DOI:
10.1039/C9RA01988J
(Paper)
RSC Adv., 2019,
9, 17726-17736
A novel method for textile odor removal using engineered water nanostructures†
Received
15th March 2019
, Accepted 15th May 2019
First published on 5th June 2019
Abstract
The malodor attached to textiles not only causes indoor environmental pollution but also endangers people's health even at low concentrations. Existing technologies cannot effectively eliminate the odor. Herein, an effective and environmentally friendly technology was proposed to address this challenging issue. This technology utilizes electrospraying process to produce Engineered Water Nanostructures (EWNS) in a controllable manner. Upon application of a high voltage to the Taylor cone, EWNS can be generated from the condensed vapor water through a Peltier element. Smoking, cooking and perspiration, considered the typical indoor malodorous gases emitted from human activities, were studied in this paper. A headspace SPME method in conjunction with GC-MS was employed for the extraction, detection and quantification of any odor residues. Results indicated that EWNS played a significant role in the deodorization process with removal efficiencies for the three odors were 95.3 ± 0.1%, 100.0 ± 0.0% and 43.7 ± 2.3%, respectively. The Reactive Oxygen Species (ROS) contained in the EWNS, mainly hydroxyl (OH˙) and superoxide radicals
are the possible mechanisms for the odor removal. These ROS are strong oxidative and highly reactive and have the ability to convert odorous compounds to non-odorous compounds through various chemical reaction mechanisms. This study showed clearly the potential of the proposed method in the field of odor removal and can be applied in the battle against indoor air pollution.
1. Introduction
Terrible indoor air quality in office buildings, public places and residential dwellings can be potentially harmful to human health even at very lower concentrations.1–4 As a common odor carrier,5 textile tends to absorb odors arising from various sources (e.g. body odor, tobacco, food aromas).6 Once absorbed, the odors in textiles can become more permanent upon exposure to heat and time, which means that the odor is likely to resurface with repeated wears and laundering.7 The retained residual odor in textiles can cause the occurrence of indoor air pollution, and potentially harms to the human body.1–3 For example, third-handed smoke can enter houses through clothes and be released again, resulting in a secondary pollution.8–10 And according to the report,7,11 there are more than 71% of people that have been plagued by odor problems in their clothing. Therefore, removing odors on textiles is an urgent task to maintain a safe and healthy life.
So far, several methods have been developed to remove malodors from the textiles, such as absorption/adsorption of odorous volatiles,12–16 hot water or steam extraction,17–21 and masking unpleasant odors through adding a fragrance-carrying compound to the surface or to the air.22–25 However, these approaches only demonstrated limited efficacy.22 Firstly, the malodors absorbed by the powered activated carbon or cyclodextrins can be released back into the room due to saturation or an elevated temperature.16 The malodors may still be perceived in clothing despite laundering or steam extracting.18,26 The high costs and non-reusability of sorptive substances, the consumption of large amounts of water, electricity and various chemical detergents during the washing process undoubtedly call for more advanced solutions. Secondly, certain repeated cleaning techniques such as laundering or steam extraction can result in the deterioration of fabrics and thus shorten the life of textiles.19 For large-scale home textile products such as carpets and curtains, washing and cleaning is not an easy task. So removing odors by laundering is not an ultimate solution. What is more, rather than eliminating odors, some techniques only mask the odors with fragrance. Fragrances associated with scented powders or sprays provide temporary pleasant smells to the textiles in which they are used, but the malodors are again apparent once the fragrance disperses. Furthermore, their high fragrance or perfume content may aggravate the allergies of some users.22
Nanotechnology may provide a better choice to address this issue.20 Among them, an Engineered Water Nanostructures (EWNS) method, which is based on the conversion of atmospheric water vapor into EWNS by electrospraying, has attracted much attention recently. The EWNS technology was firstly reported by the Panasonic Group and the Harvard University in 2012. In their previous studies, EWNS was primarily used to inactivate pathogens (different types of bacteria)27–30 and food safety controls31–33 and has been successfully applied in commerce. Later, some Chinese scholars from Tongji University developed a water ion device which is similar to the EWNS technology and applied it in the air purification area.34,35 But cigarette was the only purification object in their research. It has been revealed that EWNS possess a unique set of physical and biological properties, e.g. limited average nanoscale size, extended lifetime, highly reactive (due to more Reactive Oxygen Species (ROS) produced) and strong surface charge.36 The ROS contained in the EWNS, primarily hydroxyl (OH˙) and superoxide radicals
are regarded as the major antimicrobial species that can destruct the outer microbial cell envelope.37 As ROS are molecules containing an oxygen atom with an unpaired electron in its outer shell, they are very unstable and tend to donate single electrons or steal electrons from neighboring molecules. Although ROS has been report to cause oxidative stress in cells,38,39 Pyrgiotakis et al.40 found that EWNS showed no significant adverse health effects through an inhalation toxicological study which was performed by using an animal model. And this may be because when EWNS interact with alveolar or airway fluid, the ROS contents get neutralized by the organic molecules before they contact with epithelial cells located under the lining fluid. Therefore, although the EWNS contain ROS, they do not cause any lung damage or inflammation.40 However, the application of ROS is limited by their extremely short lifetime,41,42 e.g. 10−9 seconds for OH˙43–45 and 10−5 seconds for
45 But when wrapped in the EWNS, the ROS lifespan have been reported to be extended to more than 38 min,30 in which case the ROS efficiency is improved greatly and the scope of application becomes expanded.
Based on this background, for the first time we applied the promising EWNS technology for the textile malodor removal. An EWNS experiment platform has been established to implement the odor removal experiments, and the solid-phase micro extraction (SPME) followed by Gas Chromatography-Mass Spectrometry (GC-MS) was applied for the detection and quantification of odor residues before and after EWNS treatment. Promising results suggest that the EWNS showed a great efficiency in the odor removal of textiles and has the potential to be used in the battle against indoor air pollution.
2. Materials and methods
2.1 EWNS synthesis
EWNS were synthesized based on an electrospraying technique,46 which divides liquid into fairly uniform fragments, ranging from few nanometers to hundred microns.47 Herein, water vapor from the air were condensed on an electrode cooled by a Peltier element. Above the electrode, a counter electrode was arranged concentrically. High voltage was applied between the two electrodes (condensing electrode, −5 kV, grounded counter electrodes) to form a Taylor cone due to the electrical shear stress. The capillary is placed at a negative voltage while the counter electrode is connected to a positive voltage. At this point, the liquid jet contained lots of negative ions. Subsequently, since the Coulomb repulsion instability generated by the ions was greater than the surface tension, the liquid jet continued to be dispersed into fine droplets.27,48–50 As the droplets volume decreased, the charge density of the droplets exceeded the limit of surface tension, causing these droplets spontaneously split, eventually reaching a stable radius called Rayleigh critical radius51 and producing EWNS. The generation process of EWNS is illustrated in Fig. 1.
