DOI:
10.1039/C9RA00616H
(Paper)
RSC Adv., 2019,
9, 7203-7209
Direct synthesis of 2-oxo-acetamidines from methyl ketones, aromatic amines and DMF via copper-catalyzed C(sp3)–H amidination†
Received
24th January 2019
, Accepted 25th February 2019
First published on 4th March 2019
Abstract
A convenient method for the synthesis of 2-oxo-acetamidines from methyl ketones using aromatic amines and DMF as nitrogen sources is reported via copper-catalyzed C(sp3)–H amidination. Various methyl ketones react readily with aromatic amines and DMF, producing 2-oxo-acetamidines in yields of 47 to 92%. This protocol features the simultaneous formation of C–N and C
N bonds using DMF and aromatic amines as two different nitrogen sources. It thus provides an efficient approach to construct acyclic amidines via three C(sp3)–H bond amidination. Based on the preliminary experiments, a plausible mechanism of this transformation is disclosed.
Introduction
Amidines are important structural motifs in natural products, bioactive molecules and functional materials.1 They have profound applications in diverse areas such as medicinal chemistry, synthetic intermediates, catalyst design, material science, supramolecular chemistry, and coordination chemistry.2 Therefore, the direct and convenient construction of amidines is an important and popular research field, particularly for the synthesis of 2-oxo-acetamidines due to the wide applications of their carbonyl group as a versatile intermediate in the synthesis of a broad range of acetamidine derivatives.3 Recently, transition metal and metal-free catalyzed C–N bond formation has arisen as an excellent synthetic method to build complex structures because it reduces prefunctionalization while improving atom economy and energy efficiency.4 These protocols used nitriles,5 isonitriles,6 organic azides,7 and amines8 as nitrogenous sources, which are considered important pathways to construct cyclic and acyclic amidines.
Amides are key building blocks of proteins and are broadly found in natural and manufactured organic molecules.9 Meanwhile, amides are also often used as precursor molecules to synthesize amidines.10 The general method of synthesizing amidines by amides is mainly divided into the following two strategies: (1) direct amidination of the amide and amine scaffolds without decarbonylation of amide, providing cyclic amidines as the final product (eqn (a)–(c) in Scheme 1);10 (2) decarbonylation of the amide to semicyclic amidines (Scheme 1, eqn (d)).11 Although significant progress has been made in this field, direct and efficient methods for the synthesis of acyclic amidines from amide are still highly desired.
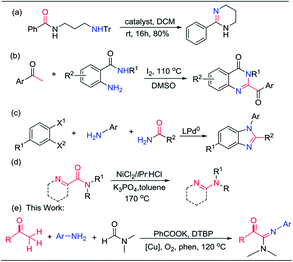 |
| Scheme 1 Methods for the synthesis of amidines. | |
As our continuing interest in carbon–nitrogen coupling fields,12 and inspired by the above-mentioned studies, we herein report a copper-catalyzed adimination of methyl ketones, aromatic amines and DMF to acyclic amidines, 2-oxo-acetamidines (Scheme 1, eqn (e)). Even though the reactions of methyl ketones with amines or amides are known to achieve the synthesis of α-ketoamides by using metal and metal-free approaches,13 the synthesis of acyclic amidines using amides and aromatic amines as two different nitrogen sources has not been described previously. This approach allows direct amidination of three C(sp3)–H bonds and affords C–N bond and C
N bond simultaneously. This protocol provides a versatile approach to 2-oxo-acetamidines with good functional group tolerance. Notably, less reactive aliphatic methyl ketone could also be converted into the corresponding amidine derivatives.
Results and discussion
Initially, the reaction of acetophenone 1a, aniline 2a and DMF catalyzed by copper salts was performed to optimize the reaction conditions. The results were listed as shown in Table 1. To our delight, under CuCl2 conditions, reaction of 1a, 2a with DMF afforded mainly oxidative amidination product 4aa in 15–85% yield (entries 1–8, Table 1). Subsequently, a number of other oxidants including DTBP, TBHP, BPO, AIBN were explored. Only the DTBP and TBHP effected the reaction, among which DTBP proved to be superior oxidant, giving 4aa in 75% yield (entries 1–4; Table 1). Gratifyingly, further improvement of the process was achieved when the reaction conducted under the ligand of phen (1,10-phenanthroline monohydrate), affording 4aa in 85% yield (entry 5; Table 1). Copper salts exhibited unique ability in this transformation, as the reaction did not occur without copper catalyst (entry 6; Table 1). After a series of copper salts were tested (entries 9–13; Table 1), CuCl2 was demonstrated to be the best choice. Further studies indicated that base can promote this transformation, PhCOOK is optimal (entries 14–17; Table 1). Under the nitrogen atmosphere, 1a and 2a could not react to form 4aa (entry 8; Table 1). This fact implied that oxygen was essential for this reaction. Reaction temperature and reaction time were also scanned to improve the yield, and 120 °C and 36 h were determined as optimum for the oxidative amidination reaction (entries 18–22; Table 1). After screening on different parameters, the highest yield of 4aa (85%) was achieved when the reaction was carried out with CuCl2 (0.1 mmol), phen (0.1 mmol), PhCOOK (0.5 mmol) and DTBP (2 mmol) at 120 °C under the atmosphere of oxygen in DMF (entry 9; Table 1).
