DOI:
10.1039/C9RA00669A
(Paper)
RSC Adv., 2019,
9, 11406-11412
Blond and blood juice supplementation in high fat diet fed mice: effect on antioxidant status and DDAH/ADMA pathway†
Received
25th January 2019
, Accepted 5th April 2019
First published on 11th April 2019
Abstract
Non-alcoholic fatty liver disease (NAFLD) is the most common liver disease spread throughout the world. The most frequent causes of death in NAFLD patients are due both to liver and cardiovascular damage. Several pathways, including the dimethylarginine dimethylaminohydrolase (DDAH)/asymmetric dimethylarginine (ADMA) pathway, are involved in the pathogenesis of NAFLD. It has been reported that ADMA plasmatic levels are increased in patients with hepatic dysfunction such as NAFLD. Although many studies demonstrated that some foods are effective in the treatment of NAFLD, few studies have evaluated their effects with respect to the prevention of the disease. It has been reported that sweet orange juice (OJ) consumption may be associated with potential health benefits. However, some varieties of sweet orange are more effective than others. The aim of the present paper was to investigate the effect of blond and blood sweet orange juice in prevention of NAFLD by evaluating its ability to improve liver steatosis in mice with diet-induced obesity, reducing oxidative stress and affecting the DDAH/ADMA pathway. Results obtained in our experimental conditions evidenced that blood orange juice rather than blond orange juice was more effective. Blood orange juice or blond orange juice enriched in anthocyanins may represent a promising dietary option for the prevention of fatty liver disease.
Introduction
Non-alcoholic fatty liver disease (NAFLD) is the most common liver disease spread throughout the world.1,2 NAFLD mainly affects the western population because of their more sedentary lifestyle and dietary habits.3,4 Insulin resistance is a common pathophysiological factor for many pathologies such as dyslipidemia, diabetes, metabolic syndrome and NAFLD.5–7 In hepatocytes of patients with NAFLD are accumulated high levels of triglycerides and consequently NAFLD can progress to steatosis or to non-alcoholic steatohepatitis (NASH) which are characterized by increased oxidative stress and inflammation. NASH in turn can lead to cirrhosis or to related complications, such as hepatocellular carcinoma. The most frequent causes of death in NAFLD patients are due both to liver and cardiovascular damage.3,8 Endothelial dysfunction is a main event in the pathogenetic cascade leading to cardiovascular damage. Asymmetric dimethylarginine (ADMA) is an endogenous inhibitor of nitric oxide synthase (NOS), which plays a key role in endothelial dysfunction.9 Several pathways, including the DDAH/ADMA pathway, are involved in the pathogenesis of NAFLD. It has been reported that ADMA plasmatic levels are increased in patients with hepatic dysfunction such as NAFLD.2,10 Although many studies demonstrated that some foods are effective in the treatment of NAFLD,11–15 few studies have evaluated their effects with respect to the prevention of the disease.16 The sweet orange (Citrus sinensis L. Osbeck) can have a wide range of beneficial effects on health.17,18 Sweet orange juice contains potent bioactive compounds including flavonoids (hesperidin and narirutin, predominantly as glycosides), carotenoids (xanthophylls and carotenes), vitamin C and other beneficial phytochemicals. It has been reported that sweet orange juice (OJ) consumption may be associated with potential health benefits. However, the different varieties of sweet oranges do not have the same effects. For example, among the two varieties of sweet orange Moro (a blood orange) and Navelina (a blond orange), only the dietary supplementation of sweet orange Moro juice, but not of sweet orange Navelina juice, significantly reduced body weight gain and fat accumulation in mice. This different effect may be due to different polyphenols content. Fruit juice analysis of the varieties sweet orange Moro and Navelina fruits, evidenced that only Moro variety is rich in anthocyanins.19 Moreover, Salamone et al.20 demonstrated that blood orange consumption counteracts liver steatogenesis in mice fed with diet-induced obesity (HFD) and thus this fruit may represent a promising dietary option for the prevention of fatty liver. Aim of the present paper was to investigate the effect of blond and blood orange juice in prevention of NAFLD by evaluating their ability to improve liver steatosis in mice fed HFD reducing oxidative stress and affecting DDAH/ADMA pathway.
Results
Animals and diets
Food and beverage consumption was measured twice a week for the entire duration of the experiments (12 weeks). To evaluate the effect of blond and blood orange juice on food and liquid intake, C57/BL6 mice were provided food and either water or one of the varieties of orange juice ad libitum. Animals tolerated enough the replacement of drinking water with orange juice (100% orange juice, as the only drinking source). Under these conditions the beverage intake of water was 4.05 ± 0.80 ml per day per mouse, comparable to the daily beverage intake of the different orange juices, equal to 3.85 ± 1.05 ml per day per mouse. Despite the consumption of approximately 3.85 ± 1.05 ml per day of blood or blond orange juice compared with water corresponds to an excess of energy intake of around 1.5 kcal per day, none of the orange juices provided altered food consumption, as indicated by the daily food intake that was approximately 3.25 ± 0.85 g per day per mouse in all the experimental conditions examined.
