DOI:
10.1039/C9RA04216D
(Paper)
RSC Adv., 2019,
9, 22749-22754
One-pot synthesis of amides via the oxidative amidation of aldehydes and amines catalyzed by a copper-MOF†
Received
4th June 2019
, Accepted 10th July 2019
First published on 23rd July 2019
Abstract
An efficient method for the oxidative amidation of aldehydes with primary aromatic and aliphatic amines has been developed for the synthesis of a wide variety of amides using inexpensive Cu2(BDC)2DABCO (Cu-metal–organic framework [MOF]) as a recyclable heterogeneous catalyst, and N-chlorosuccinimide and aqueous tert-butyl hydroperoxide as oxidants in acetonitrile. This amidation reaction is operationally straightforward and provides secondary amides in good yields in most cases, utilizing inexpensive and readily available reagents under mild conditions.
Introduction
Amide-bond formation is one of the most important organic reactions, and is essential in the synthesis of pharmaceuticals, natural products and polymers such as peptides, bortezomib (an anticancer drug) and nylon 66, for example.1 Indeed, more than 25% of drugs contain at least one amide group.2 Routine amide synthesis from amines and carboxylic acids involves the use of coupling agents including carbodiimides, carbonyldiimidazole, hydroxybenzotriazoles and 2-(1H-benzotriazol-1-yl)-1,1,3,3-tetramethyluronium hexafluorophosphate (TBTU), for example.3 Alternatively, amides are often produced from the reaction of an amine with activated carboxylic acid derivatives.4 These methods have several drawbacks, such as poor atom efficiency, use of hazardous and expensive reagents and generation of waste products leading to environmental problems. To address these challenges, novel routes to amide-bond formation have been developed over recent years, including the Staudinger reaction,5 an acid-promoted Schmidt reaction that forms an amide from an alkyl azide and a ketone,6 catalytic acylation of amines with carboxylic acid,7 aerobic oxidative coupling of alcohols and amines,8 hydroamination of alkynes,9 amino carbonylation of haloarenes10 and oxidative amidation of aldehydes.11–21 Among these, oxidative amidation of aldehydes is synthetically useful, as not only are both starting materials readily available, but the process is atomically economical and environmentally benign.
Oxidative amidation of aldehydes with amines was first reported by Nakagawa and co-workers11 using stoichiometric amounts of nickel peroxide. Subsequently, several research groups have developed new methods for the direct conversion of amides from aldehydes using iodine,12 N-bromosuccinimide (NBS),13 manganese oxide14 and tert-butylhydroperoxide (TBHP)16 as oxidizing agents. In addition, catalytic methods using copper,22 copper/silver,18 transition metals (Pd, Rh and Ru)19 and lanthanides20 have also been reported. These pioneering works provide efficient and environmentally advantageous methods for amide formation. However, in some cases, the reactions require hazardous and expensive dual catalysts, and in most cases, separation of homogenous catalysts such as CuSO4 and Cu(OAc)2 is problematic and the catalysts are not recyclable.22b,c Hence, the search for a less costly, greener, heterogeneous and simpler catalyst system remains a challenge.
As part of our ongoing work to develop metal–organic frameworks (MOFs) as efficient heterogeneous, green and recyclable catalysts in organic synthesis,23 we report herein the improved oxidative amidation of aldehydes 1 with a primary amine 2 for the synthesis of secondary amides 3, using inexpensive and a readily available Cu-MOF, Cu2(BDC)2DABCO, as the recyclable catalyst, and N-chlorosuccinimide (NCS) and tert-butyl hydroperoxide (TBHP) as the oxidants (Scheme 1).
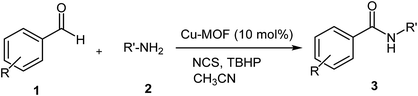 |
| Scheme 1 Oxidative amidation of aldehydes with amine catalyzed by Cu2(BDC)2DABCO (Cu-MOF). | |
Experimental section
Materials and methods
All chemicals were purchased from commercial sources and used without further purification. Cu2(BDC)2(DABCO) was synthesized according to our previously reported procedure.23a All reactions were monitored by thin-layer chromatography (TLC) using plates coated with Merck 60 HF254 silica under ultraviolet (UV) light. Infrared (IR) spectra were recorded using a Shimadzu 8400s Fourier-transform (FTIR) spectrometer. 1H-nuclear magnetic resonance (NMR) and 13C-NMR spectra were recorded using a BRUKER DRX 500-AVANCE FT-NMR instrument (CDCl3 solution) at 500 and 125 MHz, respectively. Scanning electron microscope (SEM) images were captured with a ZEISS scanning electron microscope at 30 kV with gold coating.