Table 1 Optimization of the reaction conditionsa
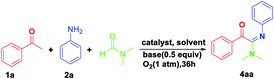
|
Entry |
[Cu] (mol%) |
Base |
Solvent |
Oxidant (equiv.) |
T (°C) |
Yieldb % |
Reaction conditions: 1a (0.5 mmol), 2a (0.5 mmol), catalyst (0.1 mmol), and base (0.5 mmol), oxidant, additive and solvent (2 mL) under O2 atmosphere at 120 °C for 36 h. Isolated yields. Phen (0.1 mmol). Air. N2 atmosphere. 12 h. 24 h. DTBP = di-tert-butyl peroxide, TBHP = tert-butyl hydroperoxide, BPO = benzoyl peroxide, AIBN = 2,2′-azobis(2-methylpropionitrile), phen = 1,10-phenanthroline monohydrate, DMSO = dimethyl sulfoxide, DMF = N,N-dimethylformamide, THF = tetrahydrofuran. |
1 |
CuCl2 (20) |
PhCOOK |
DMF |
DTBP(4) |
120 |
75 |
2 |
CuCl2 (20) |
PhCOOK |
DMF |
TBHP(4) |
120 |
15 |
3 |
CuCl2 (20) |
PhCOOK |
DMF |
BPO(4) |
120 |
0 |
4 |
CuCl2 (20) |
PhCOOK |
DMF |
AIBN(4) |
120 |
0 |
5c |
CuCl2 (20) |
PhCOOK |
DMF |
DTBP(4) |
120 |
85 |
6 |
|
PhCOOK |
DMF |
DTBP(4) |
120 |
0 |
7d |
CuCl2 (20) |
PhCOOK |
DMF |
DTBP(4) |
120 |
36 |
8e |
CuCl2 (20) |
PhCOOK |
DMF |
DTBP(4) |
120 |
0 |
9 |
CuCl (20) |
PhCOOK |
DMF |
DTBP(4) |
120 |
56 |
10 |
CuBr (20) |
PhCOOK |
DMF |
DTBP(4) |
120 |
23 |
11 |
CuI (20) |
PhCOOK |
DMF |
DTBP(4) |
120 |
19 |
12 |
Cu(OAc)2 (20) |
PhCOOK |
DMF |
DTBP(4) |
120 |
50 |
13 |
Cu(acac)2 (20) |
PhCOOK |
DMF |
DTBP(4) |
120 |
47 |
14 |
CuCl2 (20) |
PhCOONa |
DMF |
DTBP(4) |
120 |
60 |
15 |
CuCl2 (20) |
CH3COONa |
DMF |
DTBP(4) |
120 |
55 |
16 |
CuCl2 (20) |
CH3ONa |
DMF |
DTBP(4) |
120 |
45 |
17 |
CuCl2 (20) |
Cs2CO3 |
DMF |
DTBP(4) |
120 |
Trace |
18 |
CuCl2 (20) |
PhCOOK |
DMF |
DTBP(4) |
80 |
36 |
19 |
CuCl2 (20) |
PhCOOK |
DMF |
DTBP(4) |
100 |
50 |
20 |
CuCl2 (20) |
PhCOOK |
DMF |
DTBP(4) |
140 |
71 |
21f |
CuCl2 (20) |
PhCOOK |
DMF |
DTBP(4) |
120 |
55 |
22g |
CuCl2 (20) |
PhCOOK |
DMF |
DTBP(4) |
120 |
74 |
With the optimized reaction conditions in hand, the scope with respect to the methyl ketones was firstly evaluated (Table 2). A wide variety of methyl ketones bearing electron-donating and electron-withdrawing functional groups gave the corresponding products in moderate to excellent yields (4aa–4ra). The position of the substituents on the aryl ring had a minor effect on the efficiency of this transformation. For example, not only methyl ketones 1d and 1i possessing para substituents but also substrates (1c,1e) and 1b, bearing substituents in meta and ortho position, afforded the corresponding products in good yields. In addition, our newly developed protocol tolerated a variety of functionalities, including halogens (4ea, 4fa, 4ga and 4ha), methoxy (4ka), ethoxy (4la), ester groups (4ma), t-butyl (4na). Notably, reactive primary amine on the aromatic ring were also tolerated (4oa). Fortunately, when p-nitroaniline were used as substrates, the desired products were detected (4ja) in moderate yield. Heteroaryl methyl ketones were also investigated, and the corresponding products were obtained in moderate to excellent yields (4pa, 4qa, 4ra). Pyridine methyl ketones failed to participate in the reaction to obtain the desired product (4sa,4ta). Moreover, this methodology could be extended to alkyl methyl ketones as well, although in moderate yield (4ua).
