induce the NCI-H446 cell apoptosis, flow cytometry using propidium iodide (PI) and Annexin-V with NCI-H446 cells was performed. After the NCI-H446 cells were treated with SSMO-5 at 3.84, 19.2 and 96.0 μM for 48 h, the cells were labeled with the two dyes and the mixture was monitored by flow cytometry.
As shown in Fig. 2, at the dose of 3.84 μM, SSMO-5 induced 5.16% total apoptosis/necrosis and 1.32% early apoptosis, where 2.96% of cell death was caused by necrosis and 0.88% by late apoptosis. Interestingly, at the high dose of 96 μM, SSMO-5 caused 25.2% total apoptosis/necrosis and 9.04% early apoptosis, where 15.1% was by necrosis and 1.11% by late apoptosis. In both cases, necrosis contributed to cell death to a great extent. The early apoptosis rate changed from negligible to clearly visible. This indicated that the anti-cancer mechanism of SSMO-5 involved was inducing early apoptosis of the cancer cells. Furthermore, SSMO-5 exhibited a significant apoptosis against NCI-H446 cells, and performed the capability in a dose-dependent manner.
 |
| Fig. 2 SSMO-5-induced apoptosis in NCI-H446 cells. Representative scatter diagrams. NCI-H446 cells were pre-treated without the addition of any samples, the control (C) (I), with a dose of 3.84 μM SSMO-5 (II), with a dose of 19.2 μM SSMO-5 (III), and with a dose of 96.0 μM SSMO-5 (IV), respectively, for 48 h. Cells were stained with Annexin-V and PI. The apoptosis of NCI-H446 cells was detected by flow cytometry. The evaluation of apoptosis was via Annexin V: FITC Apoptosis Detection Kit per manufacture's protocol. The quantitative results are also shown. In each scatter diagram, the abscissa represents the fluorescence intensity of the cells dyed by Annexin V, and the ordinate represents the fluorescence intensity of the cells dyed by PI. The lower left quadrant shows the viable cells, the upper left shows the necrotic cells, the lower right shows the early apoptotic cells, and the upper right shows the late apoptotic cells. | |
As we know, necrosis is a form of traumatic cell death that results from acute cellular injury; while, apoptosis is a highly regulated and controlled process that confers advantages during an organism's lifecycle.19 Unlike necrosis, apoptosis produces cell fragments called apoptotic bodies that phagocytic cells are able to engulf and quickly remove before the contents of the cell can spill out onto the surrounding cells and cause damage to the neighboring cells. An early marker of apoptosis is the exposition of phosphatidylserine on the cell surface, whereas it is normally concentrated in the luminal layer of the cytoplasmic membrane.20 SSMO-5 promoted a strong early apoptosis/necrosis, and the anti-cancer mechanism was further explored.
3.4 SSMO-5-induced cell cycle arresting in NCI-H446 cells
The main property of tumor cells is uncontrollable cell growth, and the cell cycle arresting becomes a pivotal therapy target in remedies.21 The above studies showed that SSMO-5 can induce apoptosis; therefore, the effect of SSMO-5 on the cell cycle was investigated by flow cytometry (Fig. 3).