Synthesis of amides via the oxidation amidation of aldehydes and amines catalyzed by Cu2(BDC)2(DABCO)
To a solution of amine (1 mmol) in CH3CN (5 ml), NCS was added (1 mmol) and the reaction mixture was stirred for 1 h at room temperature. Then, aldehyde (1 mmol), Cu2(BDC)2DABCO (10% mol) and TBHP 70% (1 mmol) were added, and the reaction temperature was increased up to 65 °C and the reaction mixture was allowed to stir for 2 h. The reaction progress was monitored by TLC. After reaction completion, the catalyst was filtered and the filtrate was evaporated under reduced pressure, before the residue was purified using silica gel column chromatography (hexane/ethyl acetate [4
:
1]).
Synthesis of N-benzylbenzamide (2 g scale)
To a solution of benzylamine (1.4 ml, 12.8 mmol) in CH3CN (20 ml), NCS (1.71 g, 12.8 mmol) was added and the reaction mixture was stirred for 1 h at room temperature. Then, benzaldehyde (1.3 ml, 12.8 mmol), Cu2(BDC)2DABCO (10% mol) and TBHP 70% (1.76 ml, 12.8 mmol) were added, and the reaction temperature was increased up to 65 °C and the reaction mixture was allowed to stir for 2 h. The reaction progress was monitored by TLC. After reaction completion, the catalyst was filtered and the filtrate was evaporated under reduced pressure, before the residue was purified using silica gel column chromatography (hexane/ethyl acetate [4
:
1]) to yield 2.01 g of 3a (74%).
Selected spectral data
N-Benzylbenzamide, white solid; 160 mg, yield: 75%; mp: 104–106 °C. 1H NMR (500 MHz, CDCl3): δ 7.79 (d, J = 7.1 Hz, 2H), 7.48–7.51 (t, J = 7.1 Hz, 1H), 7.41–7.44 (m, 2H), 7.32–7.36 (m, 4H), 7.26–7.31 (m, 1H), 6.44 (brs, 1H), 4.65 (d, J = 5.5 Hz, 2H). 13C NMR (125 MHz, CDCl3): δ 167.5, 138.2, 134.5, 131.7, 128.9, 128.7, 128.1, 127.7, 127.1, 44.2.
Results and discussion
Cu2(BDC)2DABCO (Cu-MOF) catalysts were prepared and characterized using our previously reported method.23a First, a model reaction of benzaldehyde 1a and benzyl amine in the presence of TBHP to form N-benzylbenzamide was chosen to identify the catalytic system. Different amounts of Cu-MOF were screened at room temperature under reflux conditions, but unfortunately, only a trace of product was observed (Table 1, entries 1–4). In another attempt, benzylamine and NCS were first reacted together in acetonitrile at room temperature for 1 h followed by the addition of 5 mol% of the catalyst and stoichiometric amounts of benzaldehyde and TBHP (1.0 equiv.) at room temperature. In this case, we found that benzaldehyde was converted to N-benzylbenzamide, 3a, in only 35% yield after 24 h (entry 5). Increasing the reaction temperature from 25 to 65 °C resulted in a significant increase in the yield of up to 75% after 2 h (entry 6). With 1 mol% catalyst loading, the yield of the amide decreased slightly to 60% (entry 7), while use of 10 mol% loading did not significantly affect the yield of the reaction (Table 1, entry 8).