Table 2 Scope of methyl ketonesa
Reaction conditions: 1a (0.5 mmol), 2a (0.5 mmol), CuCl2 (0.1 mmol), PhCOOK (0.5 mmol), DTBP (2 mmol), phen (0.1 mmol), N,N-dimethylformamide (2 mL), O2 (1 atm), 36 h, 120 °C. Isolated yields. GC yields. |
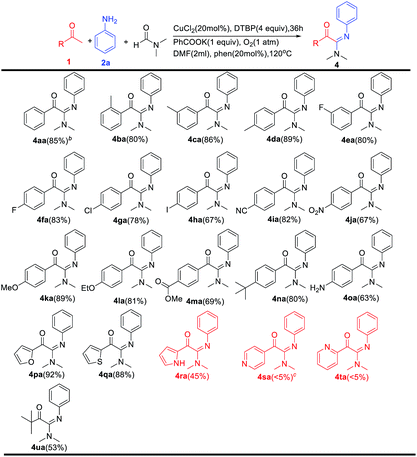 |
Next, the scope of aromatic amines was explored (Table 3). A wide range of structurally diverse aromatic amines were suitable substrates for this transformation. For example, aryl amines bearing methyl groups could generate the corresponding 2-oxo-acetamidines 4ab–4ad in excellent yields. Under the optimal reaction conditions, the meta-halogen substrates (4ae–4ag) and the para-halogen substrates (4ah–4ak) are both completely tolerated. Also, functional groups such 4-isopropyl (4am), 3,5-dimethoxy (4an) were well tolerated under our reaction conditions. Fortunately, when p-nitroaniline were used as substrates, the desired products were detected (4al) in moderate yield. Moreover, heteroaryl amines were also investigated, and the corresponding products were obtained in moderate yields (4ao,4ap). Unfortunately, pyridin-2-amine and 5-methylisoxazol-3-amine are not prone to this reaction (4aq,4ar). Meanwhile, quinolin-8-amine does not react (4as).
Table 3 Scope of aromatic aminesa
Reaction conditions: 1a (0.5 mmol), 2a (0.5 mmol), CuCl2 (0.1 mmol), PhCOOK (0.5 mmol), DTBP (2 mmol), phen (0.1 mmol), N,N-dimethylformamide (2 mL), O2 (1 atm), 36 h, 120 °C. Isolated yields. GC yields. N.D. = not detected. |
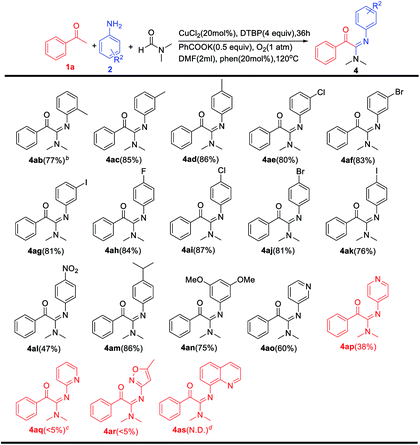 |
Then, the scope of amides was explored (Table 4). Unfortunately, this protocol was not general to a wide range of formamides including N,N-diethylformamide, N-methylformamide, N-methyl-N-phenylformamide, morpholine-4-carbaldehyde with piperidine-1-carbaldehyde, giving no desired products.
Table 4 Scope of N,N-disubstituted formamidesa
Reaction conditions: 1a (0.5 mmol), 2a (0.5 mmol), CuCl2 (0.1 mmol), PhCOOK (0.5 mmol), DTBP (2 mmol), phen (0.1 mmol), DMSO (2 mL), O2 (1 atm), 36 h, 120 °C. N.D. = not detected. GC yields. |
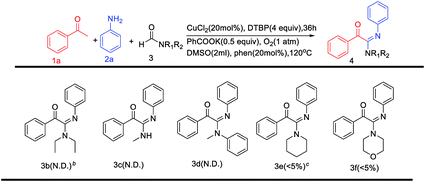 |
To elucidate the mechanism, some control experiments were performed (Scheme 2). At first, the reaction of acetophenone 1a with aniline 2a was carried out without CuCl2, and the desired product 4aa was not detected (Scheme 2 [eqn (1)]). When the reaction was done in the absence of O2, 4aa was also not detected (Scheme 2 [eqn (1)]). These results suggested that both CuCl2 and O2 were necessary for the reaction. When 2.0 equiv. of BHT (2,6-di-tert-butyl-4-methylphenol) was added under the standard conditions, the reaction was inhibited substantially (Scheme 2 [eqn (1)]). The result suggests that the reaction may involve a radical reaction. Our reaction may be involved dimethylamine generated in situ from DMF.14 To verify this pathway, dimethylamine 5 was then used instead of DMF under the same conditions (Scheme 2 [eqn (2)]), and only a little amount of 4aa was detected. This result implies that an aminyl radical, not an amine, was the intermediate in the transformation process. According to the literatures and experimental results,15 we predicted that acetophenone 1a may be oxidized to phenylglyoxal intermediate, and then form Schiff base to complete the transformation process. Therefore, we conducted a control experiment in which phenylglyoxal monohydrate 6 and imine 7 were used as substrates under standard conditions (Scheme 2 [eqn (3) and (4)]). The experimental results show that the target product is obtained in a lower yield, indicating that 6 and 7 may not be the key intermediates in