DOI:
10.1039/C8RA10263E
(Paper)
RSC Adv., 2019,
9, 4370-4379
Effect of alternan versus chitosan on the biological properties of human mesenchymal stem cells
Received
14th December 2018
, Accepted 23rd January 2019
First published on 4th February 2019
Abstract
Alternan, an α-1,3- and α-1,6-linked glucan, is a polysaccharide that is produced by bacteria. Although the structure of alternan used in this study, an α-1,3- and α-1,6-linked glucan (hereafter referred to as alternan), has been comprehensively characterized, its function on cell biology, especially relative to cell growth and differentiation, has not been fully elucidated. In this study, we set forth to compare the effect of alternan versus chitosan on the biological properties of human mesenchymal stem cells (MSCs). The effect of chitosan on MSC differentiation has already been well characterized. The treated cells were determined for cell proliferation and differentiation capacity compared to untreated cells. The result showed that treatment by alternan or chitosan increased cell proliferation, as demonstrated by increased cell number and scratched regions that were fully restored in less time than it took to fully restore controls. Further investigation found that alternan and chitosan activates the toll-like receptor (TLR) pathway suggesting that these cells may be prone to differentiation. In agreement with this result, an increase in deposited calcium was observed in alternan- or chitosan-treated cells after osteogenic differentiation induction. However, adipogenic differentiation was significantly inhibited in the presence of chitosan, but no change was observed in alternan treatment. Taken together, these results demonstrate biological effects of alternan on human MSCs. Moreover, these novel roles of alternan may have important beneficial medical applications and may provide a basis from which stem cell therapies can be developed in the future.
1. Introduction
Carbohydrate-based materials are widely used in many industries, including food, cosmetic, materials, and pharmacy. Due to their biocompatibility and biodegradability, carbohydrates have also been used in several medical applications, including carbohydrate-based scaffolding,1 drug or cell delivery,2–5 vaccine adjuvants,6 and wound dressing products.7,8 Regarding their role in cell biology, in addition to carbohydrates serving as a source of energy and a component of cell structure, carbohydrates also play a role in host immune response by increasing response against pathogens and foreign cells.9–15 The biological activities of carbohydrates are largely dependent on their structure, including factors like branching, linkage type, and molecular weight.10
Chitosan is a well-known bioactive long-chain polysaccharide carbohydrate that is most commonly found in crab and shrimp shells. Chitosan is a bioactive carbohydrate whose effect on MSC differentiation has already been well characterized.16–18 Due to its antifungal and bactericidal properties, chitosan is routinely used in medical materials, such as wound dressing products.8,19–21 However, the use of chitosan in cell biology research has been limited by the fact that chitosan cannot be dissolved in culture medium, which usually has a neutral or mild alkaline pH.
It is known that some bacteria and fungi can produce exopolysaccharides (EPSs), which are bioactive biopolymers. Glucan is an EPS that is composed of glucose that is covalently linked by α- or β-glycosidic bonds. The structure of glucan determines its chemical and physical properties, such as solubility, viscosity, and porosity, as well as its biological activities. Medical applications of β-glucan have been based on its anticancer, antimicrobial, and immunomodulating activities.9 Concerning cell proliferation, Przekora and Ginaska (2014) reported that addition of β-glucan to biocomposite material enhanced the efficiency of osteoblastic differentiation.22
We recently reported the synthesis of alternan, which is an α-glucan that has alternating α-1,6 and α-1,3 glycosidic linkages, from sucrose using alternansucrase (EC 2.4.1.140) from Leuconostoc citreum ABK-01, which was derived from a Thai dessert called ‘Khao Tom Mud’.23 Briefly, Khao Tom Mud is a mixture of sticky rice, banana, and coconut milk that is wrapped and steamed in banana leaf. That study revealed that alternan has physical properties different from those of other polysaccharides. For example, the physical appearance of alternan varies between nanoparticle and gel, depending on its concentration. Moreover, it can form a transparent film after dehydration, which is a property that is rarely found in other glucans.23
MSCs are considered an attractive cell source for stem cell therapy given their ability to differentiate into a wide variety of cell types, including osteocytes, adipocytes, and chondrocytes, via specific culture conditions. It has been shown that carbohydrate molecules can modulate MSC growth and differentiation mechanisms. For example, chitin and chitosan hexamer promote osteogenic differentiation in MSCs by enhancing the expression of YKL-40 and TLR3, and by inducing secretion of IL-6 and IL-8 cytokines.24 Recently, chitosan was shown to inhibit adipogenesis in 3T3-L1 cell line,25 and in orbital fibroblasts in Graves ophthalmopathy.26 However and to our knowledge, the biological effects of alternan on animal or human MSCs have never been demonstrated or reported.
Accordingly, the aim of this study was to compare the effect of alternan versus chitosan on the biological properties of human MSCs. The findings of this study revealed that alternan has the ability to regulate the biological properties of MSCs, similar to chitosan. However – in contrast to chitosan, alternan did not inhibit adipogenic differentiation. Taken together, these results demonstrate biological effects of alternan on human MSCs, and this result could be used as a theoretical reference to alternan-type glucan for future medical applications.
2. Materials and methods
2.1 Alternan preparation
Alternan was produced from sucrose using alternansucrase derived from Le. citreum ABK-01 (GenBank accession number KM083061.2).23 Briefly, one unit per milliliter of alternansucrase was incubated with 20% (w/v) sucrose in 50 mM citrate buffer (pH 5.0) at 37 °C overnight. The polymer was harvested by acetone precipitation. The derived white polymer was dialyzed against deionized water, and then lyophilized. To produce soluble alternan, 20% (w/v) of the alternan volume was partially hydrolyzed by ultrasonication (Sonics® Vibra-Cell™ ultrasonic liquid processor; Sonics and Materials, Inc., Newton, CT, USA) at 50% power output on ice for 15 minutes. The sonicated alternan was lyophilized and maintained at −20 °C until use.