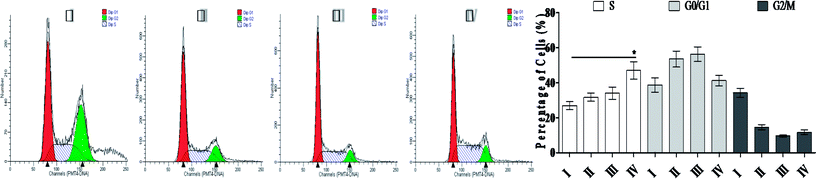 |
| Fig. 3 Cell cycle analysis of NCI-H446 cells exposed to SSMO-5. NCI-H446 cells were pre-treated without the addition of any samples, the control (C) (I), with a dose of 3.84 μM SSMO-5 (II), with a dose of 19.2 μM SSMO-5 (III), and with a dose of 96.0 μM SSMO-5 (IV), respectively, for 48 h. C was the control group, without the addition of any tested compounds. Cells were collected, fixed in 70% ethanol, and stained with propidium iodide solution. G0/G1: quiescent state/growth phase; S: initiation of DNA replication; G2/M: biosynthesis/mitosis phases. | |
On treatment with SSMO-5 at 3.84, 19.2 and 96.0 μM for 48 h, compared with the control group, an accumulation of 4.81%, 7.04% and 20.1% at the S phase was observed, and compared with the control group, SSMO-5 clearly arrested NCI-H446 cells in the S phase. These data suggested that SSMO-5 may possess a strong ability of inducing cell cycle arresting in NCI-H446 cells.
This fact suggests that the cell cycle arrest is one of the primary mechanisms responsible for the anticancer activities of SSMO-5. SSMO-5 alters the manner of cell cycle arrest to the S state greatly. Usually, the ensuing S phase starts when DNA synthesis commences; when this phase is complete, all of the chromosomes would have been replicated, i.e., each chromosome will have two (sister) chromatids. If DNA can't be replicated, the cell cycle will be stopped at this stage.22 Based on the current result, it was suggested that SSMO-5 may inhibit the DNA replication and make the cell cycle stop in the S phase.
3.5 SSMO-5 increased the ROS level in NCI-H446 cells
ROS generation plays an important role in apoptosis.23 Oxidative stress results from an imbalance between free radical generation and antioxidant defenses, and is considered to be an important pathogenic mechanism.24 Oxidative stresses refers to elevated levels of intracellular ROS that cause damage to lipids, proteins and DNA and other macromolecules that can regulate the initiation of apoptotic signaling. We therefore evaluated whether the accumulation of ROS is involved in SSMO-5-induced cell apoptosis. Cells were exposed to SSMO-5 at 3.84, 19.2 and 96.0 μM for 48 h, and 19.2 μM for 0, 2, 4, 8, 12, 24 and 48 h, and the level of ROS was explored by fluorescence microscopy following staining with H2DCFDA. The ROS in the control group was regarded as 1, and ROS level in the other groups is the relative value of the control group. As shown in Fig. 4A and B, on treatment with SSMO-5 at 3.84, 19.2 and 96.0 μM for 48 h, compared with the control group, an up-regulation of 1.98, 6.66 and 18.2 folds of the relative ROS level was observed. While, on treatment with SSMO-5 at 19.2 μM for 0, 2, 4, 8, 12, 24 and 48 h, compared with the control group, a 7.01-fold up-regulation of the relative ROS level was observed at 24 h. The results showed that the treatment of SCLC cells with SSMO-5 induced the accumulation of ROS in a dose- and time-dependent manner, and also demonstrated that ROS are significant factors in the induction of apoptosis and act upstream signaling molecules to initiate cell apoptosis.