the reaction. As shown in eqn (5), the reactions between 2a and 2-(dimethylamino)-1-phenylethan-1-one 8 were investigated. Under the optimized conditions, the product 4aa could be furnished in 80% yields (Scheme 2 [eqn (5)]). The results demonstrated that the reaction may have undergone 8 intermediate process. Notably, 2-oxo-N,2-diphenylacetamide 9 and 1-phenyl-2-(phenylamino)ethan-1-one 10 both were not suitable substrates for the reaction (Scheme 2 [eqn (6) and (7)]). When the reaction was carried out in the absence of acetophenone 1a, N,N-dimethyl-N′-phenylformimidamide 11 and 1,2-diphenyldiazene 12 were detected (Scheme 2 [eqn (8)]). This result indicated the cationic aniline radicals maybe formed in this reaction.16
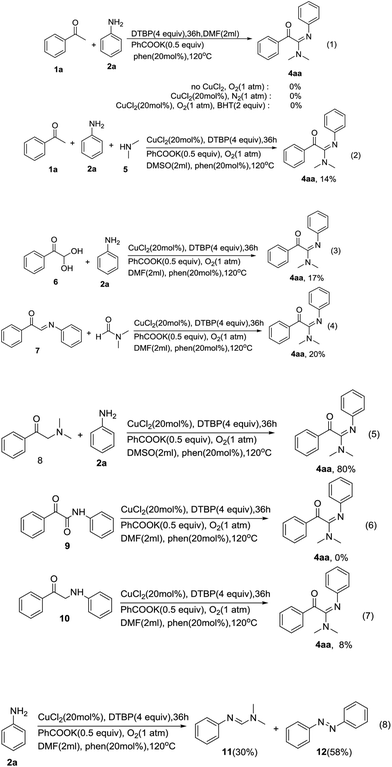 |
| Scheme 2 Different control experiments. | |
On the basis of the above results, a plausible mechanism for the copper-catalyzed aerobic oxidative coupling is illustrated in Scheme 3. In the first step, the tert-butoxyl radicals trapped hydrogen from the aryl methyl ketone and DMF respectively to form radical B13b,17 and aminyl radical A.18 Then, decarboxylation of A produced aminyl radical C, which reacted with radical B to generate intermediate D. D was then converted to E in the presence of tert-butoxyl radical. Meanwhile, a single-electron oxidation of anilines mediated by Cu(II) occurred, affording corresponding radical cations F.16 Cationic radical F coupled with radical E to obtain G. Hydrogen ion of G was removed by base to give the product H. Finally, H is oxidized under standard conditions to form the desired product I (Scheme 3).
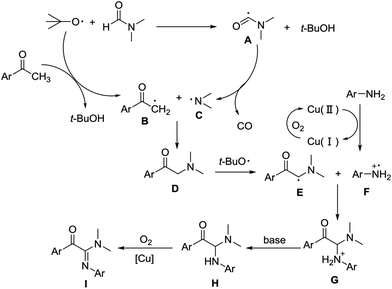 |
| Scheme 3 A proposed mechanism for the direct transformation. | |
Conclusions
In conclusion, the copper-catalyzed oxidative amidination of methyl ketones with aromatic amines and DMF has been developed. Acyclic 2-oxo-acetamidines could be obtained with moderate to good yields. This protocol features with acyclic amidines formation using DMF and aromatic amines as two different nitrogen sources. Further investigations on reaction scope and synthetic application are underway in our laboratory.
Conflicts of interest
There are no conflicts to declare.
Acknowledgements
This work was financially supported by the National Natural Science Foundation of China (21372068, 21572049), Science and Technology Program of Hunan Province, China (2014GK3115) and Science and Technology Program of Changsha, China (K1508004-11).
Notes and references
-
(a) R. J. Young, Bioorg. Med. Chem. Lett., 2000, 10, 597 CrossRef CAS PubMed;
(b) E. M. Stone, T. H. Schaller, H. Bianchi, M. D. Person and W. Fast, Biochemistry, 2005, 44, 13744 CrossRef CAS PubMed;
(c) R. G. Doveston, R. Steendam, S. Jones and R. J. K. Taylor, Org. Lett., 2012, 14, 1122 CrossRef CAS PubMed;
(d) C. Maccallini, A. Patruno, N. Besker, J. Ali, A. Ammazzalorso, B. D. Filippis, S. Franceschelli, L. Giampietro, M. Pesce and M. Reale, J. Med. Chem., 2009, 52, 1481 CrossRef CAS PubMed;
(e) C. Maccallini, A. Patruno, F. Lannutti, M. Fantacuzzi, S. Franceschelli, L. Giampietro, S. Masella and M. Felaco, Bioorg. Med. Chem. Lett., 2010, 20, 6495 CAS;
(f) A. Patruno, S. Franceschelli, M. Pesce, C. Maccallini, M. Fantacuzzi, L. Speranza, A. Ferrone, M. A. De Lutiis, E. Ricciott and R. Amoroso, Biochim. Biophys. Acta, 2012, 1820, 2095 CAS;
(g) F. T. Edelmann, Chem. Soc. Rev., 2009, 38, 2253 RSC;
(h) Y. Luo, B. Knuckley, Y. H. Lee, M. R. Stallcup and P. R. Thompson, J. Am. Chem. Soc., 2006, 128, 1092 CrossRef CAS PubMed;
(i) Q. Dai, Y. Jiang, J.-T. Yu and J. Cheng, Chem. Commun., 2015, 51, 16645 RSC.