Body weight and triglycerides content
All animals had similar body weight at the beginning of the experiment. After 12 weeks, mice fed HFD + water had higher body weight compared to SD group. Mice fed HFD + blond oranges had a weak reduction of body weight, whereas mice fed HFD + blood oranges had a body weight similar to mice fed SD (Fig. 1).
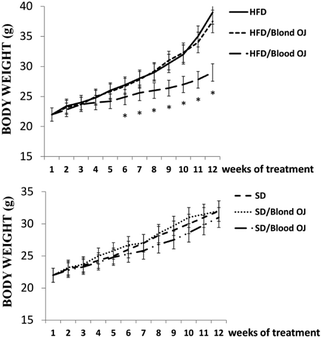 |
| Fig. 1 Effects of blond and blood sweet orange juice on body weight during the 12 weeks period. Body weight was markedly increased in mice fed high-fat diet (HFD) and was decreased to the levels of low fat diet mice by blood sweet orange juice. Values are mean ± standard deviation (S.D.) of at least three independent experiments. *p < 0.05 vs. HFD + water. | |
Mice fed HFD for 12 weeks had increased liver triglycerides levels compared to the SD group. Mice fed HFD + blond oranges had a weak reduction of triglycerides levels, whereas mice fed HFD + blood oranges had triglycerides levels similar to mice fed SD (Fig. 2). The triglyceride content is similar to changes in liver weight that is an important indicator for fatty liver. Liver weight was among 20–22 mg for mice fed with SD + water, SD + blond orange juice, SD + blood orange juice and for mice fed with HFD + blood orange juice, while it was among 25–30 mg for mice fed with HFD + water and for HFD + blond orange juice.
 |
| Fig. 2 Effects of blond and blood sweet orange juice on liver triglycerides content. Liver triglycerides content was markedly increased in mice fed high-fat diet (HFD) and was decreased to the levels of low fat diet mice by blood sweet orange juice. Values are mean ± standard deviation (S.D.) of at least three independent experiments. #p < 0.05 vs. standard diet (SD) + water; *p < 0.05 vs. HFD + water. | |
Lipid hydroperoxide levels
Mice fed HFD for 12 weeks had increased liver LOOH levels compared to the SD group. A weak reduction of LOOH levels was evidenced in mice fed HFD + blond oranges, whereas a significant reduction of LOOH levels was showed in mice fed HFD + blood oranges (Fig. 3).
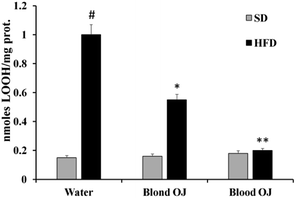 |
| Fig. 3 Effects of blond and blood sweet orange juice on liver lipid hydroperoxide (LOOH) levels. LOOH levels were markedly increased in mice fed high-fat diet (HFD), were mildly decreased to the levels of low fat diet mice by blond sweet orange juice and were significantly decreased to the levels of low fat diet mice by blood sweet orange juice. Values are mean ± standard deviation (S.D.) of at least three independent experiments. #p < 0.005 vs. standard diet (SD) + water; *p < 0.05 vs. HFD + water; **p < 0.005 vs. HFD + water. | |
Thiol group levels
Mice fed HFD for 12 weeks had decreased liver RSH levels compared to the SD group. Mice fed HFD + blond oranges had a weak increase of RSH levels, whereas mice fed HFD + blood oranges had RSH levels similar to mice fed SD (Fig. 4).
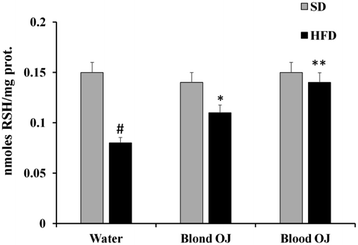 |
| Fig. 4 Effects of blond and blood sweet orange juice on liver non-proteic thiol group (RSH) levels. RSH levels were markedly decreased in mice fed high-fat diet (HFD), were mildly increased to the levels of low fat diet mice by blond sweet orange juice and were significantly increased to the levels of low fat diet mice by blood sweet orange juice. Values are mean ± standard deviation (S.D.) of at least three independent experiments. #p < 0.005 vs. standard diet (SD) + water; *p < 0.05 vs. HFD + water; **p < 0.005 vs. HFD + water. | |
Reactive oxygen species levels
Mice fed HFD for 12 weeks had increased liver ROS levels compared to the SD group. Even if decreased ROS levels were measured in mice fed HFD + blond oranges, in mice fed HFD + blood oranges ROS levels were significantly decreased and were similar to mice fed SD (Fig. 5).