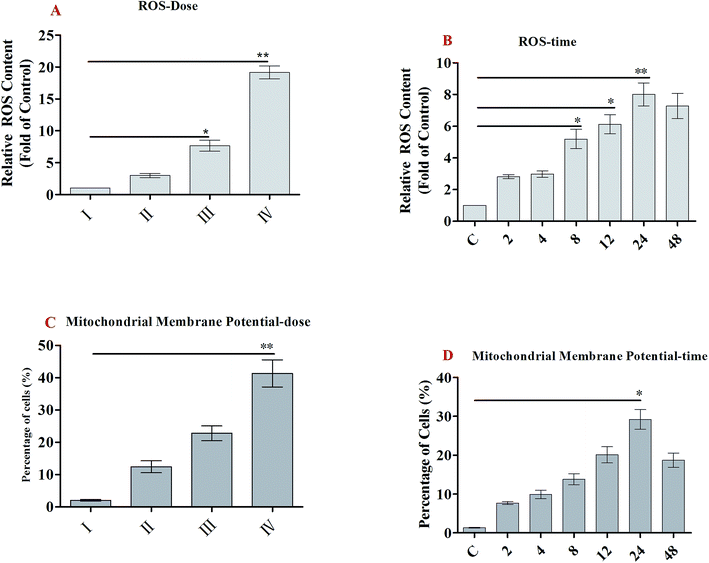 |
| Fig. 4 ROS generation and MMP decline of NCI-H446 cells exposed to SSMO-5. (A and B) NCI-H446 cells were pre-treated without the addition of any samples, the control (C) (I), with a dose of 3.84 μM SSMO-5 (II), with a dose of 19.2 μM SSMO-5 (III), and with a dose of 96.0 μM SSMO-5 (IV), respectively, for 48 h. C was the control group, without the addition of any tested compounds. Cells were treated with 19.2 μM SSMO-5 for 0, 2, 4, 8, 12, 24 and 48 h at 37 °C, stained with DCFH-DA for 20 min, and analyzed for fluorescence by flow cytometry. All data are presented as the mean ± SD of three independent experiments. *p < 0.05 and **p < 0.01. (C and D) Flow cytometry analysis of MMP based on JC-1 staining. NCI-H446 cells were pre-treated without the addition of any samples, the control (C) (I), with a dose of 3.84 μM SSMO-5 (II), with a dose of 19.2 μM SSMO-5 (III), and with a dose of 96.0 μM SSMO-5 (IV), respectively, for 48 h. C was the control group, without the addition of any tested compounds. Cells were treated with 19.2 μM SSMO-5 for 0, 2, 4, 8, 12, 24 and 48 h at 37 °C and stained with JC-1. The cells showing a loss of MMP were gated. All data are presented as the mean ± SD of three independent experiments. *p < 0.05 and **p < 0.01. The data shown are representative of three independent experiments. | |
3.6 SSMO-5-induced mitochondrial dysfunction and regulation of the proteins of Bcl-2 family
The mechanism of apoptosis can be initiated by two major apoptotic pathways, the intrinsic pathway (mitochondria-mediated) and the extrinsic pathway (death receptor-mediated).25 The intrinsic mitochondrial pathway is activated by multiple stimuli that converge at the mitochondrion and induce MMP and subsequently result in the release of pro-apoptotic mitochondrial proteins into the cytosol.26 Bcl-2 prevents the release of cyt c into the cytoplasm and thus blocks cyt c from promoting Apaf-1-mediated caspase-9 activation, leading some to hypothesize that Bcl-2 and its homologues function to keep the mitochondrial membranes intact.27
Herein, we hypothesized that mitochondria-initiated ROS activation mediates SSMO-5-induced cell death. The disruption of MMP results in the release of cytochrome c from mitochondria into the cytoplasm. ROS induce cell apoptosis by regulating the proapoptotic Bcl-2 family of proteins, such as the Bcl-2-associated X protein (Bax) and Bcl-2-antagonistic/killer (Bak), resulting in an increase in mitochondrial membrane permeabilization and cytochrome c released into the cytosol.28
Mitochondrial membrane protein (MMP) was detected with the mitochondria sensitive fluorescent dye, JC-1, by flow cytometry. As shown in Fig. 4C and D, on treatment with SSMO-5 at 3.84, 19.2 and 96.0 μM for 48 h, compared with the control group, the cells with mitochondrial membrane potential decline increased by 12.4%, 22.8% and 41.3%, respectively. While, on treatment with SSMO-5 at 19.2 μM for 0, 2, 4, 8, 12, 24 and 48 h, compared with the control group, the cells with MMP decline increased by 29.2% at 24 h. In conclusion, the treatment of NCI-H446 cells with SSMO-5 induced marked changes in MMP, and it occurred in a dose- and time-dependent manner.