-
(a) J. V. Greenhill and P. Lue, Prog. Med. Chem., 1993, 30, 203 CrossRef CAS PubMed;
(b) S. D. Guile, L. Alcaraz, T. N. Birkinshaw, K. C. Bowers, M. R. Ebden, M. Furber and M. J. Stocks, J. Med. Chem., 2009, 52, 3123 CrossRef CAS PubMed;
(c) M. Y. Lee, M. H. Kim, J. Kim, S. H. Kim, B. T. Kim, I. H. Jeong, S. Chang and S. Y. Chang, Bioorg. Med. Chem. Lett., 2010, 20, 541 CrossRef CAS PubMed;
(d) G. Brasche and S. L. Buchwald, Angew., Chem. Int. Ed., 2008, 47, 1932 (Angew. Chem., 2008, 120, 1958) CrossRef CAS PubMed;
(e) S. Caron, L. Wei, J. Douville and A. Ghosh, J. Org. Chem., 2010, 75, 945 CrossRef CAS PubMed;
(f) R. Khan, M. Arfan, J. Mahmood, S. Anjum and M. I. Choudhary, Chin. Chem. Lett., 2010, 21, 905 CrossRef CAS;
(g) J. R. Harjani, C. Liang and P. G. Jessop, J. Org. Chem., 2011, 76, 1683 CrossRef CAS PubMed;
(h) M. A. McGowan, C. Z. McAvoy and S. L. Buchwald, Org. Lett., 2012, 14, 3800 CrossRef CAS PubMed;
(i) Y. F. Wang, X. Zhu and S. Chiba, J. Am. Chem. Soc., 2012, 134, 3679 (J. Am. Chem. Soc., 2012, 134, 11980) CrossRef CAS PubMed;
(j) J. E. Taylor, S. D. Bull and J. M. J. Williams, Chem. Soc. Rev., 2012, 41, 2109 RSC;
(k) M. Mączka, J. Janczak, M. Trzebiatowska, A. Sieradzki, S. Pawlus and A. Pikul, Dalton Trans., 2017, 46, 8476 RSC;
(l) J. Barker and M. Kilner, Coord. Chem. Rev., 1994, 133, 219 CrossRef CASS. H. Oakley, D. B. Soria, M. P. Coles and P. B. Hitchcock, Dalton Trans., 2004, 537 RSC; F. T. Edelmann, Adv. Organomet. Chem., 2008, 57, 183 CrossRef CAS.
-
(a) V. A. Mamedov, N. A. Zhukova, V. V. Syakaev, A. T. Gubaidullin, T. N. Beschastnova, D. I. Adgamova, A. I. Samigullina and S. K. Latypov, Tetrahedron, 2013, 69, 1403 CrossRef CAS;
(b) D.-H. Wang, Y.-X. Yang, Y.-Q. Yang, T.-C. Zhao, X. Wu and S.-K. Wang, Sci. Bull., 2006, 51, 785 CrossRef CAS;
(c) A. Tantawy, A. E Barghash, S. Badr and R. Gomaa, Heterocycl. Commun., 2013, 19, 125 CAS;
(d) N. R. Perl and J. L. Leighton, Org. Lett., 2007, 9, 3699 CrossRef CAS PubMed;
(e) J.-L. Du, L.-J. Li and Y.-F. Li, Inorg. Chem. Commun., 2005, 8, 246 CrossRef CAS;
(f) H. C Chang, B. C. Son, G. Y. Song, J. Y. Shin, C. S. Ha, H. S. Suh and I. Kim, Macromol. Res., 2013, 21, 118 CrossRef;
(g) V. A. Mamedov, A. M. Murtazina, N. A. Zhukova, T. N. Beschastnova, Il. K. Rizvanov and S. K. Latypov, Tetrahedron, 2014, 70, 7567 CrossRef CAS.
-
(a) B. Wang, H.-F. Du and Y. A. Shi, Angew. Chem., 2008, 120, 8348 (Angew. Chem., Int. Ed., 2008, 47, 8224) CrossRef;
(b) J. J. Neumann, S. Rakshit, T. Droge and F. Glorius, Angew. Chem., 2009, 121, 7024 (Angew. Chem., Int. Ed., 2009, 48, 6892) CrossRef;
(c) Y. Tan and J. F. Hartwig, J. Am. Chem. Soc., 2010, 132, 3676 CrossRef CAS PubMed;
(d) K. Shin, H. Kim and S. Chang, Acc. Chem. Res., 2015, 48, 1040 CrossRef CAS PubMed;
(e) J. Jiao, K. Murakami and K. Itami, ACS Catal., 2016, 6, 610 CrossRef CAS;
(f) W. Choi, J. Kim, T. Ryu, K.-B. Kim and P. H. Lee, Org. Lett., 2015, 17, 3330 CAS;
(g) C. Du, P.-X. Li, X.-J. Zhu, J.-N. Han, J.-L. Niu and M.-P. Song, ACS Catal., 2017, 7, 2810 CrossRef CAS;
(h) Y. Park, Y. Kim and S. Chang, Chem. Rev., 2017, 117, 9247 CrossRef CAS PubMed;
(i) S. B. Lafollée, R. Gil, D. Prim and J. Hannedouche, Molecules, 2017, 22, 1901 CrossRef PubMed;
(j) Y. N. Timsina, B. F. Gupton and K. C. Ellis, ACS Catal., 2018, 8, 5732 CrossRef CAS.