2.2 Chitosan preparation
Shrimp chitosan was hydrolyzed with Bacillus licheniformis SK-1 chitinolytic enzymes (chitinase and chitosanase) for 4 hours in acetate buffer (pH 4.5) at 45 °C. The hydrolyzed product was deprotonated and precipitated in a single step by addition of sodium hydroxide (NaOH) to a final concentration of 1 N. The precipitant was washed with deionized water to achieve a neutral pH. Chitosan precipitant was then washed with ethanol and acetone, consecutively. Finally, the derived chitosan was dried at room temperature.21
2.3 Isolation and culture of human chorionic mesenchymal stem cells (MSCs)
This study was approved by the ethics committee of the Siriraj Institutional Review Board (SIRB) (COA no. Si101/2015), Faculty of Medicine Siriraj Hospital, Mahidol University, Bangkok, Thailand. The protocol for this study complied with all statements and principles set forth in the Declaration of Helsinki and all of its subsequent amendments, the Belmont Report, the CIOMS guidelines, and the ICH-GCP guidelines. Human chorionic tissue (hCr) was obtained from healthy newborns after receiving written informed consent to do so from their mothers. The hCr was cut into small pieces and incubated with 0.25% (w/v) trypsin–EDTA (GIBCO™; Invitrogen Corporation, Carlsbad, CA, USA) for 30 min at 37 °C. Cells were collected and washed with phosphate buffered saline (PBS) before being resuspended with culture medium and plated in culture vessels (Corning Incorporated, Corning, NY, USA). Cultures were maintained at 37 °C in a humidified atmosphere containing 5% CO2. The culture medium was replaced every other day.
2.4 Cell viability determination
Human chorion-derived mesenchymal stem cells (hCr-MSCs) (8000 cells per well) were seeded in 96-well plates and cultured in complete Dulbecco's Modified Eagle Medium (DMEM) overnight. Cells were then treated with different concentrations of alternan or chitosan (0, 0.5, 5, 50, or 500 μg ml−1) for 24 hours. Cell viability was determined by 3-[4,5-dimethylthiazol-2-yl]-2,5 diphenyl tetrazolium bromide (MTT) assay. Cells were then incubated with MTT reagent at 37 °C for 4 hours, followed by the addition of dimethyl sulfoxide (DMSO). Absorbance was measured at 570 nm using a microplate reader.
2.5 Cell proliferation assay
MSCs (10
000 cells per well) were seeded in 12-well plates and cultured in complete DMEM supplemented with different concentrations of alternan or chitosan (0, 0.5, 5, 50, or 500 μg ml−1). At day 1, 4, and 8 of culture, cells were trypsinized and resuspended in tryphan blue. The number of viable and dead cells were differentiated by trypan blue exclusion and counted by Corning Cell Counter (Corning) using CytoSMART™ software (CytoSMART Technologies, Eindhoven, The Netherlands&ZeroWidthSpace).
2.6 Cell migration
Cell migration was analyzed by scratch wound migration assay.27 MSCs were plated on 12-well plates and cultured in complete DMEM until the cells completely covered the wells. To create the ‘scratch’ region, cells were scratched in a straight line using a sterile P1000 pipette tip. Debris was removed by washing once with 1 ml of DMEM. New DMEM was then added that contained different concentrations of alternan or chitosan. Scratch distance was measured at days 0, 1, and 2 under inverted microscope using NIS-Elements software (Nikon Instruments, Inc., Melville, NY, USA).
2.7 Quantitative real-time polymerase chain reaction (PCR)
Total mRNA from treated and untreated MSCs was extracted using TRIZOL reagent, after which 1 μg of RNA was reverse-transcribed to cDNA using the High-Capacity cDNA Reverse Transcription Kit (Applied Biosystems, Inc., Foster City, CA, USA). Quantitative real-time PCR was performed using Luna® Universal qPCR Master Mix (New England Biolabs, Inc., Ipswich, MA, USA) in a final volume of 10 μl. The sequence of the primers used for qPCR is listed in Table 1. Real-time PCR assays were performed using a CFX384 Touch™ Real-Time PCR Detection System (Bio-Rad Laboratories, Inc., Hercules, CA, USA).
Table 1 Primer sequences used for quantitative real-time PCR
Human TLR |
Primers |
TLR2
|
5′-TGTGAACCTCCAGGCTCTG-3′ |
5′-GTCCATATTTCCCACTCTCAGG-3′ |
TLR3
|
5′-AGCCGCCAACTTCACAAG-3′ |
5′-AGCTCTTGGAGATTTTCCAGC-3′ |
TLR4
|
5′-ACAGAAGCTGGTGGCTGTG-3′ |
5′-TCTTTAAATGCACCTGGTTGG-3′ |
2.8 Osteogenic differentiation
MSCs were plated into 35 mm tissue culture dishes and cultured in complete DMEM until 90% confluence was achieved (approximately 5 × 104 cells). NH OsteoDiff® Medium (Miltenyi Biotec, Bergisch Gladbach, Germany) supplemented with variousconcentrations of alternan or chitosan was then added to induce osteogenic differentiation. After 3 weeks of culture, cells were stained with 40 mM Alizalin Red S (Sigma-Aldrich Corporation, St. Louis, MO, USA) for 20 min at room temperature to determine the number of MSC-derived osteocytes in culture. Alizalin Red S staining was quantified by measuring the area of coloration using ImageJ software.28
2.9 Adipogenic differentiation
MSCs were seeded in 6-well plates and cultured in complete DMEM until 90% confluence was achieved. Adipogenic Differentiation Medium (NH AdipoDiff® Medium; Miltenyi Biotec) supplemented with various concentrations of alternan or chitosan was added. The medium was replaced every 2–3 days. After 3 weeks, adipogenesis was determined by Oil Red O staining according to the manufacturer's instructions (Sigma-Aldrich Corporation, St. Louis, MO, USA). Quantitative assessment was performed by measuring the amount of Oil Red O extracted from cells using a spectrophotometer at 510 nm.29
2.10 Statistical analysis
All results are presented as mean ± standard error of mean (SEM). Statistical differences were tested by one-way analysis of variance (ANOVA) using SPSS Statistics version 22.0 (SPSS, Inc., Chicago, IL, USA). A p-value less than 0.05 was regarded as being statistically significant.