Table 1 Optimization of the reaction conditions for amide bond formation from aldehydesa
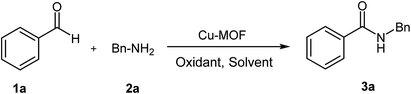
|
Entry |
Catalyst Cu-MOF (mol%) |
Oxidant |
Solvent |
T (°C) |
Yield (%) |
Reaction conditions: benzaldehyde (1 mmol), benzylamine (1 mmol), oxidant (1.0 mmol), solvent (5 ml), time: 2 h. |
1 |
5 |
TBHP |
CH3CN |
25 |
Trace |
2 |
10 |
TBHP |
CH3CN |
25 |
Trace |
3 |
20 |
TBHP |
CH3CN |
25 |
Trace |
4 |
20 |
TBHP |
CH3CN |
80 |
10 |
5 |
5 |
NCS/TBHP |
CH3CN |
25 |
35 |
6 |
5 |
NCS/TBHP |
CH3CN |
65 |
75 |
7 |
1 |
NCS/TBHP |
CH3CN |
65 |
60 |
8 |
10 |
NCS/TBHP |
CH3CN |
65 |
76 |
9 |
5 |
NCS/TBHP |
DMF |
80 |
51 |
10 |
5 |
NCS/TBHP |
THF |
65 |
62 |
11 |
5 |
NCS |
CH3CN |
65 |
Trace |
12 |
— |
NCS/TBHP |
CH3CN |
65 |
— |
The effect of the solvents was also investigated, and benzaldehyde and benzylamine were subjected to the same reaction conditions with two other solvents, dimethylformamide (DMF) and tetrahydrofuran (THF). It was found that acetonitrile was the best solvent for this reaction (entries 9–10), possibly due to the dependence of the proton-transfer-mediated electron transfer on the solvent type. The donor number of the solvent decreases in the order of DMF (26.6) > THF (20.0) > CH3CN (14.8), and as a result, the yield of products increased accordingly.24 Control experiments (entries 11–12) revealed that an oxidant (TBHP; entry 11) and Cu-MOF catalyst (entry 12), are both necessary for amide bond formation.
After optimization of the model reaction, and in order to understand the substrate scope of this reaction, a wide range of aromatic aldehydes and the amine were reacted under the optimized reaction conditions (Table 2). Good yields of secondary amides were obtained in most cases (3a–g). Aldehydes with electron-donating or electron-withdrawing substituents achieved good to excellent yields of secondary amides (3k–p), indicating that the reaction is not sensitive to electronic effects. Benzyl and aliphatic amines (2a–g) were the most favored substrates, and provided good yields of the amide products.
Table 2 Cu-MOF catalyzed synthesis of secondary amides from aldehydes and primary aminesa
The catalyst Cu-MOF also exhibited good recyclability and stability. For instance, the catalyst was recovered by simple filtration, washed with methanol and dried in an oven, and could be reused four times (Table 3). The catalyst could also be stored for a long time under an air atmosphere without any significant loss of catalytic activity.
Table 3 Recovery and reuse of Cu-MOF in the oxidative amination of aldehyde
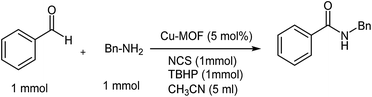
|
Run |
1 |
2 |
3 |
4 |
Yield (%) |
75 |
75 |
74 |
72 |
The X-ray diffraction (XRD) pattern of Cu2(BDC)2DABCO shows that the crystalline structure of Cu2(BDC)2DABCO is maintained after four runs (Fig. 1).23
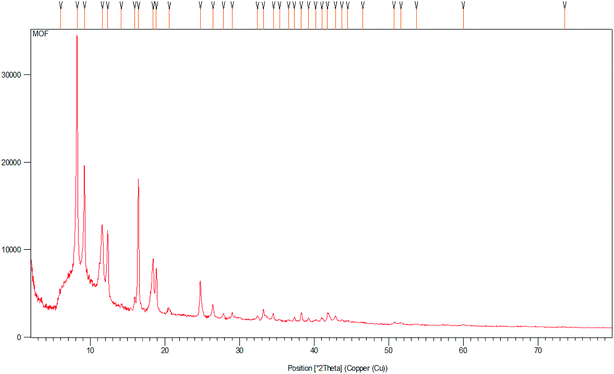 |
| Fig. 1 XRD pattern of recycled Cu2(BDC)2DABCO. | |
Based on previously reported studies,25 we considered a possible mechanism for this reaction, as shown in Scheme 2. Cu(II) oxidized TBHP in such a way that TBHP was transformed into a tert-butylperoxy radical and Cu(II) was also reduced to Cu(I). The tert-butylperoxy radical captured a hydrogen from benzaldehyde, thereby converting the aldehyde into a benzoyl radical, as reported by Xu et al.26 With the redox reaction, the N-chloramine (resulting from the reaction of benzylamine and NCS) was transformed into an amino radical, as reported as reported by Minisci.21,22,27 In the last step, the amino radical and the benzoyl radical were coupled to yield the corresponding amide products.
