DOI:
10.1039/C9RA00090A
(Paper)
RSC Adv., 2019,
9, 8300-8309
Retracted Article: Structural characterization of Momordica charantia L. (Cucurbitaceae) oligopeptides and the detection of their capability in non-small cell lung cancer A549 cells: induction of apoptosis
Received
5th January 2019
, Accepted 23rd February 2019
First published on 12th March 2019
Abstract
Oligopeptides are rarely reported from Chinese herbal medicine because they are often present in very low concentrations in a complex matrix. Twenty-eight oligopeptides were recently identified by high-performance liquid chromatography and quadrupole-time-of-flight-mass spectrometry (HPLC-Q-TOF-MS) from Momordica charantia L. (Cucurbitaceae), and a septapeptide, FHGKGHE (Phe-His-Gly-Lys-Gly-His-Glu), named MCLO-12, showed the best anticancer activity against the non-small cell lung cancer A549 cell line in vitro, with an IC50 value of 21.4 ± 2.21 mM. The anti-proliferative activity assay results showed that MCLO-12 induced apoptosis of A549 cells in a concentration-dependent manner. Treatment of the cells with MCLO-12 (10.7–42.8 mM mL−1) caused strong intracellular reactive oxygen species (ROS) up-regulating activities and activated caspase expression. MCLO-12 also suppressed the Trx system and subsequently activated a number of Trx-dependent pathways, including the ASK1, MAPK-p38 and JNK pathways. Thus, our research provides a good reference point for anti-NSCLC research into oligopeptides.
1 Introduction
Lung cancer is the major cause of cancer-related death globally and non-small cell lung cancer (NSCLC) is the most common type of lung cancer.1 Currently, available treatment strategies for NSCLC, including surgery, radiotherapy, and chemotherapy, remain generally unsuccessful.2 However, NSCLC usually reveals better responsiveness to chemotherapy, for example, gefitinib (ZD1839, Iressa), which is an EGFR tyrosine kinase inhibitor (TKI) that received approval from the FDA in 2003, inhibits EGFR activity and EGFR downstream signaling, resulting in tumor shrinkage, and patients exhibit a good initial response.3 However, the presence of tumor immunity reduces the anti-tumor effect and increases the dosage required for treatment. Furthermore, large doses of anti-tumor drugs can also result in fatal damage to normal cells.4 Hence, the identification of novel targets for more effective anti-NSCLC strategies with minimal toxicity is urgent.
Natural proteins have been recognized for centuries to have functional properties. The biological activity of a protein in physiological utilization is associated with its amino acid content.5,6 Previous studies have successfully identified that medicinal plants provide a significant diversity of proteins and peptides, which can be exploited as potential anti-cancer agents.7
Momordica charantia is a widely consumed vegetable with a bitter taste. Furthermore, Momordica charantia L. (Cucurbitaceae) is utilized in traditional Chinese medicine to treat a variety of diseases, such as cancer, inflammation, and diabetes.8,9 Momordica charantia L. (Cucurbitaceae) extract has been demonstrated to play a role in oncogenesis, and accumulated evidence has evaluated its anticancer effects, such as anti-proliferation and anti-migration activity. However, to date, no work has focused on Momordica charantia L. (Cucurbitaceae) oligopeptides (MCLOs) for cancer therapy. In this study, a serious of MCLOs were isolated and their amino acid sequences were identified, their anticancer activities against NSCLC were evaluated, and the related mechanisms were also investigated.
2 Materials and methods
2.1 Materials and cell culture
The Momordica charantia L. (Cucurbitaceae) was purchased from Ertiantang pharmacy (Guangzhou, China) and identified by Professor Zhou (Jinan University, Guangzhou, China). All of the assay kits were purchased from Beyotime (Shanghai, China). All of the chemicals were analytical reagents and were purchased from Sigma-Aldrich (St. Louis, USA). All of the antibodies were purchased from Cell Signaling Technology (Santa Cruz, USA). The human normal lung fibroblast cell line CCD19 and human NSCLC cell lines A549, L78, PGCL3, H460 and NCI-H1299 were purchased from the Cell Bank of the Chinese Academy of Sciences (Shanghai, China). The human normal lung fibroblast CCD19 cells were grown in MEM medium, whereas the NSCLC cell lines were grown in RPMI 1640 medium. All culture media were supplemented with 10% FBS with 100 U per mL penicillin and 100 μg mL−1 streptomycin, and the cells were cultured at 37 °C in an atmosphere of 5% CO2.
2.2 Isolation and identification of MCLOs
2.2.1 Preparation of crude protein. All extraction and separation procedures were carried out at 0 °C. Momordica charantia L. (Cucurbitaceae) samples were minced to a homogenate and defatted according to a previously described method.10 The homogenate and iso-propanol were mixed in a ratio of 1
:
5.5 (w/v) and stirred uninterrupted for 6 h at room temperature. The iso-propanol was replaced every 1 h. The supernatant was removed, and the sediment was freeze-dried and stored at −20 °C.The defatted precipitate (20 g) was dissolved (5%, w/v) in 0.2 M phosphate buffer solution (PBS, pH 7.0), and then a KQ-250B ultrasonic cleaner (Shanghai, China) with a straight probe and in continuous pulse mode was used to ultrasonicate the sample for 3 h. After centrifugation (9000 × g, 20 min), the supernatant was collected as total protein and then fractionated by salting-out with increasing concentrations of ammonium sulfate, and the resulting supernatant was freeze-dried and stored at −20 °C for further analysis.
2.2.2 Fractionation by ultrafiltration. The resulting supernatant was fractionated using ultrafiltration with 1 kDa molecular weight (MW) cut off membranes (Millipore, Hangzhou, China) at the lab scale. Two peptide fractions, called MCLO-A (MW < 1 kDa) and MCLO-B (MW > 1 kDa), were collected and freeze-dried.Hydrophobic Chromatography: MCLO-A was dissolved in 1.3 M (NH4)2SO4 prepared with 30 mM PBS (pH 7.5) and loaded onto a Phenyl Sepharose CL-4B hydrophobic chromatography column (3.0 cm × 70 cm), which had previously been equilibrated with the above buffer. A stepwise elution was carried out with decreasing concentrations of (NH4)2SO4 (1.3, 0.65 and 0 M) dissolved in 30 mM phosphate buffer (pH 7.5) at a flow rate of 2.0 mL min−1. Each fraction was collected at a volume of 50 mL and was monitored at 280 nm. Ten fractions were collected and freeze-dried, and then the antiproliferative activity against the NSCLC cell lines was detected. The fraction having the strongest antiproliferative activity was collected and used for anion-exchange chromatography.