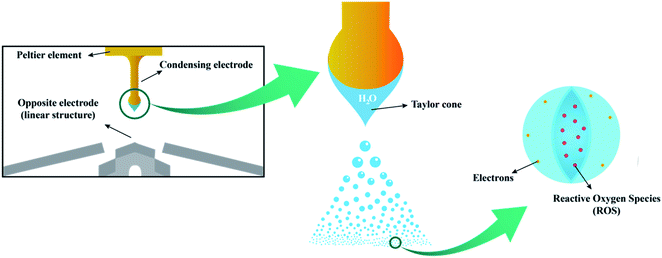 |
| Fig. 1 The generation of EWNS. | |
During this electrospray process, some water molecules and oxygen molecules were split or lost electrons under high electric field creating several kinds of ROS, like OH˙ and
52,53 It has been proven that those ROS are encapsulated in EWNS, which prevents them from neutralization by other air molecules and extends significantly their lifetime.54 Detailed information on the EWNS synthesis can be found in the series paper of the Harvard group.27–29,40 Unlike the previous studies by the Harvard group, in this study, a novel EWNS generator was employed with a new type of linear structure electrode developed by the Panasonic Corporation. It can increase significantly the discharging area and produces increased concentrations of ROS per unit of time.
2.2 ROS characterization of EWNS
Electron Spin Resonance (ESR) spin trapping was applied to examine the existence of short-lived free radical intermediates in the EWNS. And 5,5-dimethyl-L-pyrroline-N-oxide (DMPO), a widely-used spin-trapping agent55 was used for the detection of radicals. DMPO was obtained from Dojindo laboratories (Kumamoto, Japan). The detection of OH˙ were carried out on a Bruker EMX plus equipment (Brucker Instruments Inc., Billerica, MA, USA) operating with a spectrometer and a flat cell assembly. The DMPO solution with concentration of 0.22 M were used in this process. For detecting
a JEOL-FA200 ESR spectrometer equipment (Akishima, Japan) was used with a DMPO–ethanol solution (30 μL of DMPO was dissolved in 1 mL of ethanol). The DMPO solutions were all poured into glass dishes and placed 5 cm below the EWNS generator for a period of 20 minutes. The EWNS ejected from the generator react with DMPO to form intermediate products, thereby indirectly measuring the presence and content of OH˙ and
2.3 Odor removal experiment
2.3.1 Specimens preparation. Smoking, cooking and perspiration are considered as typical indoor malodorous gases produced from human behaviors.56 Therefore, to investigate the removal efficiency of EWNS on real odors, the smells of cigarette smoke, hot pot and human sweat were taken as representative odors. The final products used in the experiment and their selection basis are listed in Table 1.
Table 1 Final products used in the odor removal experiment
Odor type |
Final product |
Selection reference |
Smoking |
HONGTASHAN classic cigarette |
GB/T 18801—2015 air cleaner61 |
APIAC/LM 01—2015 indoor air cleaner's purification performance evaluation requirements |
Cooking |
HAIDILAO clear soup hot pot bottom material |
Top 1 Chinese hot pot brand |
Jingdong's best-selling product in hot pot bottom material category |
Perspiration |
20 g of isovaleric acid dissolving into 1 L of ethanol |
ISO 17299-3 2014 Textile – determination of deodorant property – part 3: Gas chromatography method62 |
Timo R. Hammer (2013)63 |
Chris Callewaert (2014)64 |
Natural fibers easily absorb odors due to their absorbent properties.57,58 Cotton is by far the most common natural fibers in the global apparel market.6 In this study, a cotton terry fabric (bottom warp yarns 32 s, upper and lower loops 21 s, no crepe, gram weight 85 g m−2) was used as substrate for uptake of the odor. The reason for using a towel structure fabric is that this structure gives a tremendous surface area per unit volume,59 making it suitable to uptake the odor emitted by the sample odors. In all cases, fabric stripes of 2 cm width and 5 cm length were used as samples, pre-washed 3 times according to the standard procedures,60 and conditioned (temperature 20 ± 2 °C, humidity 65 ± 5%) for at least 24 h before any experiment.
2.3.2 Odor analysis method. The fabrics were put into the 10 mL headspace vials bought from ANPEL Laboratory Technologies Inc. for the odor concentration test. The SPME program consisted of the extraction at 80 °C for 30 min and desorption for 1 min. The GC-MS experiments were performed using a QP-2010 DC-MS instrument (Shimadzu, Kyoto, Japan) equipped with an DB-5MS capillary column (30 m long, 0.25 mm id, 0.25 μm film thickness; Agilent Technologies, Santa Clara, CA, USA) and the NIST 11 mass spectra library. PAL Cycle Composer Control software (CTC Analytics, Zwingen, Switzerland) was used to control the GC-MS instrument and to acquire and process the data. Unless otherwise stated, the GC conditions are as follows. The injector temperature was 250 °C and the carrier gas (helium, 99.999%) was used at a constant flow rate of 1.0 mL min−1. The GC oven temperature was 40 °C for 2 min, increased at 15 °C min−1 to 280 °C, which was held for 5 min. The mass spectrometer was operated in an electron-impact mode. The scan range was 29–500 m/z with a rate of 0.3 s per scan. The temperature at ionization source and interface were 200 and 280 °C, respectively.