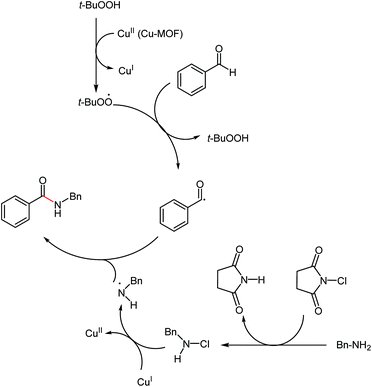 |
| Scheme 2 Proposed mechanism for the synthesis of amides catalyzed by Cu-MOF. | |
A comparison with other catalytic systems in the oxidative amidation of aldehydes and amines demonstrated that our present Cu-MOF catalyst system exhibits a better catalyst performance (Table 4).
Table 4 Comparison of activity for different catalytic systems in the oxidative amidation of aldehydes
Entry |
Catalyst |
Reaction condition |
Recyclable |
Time |
Yield |
Ref. no. |
1 |
CuBr (5 mol%) |
NBS CH3CN/CCl4, 90 °C |
No |
15 h |
87 |
13b |
2 |
NHC/DBU (20 mol%) |
HFIP as additive (1.5 equiv.), R–NH2 (2.5 equiv.), THF |
No |
8 h |
92 |
15 |
3 |
FeSO4·7H2O (5 mol%) |
TBHP, CaCO3, CH3CN, 60 °C |
No |
16 h |
72 |
21 |
4 |
CuSO4·5H2O |
TBHP, K2CO3, CH3CN/H2O, 80 °C |
No |
4 h |
63 |
22a |
5 |
Cu(OAc)2 |
TBHP, CH3CN, reflux |
No |
4 h |
74 |
22b |
6 |
CuSO4·5H2O (50 mol%) |
TBHP, CH3CN, reflux |
No |
24 h |
90 |
22c |
7 |
Cu2(BDC)2DABCO (0.05 mol%) |
TBHP, CH3CN, reflux |
Yes |
1 h |
75 |
Present work |
The structures of all products were elucidated from their 1H-NMR spectra and melting points, as described for N-phenylbenzamide. The 1H-NMR spectrum of diphenyl amine showed that the aromatic protons gave rise to multiplets in the region δ = 6.92–7.28 ppm, benzylic CH2 at 4.64 ppm and N–H at 6.45 ppm.
Conclusion
In conclusion, we have identified Cu-MOF as a green and recyclable heterogeneous catalyst for the efficient oxidative amidation of aldehydes with primary amines for the synthesis of secondary amides. A different range of amines and aldehydes are applicable in this reaction. Furthermore, this novel cost-effective amide formation reaction provides practical alternatives for the synthesis of amides under mild conditions. Further studies on the catalytic application MOFs are in progress and will be presented in the future.
Conflicts of interest
There are no conflicts to declare.
References
-
(a) J. W. Bode, Curr. Opin. Drug Discovery Dev., 2006, 9, 765 CAS;
(b) T. Cupido, J. Tulla-Puche, J. Spengler and F. Albericio, Curr. Opin. Drug Discovery Dev., 2007, 10, 768 CAS;
(c) J. M. Humphrey and A. R. Chamberlin, Chem. Rev., 1997, 97, 2243 CrossRef CAS PubMed.
-
(a) E. Valeur and M. Bradley, Chem. Soc. Rev., 2009, 38, 606 RSC;
(b) R. C. Larock, Comprehensive Organic Transformations, VCH, New York, 1999 Search PubMed;
(c) https://www.fda.gov/Drugs/DrugSafety/ucm106494.htm.
-
(a) J. M. Humphrey and A. R. Chamberlin, Chem. Rev., 1997, 97, 2243–2266 CrossRef CAS;
(b) P. Wipf, Reagents for High-Throughput Solid-Phase and Solution- Phase Organic Synthesis, in Handbook of Reagents for Organic Synthesis, Wiley & Sons, New York, 2005 Search PubMed;
(c) E. Valeur and M. Bradley, Chem. Soc. Rev., 2009, 38, 606–631 RSC.
-
(a) S. Naik, G. Bhattacharjya, B. Talukdar and B. K. Patel, Eur. J. Org. Chem., 2004, 1254–1260 CrossRef CAS;
(b) A. Teichert, K. Jantos, K. Harms and A. Studer, Org. Lett., 2004, 6, 3477–3480 CrossRef CAS PubMed;
(c) D. M. Shendage, R. Froehlich and G. Haufe, Org. Lett., 2004, 6, 3675–3678 CrossRef CAS PubMed;
(d) D. A. Black and B. A. Arndtsen, Org. Lett., 2006, 8, 1991–1993 CrossRef CAS PubMed;
(e) A. R. Katritzky, C. Cai and S. K. Singh, J. Org. Chem., 2006, 71, 3375–3380 CrossRef CAS PubMed.