2.2.3 Anion-exchange chromatography of MCLO-A-3. The MCLO-A-3 solution (2 mL, 253.0 mg mL−1) was loaded into a DEAE-52 cellulose (Yuanju, Shanghai, China) anion-exchange column (1.5 × 90 cm) pre-equilibrated with deionized water and stepwise eluted with 600 mL distilled water, and 0.1, 0.6, and 1.2 M (NH4)2SO4 solutions at a flow rate of 1.5 mL min−1. Each eluted fraction (40 mL) was collected and detected at 280 nm. Five fractions (A-MCLO-3-1 to A-MCLO-3-5) were collected and freeze-dried, and then the antiproliferative activity against the NSCLC cell lines was detected. The fraction having the strongest antiproliferative activity was collected and used for gel filtration chromatography.
2.2.4 Gel filtration chromatography of MCLO-A-3-3. The MCLO-A-3-3 solution (3 mL, 14.1 mg mL−1) was fractionated on a Sephadex G-25 (Sigma-Aldrich, Shanghai, China) column (2.0 × 140 cm) at a flow rate of 1.0 mL min−1. Each eluate (15 mL) was collected and monitored at 280 nm, and four fractions (MCLO-A-3-3-1 to MCLOA-3-3-4) were collected and freeze-dried, and then their antiproliferative activity against the NSCLC cell lines was determined. The fraction having the strongest antiproliferative activity was collected and used for reversed phase-high performance liquid chromatography (RP-HPLC).
2.2.5 Isolation peptides from MCLOA-3-3-4 by RP-HPLC. MCLOA-3-3-4 was finally separated by RP-HPLC (Agilent 1200 HPLC) on a Zorbax, SB C-18 column (4.6 × 250 mm, 5 μm). The elution solvent system was composed of water-trifluoroacetic acid (solvent A; 100
:
0.1, v/v) and acetonitrile-trifluoroacetic acid (solvent B; 100
:
0.1, v/v). The peptide was separated using a gradient elution from 30% to 80% of solvent B for 60 min at a flow rate of 1.0 mL min−1. The detection wavelength was set at 280 nm and the column temperature was 20 °C. The eluate was assessed at 280 nm, and the peptides were isolated and freeze-dried.
2.2.6 Amino acid sequence analysis and molecular mass determination by HPLC-ESI-MS. Prior to HPLC-ESI-MS analysis, the freeze-dried oligopeptide was rehydrated with 1.0 mL of Milli-Q water. Before being used, the water was boiled for 5 min and then cooled to 4 °C. The rehydrated solution was stored at −20 °C until analysis.HPLC-ESI-MS was carried out on a SCIEX X500R Q-TOF mass spectrometer (Framingham, U.S.A.). The MS conditions were as follows: ESI-MS analysis was performed using a SCIEX X500R Q-TOF mass spectrometer equipped with an ESI source. The mass range was set at m/z 100–1500. The Q-TOF MS data were acquired in positive mode and the conditions of MS analysis were as follows: CAD gas flow-rate, 7 L min−1; drying gas temperature, 550 °C; ion spray voltage, 5500 V; declustering potential, 80 V. Software generated data file: SCIEX OS 1.0.
2.3 Cell proliferation analysis (MTT)
The MCLOs were dissolved in PBS as 200.0 mM stock solutions and diluted in cell culture medium to different concentrations. The cell cytotoxicity was determined by the MTT method published before.11
The CCD19 and NSCLC cells were seeded on 96-well plates at a density of 5 × 103/well and cultured overnight. Then, the cells were treated with different concentrations of the MCLOs for 48 h, and PBS served as the vehicle control. Each dosage was repeated in triplicate, and three independent experiments were performed.
After 48 h of treatment, 10 μL of MTT (10 μL per well) was added and the medium was removed after 4 h of incubation. The absorbance of the converted dye in living cells was measured at a wavelength of 570 nm after 100 μL of PBS was added. IC50 values were determined by the nonlinear multipurpose curve fitting program GraphPad Prism.
2.4 Cell apoptosis assay
A549 cells were plated on a 6-well plate with a cell density of 2 × 105 cells per well and treated with MCLO-12 (0, 10.7, 21.4 and 42.8 mM) for 48 h. After treatment, the cells were harvested and collected by centrifugation and then apoptosis was determined by Annexin V and PI staining according to the manufacturer's protocol and detected with flow cytometric analysis (BD FACS Calibur, Franklin Lakes, CA, USA).12 All of the tests were repeated at least 3 times.
2.5 Cell cycle analysis
A549 cells were plated on a 6-well plate with a density of 2 × 105 cells per well and treated with MCLO-12 (0, 10.7, 21.4 and 42.8 mM) for 48 h. Then, the cells were harvested and fixed in 70% ethanol and stored at −20 °C overnight. Then, the cells were washed with PBS and the cell cycle distribution was detected by PI (propidium iodide) staining and analyzed by flow cytometry (BD FACS Calibur, Franklin Lakes, CA, USA).13 All of the tests were repeated at least 3 times.
2.6 Intracellular ROS assay
DCFH-DA can pass through the cell membrane to produce DCFH by hydrolysis by the intracellular esterase.14 ROS can oxidize DCFH to produce fluorescent DCF. Therefore, the detection of DCF fluorescence can reveal the level of ROS. So, the intracellular ROS level of A549 cells was measured with a Reactive Oxygen Species assay kit. 1 mL of 1 × 106 cells per mL cells were induced with MCLO-12 (0, 10.7, 21.4 and 42.8 mM) at 37 °C for 4 h, and 21.4 mM MCLO-12 for 0, 2, 4, 8, 12, 24 and 48 h, and then, the cells were washed with serum-free medium three times.15 The A549 cells were incubated in 200 μL of serum-free medium containing DCFH-DA (25 μM) for 30 min at room temperature and then washed with serum-free medium three times. The cells were collected and assayed by flow cytometric analysis (BD FACS Calibur, Franklin Lakes, CA, USA).
2.7 Mitochondrial membrane potential assay
The changes in mitochondrial membrane potential of A549 cells induced by MCLO-12 (0, 10.7, 21.4 and 42.8 mM) were detected by JC-1 staining according to the manufacturer's protocol (Beyotime, China). Briefly, 2 mL of 1 × 106/mL cells were treated with MCLO-12 in 6-well plates for 8 h. The cells were washed three times with cold PBS and incubated with 1 μg mL−1 of JC-1 at 37 °C for 30 min without light. The supernatant was removed and washed three times with cold PBS and then assayed by flow cytometric analysis (FACScan, CA).16
2.8 Western blot analysis
A549 cells were plated in 6-well culture dishes and treated with MCLO-12 (0, 10.7, 21.4 and 42.8 mM) in cell culture medium for 48 h. After incubation, the cells were washed with ice-cold PBS, scraped, pelleted and lysed in radioimmunoprecipitation assay (RIPA) buffer. After incubation for 1 h on ice, the cell lysates were centrifuged at 3000g for half an hour at 4 °C.