2.3.3 Experimental procedures. The deodorization efficiency of EWNS was evaluated in this study. The experimental group and the control group were comparatively studied. The experimental group indicate that the odor was treated by the EWNS, while the control group was only under the condition of natural attenuation. The treatment time was set to 1 h, 3 h and 5 h and each experiment was conducted in triplicate.For the smoke removal experiment, all fabric strips were firstly hung in a closed square acrylic box (50 cm × 50 cm × 50 cm) to absorb these smoke (as shown in Fig. 2). These strips were evenly distributed in order to absorb the same amount of the odor. The distance between the left and right sides of each strip was 4.25 cm, and the distance between the front and back rows was about 16.6 cm. And the bottom of the strip is approximately 32.5 cm from the bottom of the cabinet. Three cigarettes were used in the experiment to amplify the results and the smoke sorption time was set to 15 min. A small direct-current fan was placed in the edge of the box to spread the smoke. The air volume of the fan was not too large to blow the fabric stripes. To determine the initial smoke concentration of each fabric in the box, we randomly selected strips at 10 locations for analysis. Odor concentrations were tested by the GC-MS and the one-way ANOVA method was used with SPSS software.65,66 The results showed that under the experimental condition, no significant correlation between the smoke concentration absorbed by the strip and the position was observed (as shown in Table 2). Hence, the amount of initial smoke absorbed by the strips at different locations was very similar and can be considered as identical. As for the cooking smell removal experiment, the fabrics were prepared in a similar way. For the perspiration smell experiment, the preparation of the original fabrics was implemented according to ISO 17299-3.62
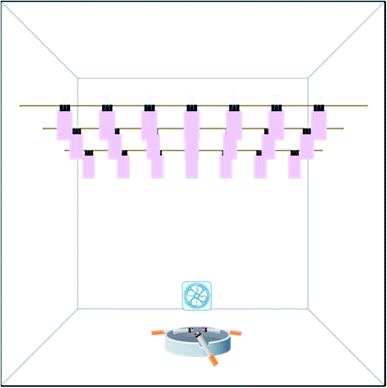 |
| Fig. 2 Schematic diagram of the box to absorb the smoke smell. | |
Table 2 The one-way ANOVA result (initial smoke concentration).
Source of variation |
Sum of squares |
df |
Mean square |
F |
Sig. |
Between groups |
1.979 × 1013 |
9 |
2.199 × 1012 |
0.839 |
0.599 |
Within groups |
2.622 × 1013 |
10 |
2.622 × 1012 |
|
|
Total |
4.601 × 1013 |
19 |
|
|
|
After each initial treatment, three fabric strips were taken out and put into the headspace vials for the GC-MS measurement. Three pieces of fabric were used to mark the initial smoke concentration value. The other 18 strips were placed into two identical semi-closed cabinets (23 cm × 10 cm × 15 cm) for the EWNS treatment or the control experiments. In each cabinet, 9 samples were laid flat at equal intervals in the 3 × 3 arrangement at the bottom. The EWNS generator was placed in the middle of the box, 13 cm from the bottom. The two group experiments were carried out at a temperature of 20 ± 2 °C and a humidity of 65 ± 5%. When treating after given time intervals, all strips were taken out and put into the headspace vials for the GC-MS analysis.
3. Results and discussion
3.1 Detection and quantification of ROS in EWNS
The ESR spectra during the treatment were presented in Fig. 3. The ESR spectrum clearly indicates the presence of OH˙ and
by EWNS, consists with former literature.30 The generation speeds of ROS from EWNS were calculated by dividing the total number of the ROS reacted with the spin trap over the detection time (20 minutes) for three measurements. The content of OH˙ is estimated about 4.58 ± 0.41 × 1012 spins per second and O2− is approximately 6.92 ± 0.78 × 1012 spins per second. The productivity of ROS is relatively stable and remains nearly unchanged during the experiment (from 1 h to 5 h). This is consistent with the previous research.31 The ROS were mainly generated due to the corona discharge.67–70 A stable corona discharge was observed at the tip of Taylor cone, which is similar to the research by Seto et al.50 The high voltage in the corona discharge can induce a chain reaction of high-velocity particle collisions, resulting in the generation of more ions.71,72 The OH˙ and
were mainly produced by this electron-molecule collision process, and the reactions are as follows:73–75 H2O + e− → H˙ + OH˙ + e− and
It should be mentioned that limited by the existing inspection technology, we found that the OH˙ and
are the main ROS contained in the EWNS, which consisting with the former literature.42 However, future research is still needed to figure out whether there are other trace substances in the EWNS which may influence the property of EWNS.
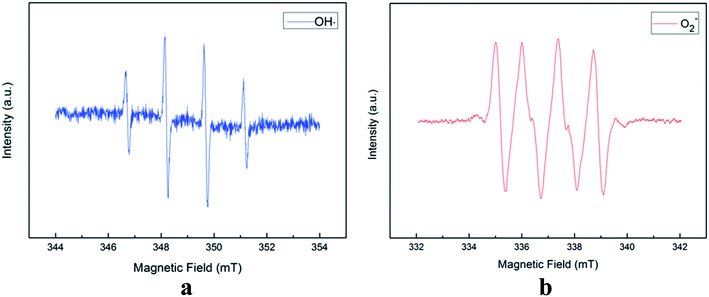 |
| Fig. 3 ROS characterization of EWNS by ESR. (a) Detection of OH˙. (b) Detection of  | |
3.2 Odor removal experiments
3.2.1 Determination of characteristic substances. Since the malodors investigated in this study are actually in mixed forms, it is necessary to figure out the characteristic substance of each type. The characteristic substances were rationally chosen based on the literature as well as the actual GC-MS results. For the smoke smell, nicotine was chosen as the marker since it is the most important tobacco smoke constituent containing critical biological properties.76 It has been already used as a marker for tobacco smoke exposure.77–79 The GC-MS results also proved the presence of nicotine in the smoke. The composition of the hot pot smell, its composition is rather complicated. As reported by Zeng et al.,80 the common compounds detected in the red oil hot pot were linalool, 4-terpineol, linalyl acetate, methyl palmitate, pinene, camphene, 3-methylene-6-(1-methyl ethyl) cyclohexene, myrcene, ocimene, γ-terpinene, caryophyllene and anethole. Linalool and anethole have been identified as the most abundant compounds in the hot pot seasoning, as reported by Zhang et al.81 We tried to extract all the substances listed above in our sample and finally chose the substance with the highest concentration as the odor marker for the hot pot smell. Due to the complexity of the hot pot, two substances with the highest concentrations were selected – linalool and anethole. For the perspiration smell, single isovaleric acid was used as the characteristic substance because it is the dominant smelling component in the human sweat. The detailed information about the characteristic substances for each odor is listed in the Table 3.