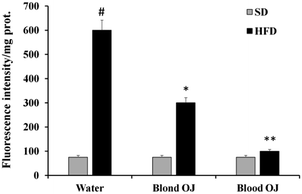 |
| Fig. 5 Effects of blond and blood sweet orange juice on liver reactive oxygen species (ROS) levels. ROS levels were markedly increased in mice fed high-fat diet (HFD), were mildly decreased to the levels of low fat diet mice by blond sweet orange juice and were significantly decreased to the levels of low fat diet mice by blood sweet orange juice; #p < 0.005 vs. standard diet (SD) + water; *p < 0.05 vs. HFD + water; **p < 0.005 vs. HFD + water. | |
SREBP-1c and DDAH-1 proteins levels
Mice fed HFD for 12 weeks had increased liver SREBP-1c expression compared to the SD group. Mice fed HFD + blond oranges had SREBP-1c expression similar to mice fed HFD + water, whereas mice fed HFD + blood oranges had SREBP-1c expression similar to mice fed SD (Fig. 6).
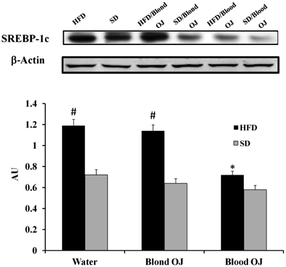 |
| Fig. 6 Effects of blond and blood sweet orange juice on liver sterol response element binding protein-1c (SREBP-1c) expression. SREBP-1c expression was markedly increased in mice fed high-fat diet (HFD) and was significantly decreased to the levels of low fat diet mice by blood sweet orange juice. Values are mean ± standard deviation (S.D.) of at least three independent experiments. #p < 0.05 vs. standard diet (SD) + water; *p < 0.005 vs. HFD + water. | |
Mice fed HFD for 12 weeks had decreased liver DDAH-1 expression compared to the SD group. Mice fed HFD + blond oranges had DDAH-1 expression similar to mice fed HFD + water, whereas mice fed HFD + blood oranges had DDAH-1 expression similar to mice fed SD (Fig. 7).
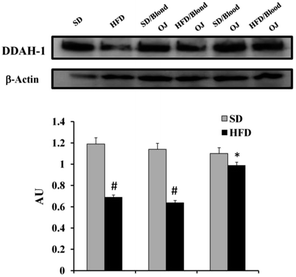 |
| Fig. 7 Effects of blond and blood sweet orange juice on liver dimethylarginine dimethylaminohydrolase-1 (DDAH-1) expression. DDAH-1 expression was markedly decreased in mice fed high-fat diet (HFD) and was significantly increased to the levels of low fat diet mice by blood sweet orange juice. Values are mean ± standard deviation (S.D.) of at least three independent experiments. #p < 0.05 vs. standard diet (SD) + water; *p < 0.05 vs. HFD + water. | |
Liver DDAH activity
Mice fed HFD for 12 weeks had decreased liver DDAH activity compared to the SD group. Mice fed HFD + blond oranges had a weak increase of DDAH activity, whereas mice fed HFD + blood oranges had DDAH activity similar to mice fed SD (Fig. 8).
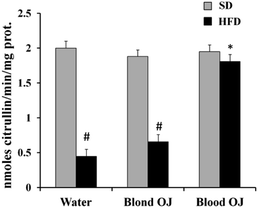 |
| Fig. 8 Effects of blond and blood sweet orange juice on liver dimethylarginine dimethylaminohydrolase-1 (DDAH-1) activity. Enzymatic activity was determined by measuring L-citrulline formation. DDAH-1 activity was markedly decreased in mice fed high-fat diet (HFD) and was significantly increased to the levels of low fat diet mice by blood sweet orange juice. Values are mean ± standard deviation (S.D.) of at least three independent experiments. #p < 0.05 vs. standard diet (SD) + water; *p < 0.05 vs. HFD + water. | |
Liver ADMA levels
Mice fed HFD for 12 weeks had increased liver ADMA levels compared to the SD group. Mice fed HFD + blond oranges had ADMA levels similar to mice fed HFD + water, whereas mice fed HFD + blood oranges had ADMA levels similar to mice fed SD (Fig. 9).
