DOI:
10.1039/C9RA03207J
(Paper)
RSC Adv., 2019,
9, 22348-22354
A real-time ratiometric fluorescent probe for imaging of SO2 derivatives in mitochondria of living cells†
Received
29th April 2019
, Accepted 20th June 2019
First published on 18th July 2019
Abstract
A real-time ratiometric fluorescent probe (IN-CZ) for highly selective detection of sulfite was designed and synthesized, which is based on modulating the intramolecular charge transfer (ICT) of the hemicyanine dye platform. The mechanism of using the probe is mainly through the Michael addition that occurs between IN-CZ and sulfite with a detection limit of 2.99 × 10−5 M. IN-CZ displays a fast response (within 1 minute) and is highly selective for SO32−/HSO3− over ROS, biologically relevant ions, biological mercaptans and other reactive species. More importantly, IN-CZ was suitable for ratiometric fluorescence imaging in living cells, by real-time monitoring of SO32−/HSO3− changes in mitochondria targeted in living cells.
1. Introduction
Sulfur dioxide (SO2) is an inevitable product from the combustion of coal and fossil fuels as well automobile exhausts, causing a serious threat to the ecological environment and human health.1 In general, SO2 is inhaled into the human body through the respiratory tract, and then it mainly exists in a two anions equilibrium state, sulfite and bisulfite (SO32−/HSO3−) in physiological systems.2 Of which, sulfite has been widely used as an antioxidant in food, beverages, pharmaceuticals and preservatives.3 However, prolonged exposure to excessive SO2 may cause respiratory tract infection, even leading to lung cancer, cardiovascular disease and neurological disorders.4 SO2 and its derivatives (SO32−/HSO3−) can alter the intracellular thiol levels, and then destroy the redox balance in the cells leading to neuron disorders.5 Mitochondria as the main place for energy supply and transformation, provide a large number of reactive oxygen species (ROS) and reactive sulfur species (RSS).6 Moreover, in the process of energy supply and transformation in mitochondria of cells, SO2 and its derivatives (SO32−/HSO3−) can also be produced from L-cysteine in reactions catalyzed by aspartate aminotransferase-2. The abnormal endogenous levels of SO2 and its derivatives (SO32−/HSO3−) can also cause a series of pathological changes.7 The United Nations Food and Agriculture Organization (FAO) and The World Health Organization (WHO) have regulated an acceptable daily intake of sulfite to be lower than 0.7 mg kg−1 of body weight.8 Thus, developing methods to detect exogenous and endogenous SO2 and its derivatives (SO32−/HSO3−) is of great significance.
At present, there are many detection strategies for SO32−/HSO3−, including Monier-Williams,9 electrochemical,10 fluorescence spectrophotometry,11 chromatography,12 capillary electrophoresis,13 titration14 and fluorescent probe.15 Among which, fluorescent indicators for SO32−/HSO3− have attracted much attention due to their apparent advantages over other methods, such as high selectivity and specificity, operational simplicity, as well as high space–time resolution real-time detection and imaging in vivo.16 As SO32−/HSO3− is mainly expressed in mitochondria of cells, the quantitative detection of SO32−/HSO3− is of great importance in both chemical and mitochondria targeted in living cells.17
In this work, we designed and synthesized a new ratiometric fluorescent probe IN-CZ for real-time monitoring of SO32−/HSO3− changes in mitochondria targeted in living cells. The synthesis route for IN-CZ is shown in Scheme 1. The Michael addition that occurs between IN-CZ and sulfite makes the conjugate structure change (as shown in Scheme 2). The probe can not only realize ratiometric detection of SO32−/HSO3−, but also shows high selectivity and sensitivity for SO32−/HSO3− against various interferences with a detection limit of 2.99 × 10−5 M. IN-CZ exhibits excellent stability and localizes well in the mitochondria. Moreover, IN-CZ displayed a fast response (within 1 minute) and lower cell toxicity, which endows IN-CZ with good permeability and application to imaging SO32−/HSO3− in living cells.