Table 3 Introduction of characteristic substances
Odor type |
Characteristic substance |
CAS number |
Molecular formula |
2D structure |
Smoke |
Nicotine |
54-11-5 |
C10H14N2 |
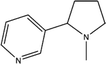 |
Cooking |
Linalool |
78-70-6 |
C10H18O |
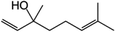 |
Anethole |
104-46-1 |
C10H12O2 |
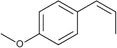 |
Perspiration |
Isovaleric acid |
503-74-2 |
C5H10O2 |
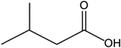 |
3.2.2 Removal of smoke. The smoke odor removal results are shown in Fig. 4(a and b). It should be mentioned that the odors in the control group naturally decayed without any treatment. Therefore, a comparison between the experimental and control groups can demonstrate, whether EWNS has an effect on odor removal. As shown in Fig. 4(a), the ordinate peak area indicates the relative concentration of nicotine. The natural decay of nicotine is very slow and the concentration showed little attenuation from 1 h to 5 h. Contrarily, the concentration of nicotine was reduced significantly by the treatment with EWNS, especially after 3 h. Fig. 4(b) illustrates the abundance–time relationship of nicotine in different experiments. The areas of various colors indicate the different amounts of nicotine remaining after 1–5 h of EWNS treatment. Further, as shown in Fig. 5, in the case of an EWNS treatment for 5 h, the residual rate of nicotine was only 4.7 ± 0.1% and the smoke removal efficiency by EWNS reached 95.3 ± 0.1%. All original data are available in the ESI.†
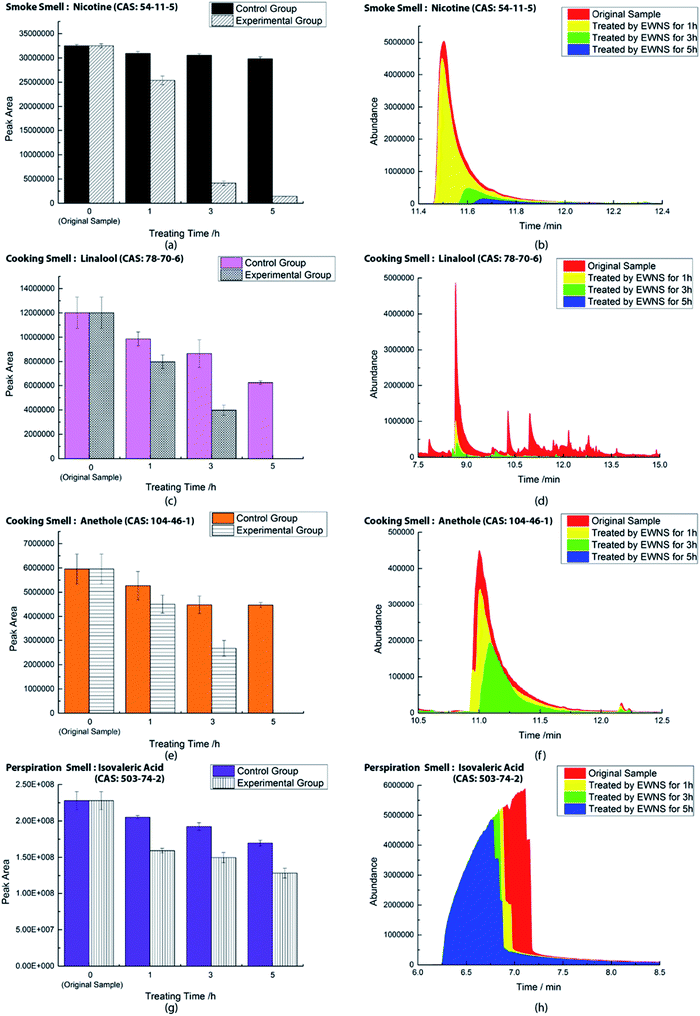 |
| Fig. 4 Odor removal data for nicotine, linalool, anethole and isovaleric acid. (a and b) The peak area and abundance of nicotine (smoke smell) among groups; (c–f) the peak area and abundance of linalool and anethole (cooking smell) among groups; (g and h) the peak area and abundance of isovaleric acid (perspiration smell) among groups. | |
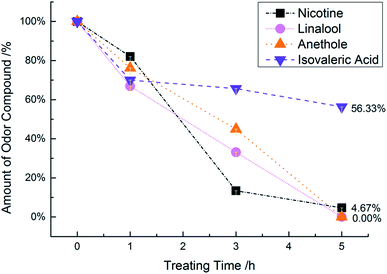 |
| Fig. 5 The decreasing concentrations of nicotine, linalool, anethole and isovaleric acid. | |
The working mechanisms of EWNS may be due to a series of chemical reactions. The ROS contained in the EWNS possess exceptionally high reactivity.75 Preliminary studies on the pulse radiolysis and gamma radiolysis of nicotine by Cercek and Ebert82 proved that OH˙ radicals can react with nicotine molecule mainly by preferentially attacking the pyrrolidine moiety to form the corresponding adducts. Similar results obtained by many other groups also confirmed this conclusion.83–85 And in some biological studies, nicotine has been confirmed to decrease ROS from human leukemia cells (e.g. HL60).86,87 It also has been found that nicotine has the ability to scavenge OH˙,
and other free radicals in gas-phase cigarette smoke.88 Thus, it can be assumed that the ROS contained in the EWNS can react with nicotine, and, thus, decrease the concentration of the smoke odor from textile products. One thing should be considered for this application is that there are any harmful intermediates produced during the reactions. In one paper,76 isocyanic acid (HNCO), a toxic molecule was speculated to form in the reaction between nicotine and OH˙, which might be a potential indoor pollution. However, in this study, we did not detect the existence of HNCO. It may be attributed to that the special conditions are needed for the reaction.
3.2.3 Removal of cooking smell. Due to the complexity of the ingredients in the hot pot taste, two representative substances – linalool and anethole were selected and the experimental results were summarized in Fig. 4(c–f). The concentrations of linalool and anethole were decreased gradually with the increase of EWNS treatment time. When treated for 5 h, the residual amount of the two characteristic substances was below the detection limit. Fig. 5 also verifies the fact that the removal efficiency of linalool and anethole both reached 100 ± 0.0% after treatment for 5 h, indicating that EWNS plays a significant role in removing the odor of hot pot.Linalool is a terpene derivative produced by plants, including orange blossoms and certain trees and the vegetation in the Mediterranean area. It has been reported that linalool reacts rapidly in the gas phase in the troposphere with OH˙ and O3 with a calculated lifetime of ∼1 h or less.89–91 Gunaseelan et al.92 applied the vitro free radical assay and found out that linalool has a strong ability to scavenge
and OH˙. Atkinson et al.89,93,94 proved that the main route for the reaction of OH˙ and linalool is by initially adding the OH˙ to the
C
C
bonds. And the terminal addition of the OH˙ to the
C
C
group results in the formation of 6-methyl-5-hepten-2-one,89 which proves to be a safe product and does not cause eye or skin irritations while linalool does.95–97 As for the removal of anethole, it has been proved that anethole is an effective free radical scavenger because it can easily form a conjugated free radical cation that can be delocalized with the aromatic ring and further stabilized the methoxy group through 1,4 interaction.91,98,99 Meylan et al.100 found that anethole can react with photochemically produced OH˙ in the atmosphere. And anethole has also been shown to inhibit the generation of ROS in human cells.91,101,102 All of these indicate the possible reaction mechanisms between ROS and anethole.