3. Results
3.1 Production of soluble alternans
Alternan was prepared according to the protocol described by Wangpaiboon, et al., 2018.23 Briefly, alternans were synthesized from sucrose using recombinant alternansucrase from Le. citreum ABK-01 that was derived from a Thai dessert that is called ‘Kao tom mud’. A transparent biofilm can be observed around the bacterial colonies, which indicates that this bacterial strain produces an enzyme with transferase activity (Fig. 1A). Alternansucrase was cloned and expressed by Escherichia coli under isopropyl β-D-1-thiogalactopyranoside (IPTG) induction. The yield of derived alternan was approximately 0.15 g g−1 sucrose.
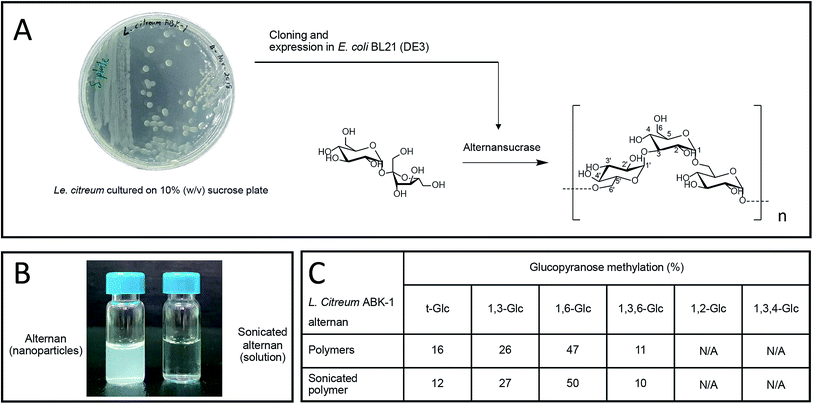 |
| Fig. 1 Alternan isolation and preparation. (A) Synthesis of alternan using recombinant alternansucrase from Leuconostoc citreum ABK-01. (B) Solubility of alternan in nanoparticle and solution formats. (C) Mole percentage of methylated glucoses in alternan and sonicated alternan hydrolysate. | |
Derived alternans are insoluble polymers that usually form colloidal nanoparticles in aqueous solution. However, the solubility of alternan can be improved by ultrasonication. This technique produces high-energy ultrasonic waves stop non-covalent and covalent interactions within molecules (Fig. 1B). In the case of polymers, ultrasonication randomly hydrolyzes the covalent linkages of polymers, which results in many smaller fragments with higher solubility. The structure of sonicated alternan, as evaluated by linkage analysis,23 was slightly changed compared to that of the pre-sonicated alternan (Fig. 1C). This resulting soluble form of alternan facilitated its use in subsequent experiments.
3.2 Effects of soluble alternan and chitosan on MSC viability and proliferation
In order to study the effect of soluble alternan and chitosan on MSC functions, we first investigated the contribution of these saccharides to MSC viability. MSCs were exposed to different concentrations of alternan and chitosan (0, 0.5, 5, 50 and 500 μg ml−1) for 24 hours before MTT assay. As shown in Fig. 2A, the viability of MSCs exposed to alternan gradually decreased as the alternan concentration increased. At 500 μg ml−1 alternan, the viability of MSCs was significantly decreased to 58% compared to control. In contrast, the viability of MSCs exposed to chitosan was constant for concentrations of chitosan up to 50 μg ml−1 (Fig. 2B). However, cell viability was dramatically decreased when exposed to 500 μg ml−1 chitosan. This result suggests chitosan to be more toxic to MSCs than alternan at the 500 μg ml−1 concentration. From these results, a 5 μg ml−1 concentration of both alternan and chitosan was selected to study the effect these sugars on the biological properties of human MSCs. As shown in Fig. 1A, even though the 500 μg ml−1 dose of alternan did not show significant difference from the 5 μg ml−1 dose relative to cell viability, it is possible that the 500 μg ml−1 dose could effectuate different proliferation and differentiation capacity effects on MSCs. We, therefore, include 500 μg ml−1 of alternan in further experiments.