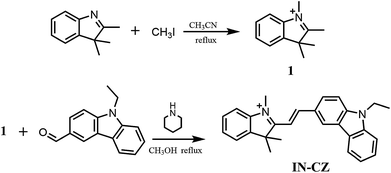 |
| Scheme 1 Synthesis route for IN-CZ. | |
 |
| Scheme 2 Proposed sensing mechanism of IN-CZ towards SO32−/HSO3−. | |
2. Experimental
2.1 Materials and instruments
All the chemicals and reagents were obtained from commercial suppliers and prepared in stock with standard methods before use. Silica gel (200–300 mesh, Qingdao Haiyang Chemical Co.) was used for column chromatography. NMR spectra were recorded on a Bruker Advance III at 400 MHz for 1H NMR and at 100 MHz for 13C NMR with chemical shifts reported as ppm (in CDCl3, TMS as internal standard). Mass spectra (MS) were measured with a Bruker Apex IV FTMS using electrospray ionization (ESI†). Absorption spectra were recorded on a Purkinje TU-1901 spectrophotometer. Fluorescence measurements were taken on a Hitachi F-7000 fluorescence spectrometer with a 10 mm quartz cuvette. Excitation wavelength was 420 nm.
2.2. Synthesis of fluorescent probe IN-CZ
2.2.1 Synthesis of compound 1. In 10 mL of acetonitrile, 2,3,3-trimethylindolenine (1.0 g, 6.3 mmol) and iodomethane (4.43 g, 31.4 mmol) were added, the mixture was stirred and refluxed overnight. Afterwards, cooling to room temperature (RT), the mixture was filtered and pink solid 1 was obtained.
2.2.2 Synthesis of compound IN-CZ. In 1 mL of piperidine and 25 mL of ethanol, a mixture of compound 1 (215 mg, 0.75 mmol) and N-ethyl-3-carbazolecarboxaldehyde (167 mg, 0.75 mmol) was stirred at 80 °C for 24 h. Afterwards, the mixture was extracted with DCM (30 × 3 mL) and washed with water (50 × 3 mL). The residue was purified by silica gel column chromatography using DCM/methanol (v/v, 30/1) as eluent to give white solid IN-CZ. 1H NMR (DMSO-d6, 400 MHz, TMS), δ (ppm): 9.10 (s, 1H), 8.64 (d, J = 7.82 Hz, 1H), 8.38 (d, J = 7.78 Hz, 1H), 8.24 (d, J = 6.5 Hz, 1H), 7.86 (t, J = 7.93 Hz, 3H), 7.72–7.68 (m, 2H), 7.63–7.58 (m, 3H), 7.38 (t, J = 6.23 Hz, 1H), 4.58–4.53 (m, 2H), 4.15 (t, J = 6 Hz, 3H), 1.85 (s, 6H), 1.38 (t, J = 8.1 Hz, 3H). 13C NMR (100 MHz, CDCl3), δ (ppm): 181.12, 156.94, 144.09, 142.52, 141.51, 140.64, 130.47, 129.38, 128.83, 127.17, 126.57, 125.42, 124.38, 123.45, 122.44, 122.36, 121.12, 110.76, 109.85, 108.94, 51.76, 38.15, 36.72, 27.43, 13.91. MALDI-MS (m/z): 379.216 for [M+].
2.3 General procedure for analysis
Parent stock solution of fluorescent probe IN-CZ (10 mM) was prepared in absolute DMSO, and stored at 5 °C in a refrigerator as standby application. The various testing species were prepared from NaBr, NaCl, NaClO, Cys, Hcy, GSH, FeCl3, FeCl2, NaNO2, NaNO3, NaHSO4, NaHCO3 and NaHSO3, and the stock solution concentrations of various species (10 mM) were prepared in twice-distilled water. The test solutions of IN-CZ (10 μM) were prepared by placing 100 μL of the corresponding stock solution into 100 mL PBS (10 mM, pH = 7.4). PBS solution was prepared with Na2HPO4 and KH2PO4, and adjusted to pH 7.4.
2.4 Determination of detection limit
According to the relevant literature published,18 the detection limit was calculated based on the fluorescence titration. Fluorescence titration was carried out in the aqueous buffer (10 mM PBS, pH = 7.4) to determine the detection limit, which was calculated with the following equation:
Where σ is the standard deviation of blank measurements, k is the slope between the fluorescence intensity vs. SO32−/HSO3− concentration.
