DOI:
10.1039/C9RA04743C
(Paper)
RSC Adv., 2019,
9, 29856-29863
Development of a fused imidazo[1,2-a]pyridine based fluorescent probe for Fe3+ and Hg2+ in aqueous media and HeLa cells†
Received
24th June 2019
, Accepted 15th September 2019
First published on 20th September 2019
Abstract
A new fluorescent sensor 5 based on a fused imidazopyridine scaffold has been designed and synthesized via cascade cyclization. The reaction features the formation of three different C–N bonds in sequence. Imidazopyridine based fluorescent probe 5 exhibits highly sensitive and selective fluorescent sensing for Fe3+(‘turn-on’) and Hg2+(‘turn-off’). The excellent selectivity of imidazopyridine for Fe3+/Hg2+ was not hampered in the presence of any of the competing cations. The limit of detection (LOD) of 5 toward Fe3+ and Hg2+ has been estimated to be 4.0 ppb and 1.0 ppb, respectively, with a good linear relationship (R2 = 0.99). Notably, 5 selectively detects Fe3+/Hg2+ through fluorescence enhancement signalling both in vitro and in HeLa cells.
Introduction
The development of novel and synthetically feasible fluorescent sensors particularly for heavy metal ions (HTMs) is of growing interest because these metal ions are indispensable owing to their involvement in a variety of fundamental biological processes.1 Iron is an essential trace element for both plants and animals, and plays an important role in cellular metabolism and enzyme catalysis.2 On the other hand, mercury is known for its toxic effects, is widely distributed in the environment and bioaccumulates through the food chain.3 To serve the purpose of sensing, different small molecule fluorescent probes have been developed for selective detection of iron (Fe3+)4 and mercury (Hg2+).5,6 Further, reports dealing with the detection of HTMs under aqueous conditions or in a viable aqueous-ethanol media are rather scarce.7–9
In addition, imidazo[1,2-a]pyridine ring systems have fascinated a great deal of attention due to their biological activities, associated with the hybrid structure of imidazole and pyridine.10 Imidazo[1,2-a]pyridine ring systems are also important in the field of material sciences,11 because a few of the compounds belong to this class exhibit excited state intramolecular proton transfer (ESIPT) behavior.12 Therefore, a large number of methodologies have been developed for the synthesis of imidazo[1,2-a]pyridine derivatives.13 The immense applications of this class of compounds also prompted us to explore the synthetic methodologies for the preparation of fused imidazo[1,2-a]pyridines. Following our interest in the area of designing and synthesis of small bioactive molecule via ecofriendly route,14 we report herein the synthesis, characterization and application of fused imidazopyridine compound for the detection of Fe3+ and Hg2+ in aqueous-alcoholic media. Notably, the systematic fluorescence intensity change can be associated to the detection ability for in vitro measurements however, sensing of analytes in cellular systems (inside biological cells) is a daunting task.6b Further, variation in fluorescence intensity in cellular systems may occur due to several other reasons.6b,15,16
Results and discussion
The imidazo[1,2-a]pyridines probe (5) was synthesized in three steps with overall yield 63%. Formylation of 4-methoxyacetophenone furnished 3-chloro-3-(4-methoxyphenyl) acrylaldehyde (2).17 Sonogashira coupling of 2 with phenyl acetylene (3a) gives 2-(2-phenylethynyl) benzaldehyde (4).18
Condensation of o-phenylenediamine with 4 in DMF in presence of acetic acid under refluxing condition resulted in an oxidative cyclization to give desired probe 3-(4-methoxyphenyl)-1-phenylbenzo[4,5]imidazo[1,2-a]-pyridine, (5) (Scheme 1). Characterization of 5 has been done by NMR (1H & 13C), HRMS and single X-ray single crystal analysis. It is apparent from the mechanism that formation of 5 takes place by condensation cum cyclization as shown below (Scheme 2). Imine formation followed by attack of amine nitrogen on imine carbon which leads to dihydrobenzimidazole (4b). Nitrogen atom of dihydrobenzimidazole attacks on alkynyl carbon as shown to obtain dihydrobenzimidazo pyridine (4d) after protonation. Oxidation of dihydrobenzimidazo pyridine (4d) yielded benzimidazo pyridine (5).