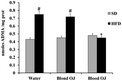 |
| Fig. 9 Effects of blond and blood sweet orange juice on liver asymmetric dimethylarginine (ADMA) levels. ADMA levels were markedly increased in mice fed high-fat diet (HFD) and was significantly decreased to the levels of low fat diet mice by blood sweet orange juice. Values are mean ± standard deviation (S.D.) of at least three independent experiments. #p < 0.005 vs. standard diet (SD) + water; *p < 0.05 vs. HFD + water. | |
Discussion
Asymmetric dimethylarginine (ADMA) is an endogenous inhibitor of nitric oxide synthase (NOS) that reduces nitric oxide (NO) levels23 with consequent increase of reactive oxygen species (ROS) production.24 Methylation of arginine residues by type I protein arginine methyltransferases (PRMTs; e.g., PRMT1 and PRMT3) and subsequent proteolysis leads to ADMA synthesis.25 ADMA is metabolized to citrulline and dimethylamine by two isoforms of dimethylarginine dimethylaminohydrolase (DDAH) namely DDAH-1 and DDAH-2. The liver is crucial to ADMA metabolism.26,27 In this organ, ADMA is mainly metabolized by isoform DDAH-1.28 Li et al.29 demonstrated in HepG2 cells that DDAH-1 deficiency and consequently ADMA increase, promote palmitic acid induced-steatosis and oxidative stress, whereas overexpression of DDAH-1 attenuated palmitic acid-induced steatosis and oxidative stress. Moreover, several in vivo studies report that increased plasma levels of ADMA are measured in patients with hepatic dysfunction, such as acute liver disease30 cirrhosis,31 NAFLD2,10 and alcoholic hepatitis.32 It has been reported that oxidative stress induced by HFD diet is responsible of increased levels of the transcription factor sterol response element binding protein-1c (SREBP1c). This transcription factor regulates the expression of genes involved in hepatic triglycerides synthesis and therefore it is implicated in the pathogenesis of NAFLD.33 Moreover, Ivashchenko et al. reported that SREBP1c is a transcriptional repressor of the DDAH-1 promoter and therefore it might be responsible of DDAH dysregulation occurring in NAFLD. Therefore, the regulation by SREBP-1c of the DDAH-ADMA system, may represent a potential link between cellular lipid increase and endothelial dysfunction observed in NAFLD.34 According to these evidences, Li et al.29 reported that ADMA/DDAH-1 pathway is involved in hepatic lipogenesis and steatosis induced by HFD feeding. It has been reported that natural compounds, such as epigallocatechin gallate (EGCG), a polyphenolic catechin, was able to attenuate endothelial dysfunction in diabetic models by decreasing ADMA level via increasing DDAH activity and that silibinin, a flavonolignan, markedly improved endothelial function in T2D mice by reducing circulating and vascular ADMA levels.35,36 In the present paper we investigated the effect of blond and blood orange juice in prevention of NAFLD by evaluating their ability to improve liver steatosis in mice fed HFD reducing oxidative stress and affecting DDAH/ADMA pathway. Results obtained in our experimental conditions evidenced that, blood oranges juice rather than blond oranges juice, was more effective in reducing body weight and liver triglycerides content in mice fed HFD. Moreover, a moderate antioxidant activity was observed in mice fed HFD + blond orange juice respect to mice fed HFD, while blood oranges juice was able to significantly decrease oxidative stress as demonstrated by reduction of ROS and LOOH levels and by increase of RSH levels in liver of mice fed HFD + blood orange respect to mice fed HFD. These data, according to Titta et al.19 may be explained by the different content in bioactive compounds present in two types of oranges juices, respectively blood and blond oranges juices, and by the high content in anthocyanins, particularly C3G, present in blood orange juice respect to blond orange juice. SREBP-1c downregulation and DDAH-1 upregulation with consequent ADMA increase was measured only in liver of mice fed HFD + blood orange juice respect to mice fed HFD. The ability of blood orange juice, but not of blond orange juice, to modulate SREBP-1c/DDAH-1 expressions may be due to its high content in anthocyanins. Suzuki et al. reported that anthocyanidins may decrease adipocyte differentiation through modulation of key adipocyte differentiation-associated marker SREBP-1c.37 Moreover, Jia et al.38 demonstrated that cyanidin is an agonistic ligand for liver X receptor alpha (LXRα) and beta (LXRβ) and reduces cellular lipid accumulation in hepatocytes. According to these data anthocyanidins present only in blood oranges, may be responsible of SREBP-1c reduction through their direct interaction with LXRα and LXRβ, and then through modulation of cellular LXR activity.