2.5 Cytotoxicity
HeLa cells were cultured in the DMEM in a 5% CO2 humidity incubator at 37 °C. Cells were inoculated in 96-well plates at 5000 cells per plate. After overnight culture, the medium in each well was replaced by fresh medium containing different concentrations of IN-CZ (0, 5, 10, 15, 20, 25 μM). After 24 h, 3-(4,5-dimethylthiazol-2-yl)-2,5-diphenyltetrazolium bromide (MTT, 5 mg mL−1, 20 μL) was added to each plate for 3 h. Then medium was discarded, DMSO (100 μL) was added to dissolve the MTT formazan crystals and cultured for another 4 h. The absorbance in proportion to viable cell count was directly measured using a Bio-Rad 680 ELISA reader at 570 nm and all the measurements were completed three times under the condition of blank control.
2.6 Fluorescence imaging of SO32−/HSO3− in living cells
HeLa cells were grown on glass-bottom culture dishes in DMEM supplemented with 10% fetal bovine serum and 1% penicillin. The cells were incubated under an atmosphere of 5% CO2 and 95% air at 37 °C for 24 h. Before use, the cells were washed with PBS buffer solution three times. For the experiment of imaging of NaHSO3, cells were incubated with 100 μM of NaHSO3 for 30 min at 37 °C. Afterwards, cells were washed three times with PBS-free DMEM, the pretreated cells were incubated in culture media with IN-CZ (5 μM) for an additional 10 min at 37 °C, and then washed with PBS (pH = 7.4) three times. Fluorescence imaging of HeLa cells was conducted with an Olympus IX81 confocal fluorescence microscope with excitation wavelength of 405 nm.
3. Results and discussion
3.1 Spectral response of IN-CZ to SO32−/HSO3−
The absorption and fluorescence spectra of IN-CZ in the PBS buffer (pH = 7.4, PBS = 10 mM) were studied. We first explored the spectra changes in the absence and presence of SO32−/HSO3−. As shown in Fig. 1, the probe displayed a major absorption band centered at 480 nm with a corresponding fluorescence emission peak at 579 nm. With the addition of NaHSO3, the absorption intensity of the peak at 480 nm significantly receded, revealing that the structure of IN-CZ has changed. Meanwhile the emission peak at 579 nm significantly reduced, and the corresponding fluorescence emission peak at 494 nm enhanced. The main reason for the spectra change is attributed to a 1,4-addition reaction that occurs between IN-CZ and NaHSO3, the C
C conjugation between hemicyanine and carbazole is broken by the attack of SO32−/HSO3− to obtain a blue-emission which is attributed to carbazole. These results indicate that IN-CZ has potential as a ratiometric fluorescent indicator for detection of SO32−/HSO3−.
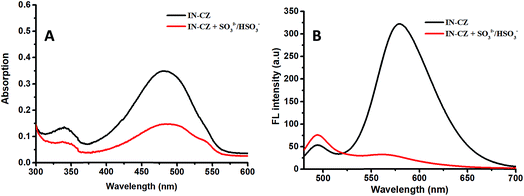 |
| Fig. 1 Spectroscopic properties of IN-CZ (10 μM) in PBS buffer (pH = 7.4, PBS = 10 mM). (A) UV-vis absorption response of IN-CZ (10 μM) in the presence of SO32−/HSO3− (10 μM). (B) Fluorescence emission intensity change of IN-CZ (10 μM) in the presence of SO32−/HSO3− (100 μM). Excitation at 420 nm. | |
3.2 Recognition mechanism of IN-CZ for SO32−/HSO3−
The recognition mechanism of IN-CZ for SO32−/HSO3− is presumably due to the 1,4-addition reaction that occurs between IN-CZ and NaHSO3 (Scheme 3). In order to verify the sensing mechanism, high resolution mass spectrometry (HRMS) was utilized to provide evidence in the absence and presence of NaHSO3 (as shown in Fig. 2). In the absence of NaHSO3, HRMS of the probe in PBS buffer (pH = 7.4, PBS = 10 mM) was conducted and the peak exhibited at m/z = 379.2161. In the presence of NaHSO3, the HRMS of the compound in PBS buffer (pH = 7.4, PBS = 10 mM) was conducted and the peak displayed at m/z = 459.2043, which is almost identical to the molecular weight of the adduct (calcd for C27H27N2O3S, m/z = 459.18). Both the literature and experimental results provide strong support for the proposed mechanism.17e,f The reaction changes the intramolecular charge transfer (ICT) of IN-CZ, and then a ratiometric fluorescent probe for detection of SO32−/HSO3− was obtained.