The lysate protein concentrations were determined by a BCA protein assay kit (Thermo Scientific, USA) and the lysates were adjusted with lysis buffer.17 The proteins were resolved by 15% SDS-PAGE and transferred to an immobilon polyvinylidene difluoride (PVDF) membrane. The blots were blocked with blocking buffer for 10 min at room temperature and then probed with rabbit anti-human antibodies for 1 h at room temperature. Then, the blots were incubated with a peroxidase-conjugated donkey anti-rabbit secondary antibody (1
:
3000 dilution) for 1 h at room temperature. Signals were visualized by enhanced chemiluminescence with Kodak X-OMAT LS film (Eastman Kodak, USA).
2.9 Statistical analysis
Experiments were repeated at least three times and the results are expressed as mean ± SEM. Data were analyzed by Student's t-test and an analysis of variance (ANOVA) test followed by a Tukey post test to determine the significant differences between groups. p < 0.05 was considered to be significant. All statistical analyses were performed with GraphPad-Prism 5 (San Diego, USA).
3 Results
3.1 Isolation and identification of MCLOs
Twenty-eight MCLOs were isolated, and their amino acid sequences were identified by HPLC-ESI-MS analysis. The peptides are usually protonated under ESI-MS/MS conditions, and fragmentations mostly occur at the amide bonds because it is difficult to break the chemical bonds of the side chains at such low energy.18 Therefore, the b and y ions are the main fragment ions when the collision energy is <200 eV. MCLO-12 was analyzed by HPLC-ESI-MS for molecular mass determination and peptide characterization. The molecular mass of the peptide was determined to be 811.3761 Da. The ion fragment m/z 285.1325 was regarded as the y5 ion, while m/z 470.2461 was regarded as the b3 ion and m/z 527.2581 was regarded as the y3 ion. The ion at m/z 793.3729 was the typical fragment [M-NH2 + H]+, and m/z 664.3254 was the b1 ion. On the basis of this, we concluded that the sequence of the peptide was FHGKGHE. The rest of the MCLOs were identified with reference to MCLO-12, and after the analysis by MS/MS spectra processing with the BioTools database, we successfully identified twenty-eight MCLOs and their amino acid sequences are listed in Table 1.
Table 1 Amino acid sequences and cell growth inhibition of MCLOs against different cancer cell linesa
MCLO |
Amino acid sequence |
IC50 (mM) |
A549 |
NCI-H1299 |
L78 |
PGCL3 |
H460 |
IC50 values are shown as mean ± standard error of the mean (SD), from at least three independent experiments. |
MCLO-1 |
FRHDLS |
56.5 ± 3.66 |
92.1 ± 10.2 |
33.1 ± 3.22 |
52.6 ± 4.24 |
92.8 ± 6.92 |
MCLO-2 |
FRHALS |
46.2 ± 7.04 |
76.6 ± 7.82 |
46.2 ± 3.94 |
86.3 ± 5.87 |
72.5 ± 6.12 |
MCLO-3 |
WAGHAYE |
>200 |
>200 |
>200 |
74.9 ± 7.60 |
>200 |
MCLO-4 |
WARYQHG |
66.3 ± 7.17 |
94.2 ± 8.53 |
66.3 ± 5.18 |
>200 |
54.6 ± 4.65 |
MCLO-5 |
HRWQGKH |
>200 |
>200 |
>200 |
>200 |
>200 |
MCLO-6 |
HREWKGH |
40.1 ± 5.77 |
75.1 ± 8.16 |
49.6 ± 3.82 |
66.4 ± 5.17 |
106 ± 8.18 |
MCLO-7 |
HGEHRVY |
51.3 ± 6.02 |
83.6 ± 9.21 |
61.3 ± 6.02 |
93.6 ± 7.21 |
111 ± 9.60 |
MCLO-8 |
WEGHES |
>200 |
>200 |
46.3 ± 4.18 |
>200 |
64.3 ± 3.63 |
MCLO-9 |
SHAYSH |
>200 |
>200 |
64.5 ± 6.19 |
79.7 ± 6.64 |
>200 |
MCLO-10 |
HKYRHD |
46.3 ± 4.37 |
65.2 ± 5.32 |
>200 |
56.2 ± 5.34 |
66.7 ± 6.35 |
MCLO-11 |
FSHRGH |
64.3 ± 7.58 |
89.1 ± 7.50 |
74.8 ± 7.29 |
>200 |
59.6 ± 5.60 |
MCLO-12 |
FHGKGHE |
21.4 ± 2.21 |
33.7 ± 2.72 |
41.0 ± 3.25 |
53.3 ± 6.15 |
103 ± 9.86 |
MCLO-13 |
KYGHEHS |
>200 |
>200 |
>200 |
102 ± 8.83 |
113 ± 9.95 |
MCLO-14 |
FDHGWK |
96.7 ± 9.82 |
>200 |
86.4 ± 9.16 |
>200 |
>200 |
MCLO-15 |
HESGWL |
31.3 ± 2.17 |
56.2 ± 4.23 |
56.3 ± 8.94 |
76.6 ± 7.25 |
56.7 ± 5.34 |
MCLO-16 |
KSHEFG |
36.7 ± 4.08 |
36.2 ± 4.74 |
>200 |
>200 |
>200 |
MCLO-17 |
KYAVHS |
>200 |
55.5 ± 2.41 |
76.8 ± 3.17 |
54.2 ± 4.66 |
56.0 ± 5.15 |
MCLO-18 |
TYKRHS |
56.8 ± 6.60 |
46.1 ± 4.03 |
>200 |
>200 |
89.5 ± 8.86 |
MCLO-19 |
GEYHSHE |
39.2 ± 2.60 |
73.7 ± 5.37 |
89.4 ± 5.80 |
46.1 ± 4.13 |
>200 |
MCLO-20 |
EHGEYF |
53.5 ± 4.10 |
33.5 ± 2.26 |
41.6 ± 3.91 |
113 ± 9.20 |
51.6 ± 4.96 |
MCLO-21 |
EGFHL |
36.1 ± 2.69 |
71.0 ± 4.76 |
>200 |
68.7 ± 5.17 |
104 ± 8.66 |
MCLO-22 |
KHGEL |
61.7 ± 6.01 |
74.2 ± 5.75 |
56.5 ± 5.17 |
74.9 ± 6.67 |
>200 |
MCLO-23 |
EGHGF |
>200 |
65.7 ± 7.37 |
36.2 ± 3.13 |
44.1 ± 3.67 |
36.3 ± 4.16 |
MCLO-24 |
YEEGAH |
53.6 ± 5.51 |
47.5 ± 4.76 |
>200 |
>200 |
39.7 ± 2.84 |
MCLO-25 |
AHEFEL |
26.0 ± 2.07 |
>200 |
39.5 ± 2.60 |
66.3 ± 5.14 |
>200 |
MCLO-26 |
EAHGHSF |
>200 |
41.6 ± 4.31 |
41.1 ± 4.52 |
103 ± 6.27 |
41.2 ± 2.97 |
MCLO-27 |
YSLHLHG |
>200 |
31.5 ± 3.28 |
>200 |
78.1 ± 7.18 |
101 ± 7.63 |
MCLO-28 |
YESHGA |
>200 |
74.4 ± 5.12 |
46.5 ± 5.12 |
54.9 ± 5.63 |
>200 |
3.2 Anti proliferation effects of MCLOs against NSCLC and normal cells
All of the MCLOs were screened for anti-proliferative activities against A549, L78, PGCL3, H460 and NCI-H1299 cells by MTT assay and the results are depicted in Table 1.