-
(a) G. Rousselet, P. Capdeviclle and M. Maumy, Tetrahedron Lett., 1993, 34, 6395 CAS;
(b) V. Y. Kukushkin and A. J. L. Pombeiro, Chem. Rev., 2002, 102, 1771 CAS;
(c) J.-F. Wang, F. Xu, T. Cai and Q. Shen, Org. Lett., 2008, 10, 445 CrossRef CAS PubMed;
(d) S. Ueda and H. Nagasawa, J. Am. Chem. Soc., 2009, 131, 15080 CAS;
(e) J. Savmarker, J. Rydfjord, J. Gising, L. R. Odell and M. Larhed, Org. Lett., 2012, 14, 2394 CrossRef PubMed;
(f) J. Rydfjord, F. Svensson and A. Trejos, Mats Larhed, Chem.–Eur. J., 2013, 19, 13803 CrossRef CAS PubMed.
-
(a) A. T. Khan, R. Sidick Basha, M. Lala and M. H. Mir, RSC Adv., 2012, 2, 5506–5509 CAS;
(b) A. Kumar, D. Saxena and M. K. Gupta, Green Chem., 2013, 15, 2699 CAS;
(c) T. Vlaar, E. Ruijter, B. U. W. Maes and R. V. A. Orru, Angew. Chem., Int. Ed., 2013, 52, 7084 CAS;
(d) P. Mampuys, Y.-P. Zhu, T. Vlaar, E. Ruijter, R. V. A. Orru and B. U. W. Maes, Angew. Chem., Int. Ed., 2014, 15, 12849 CrossRef PubMed;
(e) Q. Dai, Y. Jiang, J.-T. Yu and J. Cheng, Chem. Commun., 2015, 51, 16645 RSC;
(f) F.-X. Zhu, Y.-H. Li, Z.-C. Wang and X.-F. Wu, Chem.–Eur. J., 2016, 22, 7743 CrossRef CAS PubMedZ.-Y. Gu, C.-G. Liu, S.-Y. Wang and S.-J. Ji, J. Org. Chem., 2017, 82, 2223 CAS.
-
(a) I. Bae, H. Han and S. Chang, J. Am. Chem. Soc., 2005, 127, 2038 CrossRef CAS PubMed;
(b) S. Brase, C. Gil, K. Knepper and V. Zimmermann, Angew. Chem., Int. Ed., 2005, 44, 5188 CAS;
(c) X.-L. Xu, X.-N. Li, L. Ma, N. Ye and B.-J. Weng, J. Am. Chem. Soc., 2008, 130, 14048 CrossRef CAS PubMed;
(d) E. J. Yoo, M. Ahlquist, I. Bae, K. B. Sharpless, V. V. Fokin and S. Chang, J. Org. Chem., 2008, 73, 5520 CrossRef CAS PubMed;
(e) X.-L. Xu, Z.-C. Ge, D.-P. Cheng, L. Ma, C.-S. Lu, Q.-F. Zhang, N. Yao and X.-N. Li, Org. Lett., 2010, 12, 897 CrossRef CAS PubMed;
(f) M. Aswad, J. Chiba, T. Tomohiro and Y. Hatanaka, Chem. Commun., 2013, 49, 10242 RSC;
(g) G. Murugavel and T. Punniyamurthy, J. Org. Chem., 2015, 80, 6291 CrossRef CAS PubMed;
(h) S. Y. Chow and L. R. Odell, J. Org. Chem., 2017, 82, 2515 CAS.
-
(a) C. Zhang, L. Zhang and N. Jiao, Adv. Synth. Catal., 2012, 354, 1293 CrossRef CAS;
(b) G. Martinez-Ariza, M. Ayaz and C. Hulme, Tetrahedron Lett., 2013, 54, 6719 CrossRef CAS PubMed;
(c) A. Kumar, N. Battini, R. R. Kumar, S. Athimoolam and Q. N. Ahmed, Eur. J. Org. Chem., 2016, 3344 CrossRef CAS;
(d) G. Martinez-Ariza, N. McConnell and C. Hulme, Org. Lett., 2016, 18, 1864 CrossRef CAS PubMed;
(e) X.-X. Liu, Z.-Y. Wu, Y.-Q. He, X.-Q. Zhou, T. Hu, C.-W. Ma and G.-S. Huang, Adv. Synth. Catal., 2016, 358, 2385 CrossRef CAS;
(f) C. Chen, M.-H. Zhu, L.-H. Jiang, Z.-B. Zeng, N.-N. Yi and J.-N. Xiang, Org. Biomol. Chem., 2017, 15, 8134 RSC;
(g) J. Zhang, X. Wu, Q. Gao, X. Geng, P. Zhao, Y.-D. Wu and A.-X. Wu, Org. Lett., 2017, 19, 408 CrossRef CAS PubMed;
(h) L. Dutta and P. J. Bhuyan, Tetrahedron, 2018, 74, 5770 CrossRef CAS.