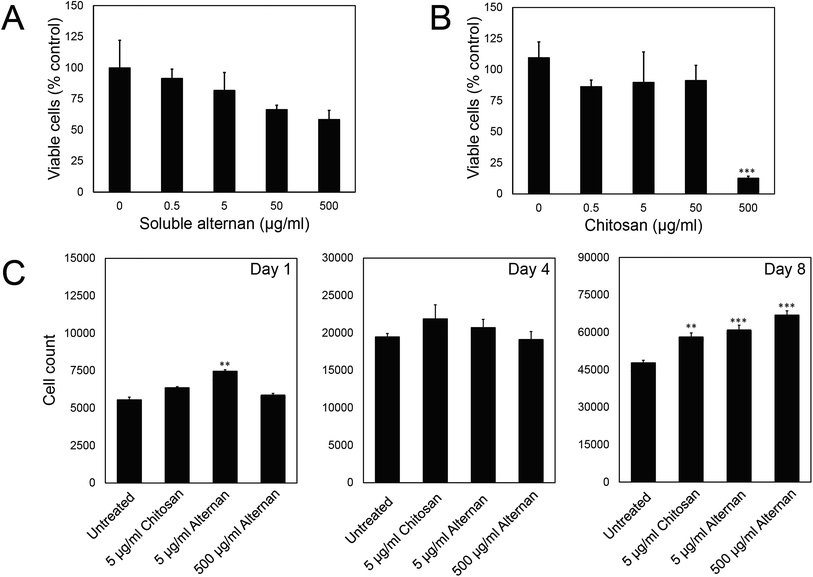 |
| Fig. 2 Effects of alternan and chitosan on MSC viability and proliferation. The viability of MSCs treated with different concentrations of (A) soluble alternan and (B) chitosan was measure by MTT assay. (C) Time course analysis for cell proliferation property after MSCs were treated with selected concentrations of alternan and chitosan for 1, 4, and 8 days. Each data point is presented as mean ± SEM. Asterisk (*) indicates significant difference between the total cell value of the treated group compared with that of the untreated group (*p < 0.05, **p < 0.01, and ***p < 0.001). | |
To determine the effect of alternan and chitosan on cell proliferation, different concentrations of alternan and chitosan were supplemented into the culture media. Treated cells were collected on days 1, 4, and 8 of treatment to determine the total number of cells (Fig. 1C). We found the total cell count to be significantly higher in the treatment groups than in the untreated control groups, especially on day 8. However, no significant difference was observed between the chitosan- and alternan-treated groups for cell count. This result indicates that treatment with alternan promotes the same amount of MSC proliferation as treatment with chitosan. There was also no significant difference observed for MSC proliferation between low- and high-dose alternan.
3.3 Soluble alternan promotes migration of MSCs
The migration of MSCs plays a key role in medical applications, such as tumor-targeted drug delivery. As described earlier, alternan and chitosan were both found to increase cell proliferation of MSCs. We, therefore, decided to investigate whether chitosan and alternan could also induce MSCs migration. In this study, MSCs migration was determined by scratch wound healing assay. The results of this investigation revealed that MSCs treated with soluble alternan or chitosan completely restored the scratched region within 2 days; whereas, empty area could still be observed in the scratched regions of plates cultured with untreated MSCs. This finding indicates that both alternan and chitosan enhanced migration of MSCs (Fig. 3B). Taken together, these findings suggest alternan and chitosan as having the potential to enhance the therapeutic effect of MSCs.
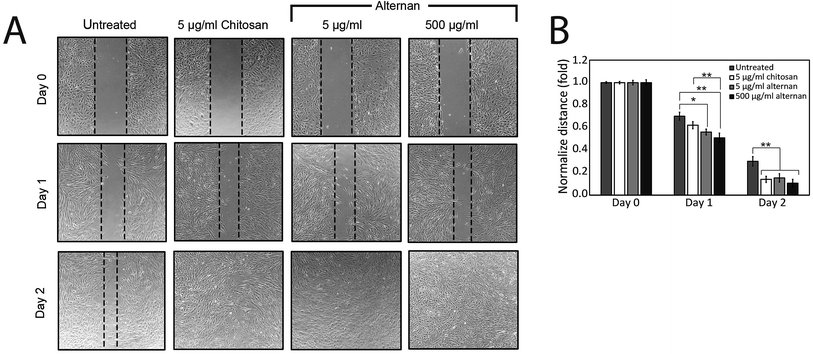 |
| Fig. 3 Effect of soluble alternan and chitosan on MSC migration. (A) Migration analysis was performed by scratch wound healing assay. (B) The distance between the edges of the scratched region measured by NIS-Elements software. Each data point is presented as mean ± SEM (*p < 0.05 vs. untreated group, **p < 0.01 vs. untreated group, and ***p < 0.001). | |
3.4 Soluble alternan stimulates toll-like receptor (TLR) expression in MSCs
Since both soluble alternan and chitosan can promote proliferation and migration of MSCs, we set forth to investigate and determine which cell proliferation controlling pathway was affected. It was shown that carbohydrate molecules, including chitosan, regulate cell proliferation and differentiation via toll-like receptors (TLRs).24 Therefore, expressions of TLR2, TLR3, and TLR4 in MSCs treated with soluble alternan or chitosan were investigated. As shown in Fig. 4A–C, soluble alternan enhanced the expressions of TLR2, TLR3, and TLR4; whereas, chitosan enhanced the expressions of only TLR2 and TLR4. These results suggest that alternan and chitosan activates cell proliferation via the TLR pathway, and that these cells may be prone to differentiation due to the upregulation of TLRs.