As shown in Table 1, most of the MCLOs exhibited anti-proliferative activities, and MCLO-12 (Fig. 1) showed the best anti-proliferation activity against A549 cells with an IC50 value of 21.4 ± 2.21 mM. These results also indicated that MCLO-12 showed a broad range of growth inhibition effects against the tested cell lines. Therefore, further studies on MCLO-12 were carried to check its ability as an anti-tumor agent against A549 cells.
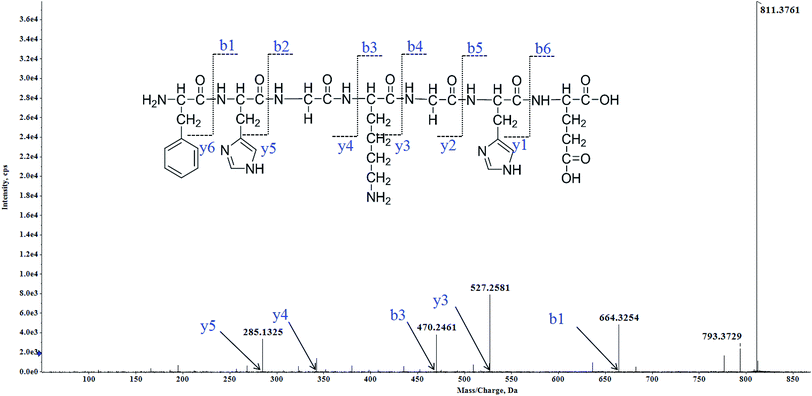 |
| Fig. 1 Structure and MS/MS spectra of MCLO-12 (m/z = 811.3761). | |
We also evaluated the cytotoxicity of MCLO-12 against normal cells after 48 h of incubation with different doses. The IC50 against the human lung fibroblast CDD19 cell line is >200 mM. Therefore, we inferred that MCLO-12 exhibited lower cytotoxicity to human normal lung fibroblast cells. Furthermore, we speculated that MCLO-12 is worthy of further research.
3.3 MCLO-12 induces apoptosis
Apoptosis, the process of programmed cell death, is an important therapy target and apoptotic cells are different from normal cells with certain distinct morphological features, such as cytoplasmic shrinkage, membrane blebbing and chromatin condensation.19,20 As reported, necrosis is a form of traumatic cell death that results from acute cellular injury; in contrast, apoptosis is a highly regulated and controlled process that confers advantages during an organism's lifecycle. Different from necrosis, apoptosis produces cell fragments called apoptotic bodies that phagocytic cells are able to engulf and quickly remove before the contents of the cell can spill out onto the surrounding cells and cause damage to the neighboring cells. It has been reported that scorpion venom can induce apoptosis and has a significant anti-tumor effect in vitro.21,22 In order to investigate the apoptosis-inducing effect of MCLO-12, flow cytometry using propidium iodide (PI) and Annexin-V in A549 cells was performed. After incubation with the MCLO-12 described above for 48 h, the cells were stained with Annexin V/PI, and the percentage of apoptotic cells was measured using a flow cytometer.
An early marker of apoptosis is the exposition of phosphatidylserine on the cell surface, whereas it is normally concentrated in the luminal layer of the cytoplasmic membrane.23 As shown in Fig. 2, after MCLO-12 treatment, the proportion of A549 apoptotic cells was significantly increased compared with the control group.
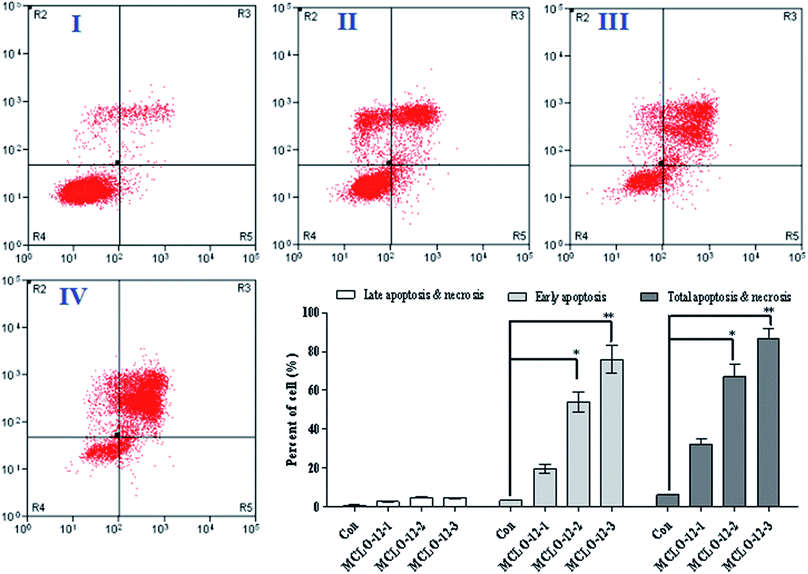 |
| Fig. 2 MCLO-12-induced apoptosis in A549 cells. Representative scatter diagrams. A549 cells were pre-treated without the addition of MCLO-12 as the control (I), with a dose of 10.7 mM MCLO-12 (II), with a dose of 21.4 mM MCLO-12 (III), and with a dose of 42.8 mM MCLO-12 (IV), respectively, for 48 h. Cells were stained with Annexin-V and PI. The apoptosis of A549 cells was detected by flow cytometry. The evaluation of apoptosis was performed via an Annexin V: FITC Apoptosis Detection Kit following the manufacturer's protocol. In each scatter diagram, the abscissa represents the fluorescence intensity of the cells dyed by Annexin V, and the ordinate represents the fluorescence intensity of the cells dyed by PI. The lower left quadrant shows the viable cells, the upper left shows necrotic cells, the lower right shows the early apoptotic cells and the upper right shows late apoptotic cells. | |
As shown in Fig. 2, at the dose of 10.7 mM, MCLO-12 caused 32.3% total apoptosis/necrosis rate, while 21.4 mM MCLO-12 showed better inhibition activity, in which about 67.2% total apoptosis/necrosis rate (with 54.0% for early and 4.81% for late) was found. Interestingly, 42.8 mM MCLO-12 was found to induce 86.8% total apoptosis/necrosis rate (with 75.9% for early and 4.51% for late). The results indicate that MCLO-12 effectively inhibits the growth of A549 cells and a dose-dependent increase was also observed.