Western blot analysis showed that treatment of NCI-H446 cells with SSMO-5 increased the levels of Bax and Bak, and reduced the expression of Bcl-xL and Bcl-2 (Fig. 5A). Therefore, we inferred that mitochondrial dysfunction may also be involved in SSMO-5-mediated cell apoptosis in the NCI-H446 cells. Furthermore, SSMO-5 also reduced mitochondrial cytochrome c expression (Fig. 5A).
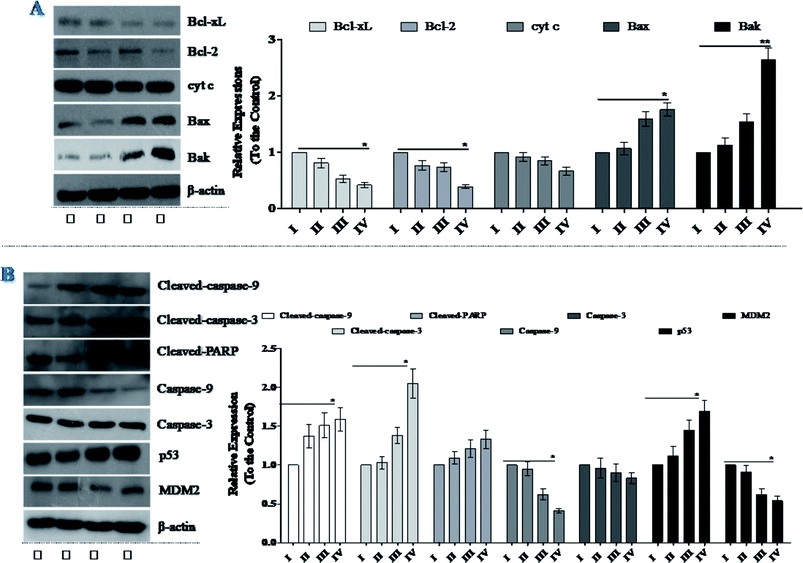 |
| Fig. 5 Effects of SSMO-5 on the expressions of Bcl-2 family, caspases and p53/MDM2. NCI-H446 cells were pre-treated without the addition of any samples, the control (C) (I), with a dose of 3.84 μM SSMO-5 (II), with a dose of 19.2 μM SSMO-5 (III), and with a dose of 96.0 μM SSMO-5 (IV), respectively, for 48 h. C was the control group, without the addition of any tested compounds. The protein expression levels were measured using western blot. The density of each lane was presented as mean ± standard deviation (SD) for at least three individual experiments. Blots were quantified using Image J software. β-Actin was used as the internal control. | |
All these results suggest that SSMO-5-induced cell apoptosis may take place through the mitochondrial dysfunction in NCI-H446 cells.
3.7 SSMO-5 induced the activation of caspases in NCI-H446 cells
Caspases are a family of cysteine protease enzymes that play an essential role in programmed cell death.29 The proteolytic cascade of caspases mediates cell apoptosis. We therefore examined the involvement of caspases in SSMO-5-induced apoptosis. In cells treated with SSMO-5, the levels of cleaved-PARP, cleaved-caspase-3 and cleaved-caspase-9 significantly increased, while, the caspase-3 and caspase-9 expressions were down-regulated significantly (Fig. 5B). These results demonstrate that SSMO-5 may induce apoptosis in SCLC cells via a caspase-dependent pathway.
3.8 SSMO-5 regulated the interaction of p53/MDM2
We noticed the fact that small molecules can inhibit the interaction of p53/MDM2, in which p53 is a tumor suppressor which plays a role in inducing cell cycle arrest, DNA repair, senescence, and apoptosis,30 while MDM2 (murine double minute 2) is the main endogenous negative regulator.31 This oncoprotein MDM2 binds to p53 and negatively regulates p53 activity by the direct inhibition of p53 transcriptional activity and enhancement of p53 degradation via the ubiquitin-proteasome pathway. The inhibition of the p53/MDM2 interaction to restore p53 activity represents an appealing therapeutic strategy for many wild-type p53 tumors with over expressed MDM2.32 Therefore, we were interested in whether SSMO-5 can regulate the p53/MDM2 interaction.