3.2.4 Removal of perspiration smell. The results of the removal of the perspiration odor are described in Fig. 4(g, h) and 5. The results showed that in the first hour, the residue amount of the isovaleric acid was rapidly reduced by nearly 30%, while the amount was decreased by less than 14% in the next four hours. And there were still 56.3 ± 2.3% of isovaleric acid detected at the end of the experiment. But it should be noted that the original concentration of each substance was different because it is hard to control their amount in the fabric by absorbing the odor from real product (in order to simulate the real scenarios, we used the real commodities in the experiments which can be found in Table 1). Thus, the removal data are not directly comparable. However, the data here show that EWNS have the potential of reducing the concentration of isovaleric acid.Again, this may be explained by the fact that the ROS in the EWNS can react with the isovaleric acid.103 Assadi et al.104 investigated the removal of isovaleric acid using a nonthermal plasma and found that the removal rate of isovaleric acid can be improved by increasing the energy density. They considered that this might be due to an increase in the production of OH˙ radicals, since the C–O substituent in isovaleric acid molecule can be easily oxidized by free radicals. Furthermore, the vapor-phase reaction of isovaleric acid has been estimated to react with photochemically produced OH˙ under the rate of 4.1 × 10−12 cm3 per molecule per second by the structure estimation method.100 Consequently, we believe that the ROS in the EWNS play the key role in the odor removal process. However, it is unclear whether only ROS work in this scenario. Further investigations are needed to better understand the deep mechanisms involved in these chemical reactions.
3.2.5 Comparison with other methods. The comparison between traditional odor removal methods and the EWNS methods were summarized in Table 4. Compared with the traditional methods, the odor compounds from pollutions can be ultimately decomposed by the EWNS method due to the highly active ROS while other methods can only eliminate the odors temporarily by dissolving them and transfer them elsewhere. Besides, these conventional technologies have unavoidable disadvantages, such as large consumption of non-renewable resources such as water and sorbing materials, which greatly increase the pressure on the environment. Although, for now, the EWNS method is more time-consuming than other methods, the parameters of the generated EWNS can be optimized in the further research. In future, we plan to build a more optimized generator to fully control the parameters of the ENWS and shorten its working time to achieve better performance.
Table 4 Comparison among existing textile odor removal methods
Method |
Brief experimental descriptions |
Comparison with efficacy |
Other risks |
Absorption/adsorption12–16 |
Activated carbon and cyclodextrins were applied as the adsorption material |
Not ultimately decompose the odor compounds |
Odors can be released back due to saturation or an elevated temperature |
Large consumption of non-renewable resources |
Hot water/steam extraction17–21 |
Water and hot steam were used to remove the odors in the washing machine or clothes dryer |
Not ultimately decompose the odor compounds |
Shorten the life of textiles |
Not suitable for large-scale home textile products |
Large consumption of water |
Water pollution |
Masking with fragrance compound22–25 |
Scented powder or spray were used to mask malodors |
Not ultimately decompose the odor compounds |
Odors are again apparent once the fragrance disperses |
Allergy aggravation |
EWNS method |
EWNS technology was applied to remove malodors |
Ultimately decompose the odor compounds |
Time-consuming |
4. Conclusion
In this study, an effective and environmentally friendly technology has been introduced to reduce the malodors from textiles. Results suggest that the EWNS played a significant role in the removal of various smells. Thus, it has the potential to clean the indoor environment from odor pollution. The highly reactive ROS from EWNS are considered as the main reason for the deodorization. These reactive species are highly reactive and capable to convert odorous compounds to non-odorous compounds through various chemical reaction mechanisms. But it is still not conclusive and there might be other synergistic pathways associated with other conspiracy features of EWNS, such as their excess charge and quantum phenomena associated with nanoscale. Further investigations are needed to better understand the chemical mechanisms involved in the odor removal. Compared with the traditional method, the EWNS can ultimately break down the odors with high efficacy. The results of this study show clearly the potential for deodorization and may also be used in a wide range of fields including wastewater odor treatment in the chemical or the livestock breeding industry.
Conflicts of interest
There are no conflicts of interest to declare.
Acknowledgements
The funding supports to this research are provided by National Key R&D Program of China through project 2018YFF0215703, Shanghai Science and Technology Committee through project 17DZ2202900, Shanghai Summit Discipline in Design through project DD18005 and Institute of Nonlinear Sciences of Donghua University respectively.
References
- E. M. Carter, L. E. Katz, G. E. Speitel and R. David, Environ. Sci. Technol., 2011, 45, 6498–6503 CrossRef CAS PubMed.
- C. J. Weschler and N. Carslaw, Environ. Sci. Technol., 2018, 52, 2419–2428 CrossRef CAS PubMed.
- A. Y. Bigazzi, M. A. Figliozzi, W. Luo and J. F. Pankow, Environ. Sci. Technol., 2016, 50, 5357–5363 CrossRef CAS PubMed.
- S. Peng, Y. Deng, W. Li, J. Chen, H. Liu and Y. Chen, Environ. Sci.: Nano, 2018, 5, 2663–2671 RSC.
- A. J. West and K. E. Annett-Hitchcock, Journal of Textile and Apparel, Technology and Management, 2014, 9, 1–13 Search PubMed.
- R. H. Mcqueen, J. J. Harynuk, W. V. Wismer, M. Keelan, Y. Xu and A. P. D. L. Mata, Int. J. Cloth. Sci. Tech., 2014, 26, 274–290 CrossRef.
- Cottonworks, Fabric Fail: Odor, https://www.cottonworks.com/topics/sourcing-manufacturing/quality-assurance/fabric-fail-odor/, accessed 18th February, 2019.