3.4 MCLO-12 delays the cell cycle and activates caspases
The main property of tumor cells is uncontrollable proliferation and arresting the cell cycle has become a pivotal therapy target in various remedies. The most important point in cell cycle regulation is the point from G0/G1 phase to S phase.24
As the above results showed that MCLO-12 can induce apoptosis, the effect of MCLO-12 on the cell cycle was also investigated by flow cytometry.
As shown in Fig. 3, treatment with MCLO-12 at 10.7, 21.4 and 42.8 mM lead to the accumulation of 63.2%, 70.3% and 73.1% of A549 cells in G0/G1 phase, compared with 61.3% of cells in G0/G1 phase in the control group; therefore, MCLO-12 clearly arrested A549 cells in the G0/G1 phase. These data suggest that MCLO-12 may induce cell apoptosis by blocking the cell cycle. The number of cells in G0/G1 phase increased and the number in S phase decreased. This suggests that MCLO-12 can induce the apoptosis of A549 cells and block the cells in G0/G1 phase, so the cells can't enter S stage to synthesize DNA, and eventually the proliferation of the tumor cells in vitro is inhibited.
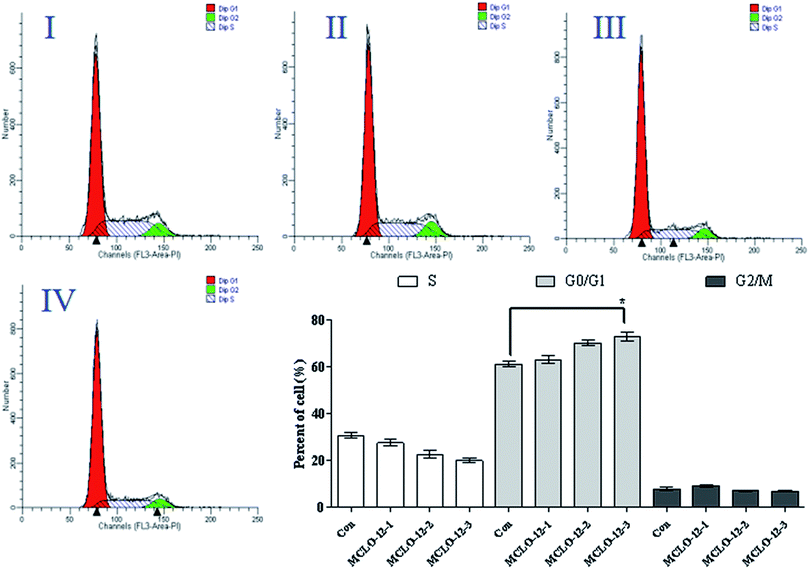 |
| Fig. 3 Cell cycle analysis of A549 cells exposed to MCLO-12. A549 cells were pre-treated without the addition of MCLO-12 as the control (I), with a dose of 10.7 mM MCLO-12 (II), with a dose of 21.4 mM MCLO-12 (III), and with a dose of 42.8 mM MCLO-12 (IV), respectively, for 48 h. The cells were collected, fixed in 70% ethanol, and stained with propidium iodide solution. G0/G1: quiescent state/growth phase; S: initiation of DNA replication; G2/M: biosynthesis/mitosis phases. | |
As we know, caspase 3 is involved in the apoptotic process and caspase 9 is an initiator caspase. PARP, a DNA-repair enzyme, serves as a substrate for caspase 3.25 In order to disclose the mechanism via which MCLO-12 induces cell apoptosis, we also analyzed caspase activation and PARP cleavage.
As shown in Fig. 5A, the levels of caspases 9 and 3 were significantly decreased with MCLO-12 treatment. Additionally, the levels of cleaved caspase 9 and 3 were up-regulated; furthermore, MCLO-12 treatment also led to specific proteolytic cleavage of PARP in A549 cells. These results indicate that MCLO-12 may induce cell apoptosis by regulating caspase 3, caspase 9 and PARP, which are closely involved in the program of cell death.
3.5 MCLO-12 increases the cellular ROS level and induces MMP loss
The overproduction of free radicals, such as reactive oxygen species (ROS), or antioxidant capacity decline will lead to balance disorders and cause ROS accumulation and oxidative stress that can lead to fatal damage to DNA and proteins within the cell to subsequently induce apoptosis.25
To examine the intracellular ROS in A549 cells after MCLO-12 treatment, we checked the ROS level in A549 cells. As shown in Fig. 4A and B, MCLO-12 caused clear ROS accumulation and acted in a dose-and time-dependent manner.
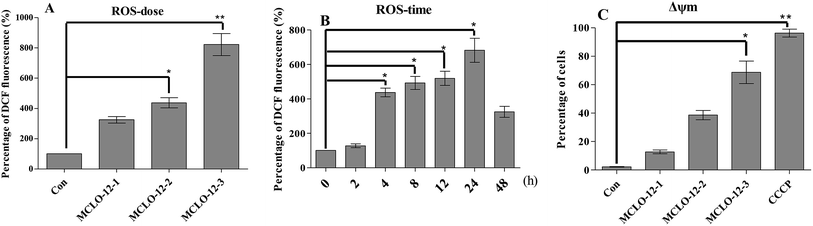 |
| Fig. 4 MCLO-12-induced ROS production and interference with cellular redox homeostasis. (A) Cells were treated with MCLO-12 (0, 10.7, 21.4 and 42.8 mM) at 37 °C for 4 h, stained with DCFH-DA for 20 min, and analyzed for fluorescence by flow cytometry. All data are presented as the mean ± SEM of three independent experiments. *p < 0.05 and **p < 0.01. (B) Cells were treated with 21.4 mM MCLO-12 at 37 °C for 0, 2, 4, 8, 12, 24 and 48 h, stained with DCFH-DA for 20 min, and analyzed for fluorescence by flow cytometry. All data are presented as the mean ± SEM of three independent experiments. *p < 0.05. (C) Flow cytometry analysis of MMP based on JC-1 staining. Cells were treated with MCLO-12 (0, 10.7, 21.4 and 42.8 mM) for 24 h and stained with JC-1. The cells showing a loss of MMP were gated. The cells were exposed to the MMP disrupter carbonyl cyanide 3-chlorophenylhydrazone (CCCP, 10 μM) for 20 min as a positive control. All data are presented as the mean ± SEM of three independent experiments. *p < 0.05 and **p < 0.01. The data shown are representative of three independent experiments. | |
Mitochondrial membrane permeabilization is a critical event in the process leading to physiological or chemotherapy-induced apoptosis (programmed cell death).26 The balance of mitochondrial membrane potential (Δψm) and mitochondrial integrity is significant for the physiological function of cells.27 Previous studies have reported that the collapse of Δψm is correlated to the events of the apoptotic process.28 The apoptotic changes of mitochondria include Δψm loss, transient swelling of the mitochondrial matrix, mechanical rupture of the membrane and/or its nonspecific permeabilization by giant protein permeant pores, and release of soluble intermembrane proteins through the outer membrane.29 When the concentration of ROS and oxygen stress reach a certain level, the mitochondrial membrane permeability will be changed, resulting in the release of apoptosis factors.30 Once the mitochondrial membrane barrier function is lost, several factors, including the loss of redox homeostasis, the metabolic consequences at the bioenergetic level, and the perturbation of ion homeostasis, lead to cell death. Here, JC-1 fluorescent probe is used to detect Δψm.