Experimental
Animals and treatments
All animal procedures were performed in accordance with the Guidelines for Care and Use of Laboratory Animals of “Catania University” and experiments were approved by the Animal Ethics Committee of “Ministry of Health (Directorate General for Animal Health and Veterinary Medicines) (Italy)”. Eight-week-old male C57BL6/J mice were purchased from Charles River Labs (Lecco, Italy). Animals were maintained in a temperature- and light-controlled facility for 12 weeks. Diets were obtained by Harlan Teklad (Madison, WI, USA). The standard diet (SD) provided 3.3 kcal g−1 with 60% carbohydrates, 23% proteins and 17% fat. The HFD provided 5.2 kcal g−1 with 60% fat, 20% proteins and 20% carbohydrates (for detailed diets composition see ESI†). Mice were distributed in six groups: group I included six mice fed SD and permitted ad libitum consumption of water (SD + water); group II included six mice fed SD and permitted ad libitum consumption of blond orange juice (SD + blond orange); group III included six mice fed SD and permitted ad libitum consumption of blood orange juice (SD + blood orange); group IV comprised six mice fed HFD and permitted ad libitum consumption water (HFD + water); group V comprised six mice fed HFD and permitted ad libitum consumption of blond orange juice instead of water (HFD + blond orange); group VI comprised six mice fed HFD and permitted ad libitum consumption of blood orange juice instead of water (HFD + blood orange). Fresh hand squeezed orange juice were obtained from ripe oranges, harvested in the Plain of Catania (Catania, Italy), of the Moro (a blood orange) and Navelina (a blond orange) varieties. Fruits were immediately stored at 4 °C and squeezed a few days later; the juice obtained was pre-filtered and stored at −20 °C in aliquots of 0.5 L. Every 2 d, frozen juice aliquots were thawed, filtered and put in the bottle of each cage. Food and beverage consumption was recorded twice weekly; body weight was recorded weekly. After sacrifice by CO2 asphyxiation, liver samples were obtained, processed and stored for further analysis. Livers (20–30 mg) were collected from mice supplied with Moro orange Juice, Navelina orange juice and water, and fed with a SD or HFD. Tissues were immediately frozen and stored at −80 °C.
Tissue processing
The frozen liver tissue was homogenized in nine volumes of cold PBS. Aliquots of homogenate were used to evaluate hepatic triglycerides content, ROS, RSH, LOOH and ADMA levels, DDAH enzyme activity assay, DDAH-1, and SREBP-1c expressions. Protein concentration was measured using TAKE 3 nanodrop.
Liver triglycerides content
Liver triglycerides content was measured using a serum/tissue triglyceride colorimetric kit (Biovision, Milpitas, CA, United States). Results are reported as mg triglycerides per g prot.
Determination of lipid hydroperoxide levels
Hepatic levels of lipid hydroperoxide were evaluated following the oxidation of Fe2+ to Fe3+ in the presence of xylenol orange at λ = 560 nm as previously described.21 Results are reported as nmol LOOH per mg prot.
Thiol group determination
Hepatic levels of thiol groups were measured, in 200 μl of liver homogenate, by using a spectrophotometric assay as previously described.21 Results are reported as nmol RSH per mg prot.
Reactive oxygen species determination
Determination of ROS was performed in samples of tissue homogenate by using a fluorescent probe 2′,7′-dichlorofluorescein diacetate (DCFH-DA) as previously described.22 The fluorescence [corresponding to the oxidized radical species 2′,7′-dichlorofluorescein (DCF)] was monitored spectrofluorometrically (excitation, λ = 488 nm; emission, λ = 525 nm). Results are reported as fluorescence intensity per mg protein.
Western blotting
Tissue homogenates (30 μg proteins) containing a protease-inhibitor cocktail were loaded onto 12% SDS-polyacrylamide (SDS-PAGE) gels and subjected to electrophoresis (120 V, 90 min). The separated proteins were transferred to nitrocellulose membranes (Bio-Rad, Hercules, CA, USA). After transfer, the blots were incubated with Li-COR blocking buffer for 1 h, followed by overnight incubation with a 1
:
1.000 dilution of primary antibodies directed against DDAH-1 [Calbiochem EMD Biosciences (Darmstadt, Germany)], SREPB1c (SantaCruz Biotechnology, Santa Cruz, CA, USA) and β-actin (Cell Signaling Technology, Inc., Danvers, MA, USA). After washing with TBS, the blots were incubated for 1 h with the secondary antibody (1
:
1.000). Protein detection was carried out using a secondary infrared fluorescent dye-conjugated antibody absorbing at λ 800 and λ 700 nm. The blots were visualized using an Odyssey Infrared imaging scanner (LI-COR Biosciences) and quantified by densitometric analysis performed after normalization with β-actin. Results are expressed as arbitrary units (A.U.).
DDAH activity assay
Hepatic homogenate was centrifuged at 5000g for 60 min at 4 °C and supernatants were collected for DDAH activity assay, performed by determining L-citrulline formation in 96-well microtiter plate, according to Knipp's method as previously described.21 Results are expressed as nmol citrulline per min per mg prot.