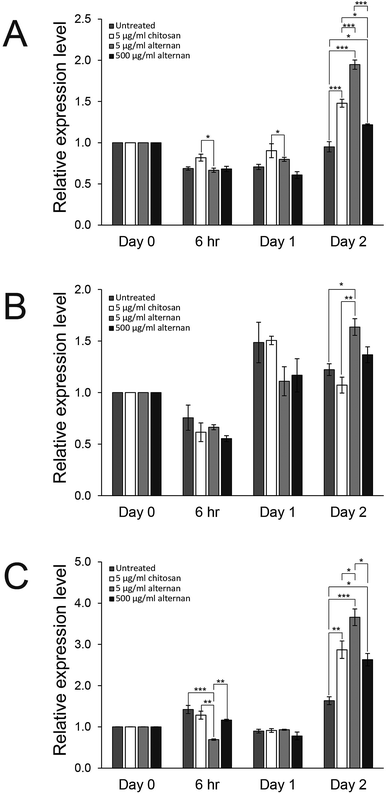 |
| Fig. 4 Expression profile of (A) TLR2, (B) TLR3, and (C) TLR4 of MSCs untreated and treated with chitosan (5 μg ml−1) or soluble alternan (5 and 500 μg ml−1). Each data point is presented as mean ± SEM (*p < 0.05 vs. untreated group, **p < 0.01 vs. untreated group, and ***p < 0.001). | |
3.5 Soluble alternan does not inhibit osteogenic and adipogenic differentiation of MSCs
TLR signaling has been shown to play a role in regulating differentiation of several cell types, including human neural stem cells,30 and immunity-related cells like T-cells, B-cells,31,32 and macrophages.33 In MSCs, Pevsner-Fischer and colleagues showed that TLRs and their ligands can serve as a regulator of MSC proliferation and differentiation.34 However, it has never been fully demonstrated whether carbohydrate-triggered TLR signaling could or would affect the maintenance of MSC multipotency.
In this study, MSCs were subjected to osteocyte and adipocyte differentiation media supplemented with alternan or chitosan for 3 weeks. As shown in Fig. 5A, all MSC samples could be differentiated into osteocytes and adipocytes. Quantitative analysis revealed that alternan or chitosan treatment slightly increased osteogenic differentiation and calcium deposition by MSCs (Fig. 5B). However, adipogenic differentiation was dramatically inhibited in the presence of chitosan, but no change was observed in the presence of alternan. This finding indicates that alternan does not inhibit the osteogenic and adipogenic differentiation of MSCs.
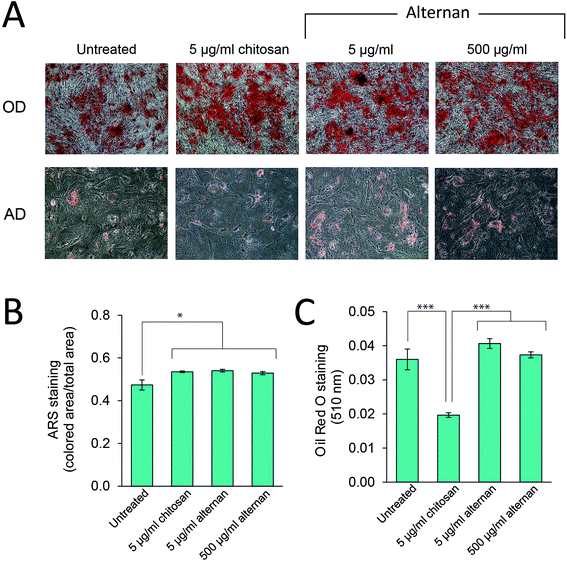 |
| Fig. 5 Effect of soluble alternan on osteogenic (OD) and adipogenic (AD) differentiation. (A) Osteogenic differentiation of MSCs was detected by Alizarin Red S (ARS) staining, while adipogenic differentiation was detected by Oil Red O staining. (B) ARS staining was quantified by measuring the area of coloration using ImageJ software. (C) Oil Red O staining was quantified by spectrophotometer at 510 nm. Each data point is presented as mean ± SEM (*p < 0.05 vs. untreated group, **p < 0.01 vs. untreated group, and ***p < 0.001). | |
4. Discussion
Alternan is an exopolysaccharide that can be found in some microorganism species, including Leuconostoc mesenteroides35 and Leuconostoc citreum.23,36 The physical property of alternan was recently reported,23 but whether alternan plays a role in the biological properties of mammalian cells has never been elucidated. In this study, the function of alternan on mammalian cells was studied using human MSCs as a model. Our results showed that alternan accelerates MSC proliferation and migration. Moreover, alternan treatment was found to enhance differentiation of MSCs to both osteocytes and adipocytes. Cell proliferation and migration were both found to be similar when compared between alternan and chitosan. Similar to what has been reported in other adipocyte cell lines, we found that adding chitosan into the differentiation medium significantly inhibited adipogenic differentiation of human MSCs.
Previous studies showed that exopolysaccharides derived from microorganisms play a role in modulating the biological activities of several cell types, including mutan, which is a mixed α-1,3 and α-1,6 glucan derived from dental plaque that can activate osteoclast differentiation and that can enhance the expression of proinflammatory cytokines in RAW264.7 cells;11 dextran, which is an α-1,6 glucan that can modulate cytokine expression via TLR4 signaling, and that might play a crucial role in immune response;10 pullulan, which is an α-1,6-linked maltrotiose unit that induces the expression of proinflammatory cytokines in human pDC-like CAL-1 cell line;37 and, functional β-glucan, which is a glucan that contains β-1,3 in its backbone, that has anticancer activity,12 and that can promote the proliferation and differentiation of C2C12 myoblasts.38 In this study, we showed that the exopolysaccharide alternan, α-1,3- and α-1,6-linked glucan, promotes proliferation, migration, and differentiation of human MSCs. Taken together, these results confirm the role of exopolysaccharides derived from microorganisms in cell biology and reaffirm the fact that different structure of glucan could result in different biological function depending on the cellular context (Table 2).