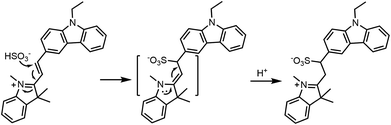 |
| Scheme 3 Proposed reaction mechanism of IN-CZ with SO32−/HSO3−. | |
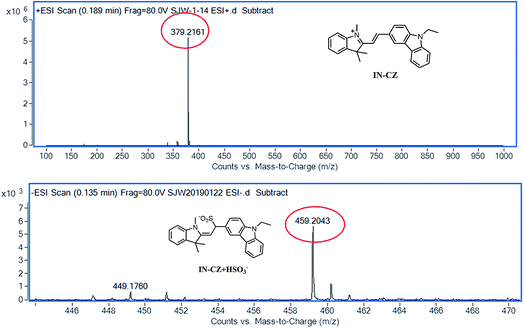 |
| Fig. 2 HRMS spectra of probe IN-CZ treated without and with HSO3−. | |
3.3 The selectivity of IN-CZ for various analytes
To confirm the selectivity of IN-CZ towards HSO3−, the probe was incubated with various analytes. As shown in Fig. 3A, with the addition of Br−, Cl−, ClO−, Hcy, Cys, GSH, HSO42−, HCO3−, Fe2+, Fe3+, NO2−, NO3−, S2−, CN−, HS− and S2O32−, there were nearly no fluorescence changes or weak fluorescence changes, with only SO32−/HSO3− causing an obvious fluorescence change. Moreover, the probe was incubated with various analytes and HSO3− together. As shown in Fig. 3B, when other analytes coexist with HSO3−, the response of the probe to HSO3− was not affected. These results demonstrated that IN-CZ is not affected by these species and the emission of IN-CZ is stable in the presence of interference species; IN-CZ can detect SO32−/HSO3− specifically.
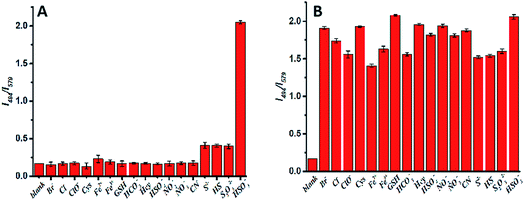 |
| Fig. 3 (A) Relative fluorescence of IN-CZ (I494/I579) in the presence of various analytes. (B) Relative fluorescence of IN-CZ (I494/I579) towards NaHSO3 (50 μM) when coexisting with various other analytes. (100 μM): Br−; Cl−; ClO−; Hcy; Cys; GSH; HSO42−; HCO3−; Fe2+; Fe3+; NO2−; NO3−; CN−; S2−; HS−; S2O32−; SO32−/HSO3− (50 μM) (except: GSH 1 mM, Hcy 1 mM, Cys 1 mM) in PBS buffer solution (pH = 7.4, PBS = 10 mM, IN-CZ = 10 μM). Excitation at 420 nm. | |
Through selectivity and interference experiments, the photostability of IN-CZ was studied by continuous laser scanning at 420 nm. The fluorescence signal loss was calculated to be less than 3% over 30 min (Fig. S1†), indicating the excellent photostability of IN-CZ. Then, the kinetic profile of the recognition of IN-CZ for SO32−/HSO3− was carried out, the response time of IN-CZ in the presence of NaHSO3 was evaluated (Fig. S2†). When NaHSO3 (50 μM) was added to the solution of IN-CZ (10 μM), the relative fluorescence of I494/I579 increased immediately. This is consistent with the fact that the 1,4-addition reaction occurred between IN-CZ and NaHSO3, making the probe conjugate structure change rapidly. The results above demonstrate the excellent photostability and sensitivity of IN-CZ.