Liver ADMA
Liver ADMA concentration was determined by using a commercially available enzyme linked immunosorbent assay kit (DLD Diagnostika GmbH, Hamburg, Germany) according to the manufacturer's instructions. Results are expressed as nmol ADMA per mg prot.
Statistical analysis
Data are reported as mean ± standard deviation (S.D.) values of at least three independent experiments. The results were analysed for statistical significance using ANOVA. A p-value < 0.05 was considered as significant.
Conclusions
Our findings suggest that strategies to increase DDAH-1 expression/activity in hepatocytes may provide a novel approach to attenuate NAFLD development. Blood orange juice or blond orange juice enriched in anthocyanins may represent a promising dietary option for the prevention of fatty liver.
Conflicts of interest
There are no conflicts to declare.
References
- S. Milic and D. Stimac, Nonalcoholic fatty liver disease/steatohepatitis: epidemiology, pathogenesis, clinical presentation and treatment, Dig. Dis., 2012, 30, 158–162 CrossRef PubMed.
- S. Boga, H. Alkim, A. R. Koksal, M. Bayram, M. B. Ozguven, M. Ergun, S. T. Neijmann, G. Ozgon and C. Alkim, Increased Plasma Levels of Asymmetric Dimethylarginine in Nonalcoholic Fatty Liver Disease: Relation With Insulin Resistance, Inflammation, and Liver Histology, J. Invest. Med., 2015, 63, 871–877 CrossRef CAS PubMed.
- N. Rafiq, C. Bai, Y. Fang, M. Srishord, A. McCullough, T. Gramlich and Z. M. Younossi, Long-term follow-up of patients with nonalcoholic fatty liver, Clin. Gastroenterol. Hepatol., 2009, 7, 234–238 CrossRef PubMed.
- W. Nseir, E. Hellou and N. Assy, Role of diet and lifestyle changes in nonalcoholic fatty liver disease, World J. Gastroenterol., 2014, 20, 9338–9344 CAS.
- J. T. Haas, S. Francque and B. Staels, Pathophysiology and Mechanisms of Nonalcoholic Fatty Liver Disease, Annu. Rev. Physiol., 2016, 78, 181–205 CrossRef CAS PubMed.
- G. Marchesini, M. Brizi, A. M. Morselli-Labate, G. Bianchi, E. Bugianesi, A. J. McCullough, G. Forlani and N. Melchionda, Association of nonalcoholic fatty liver disease with insulin resistance, Am. J. Med., 1999, 107, 450–455 CrossRef CAS PubMed.
- R. Loomba, M. Abraham, A. Unalp, L. Wilson, J. Lavine, E. Doo, N. M. Bass and Nonalcoholic Steatohepatitis Clinical Research, Association between diabetes, family history of diabetes, and risk of nonalcoholic steatohepatitis and fibrosis, Hepatology, 2012, 56, 943–951 CrossRef PubMed.
- J. P. Ong, A. Pitts and Z. M. Younossi, Increased overall mortality and liver-related mortality in non-alcoholic fatty liver disease, J. Hepatol., 2008, 49, 608–612 CrossRef PubMed.
- J. S. Jin and L. G. D'Alecy, Central and peripheral effects of asymmetric dimethylarginine, an endogenous nitric oxide synthetase inhibitor, J. Cardiovasc. Pharmacol., 1996, 28, 439–446 CrossRef CAS PubMed.
- T. Dogru, H. Genc, S. Tapan, F. Aslan, C. N. Ercin, F. Ors, M. Kara, E. Sertoglu, Y. Karslioglu, S. Bagci, I. Kurt and A. Sonmez, Plasma fetuin-A is associated with endothelial dysfunction and subclinical atherosclerosis in subjects with nonalcoholic fatty liver disease, Clin. Endocrinol., 2013, 78, 712–717 CrossRef CAS PubMed.
- F. Askari, B. Rashidkhani and A. Hekmatdoost, Cinnamon may have therapeutic benefits on lipid profile, liver enzymes, insulin resistance, and high-sensitivity C-reactive protein in nonalcoholic fatty liver disease patients, Nutr. Res., 2014, 34, 143–148 CrossRef CAS PubMed.
- F. Faghihzadeh, P. Adibi and A. Hekmatdoost, The effects of resveratrol supplementation on cardiovascular risk factors in patients with non-alcoholic fatty liver disease: a randomised, double-blind, placebo-controlled study, Br. J. Nutr., 2015, 114, 796–803 CrossRef CAS PubMed.