-
(a) B. L. Nilson, L. L. Kiessling and R. T. Raines, Org. Lett., 2000, 2, 1939–1940 CrossRef;
(b) F. Damakaci and P. DeShong, J. Am. Chem. Soc., 2003, 125, 4408–4409 CrossRef PubMed;
(c) N. Shangguan, S. Katukojvala, R. Greenberg and L. J. Williams, J. Am. Chem. Soc., 2003, 125, 7754–7755 CrossRef CAS PubMed;
(d) R. Merkx, A. J. Brouwer, D. T. S. Rijkers and R. M. J. Liskamp, Org. Lett., 2005, 7, 1125–1128 CrossRef CAS PubMed.
-
(a) R. F. Schmidt, Ber. Dtsch. Chem. Ges., 1924, 57, 704–706 CrossRef;
(b) L. Yao and J. Aube, J. Am. Chem. Soc., 2007, 129, 2766–2767 CrossRef CAS PubMed.
-
(a) K. Ishihara, S. Ohara and H. Yamamoto, J. Org. Chem., 1996, 61, 4196 CrossRef CAS PubMed;
(b) P. Tang, Org. Synth., 2005, 81, 262 CrossRef CAS;
(c) H. Charville, D. Jackson, G. Hodges and A. Whiting, Chem. Commun., 2010, 46, 1813 RSC;
(d) C. L. Allen, R. A. Chhatwal and J. M. J. Williams, Chem. Commun., 2012, 48, 666 RSC.
- L. Zhang, W. Wang, A. Wang, Y. Cui, X. Yang, Y. Huang, X. Liu, W. Liu, J.-Y. Son, H. Ojic and T. Zhang, Green Chem., 2013, 15, 2680–2684 RSC.
-
(a) S. Cho, E. Yoo, I. Bae and S. Chang, J. Am. Chem. Soc., 2005, 127, 16046 CrossRef CAS PubMed;
(b) Z.-W. Chen, H.-F. Jiang, X.-Y. Pan and Z.-J. He, Tetrahedron, 2011, 67, 5920 CrossRef CAS.
-
(a) J. R. Martinelli, T. P. Clark, D. A. Watson, R. H. Munday and S. L. Buchwald, Angew. Chem., Int. Ed., 2007, 46, 8460 CrossRef CAS PubMed;
(b) A. Brennführer, H. Neumann and M. Beller, Angew. Chem., Int. Ed., 2009, 48, 4114 CrossRef PubMed;
(c) T. T. Dang, Y. Zhu, S. C. Ghosh, A. Chen, C. L. L. Chai and A. M. Seayad, Chem. Commun., 2012, 48, 1805 RSC.
-
(a) K. Nakagawa, H. Inoue and K. Minami, Chem. Commun., 1966, 17 RSC;
(b) K. Nakagawa, S. Mineo, S. Kawamura, M. Horikawa, T. Tokumoto and O. Mori, Synth. Commun., 1979, 9, 529 CrossRef CAS.
- J. Shie and J. Fang, J. Org. Chem., 2003, 68, 1158 CrossRef CAS PubMed.
-
(a) E. I. Marks and A. Mekhalfia, Tetrahedron Lett., 1990, 31, 7237 CrossRef;
(b) L. Wang, H. Fu, Y. Jiang and Y. Zhao, Chem.–Eur. J., 2008, 14, 10722 CrossRef CAS PubMed.
- N. W. Gilman, J. Chem. Soc. D, 1971, 733 RSC.
- S. D. Sarkar and A. Studer, Org. Lett., 2010, 12, 1992 CrossRef PubMed.
-
(a) K. Ekoue-Kovi and C. Wolf, Org. Lett., 2007, 9, 3429 CrossRef CAS PubMed;
(b) K. Ekoue-Kovi and C. Wolf, Chem.–Eur. J., 2008, 14, 6302 CrossRef CAS PubMed.
-
(a) H. U. Vora and T. Rovis, J. Am. Chem. Soc., 2007, 129, 13796 CrossRef CAS PubMed;
(b) J. W. Bode and S. S. Sohn, J. Am. Chem. Soc., 2007, 129, 13798 CrossRef CAS PubMed.
- W.-J. Yoo and C.-J. Li, J. Am. Chem. Soc., 2006, 128, 13064 CrossRef CAS PubMed.