3.4 Quantification of SO32−/HSO3− and detection limit
The optical response validity of IN-CZ toward SO32−/HSO3− was investigated. The fluorescence spectra of IN-CZ with various concentrations of NaHSO3 (1, 2, 3, 4, 5, 6, 7, 8, 9, 10, 11, 12, 13, 14, 15, 16, 17, 18, 19, 20, 25, 30, 40, 50 μM) are shown in Fig. 4A. Upon gradually increasing the concentration of NaHSO3, the fluorescence band centered at around 579 nm decreased immediately, and the fluorescence band centered at around 494 nm increased. The relative fluorescence intensity of I494/I579 vs. the concentration of NaHSO3 in the range of 1–13 μM was linear with a detection limit of 299 nM, as shown in Fig. 4B. Therefore, the probe IN-CZ is capable of the identification and quantification of SO32−/HSO3−.
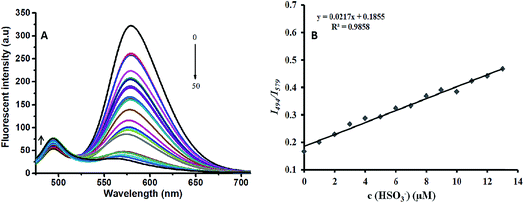 |
| Fig. 4 (A) Fluorescence spectra of IN-CZ (10 μM) upon addition of NaHSO3 (1, 2, 3, 4, 5, 6, 7, 8, 9, 10, 11, 12, 13, 14, 15, 16, 17, 18, 19, 20, 25, 30, 40, 50 μM). The reaction was performed in PBS buffer (pH = 7.4, 10 mM). (B) The linear relationship between the fluorescence intensity ratio of I494/I579 and the SO32−/HSO3− concentration (1, 2, 3, 4, 5, 6, 7, 8, 9, 10, 11, 12, 13 μM). The reaction was performed in PBS buffer (pH = 7.4, 10 mM). Excitation at 420 nm. | |
We also investigated the pH effect on the fluorescence spectrum of IN-CZ in the absence and presence of NaHSO3 under a wider pH range (1–12). As shown in Fig. S3,† the spectral response of IN-CZ was evaluated in PBS buffer (pH = 7.4, 10 mM). The fluorescence intensity ratio (I494/I579) of the probe was barely affected in the pH range 5.0–10.0, which suggested that the probe was stable within this pH range. Afterwards, in the presence of NaHSO3, the probe could respond to NaHSO3 in the pH range 5.0–10.0, indicating that IN-CZ could be used for the detection of SO32−/HSO3− under physiological conditions.
3.5 Cellular fluorescence imaging and cytotoxicity
To explore the application of IN-CZ in living cells, its cytotoxicity was evaluated in HeLa cells, using the 3-(4,5-dimethyl-2-thiazolyl)-2,5-diphenyltetrazolium bromide (MTT) assay under different probe concentrations. As displayed in Fig. 5, no significant change in the cell viability was observed when the cells were cultured in the presence of a high concentration of IN-CZ of 25 μM for 24 h. This indicates that IN-CZ shows no cytotoxicity and good biocompatibility in live cells.
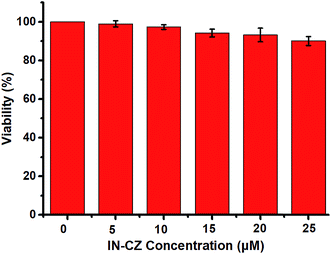 |
| Fig. 5 Cytotoxicity assays for IN-CZ in HeLa cells. Cells were treated with different concentrations of probe IN-CZ for 24 h. Concentrations of IN-CZ (0 μM, 5 μM, 10 μM, 15 μM, 20 μM, 25 μM). | |
To confirm the specific stain of mitochondria in living cells, we carried out a colocalization experiment (Fig. 6). HeLa cells were incubated with IN-CZ (5 μM) and Mito-tracker Red (100 nM, a commercial red-fluorescent mitochondrial dye) for 15 min at 37 °C, and the excess dye was washed away with phosphate buffered saline. The images of yellow channel and red channel merged well in the HeLa cells. Along the red line, the intensity trends of Mito-tracker Red and IN-CZ have a high degree of overlap. Moreover, the Pearson’s correlation coefficient was calculated to be 0.838, indicating that IN-CZ could localize in the mitochondria, which is one of the main organelles for generation of endogenous SO2 in vivo. These results imply the potential capacity of IN-CZ for monitoring endogenous SO2 in living cells.