- The Amide Linkage: Structural Significance in Chemistry,
Biochemistry and Materials Science, ed.A. Greenberg, C. M. Breneman and J. F. Liebman, Wiley, New York, 2003 Search PubMed.
-
(a) Q. Zhu and Y.-X. Lu, Org. Lett., 2010, 12, 4156 CrossRef CAS PubMed;
(b) Y. Jiao and C.-S. Cho, Appl. Organomet. Chem., 2015, 29, 372 CrossRef CAS;
(c) W. Phakhodee, S. Wangngae, N. Wiriya and M. Pattarawarapan, Tetrahedron Lett., 2016, 57, 5351 CrossRef CAS;
(d) W. Phakhodee, S. Wangngae and M. Pattarawarapan, J. Org. Chem., 2017, 82, 8058 CrossRef CAS PubMed;
(e) W. A. Loughlin, I. D. Jenkins and M. J. Petersson, J. Org. Chem., 2013, 78, 7356 CrossRef CAS PubMed;
(f) Y.-P. Zhu, Z. Fei, M.-C. Liu, F.-C. Jia and A.-X. Wu, Org. Lett., 2013, 15, 378 CrossRef CAS PubMed;
(g) N. T. Jui and S. L. Buchwald, Angew. Chem., Int. Ed., 2013, 52, 11624 CrossRef CAS PubMed;
(h) V. Ganesh, D. Sureshkumar, D. Chanda and S. Chandrasekaran, Chem.–Eur. J., 2012, 18, 12498 CrossRef CAS PubMed.
- X.-Q. Liu, H.-F. Yue, J.-Q. Jia, L. Guo and M. Rueping, Chem.–Eur. J., 2017, 23, 11771 CrossRef CAS PubMed.
-
(a) J.-H. Xu, Q. Jiang and C.-C. Guo, J. Org. Chem., 2013, 78, 11881 CrossRef CAS PubMed;
(b) Q. Jiang, B. Xu, A. Zhao, J. Jia, T. Liu and C.-C. Guo, J. Org. Chem., 2014, 79, 8750 CrossRef CAS PubMed;
(c) Q. Jiang, J.-Y. Wang and C.-C. Guo, J. Org. Chem., 2014, 79, 8768 CrossRef CAS PubMed;
(d) M.-Z. Zhang, Q.-H. Guo, W.-B. Sheng and C.-C. Guo, Adv. Synth. Catal., 2015, 357, 2855 CrossRef CAS;
(e) B. Xu, Q. Jiang, A. Zhao, J. Jia, Q. Liu, W.-P. Luo and C.-C. Guo, Chem. Commun., 2015, 51, 11264 RSC;
(f) Y.-F. Liu, Y.-Q. Hu, Z.-Z. Cao, X. Zhan, W.-P. Luo, Q. Liu and C.-C. Guo, Adv. Synth. Catal., 2018, 360, 2691 CrossRef CAS.
-
(a) W. Wei, Y. Shao, H.-Y. Hu, F. Zhang, C. Zhang, Y. Xu and X.-B. Wan, J. Org. Chem., 2012, 77, 7157 CrossRef CAS PubMed;
(b) W.-P. Mai, H.-H. Wang, Z.-C. Li, J.-W. Yuan, Y.-M. Xiao, L.-R. Yang, P. Mao and L.-B. Qu, Chem. Commun., 2012, 48, 10117 CAS;
(c) J. Zhang, Y. Wei, S.-X. Lin, F.-S. Liang and P.-J. Liu, Org. Biomol. Chem., 2012, 10, 9237 RSC;
(d) Q. Zhao, T. Miao, X.-B. Zhang, W. Zhou and L. Wang, Org. Biomol. Chem., 2013, 11, 1867 RSC;
(e) H.-L. Huang, G.-Q. Yuan, X.-W. Li and H.-F. Jiang, Tetrahedron Lett., 2013, 54, 7156 CrossRef CAS;
(f) X. Wu, Q.-H. Gao, S. Liu and A.-X. Wu, Org. Lett., 2014, 16, 2888 CrossRef CAS PubMed;
(g) W.-B. Liu, S.-S. Xu, C. Chen and Z.-B. Zhu, ChemistrySelect, 2016, 3, 612 CrossRef;
(h) D. Wang, K. Zhang, L.-H. Jia, D.-T. Zhang, Y. Zhang and Y.-J. Cheng, Org. Biomol. Chem., 2017, 15, 3427 CAS.