From Fig. 4C we can observed that A549 cells treated with MCLO-12 showed a Δψm decrease, which meant that MCLO-12 could lead to the collapse of the Δψm. In particular, the Δψm of the cells incubated with 42.8 mM MCLO-12 was almost completely lost. Therefore, we concluded that MCLO-12 may play a role in inducing apoptosis by interfering with the function of mitochondria.
3.6 MCLO-12 suppresses the Trx system and activates the ASK1 and MAPK pathways
The Trx system is an important antioxidant systems for the balance of the intracellular redox state.31 Mechanism studies have shown that Trx/TrxR is often over-expressed in tumor cells.32 The effects of MCLO-12 on Trx and TrxR expression were also examined in A549 cells.
As shown in Fig. 5B, the expression levels of Trx and TrxR were markedly down regulated by MCLO-12 compared with the control group.
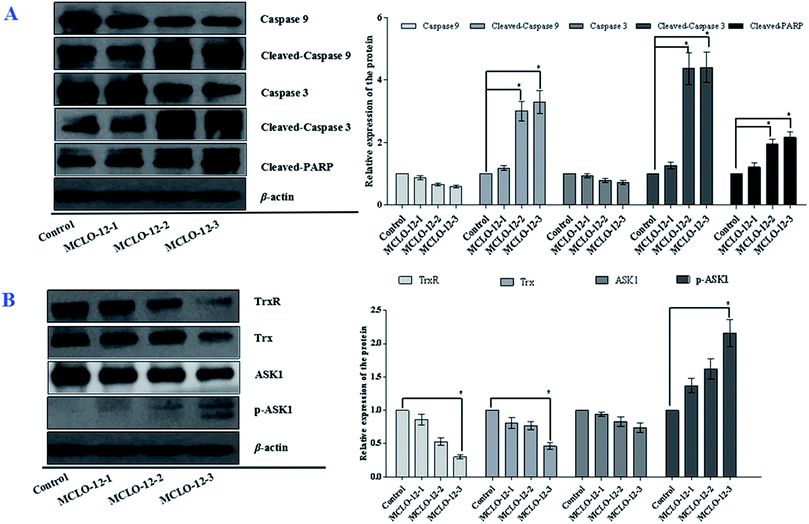 |
| Fig. 5 Effects of MCLO-12 on the expression of caspases, Trx, TrxR and ASK1. A549 cells were treated with MCLO-12 for 48 h and then subjected to western blotting. β-Actin was used as an internal control. The data shown are representative of three independent experiments. All data are presented as the mean ± SEM of three independent experiments. *p < 0.05 and **p < 0.01. | |
Studies have reported that ASK1/MAPK is involved in many cellular and immune responses, such as cell cycle regulation, and apoptosis. ASK1 is activated by a variety of stresses, such as the accumulation of ROS.32 The reduced type of Trx is a significant inhibitor of ASK1. ASK1 is activated by phosphorylation and with Trx disassociation may lead to the downstream activation of the JNK, ERK and p38 MAPK pathways.32 When Trx is oxidized, it dissociates from ASK1, which is then activated by the autophosphorylation of the kinase domain.33 As shown in Fig. 5B, MCLO-12 activates endogenous ASK1 in a dose-dependent manner in A549 cells. It is interesting that ASK1 expression dose-dependently decreased following treatment with MCLO-12. Next, we investigated how treatment with MCLO-12 affects the phosphorylation statuses of three different MAPKs (p38, JNK, and ERK1/2) in A549 cells by western blotting analysis. As shown in Fig. 6, p38, JNK and ERK were dose-dependently down-regulated following MCLO-12 treatment. These results indicate that MCLO-12 may increase cellular ROS levels by inhibiting the Trx system and activating the ASK1 and MAPK pathways; furthermore, MAPK activation might be involved in MCLO-12-mediated apoptosis.
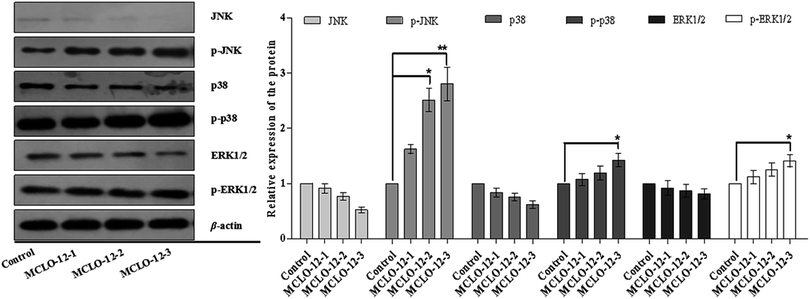 |
| Fig. 6 Effects of MCLO-12 on p38/JNK/ERK MAPK activation. A549 cells were treated with MCLO-12 for 48 h and then subjected to western blotting. The expressions of p38, JNK, and ERK1/2 were detected by western blotting. All protein levels were normalized to β-actin. The data shown are representative of three independent experiments. All data are presented as the mean ± SEM of three independent experiments. *p < 0.05 and **p < 0.01. | |
4 Discussion
Non-small cell lung cancer (NSCLC) is associated with high incidence and mortality.34 Molecular targeting has highly improved the treatment efficacy against NSCLC, but tumor growth involves a complex cascade of events and exhibits remarkable tumor cell proliferation. Therefore, new challenges with new chemotherapeutic agents are urgently needed. Apoptosis, a programmed mode of cell death, involves the degradation of cellular constituents by a serious of cysteine proteases named caspases. The intrinsic apoptotic pathway is characterized by mitochondrial permeabilization, causing the release of pro-apoptotic proteins into the cytosol.35 We found that MCLO-12 significantly reduced Δψm in A549 cells and up-regulated caspase signaling cascades (caspases 9 and 3), which led to the activation of the downstream cellular death substrate PARP. These data indicate that MCLO-12 may induce apoptosis via the intrinsic apoptotic pathway.
The mitochondrial respiratory chain is a major source of intracellular ROS generation.36 Excessive intracellular production of ROS will induce the cells to undergo apoptosis. In this study, MCLO-12 clearly induced mitochondrial dysfunction and increased the intracellular ROS levels in A549 cells. These results indicate that MCLO-12-mediated apoptosis in A549 cells might be governed by ROS-mediated mechanisms.