Table 2 Selected example of glucan from different sources grouped according to structure, type and biological activity
Glucan name/structure |
Source |
Animal/cell type |
Biological activity |
Reference |
Alternan/(α-1,3)(α-1,6)-D-glucan |
Synthesis |
Human mesenchymal stem cell |
Increase cell proliferation |
This work |
Increase cell migration |
Increase the expression of TLR2, TLR3 and TLR4 |
Enhance osteogenic differentiation |
β-Glucan/(β-1,3)(β-1,4)-D-glucan |
Barley |
Mouse C2C12 myoblast cell |
Increase cell proliferation |
38
|
Increase cell differentiation |
Decrease the fibrotic process |
38 Mutan/(α-1,3)-D-glucan in main chain with some α-1,6 in side chain |
Streptococcus mutant
|
RAW264.7 cells |
Increase osteoclast differentiation |
11
|
Enhance alveolar bone loss in rat maxillae |
Increase expression of IL-1β, IL-1α, IL-6, and chemokine ligands |
Dextran/((α-1,6)-D-glucan) |
Synthesis |
Primary macrophages from rats |
Increase TNFα, IL-6 and nitric oxide |
10
|
(α-1,6)-D-glucan |
Ipomoea batatas (root) |
KM mice; YAC-1 cells |
Increase proliferation of spleen cells |
14
|
Increase NK cell cytotoxicity |
Increase phagocytic function of MO |
Increase hemolytic activity |
Increase serum IgG |
(α-1,6)-Branched, (α-1,4)-D-glucan |
Tinospora cordifolia
|
Human lymphocytes; human complement kits |
Activate NK cells, T and B cells complement activation Th1 pathway-associated profile |
13
|
Pullulan/α-1,6-linked maltrotiose |
Unknown |
Human pDC-like CAL-1 cell line |
Increase the expressions of type IFN-α, IFN-β1, TNF-α, IL-6 and IL-23 |
15
|
In this study, MSCs treated with alternan or chitosan showed higher osteogenic differentiation than untreated MSC controls. Improvement of osteogenic differentiation in MSCs by chitin or chitosan stimulation was previously reported,24 but it is still unclear regarding how chitosan and alternan induce differentiation. The activation of TLRs, which are the controlling pathways for proliferation and differentiation, was observed in treated cells, and this may suggest that the activation of TLRs by alternan or chitosan induces MSCs to differentiate to osteocytes. This result corresponds with those of previous studies that found that activation of TLR2, TLR3, and TLR4 in MSCs after being induced by specific agonists [poly (I:C) or LPS] led to increased osteogenic differentiation capacity.34,39–41
The present study found adipogenic differentiation of MSCs not to be affected by alternan treatment; however, such differentiation was significantly decreased in the chitosan-treated group. The inhibitory effect of chitosan on adipogenesis was found in 3T3-L1 adipocyte cell line.25 However, to the best of our knowledge, the negative effect of chitosan on adipogenic differentiation of human MSCs has never been reported. Although the upregulation of TLRs is involved in cell differentiation, it was shown that TLR expression does not affect adipogenic differentiation of MSCs.39–42 Therefore, the underlying mechanism of alternan and chitosan on adipogenic differentiation of MSCs requires further elucidation.
5. Conclusion
The results of this study demonstrated that alternan has an ability to promote the proliferation, migration, and differentiation of human MSCs. A similar finding was found in chitosan treatment, except that chitosan inhibited adipogenic differentiation. These biological properties may be enhanced by an increase in TLR expression that is caused by alternan or chitosan treatment. These results clearly demonstrate the novel functions of alternan in human MSCs, and they may help to accelerate the development of novel stem cell therapies.
Funding disclosure
This work was supported by the Thailand Research Fund to CL and SI (RSA-6080089 and RTA 488-0007, respectively), and by a Commission on Higher Education grant (CHE-RES-RG-49 to SI) and Science Achievement Scholarship of Thailand (SAST) to TC.
Conflicts of interest
All authors declare no personal or professional conflicts of interest, and no financial support from the companies that produce and/or distribute the drugs, devices, or materials described in this report.
Acknowledgements
The authors would like to thank the staff at the delivery room, Siriraj Hospital, for facilitating specimen collection and all the mothers for kindly donating the chorionic tissues for this study. We would also like to thank the staff members of the Siriraj Center of Excellence for Stem Cell Research (SiSCR) for their support of this study, Miss Wasamon Korchunjit for technical support.
References
- J. Hu, P. H. Seeberger and J. Yin, Using carbohydrate-based biomaterials as scaffolds to control human stem cell fate, Org. Biomol. Chem., 2016, 14, 8648–8658 RSC.
- C. A. García-González, M. Alnaief and I. Smirnova, Polysaccharide-based aerogels—promising biodegradable carriers for drug delivery systems, Carbohydr. Polym., 2011, 86, 1425–1438 CrossRef.
- V. J. Nelson, M. F. K. Dinnunhan, P. R. Turner, J. M. Faed and J. D. Cabral, A chitosan/dextran-based hydrogel as a delivery vehicle of human bone-marrow derived mesenchymal stem cells, Biomed. Mater., 2017, 12, 035012 CrossRef PubMed.
- H. Peng, H. Xiong, J. Li, M. Xie, Y. Liu, C. Bai and L. Chen, Vanillin cross-linked chitosan microspheres for controlled release of resveratrol, Food Chem., 2010, 121, 23–28 CrossRef CAS.
- H. Peng, H. Xiong, J. Li, L. Chen and Q. Zhao, Methoxy poly(ethylene glycol)-grafted-chitosan based microcapsules: synthesis, characterization and properties as a potential hydrophilic wall material for stabilization and controlled release of algal oil, J. Food Eng., 2010, 101, 113–119 CrossRef CAS.
- S. Moreno-Mendieta, D. Guillén, R. Hernández-Pando, S. Sánchez and R. Rodríguez-Sanoja, Potential of glucans as vaccine adjuvants: a review of the α-glucans case, Carbohydr. Polym., 2017, 165, 103–114 CrossRef CAS PubMed.
- I. Sulaeva, U. Henniges, T. Rosenau and A. Potthast, Bacterial cellulose as a material for wound treatment: properties and modifications. A review, Biotechnol. Adv., 2015, 33, 1547–1571 CrossRef CAS PubMed.