As expected, it was found that SSMO-5 up-regulated p53 expression; however, on the other hand, it down-regulated MDM2 expression (Fig. 5B). Interestingly, SSMO-5 showed great activity in the regulation of p53/MDM2, which places these two proteins in a better healthy state. This positive effect might be the key mechanism by which SSMO-5 induces cancer cell death.
4 Conclusion
As one of the most frequently diagnosed cancers, SCLC represents about 15% of lung neoplasms.33 Compared with conventional chemotherapy, the surgical treatment of SCLC is much more effective. However, the high recurrence rate is still a difficult problem. Thus, agents that inhibit cell growth and induce cell death may be useful in the treatment of SCLC.
Previous studies have successfully identified that the pharmaceutical peptides have promising anticancer capacities, which can be exploited as potential anticancer agents.34 The centipede (Scolopendra subspinipes mutilans L. Koch) is a traditional Chinese medicine, which has been used to treat cancer for decades. Studies have shown that the extract of Scolopendra subspinipes mutilans L. Koch has significant antitumor capability.35,36 Herein, we showed that SSMO-5, a novel Scolopendra subspinipes mutilans oligopeptide, is effective in inducing cell apoptosis in the SCLC NCI-H446 cells. The SSMO-5 effectively inhibited the growth of NCI-H446 cells in a dose-dependent manner. Interestingly, we found that SSMO-5 inhibited cell apoptosis in the human SCLC cell lines but not in the normal lung fibroblasts. These results indicate that SSMO-5 may be more efficient at inducing cell apoptosis in SCLC NCI-H446 cells.
Mitochondria play a significant role in the apoptotic process by integrating numerous apoptotic signals.37 In addition, mitochondria coordinate the caspase-dependent and independent degradation steps of apoptosis. Mitochondrial dysfunction, including the loss of mitochondrial membrane potential, permeability transition, and release of cytochrome c into the cytosol, is associated with apoptosis.38 Intracellular ROS function as trigger or signaling molecules to initiate the downstream events in regulating cell cycle, cell differentiation and apoptosis. Our results showed that SSMO-5 induced ROS production, decreased the levels of MMP, and promoted the activation of caspases to induced apoptosis through the mitochondrial apoptotic pathway.
Mitochondrial membrane permeabilization is a central process in programmed cell death pathways and is regulated by Bcl-2 family members via multiple molecular mechanisms.39 Although Bcl-2 can prevent apoptosis, Bax triggers cytochrome c release in cells. Bcl-2 and Bcl-xL are frequently over expressed in SCLC tumors. The over expression of antiapoptotic Bcl-2 family members is known to cause resistance to apoptosis and provide therapy in a wide range of tumors; the development of therapies that target these apoptosis modulators appears to be a promising approach. Herein, we showed that SSMO-5 induced caspase-dependent apoptosis through the mitochondrial pathway, accompanied by the decreased Bcl-2 and Bcl-xL expression in SCLC cell lines.
In conclusion, we have provided a new opportunity of using SSMO-5 in SCLC therapy. Moreover, we detected that the mechanism of SSMO-5-induced apoptosis maybe via the up-regulation of ROS, mitochondrial dysfunction and caspase activation. These findings showed that SSMO-5 may be a potential anti-cancer drug in SCLC treatment.
Conflicts of interest
The authors declare no conflicts of interest.
Acknowledgements
We thank Jennifer Smith, PhD, for editing the English text of the draft of this manuscript. The authors are greatly thankful to the Shenzhen City Key Project of Science and Technology Program (No. JCYJ20140418095735538) for financial support, valuable suggestions and guidelines.
References
- L. A. Torre, R. L. Siegel and A. Jemal, Lung Cancer Statistics, Adv. Exp. Med. Biol., 2016, 893, 1 CrossRef PubMed.