- G. Matt, P. J. E. Quintana, M. Hovell, P. Chatfield, D. Ma, R. Romero and A. Uribe, Residual tobacco smoke pollution in used cars for sale: air, dust, and surfaces, Nicotine Tob. Res., 2008, 10(9), 1467–1475 CrossRef CAS PubMed.
- L. Acuff, K. Fristoe, J. Hamblen, M. Smith and J. Chen, J. Community Health, 2016, 41, 680–687 CrossRef PubMed.
- G. Matt, P. J. E. Quintana, H. Destaillats, L. Gundel, M. Sleiman, B. Singer, P. Jacob, N. Benowitz, J. Winickoff, V. Rehan, P. Talbot, S. Schick, J. Samet, Y. Wang, B. Hang, M. Martins-Green, J. F. Pankow and M. Hovell, Thirdhand Tobacco Smoke: Emerging Evidence and Arguments for a Multidisciplinary Research Agenda, Environ. Health Perspect., 2011, 119(9), 1218–1226 CrossRef CAS PubMed.
- C. Salfino, Consumers Look for Activewear That Doesn't Stink, https://lifestylemonitor.cottoninc.com/on-the-run/, accessed 18th February 2019.
- T. S. M. Eza, W. Y. W. Ahmad and M. N. Ahmad, The Activated Carbon as Anti-odour Coated and Pigment Printed Fabric, IEEE Business, Engineering & Industrial Applications Colloquium (BEIAC), 2012, pp. 210–215 Search PubMed.
- O. Hiroki, J. K. Furne, S. John, R. Sumit and M. D. Levitt, Am. J. Gastroenterol., 2005, 100, 397–400 CrossRef PubMed.
- E. Bertone, C. Chang, P. Thiel and K. O'Halloran, Analysis and Modelling of Powdered Activated Carbon Dosing for Taste and Odour Removal, Water Res., 2018, 139, 321–328 CrossRef CAS PubMed.
- H. J. Buschmann, D. Knittel and E. Schollmeyer, J. Inclusion Phenom. Macrocyclic Chem., 2001, 40, 169–172 CrossRef CAS.
- W. E. Services, Plant Air Purifier, http://www.wolvertonenvironmental.com/plantairpurifier.html, accessed 2 Feb, 2019.
- M. Harald, N. Ulrich, N. Kai and A. Ziemann, Clothes Dryer and Method for Removing Odours from Textiles, US Pat., US20070006484A1, 2007-1-11.
- K. Takeuchi, Y. Hasegawa, H. Ishida and M. Kashiwagi, Flavour Fragr. J., 2011, 27, 89–94 CrossRef.
- T. Cooper, H. Hill, J. Kinmouth, K. Townsend and M. Hughes, Design for Longevity: Guidance on Increasing the Active Life of Clothing, 2013 Search PubMed.
- A. Mikolajczyk, A. Malankowskab, G. Nowaczykc, A. Gajewicza, N. Sizochenkod, S. Hiranoe, S. Jurgac, A. Zaleska-Medynskab and T. Puzyna, Environ. Sci.: Nano, 2016, 3, 1425–1435 RSC.
- C. Denawaka, I. A. Fowlis and J. Dean, Source, impact and removal of malodour from soiled clothing, J. Chromatogr. A, 2016, 1438, 216–225 CrossRef CAS PubMed.
- L. Shulong, Composition for removal of odors and contaminants from textiles and method, US Pat., US7135449B22006, 2006-11-14.
- B. Martel, M. Morcellet, D. Ruffin, L. Ducoroy and M. Weltrowski, Finishing of Polyester Fabrics with Cyclodextrins and Polycarboxylic Acids as Crosslinking Agents, J. Inclusion Phenom. Macrocyclic Chem., 2002, 44(1–4), 443–446 CrossRef CAS.
- Y. Liu, F. Tovia, K. Balasubramian, J. Pierce and J. Dugan, Scent Infused Textiles to Enhance Consumer Experiences, J. Ind. Text., 2008, 37(37), 263–274 Search PubMed.
- C. X. Wang and S. L. Chen, J. Ind. Text., 2005, 34, 157–166 CrossRef CAS.
- S. Munk, P. Münch, L. Stahnke, J. Adler-Nissen and P. Schieberle, J. Surfactants Deterg., 2000, 3, 505–515 CrossRef CAS.
- G. Pyrgiotakis, J. McDevitt, T. Yamauchi and P. Demokritou, J. Nanopart. Res., 2012, 14, 1027 CrossRef.
- G. Pyrgiotakis, J. McDevitt, Y. Gao, M. Eleftheriadou, A. Branco, P. Demokritou and B. Lemos, Abstracts of Papers of the American Chemical Society, 2014 Search PubMed.
- G. Pyrgiotakis, J. Mcdevitt, Y. Gao, A. Branco, M. Eleftheriadou, B. Lemos, E. Nardell and P. Demokritou, Nanomedicine, 2014, 10, 1175–1183 CrossRef CAS PubMed.
- G. Pyrgiotakis, P. Vedantam, C. Cirenza, J. Mcdevitt, M. Eleftheriadou, S. S. Leonard and P. Demokritou, Sci. Rep., 2016, 6, 21073 CrossRef CAS PubMed.
- N. Vaze, Y. Jiang, L. Mena, Y. Zhang, D. Bello, S. S. Leonard, A. M. Morris, M. Eleftheriadou, G. Pyrgiotakis and P. Demokritou, Food Control, 2018, 85, 151–160 CrossRef CAS PubMed.
- N. Vaze, G. Pyrgiotakis, J. McDevitt, L. Mena, A. Melo, A. Bedugnis, L. Kobzik, M. Eleftheriadou and P. Demokritou, Nanomedicine, 2019, 18, 234–242 CrossRef CAS PubMed.
- N. Vaze, G. Pyrgiotakis, L. Mena, R. Baumann, A. Demokritou, M. Ericsson, Y. Zhang, D. Bello, M. Eleftheriadou and P. Demokritou, Food Control, 2019, 96, 365–374 CrossRef CAS.
- Z. L. Liuqing Jiang, Building Energy & Environment, 2015, 34, 12–14 Search PubMed.
- Z. L. Ling Zhang, G. Liao, F. Wang and B. Zhang, Building Energy & Environment, 2016, 35, 22–24 Search PubMed.
- G. Pyrgiotakis, P. Vedantam, C. Cirenza, J. McDevitt, M. Eleftheriadou, S. S. Leonard and P. Demokritou, Sci. Rep., 2016, 6, 21073 CrossRef CAS PubMed.