The Trx system is a ubiquitous oxidoreductase system, is over-expressed in various tumor types, and has important roles in cutting down enzymes and maintaining the intracellular protein thiol redox balance. The TrxR protein contains an active selenocysteine residue that is susceptible to electrophilic compounds and has been identified as a chemotherapeutic target for anticancer drug development.37 Recent studies have revealed that redox-regulating mechanisms, such as the Trx system, represent important targets for the treatment of malignancies. Disruption of the Trx system by MCLO-12 would interfere with the intracellular redox balance and cause the accumulation of ROS, which subsequently initiates apoptosis in A549 cells.
ASK1 is controlled by Trx and acts as a major contributor in regulating ROS-mediated apoptosis through the activation of the JNK and p38 signaling pathways.38 Our results demonstrate that MCLO-12 increases the phosphorylation of ASK1, which further phosphorylates the downstream MAPKs JNK and p38, suggesting that MCLO-12 may activate the ASK1-JNK/p38 signaling axis via an ROS-dependent pathway to exert its pro-apoptotic effects.
In general, we showed the ability of MCLO-12 to inhibit the proliferation of A549 cells by inducing apoptosis via mitochondrial pathways. Suppression of the Trx system with MCLO-12 could lead to ROS accumulation and subsequently activate a number of Trx-dependent pathways, including the ASK1, MAPK-p38 and JNK pathways, which may lead to MCLO-12-mediated apoptosis.
In summary, our study represents an analysis of the cellular signals which are affected by MCLO-12 treatment, and these findings also suggest a strong beginning to the development of Momordica charantia L. (Cucurbitaceae) peptides for anticancer applications.
Conflicts of interest
The authors declare no conflicts of interest.
Acknowledgements
We thank Professor Chen (Jinan University, Guangzhou, China) for technical assistance as well as critical editing of the manuscript.
References
- Y. L. Zhou, Y. J. Xu and C. W. Qiao, MiR-34c-3p suppresses the proliferation and invasion of non-small cell lung cancer (NSCLC) by inhibiting PAC1/MAPK pathway, Int. J. Clin. Exp. Pathol., 2015, 8, 6312–6322 CAS.
- J. Cai, J. Wu, H. Zhang, L. Fang, Y. Huang, Y. Yang, X. Zhu, R. Li and M. Li, miR-186 Downregulation Correlates with Poor Survival in Lung Adenocarcinoma, Where It Interferes with Cell-Cycle Regulation, Cancer Res., 2013, 73, 756–766 CrossRef CAS PubMed.
- F. Ciardiello, R. Caputo, G. Borriello, D. D. Bufalo, A. Biroccio, G. Zupi, A. R. Bianco and G. Tortora, ZD1839 (IRESSA), an EGFR-selective tyrosine kinase inhibitor, enhances taxane activity in bcl-2 overexpressing, multidrug-resistant MCF-7 ADR human breast cancer cells, Int. J. Cancer, 2010, 98, 463–469 CrossRef PubMed.
- R. S. Giovanni Forte, M. Antonella, L. Antonio, M. A. Ian, M. Piera, A. Claudio, C. Carla, P. Aldo and M. Silvana, Inhibition of CD73 Improves B Cell-Mediated Anti-Tumor Immunity in a Mouse Model of Melanoma, J. Immunol., 2012, 189, 2226–2233 CrossRef PubMed.
- M. Abdel-Tawwab, M. H. Ahmad, Y. A. E. Khattab and A. M. E. Shalaby, Effect of dietary protein level, initial body weight, and their interaction on the growth, feed utilization, and physiological alterations of Nile tilapia, Oreochromis niloticus (L.), Aquaculture, 2010, 298, 267–274 CrossRef CAS.
- E. Sarafraz-Yazdi, M. R. Pincus and J. Michl, Tumor-targeting peptides and small molecules as anti-cancer agents to overcome drug resistance, Curr. Med. Chem., 2014, 21, 1618–1630 CrossRef CAS PubMed.
- S. M. Simonsen, L. Sando, D. C. Ireland, M. L. Colgrave, R. Bharathi, U. Göransson and D. J. Craik, A Continent of Plant Defense Peptide Diversity: Cyclotides in Australian Hybanthus (Violaceae), Plant Cell, 2005, 17, 3176–3189 CrossRef CAS PubMed.
- A. Raman, Anti-diabetic properties and phytochemistry of Momordica charantia L. (Cucurbitaceae), Phytomedicine, 1996, 2, 349–362 CrossRef CAS PubMed.
- B. Svobodova, L. Barros, R. C. Calhelha, S. Heleno, M. J. Alves, S. Walcott, M. Bittova, V. Kuban and I. C. F. R. Ferreira, Bioactive properties and phenolic profile of Momordica charantia L. medicinal plant growing wild in Trinidad and Tobago, Ind. Crops Prod., 2017, 95, 365–373 CrossRef CAS.
- K. S. Rhee, E. S. Kim, B. K. Kim, B. M. Jung and K. C. Rhee, Extrusion of minced catfish with corn and defatted soy flours for snack foods, J. Food Process. Preserv., 2004, 28, 288–301 CrossRef.
- W. Zhang, M. Torabinejad and Y. Li, Evaluation of cytotoxicity of MTAD using the MTT-tetrazolium method, J. Endod., 2003, 29, 654–657 CrossRef PubMed.
- H. Koudelka, In situ detection of fragmented DNA (TUNEL assay) fails to discriminate among apoptosis, necrosis, and autolytic cell death: a cautionary note, Hepatology, 2010, 21, 1465–1468 Search PubMed.
- D. Tao, Y. Leng, J. Qin, H. Zhou, M. Shen and Y. U. Yuan, Analysis of cell cycle specific apoptosis by FTTC-Annexin V/PI, Chin. J. Lab. Med., 2002, 78–81 CAS.
- H. M. Yang, Y. M. Ham, W. J. Yoon, S. W. Roh, Y. J. Jeon, T. Oda, S. M. Kang, M. C. Kang, E. A. Kim and D. Kim, Quercitrin protects against ultraviolet B-induced cell death in vitro and in an in vivo zebrafish model, J. Photochem. Photobiol., B, 2012, 114, 126–131 CrossRef CAS PubMed.
- A. Prosperini, A. Juan-García, G. Font and M. J. Ruiz, Beauvericin-induced cytotoxicity via ROS production and mitochondrial damage in Caco-2 cells, Toxicol. Lett., 2013, 222, 204–211 CrossRef CAS PubMed.
- H. Rottenberg and S. Wu, Quantitative assay by flow cytometry of the mitochondrial membrane
potential in intact cells, Biochim. Biophys. Acta, Mol. Cell Res., 1998, 1404, 393–404 CrossRef CAS.