- R. Jayakumar, M. Prabaharan, P. T. Sudheesh Kumar, S. V. Nair and H. Tamura, Biomaterials based on chitin and chitosan in wound dressing applications, Biotechnol. Adv., 2011, 29, 322–337 CrossRef CAS.
- F. Y. Kagimura, M. A. A. da Cunha, A. M. Barbosa, R. F. H. Dekker and C. R. M. Malfatti, Biological activities of derivatized d-glucans: a review, Int. J. Biol. Macromol., 2015, 72, 588–598 CrossRef CAS.
- G.-H. Joe, M. Andoh, A. Shinoki, W. Lang, Y. Kumagai, J. Sadahiro, M. Okuyama, A. Kimura, H. Shimizu, H. Hara and S. Ishizuka, Megalo-type α-1,6-glucosaccharides induce production of tumor necrosis factor α in primary macrophages via toll-like receptor 4 signaling, Biomed. Res., 2016, 37, 179–186 CrossRef CAS.
- H.-J. Kwon, J. M. Kim, K.-I. Han, E.-G. Jung, Y. H. Kim, B. B. Patnaik, M. S. Yoon, S. K. Chung, W. J. Kim and M.-D. Han, Mutan: a mixed linkage α-[(1,3)- and (1,6)]-D-glucan from Streptococcus mutans, that induces osteoclast differentiation and promotes alveolar bone loss, Carbohydr. Polym., 2016, 137, 561–569 CrossRef CAS.
- G. C.-F. Chan, W. K. Chan and D. M.-Y. Sze, The effects of β-glucan on human immune and cancer cells, J. Hematol. Oncol., 2009, 2, 25 CrossRef.
- P. K. Nair, S. Rodriguez, R. Ramachandran, A. Alamo, S. J. Melnick, E. Escalon, P. I. Garcia Jr, S. F. Wnuk and C. Ramachandran, Immune stimulating properties of a novel polysaccharide from the medicinal plant Tinospora cordifolia, Int. Immunopharmacol., 2004, 4, 1645–1659 CrossRef.
- G. Zhao, J. Kan, Z. Li and Z. Chen, Characterization and immunostimulatory activity of an (1?>6)-a-d-glucan from the root of Ipomoea batatas, Int. Immunopharmacol., 2005, 5, 1436–1445 CrossRef CAS PubMed.
- F. Wang, L. Qiao, L. Chen, C. Zhang, Y. Wang, Y. Wang, Y. Liu and N. Zhang, The immunomodulatory activities of pullulan and its derivatives in human pDC-like CAL-1 cell line, Int. J. Biol. Macromol., 2016, 86, 764–771 CrossRef CAS.
- Y. J. Lee, S. C. Lee, S. C. Jee, J. S. Sung and A. A. Kadam, Surface functionalization of halloysite nanotubes with supermagnetic iron oxide, chitosan and 2-D calcium-phosphate nanoflakes for synergistic osteoconduction enhancement of human adipose tissue-derived mesenchymal stem cells, Colloids Surf., B, 2019, 173, 18–26 CrossRef CAS.
- H. Chen, H. Wang, B. Li, B. Feng, X. He, W. Fu, H. Yuan and Z. Xu, Enhanced chondrogenic differentiation of human mesenchymal stems cells on citric acid-modified chitosan hydrogel for tracheal cartilage regeneration applications, RSC Adv., 2018, 8, 16910–16917 RSC.
- S. Zhang, B. Ma, S. Wang, J. Duan, J. Qiu, D. Li, Y. Sang, S. Ge and H. Liu, Mass-production of fluorescent chitosan/graphene oxide hybrid microspheres for in vitro 3D expansion of human umbilical cord mesenchymal stem cells, Chem. Eng. J., 2018, 331, 675–684 CrossRef CAS.
- C. Muanprasat and V. Chatsudthipong, Chitosan oligosaccharide: biological activities and potential therapeutic applications, Pharmacol. Ther., 2017, 170, 80–97 CrossRef CAS.
- R. LogithKumar, A. KeshavNarayan, S. Dhivya, A. Chawla, S. Saravanan and N. Selvamurugan, A review of chitosan and its derivatives in bone tissue engineering, Carbohydr. Polym., 2016, 151, 172–188 CrossRef CAS.
- M. Yousef, R. Pichyangkura, S. Soodvilai, V. Chatsudthipong and C. Muanprasat, Chitosan oligosaccharide as potential therapy of inflammatory bowel disease: therapeutic efficacy and possible mechanisms of action, Pharmacol. Res., 2012, 66, 66–79 CrossRef CAS.
- A. Przekora and G. Ginalska, Addition of 1,3-β-d-glucan to chitosan-based composites enhances osteoblast adhesion, growth, and proliferation, Int. J. Biol. Macromol., 2014, 70, 474–481 CrossRef CAS.
- K. Wangpaiboon, P. Padungros, S. Nakapong, T. Charoenwongpaiboon, M. Rejzek, R. A. Field and R. Pichyangkura, An α-1,6-and α-1,3-linked glucan produced by Leuconostoc citreum ABK-1 alternansucrase with nanoparticle and film-forming properties, Sci. Rep., 2018, 8, 8340 CrossRef.
- R. Lieder, F. Thormodsson, C. H. Ng, J. M. Einarsson, J. Gislason, P. H. Petersen and O. E. Sigurjonsson, Chitosan and chitin hexamers affect expansion and differentiation of mesenchymal stem cells differently, Int. J. Biol. Macromol., 2012, 51, 675–680 CrossRef CAS.