- F. Faghihzadeh, P. Adibi, R. Rafiei and A. Hekmatdoost, Resveratrol supplementation improves inflammatory biomarkers in patients with nonalcoholic fatty liver disease, Nutr. Res., 2014, 34, 837–843 CrossRef CAS PubMed.
- A. Ghaemi, F. A. Taleban, A. Hekmatdoost, A. Rafiei, V. Hosseini, Z. Amiri, R. Homayounfar and H. Fakheri, How Much Weight Loss is Effective on Nonalcoholic Fatty Liver Disease?, Hepatitis Mon., 2013, 13, e15227 Search PubMed.
- A. Shavakhi, M. Minakari, H. Firouzian, R. Assali, A. Hekmatdoost and G. Ferns, Effect of a Probiotic and Metformin on Liver Aminotransferases in Non-alcoholic Steatohepatitis: A Double Blind Randomized Clinical Trial, Int. J. Prev. Med., 2013, 4, 531–537 Search PubMed.
- T. Eslamparast, S. Eghtesad, H. Poustchi and A. Hekmatdoost, Recent advances in dietary supplementation, in treating non-alcoholic fatty liver disease, World J. Hepatol., 2015, 7, 204–212 CrossRef PubMed.
- E. Tripoli, M. La Guardia, S. Giammanco, D. Di Majo and M. Giammanco, Citrus flavonoids: molecular structure, biological activity and nutritional properties: a review, Food Chem., 2007, 104, 466–479 CrossRef CAS.
- G. Pepe, F. Pagano, S. Adesso, E. Sommella, C. Ostacolo, M. Manfra, M. Chieppa, M. Sala, M. Russo, S. Marzocco and P. Campiglia, Bioavailable Citrus sinensis Extract: Polyphenolic Composition and Biological Activity, Molecules, 2017, 22, 623 CrossRef PubMed.
- L. Titta, M. Trinei, M. Stendardo, I. Berniakovich, K. Petroni, C. Tonelli, P. Riso, M. Porrini, S. Minucci, P. G. Pelicci, P. Rapisarda, G. Reforgiato Recupero and M. Giorgio, Blood orange juice inhibits fat accumulation in mice, Int. J. Obes., 2010, 34, 578–588 CrossRef CAS PubMed.
- F. Salamone, G. Li Volti, L. Titta, L. Puzzo, I. Barbagallo, F. La Delia, S. Zelber-Sagi, M. Malaguarnera, P. G. Pelicci, M. Giorgio and F. Galvano, Moro orange juice prevents fatty liver in mice, World J. Gastroenterol., 2012, 18, 3862–3868 CrossRef PubMed.
- R. Acquaviva, R. Lanteri, G. Li Destri, R. Caltabiano, L. Vanella, S. Lanzafame, A. Di Cataldo, G. Li Volti and C. Di Giacomo, Beneficial effects of rutin and L-arginine coadministration in a rat model of liver ischemia-reperfusion injury, Am. J. Physiol. Gastrointest. Liver Physiol., 2009, 296, G664–G670 CrossRef CAS PubMed.
- V. Pittala, L. Vanella, L. Salerno, C. Di Giacomo, R. Acquaviva, M. Raffaele, G. Romeo, M. N. Modica, O. Prezzavento and V. Sorrenti, Novel Caffeic Acid Phenethyl Ester (Cape) Analogues as Inducers of Heme Oxygenase-1, Curr. Pharm. Des., 2017, 23, 2657–2664 CrossRef CAS PubMed.
- P. Vallance, A. Leone, A. Calver, J. Collier and S. Moncada, Accumulation of an endogenous inhibitor of nitric oxide synthesis in chronic renal failure, Lancet, 1992, 339, 572–575 CrossRef CAS.
- L. J. Druhan, S. P. Forbes, A. J. Pope, C. A. Chen, J. L. Zweier and A. J. Cardounel, Regulation of eNOS-derived superoxide by endogenous methylarginines, Biochemistry, 2008, 47, 7256–7263 CrossRef CAS PubMed.
- J. Najbauer, B. A. Johnson, A. L. Young and D. W. Aswad, Peptides with sequences similar to glycine, arginine-rich motifs in proteins interacting with RNA are efficiently recognized by methyltransferase(s) modifying arginine in numerous proteins, J. Biol. Chem., 1993, 268, 10501–10509 CAS.
- A. Ferrigno, L. G. Di Pasqua, C. Berardo, P. Richelmi and M. Vairetti, Liver plays a central role in asymmetric dimethylarginine-mediated organ injury, World J. Gastroenterol., 2015, 21, 5131–5137 CrossRef CAS PubMed.