- G. Pyrgiotakis, A. Vasanthakumar, Y. Gao, M. Eleftheriadou, E. Toledo, A. Dearaujo, J. Mcdevitt, T. Han, G. Mainelis and R. Mitchell, Environ. Sci. Technol., 2015, 49, 3737–3745 CrossRef CAS PubMed.
- F. Tao, B. Gonzalez-Flecha and L. Kobzik, Reactive oxygen species in pulmonary inflammation by ambient particulates, Free Radical Biol. Med., 2003, 35, 327–340 CrossRef CAS PubMed.
- G. Sotiriou, E. Diaz, M. S Long, J. Godleski, J. Brain, S. Pratsinis and P. Demokritou, A novel platform for pulmonary and cardiovascular toxicological characterization of inhaled engineered nanomaterial, Nanotoxicology, 2011, 6, 680–690 CrossRef PubMed.
- G. Pyrgiotakis, J. Mcdevitt, A. Bordini, E. Diaz, R. Molina, C. Watson, G. Deloid, S. Lenard, N. Fix and Y. Mizuyama, Environ. Sci.: Nano, 2014, 1, 15–26 RSC.
- I. Jajic, T. Sarna and K. Strzalka, Plants, 2015, 4, 393–411 CrossRef CAS PubMed.
- S. J. Fuller, F. P. H. Wragg, J. Nutter and M. Kalberer, Atmos. Environ., 2014, 92, 97–103 CrossRef CAS.
- H. Kaur and B. Halliwell, Methods Enzymol., 1994, 233, 67–82 CAS.
- K. Taro, N. Keisuke, I. Hiroyo, K. Katsushi, S. Keiichi and N. Yoshimi, J. Clin. Biochem. Nutr., 2012, 51, 9–14 CrossRef PubMed.
- M. Higuchi, in Wheat and Rice in Disease Prevention and Health, 2014, pp. 181–199, DOI:10.1016/B978-0-12-401716-0.00015-5.
- N. Kumar and S. Kumbhat, Essentials in Nanoscience and Nanotechnology, 2016 Search PubMed.
- L. D. Juan and J. D. L. Mora, J. Colloid Interface Sci., 1997, 186, 280–293 CrossRef PubMed.
- A. Jaworek, A. T. Sobczyk and A. Krupa, J. Aerosol Sci., 2018, 125, 57–92 CrossRef CAS.
- A. P. Bruins, J. Chromatogr. A, 1998, 794, 345–357 CrossRef CAS.
- T. Seto, T. Maekawa, S. Osone, K. Kawamura, T. Yamauchi and Y. Otani, Mechanistic aspects of electrospray ionization, J. Chromatogr. A, 2013, 85, 46–49 CAS.
- L. Rayleigh, London, Edinburgh Dublin Philos. Mag. J. Sci., 1882, 14, 184–186 CrossRef.
- M. Wilm, Mol. Cell. Proteomics, 2011, 10, M111.009407 CrossRef PubMed.
- T. Shimokage, M. Saimoto, S. Okumoto, T. Miyata and T. Yamauchi, Method of analyzing components in fine water droplets generated by electrostatic atomization, MEW Technical Report, 2005, 53, 11–16 Search PubMed.
- G. Pyrgiotakis, J. Mcdevitt, D. Hunt, M. Ishiguro, Y. Kubo, T. Imai, H. Suda, T. Yamauchi and P. Demokritou, A novel method for bacterial inactivation using electrosprayed water nanostructures, J. Nanopart. Res., 2012, 14(8), 1027–1038 CrossRef.
- T. Yuichiro and N. Tsutomu, Biosci., Biotechnol., Biochem., 2011, 75, 34–39 CrossRef PubMed.
- C. J. Weschler, A. T. Hodgson and J. D. Wooley, Environ. Technol., 1992, 26(12), 2371–2377 CrossRef CAS.
- J. D. Payne, Color. Technol., 1997, 113, 48–50 Search PubMed.
- R. H. Mcqueen, R. M. Laing, H. J. L. Brooks and B. E. Niven, Text. Res. J., 2007, 77, 449–456 CrossRef CAS.
- P. D. Beuther, M. W. Veith and K. J. Zwick, J. Eng. Fibers Fabr., 2010, 5, 1–7 CAS.
- Standardization ISO 6330-2012 Textiles – Domestic Washing and Drying Procedures for Textile Testing, ISO, Switzerland, 2012 Search PubMed.
- Standardization GB/T 18801-2015 Air Cleaner, China Standard Press, Beijing, 2015 Search PubMed.
- Standardization ISO 17299-3: 2014 Textile – Determination of Deodorant Property Part 3: Gas Chromatography Method, ISO, Switzerland, 2014 Search PubMed.
- T. R. Hammer, N. Bernerdannenmann and D. Hoefer, Flavour Fragr. J., 2013, 28, 238–244 CrossRef CAS.
- C. Callewaert, B. Buysschaert, E. Vossen, V. Fievez, d. W. T. Van and N. Boon, J. Microbiol. Methods, 2014, 103, 6–8 CrossRef CAS PubMed.
- S. L. Braver, D. P. Mackinnon and M. C. Page, Levine's Guide to Spss for Analysis of Variance, 2005 Search PubMed.
- D. M. Erceg-Hurn and V. M. Mirosevich, Am. Psychol., 2008, 63, 591–601 Search PubMed.
- C. Maolian and K. D. Cook, Anal. Chem., 2007, 79, 2031–2036 CrossRef PubMed.
- M. Kenneth, T. Gert and M. Matthias, Rapid Commun. Mass Spectrom., 2010, 7, 738–743 Search PubMed.
- C. Wu, K. Qian, M. Nefliu and R. G. Cooks, J. Am. Soc. Mass Spectrom., 2010, 21, 261–267 CrossRef CAS PubMed.
- K. Tang and A. Gomez, J. Colloid Interface Sci., 1995, 175, 326–332 CrossRef CAS.
- J. G. Drobny, Handbook of Thermoplastic Elastomers, 2nd edn, 1979 Search PubMed.
- I. L. Kanev, A. Y. Mikheev, Y. M. Shlyapnikov, E. A. Shlyapnikova, T. Y. Morozova and V. N. Morozov, Anal. Chem., 2014, 86, 1511–1517 CrossRef CAS PubMed.
- W. Wang, S. Wang, F. Liu, W. Zheng and D. Wang, Spectrochim. Acta, Part A, 2006, 63, 477–482 CrossRef PubMed.