- E. J. Cho, A. Rahman, S. W. Kim, Y. M. Baek, H. J. Hwang, J. Y. Oh, H. S. Hwang, S. H. Lee and J. W. Yun, Chitosan oligosaccharides inhibit adipogenesis in 3T3-L1 adipocytes, J. Microbiol. Biotechnol., 2008, 18, 80–87 CAS.
- H. Xiong, M. Wu, H. Zou, S. Jiang, H. Yi, T. Yi, Q. Wang, D. Liu, Y. Zhou, C. Wei and X. Zhou, Chitosan inhibits inflammation and adipogenesis of orbital fibroblasts in Graves ophthalmopathy, Mol. Vision, 2018, 24, 509–517 CAS.
- C.-C. Liang, A. Y. Park and J.-L. Guan,
In vitro scratch assay: a convenient and inexpensive method for analysis of cell migration in vitro, Nat. Protoc., 2007, 2, 329 CrossRef CAS.
- C. A. Schneider, W. S. Rasband and K. W. Eliceiri, NIH Image to ImageJ: 25 years of image analysis, Nat. Methods, 2012, 9, 671–675 CrossRef CAS.
- N. A. Kraus, F. Ehebauer, B. Zapp, B. Rudolphi, B. J. Kraus and D. Kraus, Quantitative assessment of adipocyte differentiation in cell culture, Adipocyte, 2016, 5, 351–358 CrossRef CAS.
- C. Grasselli, D. Ferrari, C. Zalfa, M. Soncini, G. Mazzoccoli, F. A. Facchini, L. Marongiu, F. Granucci, M. Copetti, A. L. Vescovi, F. Peri and L. De Filippis, Toll-like receptor 4 modulation influences human neural stem cell proliferation and differentiation, Cell Death Dis., 2018, 9, 280 CrossRef.
- B. Jin, T. Sun, X.-H. Yu, Y.-X. Yang and A. E. T. Yeo, The effects of TLR activation on T-cell development and differentiation, Clin. Dev. Immunol., 2012, 2012, 32 Search PubMed.
- E. P. Browne, Regulation of B-cell responses by Toll-like receptors, Immunology, 2012, 136, 370–379 CrossRef CAS.
- C. Gallego, D. Golenbock, M. A. Gomez and N. G. Saravia, Toll-like receptors participate in macrophage activation and intracellular control of Leishmania (Viannia) panamensis, Infect. Immun., 2011, 79, 2871–2879 CrossRef CAS PubMed.
- M. Pevsner-Fischer, V. Morad, M. Cohen-Sfady, L. Rousso-Noori, A. Zanin-Zhorov, S. Cohen, I. R. Cohen and D. Zipori, Toll-like receptors and their ligands control mesenchymal stem cell functions, Blood, 2007, 109, 1422–1432 CrossRef CAS.
- M. A. Argüello-Morales, M. Remaud-Simeon, S. Pizzut, P. Sarçabal, R.-M. Willemot and P. Monsan, Sequence analysis of the gene encoding alternansucrase, a sucrose glucosyltransferase from Leuconostoc mesenteroides NRRL B-1355, FEMS Microbiol. Lett., 2000, 182, 81–85 CrossRef.
- A. Musa, M. Miao, T. Zhang and B. Jiang, Biotransformation of stevioside by Leuconostoc citreum SK24.002 alternansucrase acceptor reaction, Food Chem., 2014, 146, 23–29 CrossRef PubMed.
- F. Wang, L. Qiao, L. Chen, C. Zhang, Y. Wang, Y. Wang, Y. Liu and N. Zhang, The immunomodulatory activities of pullulan and its derivatives in human pDC-like CAL-1 cell line, Int. J. Biol. Macromol., 2016, 86, 764–771 CrossRef CAS.
- Y. Li, Y. Fan, H. Pan, H. Qian, X. Qi, G. Wu, H. Zhang, M. Xu, Z. Rao, L. Wang and H. Ying, Effects of functional β-glucan on proliferation, differentiation, metabolism and its anti-fibrosis properties in muscle cells, Int. J. Biol. Macromol., 2018, 117, 287–293 CrossRef CAS.
- H. Hwa Cho, Y. C. Bae and J. S. Jung, Role of toll-like receptors on human adipose-derived stromal cells, Stem Cells, 2006, 24, 2744–2752 CrossRef.
- E. Lombardo, O. DelaRosa, P. Mancheño-Corvo, R. Menta, C. Ramírez and D. Büscher, Toll-like receptor-mediated signaling in human adipose-derived stem cells: implications for immunogenicity and immunosuppressive potential, Tissue Eng., Part A, 2009, 15, 1579–1589 CrossRef CAS.
- H.-S. Kim, T.-H. Shin, S.-R. Yang, M.-S. Seo, D.-J. Kim, S.-K. Kang, J.-H. Park and K.-S. Kang, Implication of NOD1 and NOD2 for the differentiation of multipotent mesenchymal stem cells derived from human umbilical cord blood, PLoS One, 2010, 5, e15369 CrossRef.
- F. Liotta, R. Angeli, L. Cosmi, L. Filì, C. Manuelli, F. Frosali, B. Mazzinghi, L. Maggi, A. Pasini, V. Lisi, V. Santarlasci, L. Consoloni, M. L. Angelotti, P. Romagnani, P. Parronchi, M. Krampera, E. Maggi, S. Romagnani and F. Annunziato, Toll-like receptors 3 and 4 are expressed by human bone marrow-derived mesenchymal stem cells and can inhibit their T-cell modulatory activity by impairing notch signaling, Stem Cells, 2008, 26, 279–289 CrossRef CAS.
|
This journal is © The Royal Society of Chemistry 2019 |
Click here to see how this site uses Cookies. View our privacy policy here.