- Y. H. Kim, K. Goto, K. Yoh, S. Niho, H. Ohmatsu, K. Kubota, N. Saijo and Y. Nishiwaki, Performance status and sensitivity to first-line chemotherapy are significant prognostic factors in patients with recurrent small cell lung cancer receiving second-line chemotherapy, Cancer, 2008, 113, 2518–2523 CrossRef PubMed.
- F. Manapov, S. Klöcking, M. Niyazi, C. Belka, G. Hildebrandt, R. Fietkau and G. Klautke, Chemoradiotherapy duration correlates with overall survival in limited disease SCLC patients with poor initial performance status who successfully completed multimodality treatment, Strahlenther. Onkol., 2012, 188, 29–34 CrossRef CAS PubMed.
- V. R. Dirisala, R. R. Nair, K. Srirama, P. N. Reddy, K. R. S. S. Rao, N. S. S. Kumar and G. Parvatam, Recombinant pharmaceutical protein production in plants: unraveling the therapeutic potential of molecular pharming, Acta Physiol. Plant., 2017, 39, 18 CrossRef.
- F. U. Zheng, L. U. Rong, L. I. Guoli, L. Zhao, W. Gao, X. Che, J. Xu, C. Zhou and Z. Yao, Tyroserleutide tripeptide affects calcium homeostasis of human hepatocarcinoma BEL-7402 cells, Chin. Sci. Bull., 2005, 48, 523–530 Search PubMed.
- J. Jing, L. Rong, Q. Shuang, H. Li, X. Che, P. Zhao, M. Jin, H. Yang, L. Gang and Y. Zhi, Preliminary investigation of the inhibitory effects of the tyroservaltide (YSV) tripeptide on human hepatocarcinoma BEL-7402, Cancer Biol. Ther., 2005, 4, 993 CrossRef.
- L. V. Xiao-Yu and S. Y. Zhang, Effects of thymopentin on lung function, cellular immune function and inflammatory factor of the elderly patients with chronic obstructive pulmonary disease, China J. Mod. Med., 2014, 2014(7), 56–59 Search PubMed.
- J. H. Lee, I. W. Kim, S. H. Kim, M. A. Kim, E. Y. Yun, S. H. Nam, M. Y. Ahn, D. C. Kang and J. S. Hwang, Anticancer activity of the antimicrobial peptide scolopendrasin VII derived from the centipede, Scolopendra subspinipes mutilans, J. Microbiol. Biotechnol., 2015, 25, 1275 CrossRef CAS PubMed.
- L. U. Guo-Dong, Ecological Habits and Clinical Practice of Medical Centipede, Sichuan Journal of Zoology, 2006, 123–125 Search PubMed.
- Y. Q. Zhou, L. Han, Z. Q. Liu, K. C. Du and K. Y. Li, Effect of centipede extract on cervical tumor of mice and its mechanism, Zhongyaocai, 2011, 34, 859 Search PubMed.
- C. Yu, C. Jie and Y. L. L. Xiong, Chromatographic separation and tandem MS identification of active peptides in potato protein hydrolysate that inhibit autoxidation of soybean oil-in-water emulsions, J. Agric. Food Chem., 2010, 58, 8825–8832 CrossRef.
- L. Rossig, J. Haendeler, Z. Mallat, B. Hugel, J. Freyssinet, A. Tedgui, S. Dimmeler and A. Zeiher, Congestive heart failure induces endothelial cell apoptosis: protective role of carvedilol, J. Am. Coll. Cardiol., 2000, 36, 2081–2089 CrossRef CAS.
- R. C. Wilkins, B. C. Kutzner, M. Truong, J. Sanchezdardon and J. R. Mclean, Analysis of radiation-induced apoptosis in human lymphocytes: flow cytometry using Annexin V and propidium iodide versus the neutral comet assay, Cytometry, 2002, 48, 14–19 CrossRef CAS.