- T. Hu, M. Chouinard, A. L. Cox, P. Sipes, M. Marcelo, J. Ficorilli, S. Li, H. Gao, T. P. Ryan, M. D. Michael and L. F. Michael, Farnesoid X receptor agonist reduces serum asymmetric dimethylarginine levels through hepatic dimethylarginine dimethylaminohydrolase-1 gene regulation, J. Biol. Chem., 2006, 281, 39831–39838 CrossRef CAS PubMed.
- X. Hu, D. Atzler, X. Xu, P. Zhang, H. Guo, Z. Lu, J. Fassett, E. Schwedhelm, R. H. Boger, R. J. Bache and Y. Chen, Dimethylarginine dimethylaminohydrolase-1 is the critical enzyme for degrading the cardiovascular
risk factor asymmetrical dimethylarginine, Arterioscler., Thromb., Vasc. Biol., 2011, 31, 1540–1546 CrossRef CAS PubMed.
- T. Li, R. Feng, C. Zhao, Y. Wang, J. Wang, S. Liu, J. Cao, H. Wang, T. Wang, Y. Guo and Z. Lu, Dimethylarginine Dimethylaminohydrolase 1 Protects Against High-Fat Diet-Induced Hepatic Steatosis and Insulin Resistance in Mice, Antioxid. Redox Signaling, 2017, 26, 598–609 CrossRef CAS PubMed.
- R. P. Mookerjee, R. N. Dalton, N. A. Davies, S. J. Hodges, C. Turner, R. Williams and R. Jalan, Inflammation is an important determinant of levels of the endogenous nitric oxide synthase inhibitor asymmetric dimethylarginine (ADMA) in acute liver failure, Liver Transpl., 2007, 13, 400–405 CrossRef PubMed.
- P. Lluch, B. Torondel, P. Medina, G. Segarra, J. A. Del Olmo, M. A. Serra and J. M. Rodrigo, Plasma concentrations of nitric oxide and asymmetric dimethylarginine in human alcoholic cirrhosis, J. Hepatol., 2004, 41, 55–59 CrossRef CAS PubMed.
- R. P. Mookerjee, M. Malaki, N. A. Davies, S. J. Hodges, R. N. Dalton, C. Turner, S. Sen, R. Williams, J. Leiper, P. Vallance and R. Jalan, Increasing dimethylarginine levels are associated with adverse clinical outcome in severe alcoholic hepatitis, Hepatology, 2007, 45, 62–71 CrossRef CAS PubMed.
- M. Aragno, C. E. Tomasinelli, I. Vercellinatto, M. G. Catalano, M. Collino, R. Fantozzi, O. Danni and G. Boccuzzi, SREBP-1c in nonalcoholic fatty liver disease induced by Western-type high-fat diet plus fructose in rats, Free Radical Biol. Med., 2009, 47, 1067–1074 CrossRef CAS PubMed.
- C. Y. Ivashchenko, B. T. Bradley, Z. Ao, J. Leiper, P. Vallance and D. G. Johns, Regulation of the ADMA-DDAH system in endothelial cells: a novel mechanism for the sterol response element binding proteins, SREBP1c and -2, Am. J. Physiol. Heart Circ. Physiol., 2010, 298, H251–H258 CrossRef CAS PubMed.
- W. J. Tang, C. P. Hu, M. F. Chen, P. Y. Deng and Y. J. Li, Epigallocatechin gallate preserves endothelial function by reducing the endogenous nitric oxide synthase inhibitor level, Can. J. Physiol. Pharmacol., 2006, 84, 163–171 CrossRef CAS PubMed.
- G. Li Volti, S. Salomone, V. Sorrenti, A. Mangiameli, V. Urso, I. Siarkos, F. Galvano and F. Salamone, Effect of silibinin on endothelial dysfunction and ADMA levels in obese diabetic mice, Cardiovasc. Diabetol., 2011, 10, 62 CrossRef CAS PubMed.
- R. Suzuki, M. Tanaka, M. Takanashi, A. Hussain, B. Yuan, H. Toyoda and M. Kuroda, Anthocyanidins-enriched bilberry extracts inhibit 3T3-L1 adipocyte differentiation via the insulin pathway, Nutr. Metab., 2011, 8, 14 CrossRef CAS PubMed.
- Y. Jia, M. H. Hoang, H. J. Jun, J. H. Lee and S. J. Lee, Cyanidin, a natural flavonoid, is an agonistic ligand for liver X receptor alpha and beta and reduces cellular lipid accumulation in macrophages and hepatocytes, Bioorg. Med. Chem. Lett., 2013, 23, 4185–4190 CrossRef CAS PubMed.
Footnote |
† Electronic supplementary information (ESI) available. See DOI: 10.1039/c9ra00669a |
|
This journal is © The Royal Society of Chemistry 2019 |
Click here to see how this site uses Cookies. View our privacy policy here.