-
(a) Y. Tamaru, Y. Yamada and Z. Yoshida, Synthesis, 1983, 474 CrossRef CAS;
(b) T. Naota and S.-I. Murahashi, Synlett, 1991, 693 CrossRef CAS;
(c) A. Tillack, I. Rudloff and M. Beller, Eur. J. Org. Chem., 2001, 523 CrossRef CAS;
(d) W.-K. Chan, C.-M. Ho, M.-K. Wong and C.-M. Che, J. Am. Chem. Soc., 2006, 128, 14796 CrossRef CAS PubMed;
(e) Y. Suto, N. Yamagiwa and Y. Torisawa, Tetrahedron Lett., 2008, 49, 5732 CrossRef CAS;
(f) J. W. W. Chang and P. W. H. Chan, Angew. Chem., Int. Ed., 2008, 47, 1138 CrossRef CAS PubMed;
(g) S. Muthaiah, S. C. Ghosh, J.-E. Jee, C. Chen, J. Zhang and S. H. Hong, J. Org. Chem., 2010, 75, 3002 CrossRef CAS PubMed.
-
(a) S. Seo and T. J. Marks, Org. Lett., 2008, 10, 317 CrossRef CAS PubMed;
(b) C. Qian, X. Zhang, J. Li, F. Xu, Y. Zhang and Q. Shen, Organometallics, 2009, 28, 3856 CrossRef CAS;
(c) J. M. Li, F. Xu, Y. Zhang and Q. Shen, J. Org. Chem., 2009, 74, 2575 CrossRef CAS PubMed.
- S. C. Ghosh, J. S. Y. Ngiam, C. L. L. Chai, A. M. Seayad, D. T. Tuan and A. Chen, Adv. Synth. Catal., 2012, 354, 1407 CrossRef CAS.
-
(a) S. C. Ghosh, J. S. Y. Ngiam, A. M. Seayad, D. T. Tuan, C. L. L. Chai and A. Chen, J. Org. Chem., 2012, 77, 8007–8015 CrossRef CAS PubMed;
(b) R. Cadoni, A. Porcheddu, G. Giacomelli and L. D. Luca, Org. Lett., 2012, 14, 5014–5017 CrossRef CAS;
(c) J. Gu, Z. Fang, Y. Yang, Z. Yang, L. Wan, X. Li, P. Wei and K. Guo, RSC Adv., 2016, 6, 89413–89416 RSC.
-
(a) L. Panahi, M. R. Naimi-Jamal, J. Mokhtari and A. Morsali, Microporous Mesoporous Mater., 2016, 244, 208–217 CrossRef;
(b) S. Akbari, J. Mokhtari and Z. Mirjafary, RSC Adv., 2017, 7, 40881–40886 RSC;
(c) A. Khosravi, J. Mokhtari, M. R. Naimi-Jamal, S. Tahmasebi and L. Panahi, RSC Adv., 2017, 7, 46022–46027 RSC;
(d) S. Tahmasebi, A. Khosravi, J. Mokhtari, M. R. Naimi-Jamal and L. Panahi, J. Organomet. Chem., 2017, 853, 35–41 CrossRef CAS;
(e) J. Mokhtari and B. A. Hassani, Inorg. Chim. Acta, 2018, 482, 726–731 CrossRef CAS;
(f) Z. Ahmadzadeh, J. Mokhtari and M. Rouhani, RSC Adv., 2018, 8, 24203–24208 RSC.
-
(a) C. Reichardt and T. Welton, Solvents and Solvent Effects in Organic Chemistry, WILEY-VCH Verlag GmbH & Co. KGaA, Weinheim, 3rd edn, 2011 Search PubMed;
(b) V. Gutmann, Electrochim. Acta, 1976, 21, 661 CrossRef CAS;
(c) P. Neta, R. E. Huie, P. Maruthamuthu and S. Steenken, J. Phys. Chem., 1989, 93, 7654–7659 CrossRef CAS.
- Y. Yang, J. Gu, Z. Fang, Z. Yang, P. Wei and K. Guo, RSC Adv., 2017, 7, 22797 RSC.
- K. Xu, Y. Hu, S. Zhang, Z. Zha and Z. Wang, Chem.–Eur. J., 2012, 18, 9793 CrossRef CAS.
- F. Minisci, Synthesis, 1973, 1 CAS.
Footnote |
† Electronic supplementary information (ESI) available. See DOI: 10.1039/c9ra04216d |
|
This journal is © The Royal Society of Chemistry 2019 |
Click here to see how this site uses Cookies. View our privacy policy here.