- P. Smolewski, Flow cytometry in analysis of cell cycle and apoptosis, Semin. Hematol., 2001, 38, 179–193 CrossRef.
- K. A. Szychowski, K. Rybczyńska-Tkaczyk, M. L. Leja, A. K. Wójtowicz and J. Gmiński, Tetrabromobisphenol A (TBBPA)-stimulated reactive oxygen species (ROS) production in cell-free model using the 2′,7′-dichlorodihydrofluorescein diacetate (H2DCFDA) assay-limitations of method, Environ. Sci. Pollut. Res., 2016, 23, 12246–12252 CrossRef PubMed.
- G. Kalashnikova, A new method for the cytofluorimetric analysis of mitochondrial membrane potential using the J-aggregate forming lipophilic cation 5,5',6,6'-tetrachloro-1,1',3,3'-tetraethylbenzimidazolcarbocyanine iodide (JC-1), Biochem. Biophys. Res. Commun., 1993, 30, 40–45 Search PubMed.
- G. Sonsmann, A. Römer and D. Schomburg, Investigation of the influence of charge derivatization on the fragmentation of multiply protonated peptides, J. Am. Soc. Mass Spectrom., 2002, 13, 47 CrossRef CAS.
- C. S. Lai, R. H. M. H. Mas, N. K. Nair, M. I. A. Majid, S. M. Mansor and V. Navaratnam, Typhonium flagelliforme inhibits cancer cell growth in vitro and induces apoptosis: an evaluation by the bioactivity guided approach, J. Ethnopharmacol., 2008, 118, 14–20 CrossRef PubMed.
- X. Minjie, W. Wei, Z. Zhou and Y. Yongfei, Capillary electrophoresis analysis of hydrogen peroxide induced apoptosis in PC12 cells, J. Pharm. Biomed. Anal., 2005, 39, 853–860 CrossRef PubMed.
- G. Rimon, C. E. Bazenet, K. L. Philpott and L. L. Rubin, Increased surface phosphatidylserine is an early marker of neuronal apoptosis, J. Neurosci. Res., 2015, 48, 563–570 CrossRef.
- L. Zhou, Y. Bai, Y. Li, X. Liu, T. Tan, S. Meng, W. He, X. Wu and Z. Dong, Overexpression of MCPH1 inhibits uncontrolled cell growth by promoting cell apoptosis and arresting the cell cycle in S and G2/M phase in lung cancer cells, Oncol. Lett., 2016, 11, 365–372 CrossRef CAS PubMed.
- E. E. Arias and J. C. Walter, Strength in numbers: preventing rereplication via multiple mechanisms in eukaryotic cells, Genes Dev., 2007, 21, 497–518 CrossRef CAS.
- M. She, J. Li, K. Guo, W. Lin, X. Du and X. Niu, Requirement of reactive oxygen species generation in apoptosis of leukemia cells induced by 2-methoxyestradiol, Acta Pharmacol. Sin., 2007, 28, 1037–1044 CrossRef CAS PubMed.
- D. M. Small, J. S. Coombes, N. Bennett, D. W. Johnson and G. C. Gobe, Oxidative stress, anti-oxidant therapies and chronic kidney disease, Nephrology, 2012, 17, 311–321 CrossRef CAS PubMed.
- G. M. Matthews, A. Newbold and R. W. Johnstone, Intrinsic and extrinsic apoptotic pathway signaling as determinants of histone deacetylase inhibitor antitumor activity, Adv. Cancer Res., 2012, 116, 165–197 CrossRef CAS PubMed.
- K. Kadohara, M. Nagumo, S. Asami, Y. Tsukumo, H. Sugimoto, M. Igarashi, K. Nagai and T. Kataoka, Caspase-8 mediates mitochondrial release of pro-apoptotic proteins in a manner independent of its proteolytic activity in apoptosis induced by the protein synthesis inhibitor acetoxycycloheximide in human leukemia Jurkat cells, J. Biol. Chem., 2009, 284, 5478–5487 CrossRef CAS PubMed.