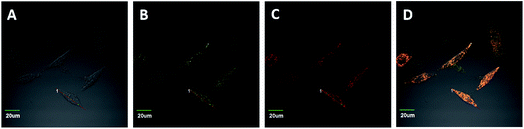 |
| Fig. 6 HeLa cells were incubated with IN-CZ (5 μM) and Mito-Tracker Red (100 nM): (A) bright-field; (B) yellow channel of IN-CZ; (C) red-channel of Mito-Tracker Red; (D) merged images (B) and (C) in bright-field. Excitation at 405 nm. Scale bar: 20 μm. | |
To determine the favorable properties of the IN-CZ for monitoring SO2 derivatives, we then evaluated the potential application of IN-CZ in living cells. As shown in Fig. 7, cells were incubated with IN-CZ (5 μM) for 10 min at 37 °C. There were nearly no fluorescence signals in the blue channel; obvious fluorescence signals can be observed in the yellow channel, which indicates the remarkable membrane permeability of the probe. Afterwards, cells were incubated with 100 μM of NaHSO3 for 30 min at 37 °C, then the cells were incubated in culture media with IN-CZ (5 μM) for an additional 10 min at 37 °C. As we expected, the blue fluorescence channel gradually became bright and the yellow fluorescence channel became darkened. The obvious changes in ratiometric fluorescence responses generated from the blue channel and yellow channel in HeLa cells were observed. These results imply that IN-CZ could monitor SO32−/HSO3− changes through the ratiometric fluorescence imaging in living cells.
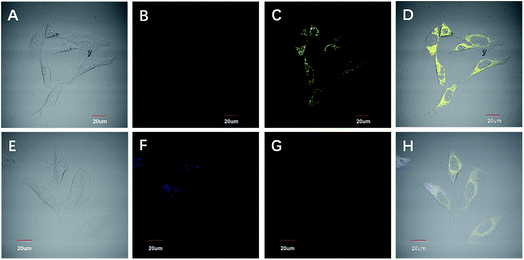 |
| Fig. 7 Images of HeLa cells after incubation with IN-CZ (5 μM) in the absence and presence of NaHSO3 (100 μM). (A and E) Bright-field; (B–D) are the images in the absence of NaHSO3, blue channel, yellow channel and merged images in bright field; (F–H) are the images in the presence of NaHSO3, blue channel, yellow channel and merged images in bright field. Excitation at 405 nm. Scale bar: 20 μm. | |
4. Conclusion
In summary, we have presented a novel ratiometric fluorescent probe IN-CZ based on a hemicyanine dye platform. The probe could be used as a ratiometric fluorescent sensor for SO32−/HSO3− anions in aqueous environment based on a Michael addition reaction. The probe displayed a fast reaction time, good sensitivity, and high selectivity for SO32−/HSO3− over other reactive species, ROS, biologically relevant ions and biological mercaptans. Moreover, IN-CZ is a ratiometric fluorescent probe for the real-time monitoring of SO32−/HSO3− changes through the ratiometric fluorescence imaging with mitochondria targeted in living cells with good permeability and low cytotoxicity. We expect that this design will be further developed as a good probe for SO2 derivatives in realistic samples and biological applications.
Conflicts of interest
There are no conflicts to declare.
Acknowledgements
We gratefully acknowledge financial support from the National Natural Science Foundation of China (No. 21575015 and 21505004).