- H. S. Kim, H. D. Han, G. N. Armaiz-Pena, R. L. Stone, E. J. Nam, J. W. Lee, M. M. Shahzad, A. M. Nick, S. J. Lee and J. W. Roh, Functional roles of Src and Fgr in ovarian carcinoma, Clin. Cancer Res., 2011, 17, 1713–1721 CrossRef CAS PubMed.
- F. Sun, W. Zong, R. Liu, M. Wang, P. Zhang and Q. Xu, The Relative Charge Ratio Between C and N Atoms in Amide Bond Acts as a Key Factor to Determine Peptide Fragment Efficiency in Different Charge States, J. Am. Soc. Mass Spectrom., 2010, 21, 1857–1862 CrossRef CAS PubMed.
- G. V. Putcha, K. L. Moulder, J. P. Golden, P. Bouillet, J. A. Adams, A. Strasser and E. M. J. Jr, Induction of BIM, a Proapoptotic BH3-Only BCL-2 Family Member, Is Critical for Neuronal Apoptosis, Neuron, 2001, 29, 615–628 CrossRef CAS PubMed.
- Y. Wei, D. Weng, F. Li, X. Zou, D. O. Young, J. Ji and P. Shen, Involvement of JNK regulation in oxidative stress-mediated murine liver injury by microcystin-LR, Apoptosis, 2008, 13, 1031 CrossRef CAS PubMed.
- A. K. Alasmari, Z. Ullah, A. A. Balowi and M. Islam, In vitro determination of the efficacy of scorpion venoms as anti-cancer agents against colorectal cancer cells: a nano-liposomal delivery approach, Int. J. Nanomed., 2017, 12, 559–574 CrossRef CAS PubMed.
- A. Díaz-García, J. L. Ruiz-Fuentes, H. Rodríguez-Sánchez and J. A. F. Castro, Rhopalurus junceusscorpion venom induces apoptosis in the triple negative human breast cancer cell line MDA-MB-231, J. Venom Res., 2017, 8, 9–13 Search PubMed.
- G. Rimon, C. E. Bazenet, K. L. Philpott and L. L. Rubin, Increased surface phosphatidylserine is an early marker of neuronal apoptosis, J. Neurosci. Res., 2015, 48, 563–570 CrossRef.
- S. K. Connors, G. Chornokur and N. B. Kumar, New Insights Into the Mechanisms of Green Tea Catechins in the Chemoprevention of Prostate Cancer, Nutr. Cancer, 2012, 64, 4–22 CrossRef CAS PubMed.
- S. Shin, B. Sung and Y. Cho, An Anti-apoptotic Protein Human Survivin Is a Direct Inhibitor of Caspase-3 and -7, Biochemistry, 2001, 40(4), 1117–1123 CrossRef CAS PubMed.
- P. Costantini, E. Jacotot, D. Decaudin and G. Kroemer, Mitochondrion as a novel target of anticancer chemotherapy, J. Natl. Cancer Inst., 2000, 92, 1042–1053 CrossRef CAS PubMed.
- J. D. Ly, D. R. Grubb and A. Lawen, The mitochondrial membrane potential (Δψm) in apoptosis; an update, Apoptosis, 2003, 8, 115–128 CrossRef CAS PubMed.
- D. Li, T. Han, S. Xu, T. Zhou, K. Tian, X. Hu, K. Cheng, Z. Li, H. Hua and J. Xu, Antitumor and Antibacterial Derivatives of Oridonin: A Main Composition of Dong-Ling-Cao, Molecules, 2016, 21, 575 CrossRef PubMed.
- A. Sesso, J. E. Belizário, M. M. Marques, M. L. Higuchi, R. I. Schumacher, A. Colquhoun, E. Ito and J. Kawakami, Mitochondrial swelling and incipient outer membrane rupture in preapoptotic and apoptotic cells, Anat. Rec., 2012, 295, 1647–1659 CrossRef CAS PubMed.
- L. L. Wang, Q. L. Yu, L. Han, X. L. Ma, R. D. Song, S. N. Zhao and W. H. Zhang, Study on the effect of reactive oxygen species-mediated oxidative stress on the activation of mitochondrial apoptosis and the tenderness of yak meat, Food Chem., 2018, 244, 394 CrossRef CAS PubMed.
- P. V. Raninga, G. D. Trapani and K. F. Tonissen, Cross Talk between Two Antioxidant Systems, Thioredoxin and DJ-1: Consequences for Cancer, Oncoscience, 2014, 1, 95–110 CrossRef PubMed.
- E. Hedstrom, S. Eriksson, J. Zawacka-Pankau, E. S. J. Arner and G. Selivanova, p53-dependent inhibition of TrxR1 contributes to the tumor-specific induction of apoptosis by RITA, Cell Cycle, 2009, 8, 3584–3591 CrossRef PubMed.
- B. M. Ji, T. Van-Long, K. Se-Yeon, N. X. N. Giang, J. Mira, S. G. Hong, L. Jong-Won and J. Woo-Sik, Induction of Nrf2/ARE-mediated cytoprotective genes by red ginseng oil through ASK1-MKK4/7-JNK and p38 MAPK signaling pathways in HepG2 cells, J. Ginseng Res., 2016, 40, 423–430 CrossRef PubMed.
- L. Li, T. Zhu, Y. F. Gao, W. Zheng, C. J. Wang, L. Xiao, M. S. Huang, J. Y. Yin, H. H. Zhou and Z. Q. Liu, Targeting DNA Damage Response in the Radio(Chemo)therapy of Non-Small Cell Lung Cancer, Int. J. Mol. Sci., 2016, 17, 839 CrossRef PubMed.
- R. M. Kluck, M. D. Esposti, G. Perkins, C. Renken, T. Kuwana, E. Bossy-Wetzel, M. Goldberg, T. Allen, M. J. Barber, D. R. Green and D. D. Newmeyer, The pro-apoptotic proteins, Bid and Bax, cause a limited permeabilization of the mitochondrial outer membrane that is enhanced by cytosol, J. Cell Biol., 1999, 147, 809–822 CrossRef CAS PubMed.
- A. A. Starkov, Measurement of mitochondrial ROS production, Methods Mol. Biol., 2010, 648, 245–255 CrossRef CAS PubMed.
- A. Fischer, J. Pallauf, K. Gohil, S. U. Weber, L. Packer and G. Rimbach, Effect of selenium and vitamin E deficiency on differential gene expression in rat liver, Biochem. Biophys. Res. Commun., 2001, 285, 470–475 CrossRef CAS PubMed.
- H.-L. Huang, M.-J. Hsieh, M.-H. Chien, H.-Y. Chen, S.-F. Yang and P.-C. Hsiao, Glabridin Mediate Caspases Activation and Induces Apoptosis through JNK1/2 and p38 MAPK Pathway in Human Promyelocytic Leukemia Cells, PLoS One, 2014, 9, 98943–98951 CrossRef PubMed.
|
This journal is © The Royal Society of Chemistry 2019 |
Click here to see how this site uses Cookies. View our privacy policy here.