- A. Luzio, S. M. Monteiro, A. A. Fontaínhas-Fernandes, O. Pinto-Carnide, M. Matos and A. M. Coimbra, Copper induced upregulation of apoptosis related genes in zebrafish (Danio rerio) gill, Aquat. Toxicol., 2013, 128–129, 183–189 CrossRef CAS PubMed.
- M. P. Crespoortiz and M. Q. Wei, Antitumor activity of artemisinin and its derivatives: from a well-known antimalarial agent to a potential anticancer drug, J. Biomed. Biotechnol., 2011, 2012, 247597 Search PubMed.
- G. M. Cohen, Caspases: the executioners of apoptosis, Biochem. J., 1997, 1–16 CrossRef CAS.
- J. Liu, C. Zhang, W. Hu and Z. Feng, Tumor suppressor p53 and its mutants in cancer metabolism, Cancer Lett., 2015, 356, 197–203 CrossRef CAS PubMed.
- C. Q. Hu and Y. Z. Hu, Small molecule inhibitors of the p53-MDM2, Curr. Med. Chem., 2008, 15, 1720–1730 CrossRef CAS.
- V. Calabrã, G. Mansueto, T. Parisi, M. Vivo, R. A. Calogero and M. G. La, The human MDM2 oncoprotein increases the transcriptional activity and the protein level of the p53 homolog p63, J. Biol. Chem., 2002, 277, 2674–2681 CrossRef PubMed.
- A. Enstone, M. Greaney, M. Povsic, R. Wyn, J. R. Penrod and Y. Yuan, The Economic Burden of Small Cell Lung Cancer: A Systematic Review of the Literature, Pharmacoecon. Open, 2017, 1–15 Search PubMed.
- Z. Jing, J. S. Zhang, B. Yang, G. P. Lv and S. P. Li, Free radical scavenging activity and characterization of sesquiterpenoids in four species of Curcuma using a TLC bioautography assay and GC-MS analysis, Molecules, 2010, 15, 7547–7557 CrossRef PubMed.
- H. Zhao, Y. Li, Y. Wang, J. Zhang, X. Ouyang, R. Peng and J. Yang, Antitumor and immunostimulatory activity of a polysaccharide-protein complex from Scolopendra subspinipes mutilans L. Koch in tumor-bearing mice, Food Chem. Toxicol., 2012, 50, 2648–2655 CrossRef CAS PubMed.
- W. G. Yoo, J. H. Lee, Y. Shin, J. Y. Shim, M. Jung, B. C. Kang, J. Oh, J. Seong, H. K. Lee and S. K. Hong, Antimicrobial peptides in the centipede Scolopendra subspinipes mutilans, Funct. Integr. Genomics, 2014, 14, 275–283 CrossRef CAS PubMed.
- T. Weber, H. Dalen, L. Andera, A. Nègre-Salvayre, N. Augé and M. Sticha, et al., Mitochondria play a central role in apoptosis induced by alpha-tocopheryl succinate, an agent with antineoplastic activity: comparison with receptor-mediated pro-apoptotic signaling, Biochemistry, 2003, 42, 4277–4291 CrossRef CAS PubMed.
- H. Düssmann, M. Rehm and J. H. Prehn, Outer mitochondrial membrane permeabilization during apoptosis triggers caspase-independent mitochondrial and caspase-dependent plasma membrane potential depolarization: a single-cell analysis, J. Cell Sci., 2003, 116, 525–536 CrossRef.
- D. G. Breckenridge and D. Xue, Regulation of mitochondrial membrane permeabilization by BCL-2 family proteins and caspases, Curr. Opin. Cell Biol., 2004, 16, 647–652 CrossRef CAS PubMed.
|
This journal is © The Royal Society of Chemistry 2019 |
Click here to see how this site uses Cookies. View our privacy policy here.