Notes and references
-
(a) T. M. Chen, W. G. Kuschner, J. Gokhale and S. Shofer, Am. J. Med. Sci., 2007, 333, 249–256 CrossRef;
(b) M. Kampa and E. Gastanas, Environ. Pollut., 2008, 151, 362–367 CrossRef CAS.
- M. H. Stipanuk and I. Ueki, J. Inherited Metab. Dis., 2011, 34, 17–32 CrossRef CAS.
-
(a) S. Iwasawa, Y. Kikuchi and Y. Nishiwaki, J. Occup. Health, 2009, 51, 38–47 CrossRef CAS;
(b) M. Reist, P. Jenner and B. Halliwell, FEBS Lett., 1998, 423, 231–234 CrossRef CAS.
-
(a) G. Li and N. Sang, Ecotoxicol. Environ. Saf., 2009, 72, 236–241 CrossRef CAS;
(b) G. L. Li, R. J. Li and Z. Q. Meng, Eur. J. Pharmacol., 2010, 645, 143–150 CrossRef PubMed.
-
(a) N. Sang, Y. Yun, H. Y. Li, L. Hou, M. Han and G. K. Li, Toxicol. Sci., 2010, 114, 226–236 CrossRef CAS;
(b) N. Sang, Y. Yun, G. Y. Yao, H. Y. Li, L. Guo and G. K. Li, Toxicol. Sci., 2011, 124, 400–413 CrossRef CAS.
-
(a) Y. Liu, K. Li, M. Y. Wu, Y. H. Liu, Y. M. Xie and X. Q. Yu, Chem. Commun., 2015, 51, 10236–10239 RSC;
(b) W. Xu, C. L. Teoh, J. J. Peng, D. D. Su, L. Yuan and Y. T. Chang, Biomaterials, 2015, 56, 1–9 CrossRef CAS PubMed.
-
(a) F. Y. Zhang, X. W. Liang, W. Z. Zhang, Y. L. Wang, H. L. Wang, Y. H. Mohammed, B. Song, R. Zhang and J. L. Yuan, Biosens. Bioelectron., 2017, 87, 1005–1011 CrossRef CAS;
(b) W. Z. Zhang, J. M. Zhang, H. L. Zhang, L. Y. Cao, R. Zhang, Z. Q. Ye and J. L. Yuan, Talanta, 2013, 116, 354–360 CrossRef CAS.
- W. J. FAO, in WHO food additives series, World Health Organization, Geneva, 60th edn, 2009 Search PubMed.
- G. W. Monier-Williams, Analyst, 1927, 52, 343–344 RSC.
-
(a) T. Yilmaz and G. Somer, Anal. Chim. Acta, 2007, 603, 30–35 CrossRef;
(b) C. S. Pundir and R. Rawal, Anal. Bioanal. Chem., 2013, 405, 3049–3062 CrossRef CAS.
-
(a) P. W. West and G. Gaeke, Anal. Chem., 1956, 28, 1816–1819 CrossRef CAS;
(b) M. A. Segundo, A. O. Rangel, A. Cladera and C. Victor, Analyst, 2000, 125, 1501–1505 RSC;
(c) T. Williams, S. Mcelvany and E. Ighodalo, Anal. Chim. Acta, 1981, 123, 351–354 CrossRef CAS.
-
(a) P. Laura, D. L. Giuseppe and Q. Enrica, Food Chem., 1998, 63, 275–279 CrossRef;
(b) R. F. McFeeters and A. O. Barish, J. Agric. Food Chem., 2003, 51, 1513–1517 CrossRef CAS PubMed.
-
(a) D. Zydrunas and P. Audrius, Electrophoresis, 2002, 23, 2439–2444 CrossRef;
(b) J. Giedre, D. Zydrunas and P. Audrius, J. Chromatogr. A, 2001, 934, 67–73 CrossRef.
- L. Denise and B. Mauro, Food Addit. Contam., 2001, 18, 773–777 CrossRef.