- S. Yuewei, I. A. Abreu, D. E. Cabelli, M. J. Maroney, M. Anne-Frances, T. Miguel and V. Joan Selverstone, Chem. Rev., 2014, 114, 3854–3918 CrossRef PubMed.
- M. Hayyan, M. A. Hashim and I. M. Alnashef, Chem. Rev., 2017, 116, 3029–3085 CrossRef PubMed.
- N. Borduas, J. G. Murphy, C. Wang, G. D. Silva and J. P. D. Abbatt, Gas Phase Oxidation of Nicotine by OH Radicals: Kinetics, Mechanisms and Formation of HNCO, Environ. Sci. Technol. Lett., 2016, 3(9), 327–331 CrossRef CAS.
- S. Irina, F. Rachel, J. Joni, H. Dorothy and S. S. Hecht, Cancer Epidemiol., Biomarkers Prev., 2006, 15, 2378 CrossRef PubMed.
- P. J. Iii, N. L. Benowitz and A. T. Shulgin, Pharmacol., Biochem. Behav., 1988, 30, 249–253 CrossRef.
- K. Sugita, M. Matsumoto, Y. Inaba, O. Endo, S. Uchiyama and N. Kunugita, Bunseki Kagaku, 2013, 62, 253–257 CrossRef CAS.
- C. Zeng, L. Zhang, W. Tian, Y. Shi, J. Tang and Z.-m. Che, Sci. Technol. Food Ind., 2016, 37, 283–287 CAS.
- L. Z. Zhang, Z. Huang, J. Tang, L. U. Jing and Z. M. Che, SDE and SPME for Analysis of Volatile Components in Hot Pot Seasoning Containing Palm Oil and Rapeseed Oil Blends, Food Sci., 2014, 35(18), 156–160 CAS.
- B. Cercek and M. Ebert, Trans. Faraday Soc., 1967, 63, 1687–1698 RSC.
- K. Kosno, I. Janik, M. Celuch, J. Mirkowski, J. Kisała and D. Pogocki, Isr. J. Chem., 2014, 54, 302–315 CrossRef CAS.
- M. Simic and M. Ebert, Int. J. Radiat. Phys. Chem., 1971, 3, 259–272 CrossRef CAS.
- S. Solar, N. Getoff, K. Sehested and J. Holcman, Radiat. Phys. Chem., 1993, 41, 825–834 CrossRef CAS.
- S.-J. Lim and Y.-S. Kim, Cancer Prev. Res., 2007, 12, 25–29 Search PubMed.
- B. Ferger, C. Spratt, C. D. Earl, P. Teismann, W. H. Oertel and K. Kuschinsky, Effects of nicotine on hydroxyl free radical formation in vitro and on MPTP-induced neurotoxicity in vivo, Naunyn-Schmiedeberg's Arch. Pharmacol., 1998, 358(3), 351–359 CrossRef CAS PubMed.
- B. Zhao, Effects of Nicotine in Models of Alzheimer's Disease, Oxid. Stress Dis., 2005, 265–275 Search PubMed.
- Y. Shu, E. S. C. Kwok, E. C. Tuazon, R. Atkinson and J. Arey, Environ. Sci. Technol., 1997, 31, 896–904 CrossRef CAS.
- M. Sidheswaran and L. L. Tavlarides, Gas and Particle Phase Chemistry of Linalool Ozone Reactions and Analysis of Resulting Reactive Oxygen Species, AIChE Meeting, 2008 Search PubMed.
- M. U. Nessa, B. Philip, C. Charles, J. Q. Yu and H. Fazlul, Anticancer Res., 2012, 32, 4843–4850 CAS.
- S. Gunaseelan, A. Balupillai, K. Govindasamy, K. Ramasamy, G. Muthusamy, M. Shanmugam, R. Thangaiyan, B. M. Robert, R. P. Nagarajan and V. K. Ponniresan, PLoS One, 2017, 12, e0176699 CrossRef PubMed.
- R. Atkinson, J. Arey, S. M. Aschmann, S. B. Corchnoy and Y. Shu, Int. J. Chem. Kinet., 1995, 27, 941–955 CrossRef CAS.
- E. S. C. Kwok and R. Atkinson, Atmos. Environ., 1995, 29, 1685–1695 CrossRef CAS.
- S. Riazanskaia, G. Blackburn, M. Harker, D. Taylor and C. L. P. Thomas, Analyst, 2008, 133, 1020–1027 RSC.
- D. L. Opdyke and C. Letizia, Food Chem. Toxicol., 1980, 18, 649–650 CrossRef CAS.
- NIOSH, NIOSH Pocket Guide to Chemical Hazards, Department of Health and Human Services and National Institute for Occupational Safety and Health, Ohio, 2007 Search PubMed.
- S. Chang, A. M. Nafchi and A. A. Karim, J. Essent. Oil Res., 2016, 28, 357–363 CrossRef CAS.
- J. Taira, T. Ikemoto, T. Yoneya, A. Hagi, A. Murakami and K. Makino, Free Radical Res. Commun., 1992, 16, 197–204 CrossRef CAS.
- W. M. Meylan and P. H. Howard, Chemosphere, 1993, 26, 2293–2299 CrossRef CAS.
- D. V. Rajakumar and M. Rao, Biochem. Pharmacol., 1993, 46, 2067–2072 CrossRef CAS PubMed.
- F. N. Ko, C. H. Liao, Y. H. Kuo and Y. L. Lin, Antioxidant properties of demethyldiisoeugenol, Biochim. Biophys. Acta, Lipids Lipid Metab., 1995, 1258(2), 145–152 CrossRef.
- G. V. Buxton, C. L. Greenstock, W. Phillips Helman and A. B. Ross, Critical Review of Rate Constants for Reactions of Hydrated Electrons, Hydrogen Atoms and Hydroxyl Radicals (·OH/·O−) in Aqueous Solution, J. Phys. Chem. Ref. Data, 2009, 17(2), 513–886 CrossRef.
- A. A. Assadi, A. Bouzaza, M. Lemasle and D. Wolbert, Chem. Eng. Res. Des., 2015, 93, 640–651 CrossRef CAS.
Footnote |
† Electronic supplementary information (ESI) available. See DOI: 10.1039/c9ra01988j |
|
This journal is © The Royal Society of Chemistry 2019 |
Click here to see how this site uses Cookies. View our privacy policy here.