-
(a) J. Muzart, Tetrahedron, 2009, 65, 8313 CrossRef CAS;
(b) S. H. Cho, J. Y. Kim, S. Y. Lee and S. Chang, Angew. Chem., Int. Ed., 2009, 48, 9127 CAS;
(c) J. Wang, J.-T. Hou, J. Wen, J. Zhang and X.-Q. Yu, Chem. Commun., 2011, 47, 3652 RSC;
(d) Y.-M. Li, Y.-S. Xie, R. Zhang, K. Jin, X.-N. Wang and C.-Y. Duan, J. Org. Chem., 2011, 76, 5444 CrossRef CAS PubMed;
(e) S.-T. Ding and N. Jiao, Angew. Chem., Int. Ed., 2012, 51, 9226 CrossRef CAS PubMed;
(f) H. Wang, L.-N. Guo and X.-H. Duan, Org. Biomol. Chem., 2013, 11, 4573 RSC;
(g) B.-N. Du, B. Jin and P.-P. Sun, Org. Biomol. Chem., 2014, 12, 4586 RSC;
(h) W.-B. Liu, C. Chen and H.-L. Liu, Beilstein J. Org. Chem., 2015, 11, 1721 CrossRef CAS PubMed;
(i) P. B. Thale, P. N. Borase and G. S. Shankarling, RSC Adv., 2016, 6, 52724 RSC;
(j) W.-B. Liu, C. Chen and P. Zhou, ChemistrySelect, 2017, 2, 5532 CrossRef CAS.
-
(a) H.-Z. Li, W.-J. Xue and A.-X. Wu, Tetrahedron, 2014, 70, 4645 CrossRef CAS;
(b) N. Mupparapu, S. Khan, S. Battula, M. Kushwaha, A. P. Gupta, Q. N. Ahmed and R. A. Vishwakarma, Org. Lett., 2014, 16, 1152 CrossRef CAS PubMed;
(c) A. K. Padala, N. Mupparapu, D. Singh, R. A. Vishwakarma and Q. N. Ahmed, Eur. J. Org. Chem., 2015, 3577 CrossRef CAS;
(d) X. Wu, Q.-H. Gao, X. Geng, J.-J. Zhang, Y.-D. Wu and A.-X. Wu, Org. Lett., 2016, 18, 2507 CrossRef CAS PubMed;
(e) P. Natarajan, Manjeet, N. Kumar, S. Devi and K. Mer, Tetrahedron Lett., 2017, 58, 658 CrossRef CAS.
-
(a) W. Hub, S. Schneider, F. Doerr, J. D. Oxman and F. D. Lewis, J. Am. Chem. Soc., 1984, 106, 701 CrossRef CAS;
(b) J. C. Scaiano, S. Garcia and H. Garcla, Tetrahedron Lett., 1997, 38, 5929 CrossRef CAS;
(c) O. Brede, A. Maroz, R. Hermann and S. Naumov, J. Phys. Chem. A, 2005, 109, 8081 CAS;
(d) A. Grirrane, A. Corma and H. Garcia, Science, 2008, 322, 1661 CrossRef CAS PubMed;
(e) C. Zhang and N. Jiao, Angew. Chem., Int. Ed., 2010, 49, 6174 CrossRef CAS PubMed;
(f) X.-Y. Chen, X.-Y. Wang, Y.-X. Sui, Y.-Z. Li, J. Ma, J.-L. Zuo and X.-P. Wang, Angew. Chem., Int. Ed., 2012, 51, 11878 CrossRef CAS PubMed;
(g) X.-Y. Chen, X.-Y. Wang, Y.-X. Sui, Y.-Z. Li, J. Ma, J.-L. Zuo and X.-P. Wang, Angew. Chem., Int. Ed., 2014, 53, 943 CAS;
(h) M. R. Talipov, J. S. Hewage, S. V. Lindeman, J. R. Gardinier and R. Rathore, Angew. Chem., Int. Ed., 2014, 53, 938 CASP. Nalawade, S. Naumov and S. Kapoor, J. Phys. Org. Chem., 2015, 28, 2 CrossRef CAS;
(i) S.-W. Zhang, W.-Q. Wang, S. Liu, Y.-X. Sui, Z.-C. Zhang, G.-W. Tan, Q. Sun and X.-P. Wan, Sci. China: Chem., 2017, 60, 1439, DOI:10.1007/s11426-017-9096-7.
- D. Wang, K. Zhang, L.-H. Jia, D.-T. Zhang, Y. Zhang, Y.-J. Cheng, C. Lin and B. Wang, Org. Biomol. Chem., 2017, 15, 3427 RSC.
-
(a) Z.-J. Liu, J. Zhang, S.-L. Chen, E.-B. Shi, Y. Xu and X.-B. Wan, Angew. Chem., Int. Ed., 2012, 51, 3231 CrossRef CAS PubMed;
(b) L. Y. M. Eymann, A. G. Tskhovrebov, A. Sienkiewicz, J. L. Bila, I. Živković, H. M. Rønnow, M. D. Wodrich, L. Vannay, C. Corminboeuf, P. Pattison, E. Solari, R. Scopelliti and K. Severin, J. Am. Chem. Soc., 2016, 138, 15126 CrossRef CAS PubMed;
(c) L.-W. Zheng, M. Griesser, D. A. Pratt and M. M. Greenberg, J. Org. Chem., 2017, 82, 3571 CAS;
(d) D. Shimizu, K. Furukawa and A. Osuka, Angew. Chem., Int. Ed., 2017, 56, 7435 CrossRef CAS PubMed.
Footnote |
† Electronic supplementary information (ESI) available. See DOI: 10.1039/c9ra00616h |
|
This journal is © The Royal Society of Chemistry 2019 |
Click here to see how this site uses Cookies. View our privacy policy here.