-
(a) L. Tan, W. Lin, S. Zhu, L. Yuan and K. B. Zheng, Org. Biomol. Chem., 2014, 12, 4637–4643 RSC;
(b) Y. Q. Sun, J. Liu, J. Zhang, T. Yang and W. Guo, Chem. Commun., 2013, 49, 2637–2639 RSC;
(c) X. F. Yang, M. Zhao and G. Wang, Sens. Actuators, B, 2011, 152, 8–13 CrossRef CAS;
(d) G. Y. Li, Y. Chen, J. Q. Wang, J. H. Wu, G. Gasser, L. N. Ji and H. Chao, Biomaterials, 2015, 63, 128–136 CrossRef CAS;
(e) W. Q. Chen, X. J. Liu, S. Chen, X. Z. Song and J. Kang, RSC Adv., 2015, 5, 25409–25415 RSC;
(f) S. Paul, K. Ghoshal, M. Bhattacharyya and D. K. Maiti, ACS Omega, 2017, 2, 8633–8639 CrossRef CAS;
(g) M. G. Choi, J. Y. Hwang, S. Y. Eor and S. K. Chang, Org. Lett., 2010, 12, 5624–5627 CrossRef CAS PubMed.
-
(a) J. Chan, S. C. Dodani and C. J. Chang, Nat. Chem., 2012, 4, 973 CrossRef CAS;
(b) Y. M. Yang, Q. Zhao, W. Feng and F. Y. Li, Chem. Rev., 2013, 113, 191–270 Search PubMed;
(c) J. J. Du, M. M. Hu, J. L. Fan and X. J. Peng, Chem. Soc. Rev., 2012, 41, 4511–4535 RSC;
(d) F. Zhou, Y. Sultanbawa, H. Feng, Y. L. Wang, Q. T. Meng, Y. Wang, Z. Q. Zhang and R. Zhang, J. Agric. Food Chem., 2019, 67, 4375–4383 CrossRef CAS;
(e) W. Q. Chen, Q. Fang, D. L. Yang, H. Y. Zhang, X. Z. Song and J. Foley, Anal. Chem., 2015, 87, 609–616 CrossRef CAS;
(f) Y. Y. Ma, Y. H. Tang, Y. P. Zhao, S. Y. Gao and W. Y. Lin, Anal. Chem., 2017, 89, 9388–9393 CrossRef CAS.
-
(a) J. Yang, K. Li, J. T. Hou, L. L. Li, C. Y. Lu, Y. M. Xie, X. Wang and X. Q. Yu, ACS Sens., 2016, 1, 166–172 CrossRef CAS;
(b) X. L. Zheng, H. Li, W. Feng, H. C. Xia and Q. H. Song, ACS Omega, 2018, 3, 11831–11837 CrossRef CAS;
(c) G. Chen, W. Zhen, C. Y. Zhao, Y. X. Liu, T. Chen, Y. L. Li and B. Tang, Anal. Chem., 2018, 90, 12442–12448 CrossRef CAS;
(d) D. Y. Li, X. W. Tian, Z. Li, J. H. Zhang and X. B. Yang, J. Agric. Food Chem., 2019, 67, 3062–3067 CrossRef CAS;
(e) C. C. Gao, Y. Tian, R. B. Zhang, J. Jing and X. L. Zhang, New J. Chem., 2019, 43, 5255–5259 RSC;
(f) Y. F. Wang, Q. T. Meng, R. Zhang, H. M. Jia, X. H. Zhang and Z. Q. Zhang, Org. Biomol. Chem., 2017, 15, 2734–2739 RSC.
-
(a) H. Chen, B. L. Dong, Y. H. Tang and W. Y. Lin, Chem.–Eur. J., 2015, 21, 11696–11700 CrossRef CAS;
(b) B. P. Guo, X. Z. Pan, Y. F. Liu, L. X. Nie, H. Z. Zhao, Y. Z. Liu, J. Jing and X. L. Zhang, Sens. Actuators, B, 2018, 256, 632–638 CrossRef CAS;
(c) C. Y. Liu, C. X. Shao, H. F. Wu, B. P. Guo, B. C. Zhu and X. L. Zhang, RSC Adv., 2014, 4, 16055–16061 RSC.
Footnote |
† Electronic supplementary information (ESI) available. See DOI: 10.1039/c9ra03207j |
|
This journal is © The Royal Society of Chemistry 2019 |
Click here to see how this site uses Cookies. View our privacy policy here.