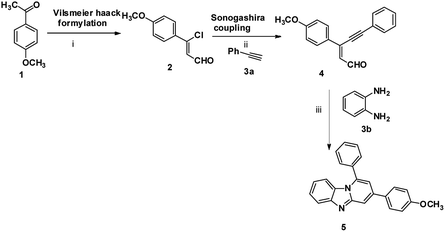 |
| Scheme 1 Synthesis of imidazopyridine: (i) POCl3, DMF, CH3COONa, 0 °C to rt, 3 h; (ii) PdCl2(PPh3)3, Et3N, CH3CN, rt, 5 h; (iii) DMF, acetic acid, 100 °C, 12 h, 63%. | |
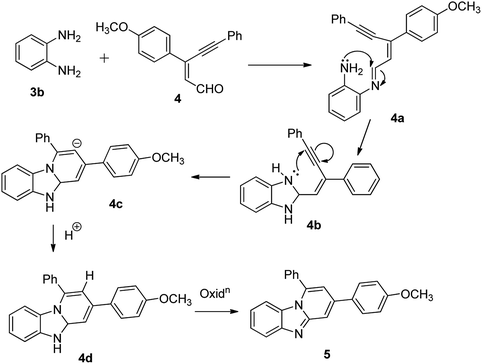 |
| Scheme 2 Proposed mechanism for the formation of 5. | |
Structural descriptions
The structure of 5 has been authenticated by X-ray single crystal analysis (CCDC no. 1921596,† Fig. 1). It crystallizes in monoclinic system with space group ‘P121/n1’. The molecular planarity is an important factor for fluorescent probes. The molecular plane of 5 comprises imidazo[1,2-a]pyridine fluorophore whereas p-methoxyphenyl ring assumes twisted position in a way that half part lying above and half part lying below the molecular plane. The molecular plane also divides 1-phenyl substituent into two equal halves. The C–N, C–O and C–C bond lengths are within the normal ranges.7 The twisting in p-methoxyphenyl ring measured through torsion angles calculated to be −27.70° (cisoid, C10–C9–C18–C23) and 154.80° (transoid, C10–C9–C18–C19). The phenyl ring assumes orientation perpendicular to the central pyridine ring. Probe 5 leads to the formation of 1D chain through edge-to-edge π⋯π stacking interaction which enables it to be a good candidate for dimer formation in the excited state (Fig. 1 and S7†).
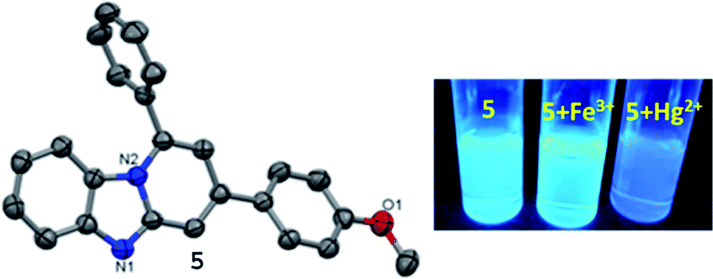 |
| Fig. 1 (Left) X-ray single crystal structure of 5 (ellipsoidal probability 50%); (right) fluorescence image of 5 + Fe3+ and 5 + Hg2+ under UV-light (365 nm). | |
Cation detection through absorption and fluorescence studies
The UV/vis spectrum of 5 displays absorption bands at 365, 326, 285 and 244 nm (pH, 7.2 in H2O
:
EtOH, 8
:
2, v/v, at rt) (Fig. 2a). The low energy (LE) bands are assignable to the n–π* transitions and high energy bands may be attributed to the π–π* transitions.7 Further, to assess the cation binding effect on absorption behavior of 5, various alkali, alkaline and transition metal ions (5 equiv.; Na+, K+, Mg2+, Ca2+, Mn2+, Fe2+, Fe3+, Co2+, Ni2+, Zn2+, Ag+, Cd2+ and Pb2+; 10 mM) were added to the solutions of 5 (10 μM; 3 mL). Among tested cations, only Fe3+ and Hg2+ induced significant changes in the spectral feature of 5 (Fig. S8†).
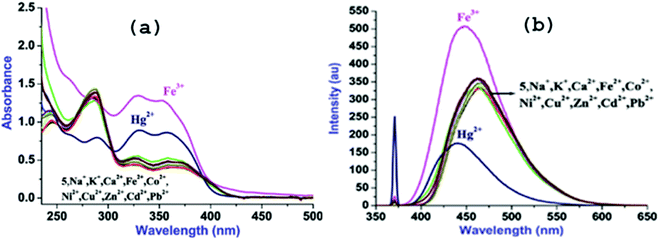 |
| Fig. 2 UV/vis (a) and fluorescence (b) plots of 5 (10 μM, H2O–EtOH, 8 : 2, v/v) in presence of various cations. | |
Addition of Fe3+ leads to the large hyperchromic shifts in both the LE bands however; hyperchromic shifts in the HE bands are relatively small to that of LE bands. In contrast, addition of Hg2+ to the solution of 5 causes ratiometric changes in the absorption bands through hyperchromic shifts in the LE bands and hypochromic shift in HE band along with appearance of a new band at 264 nm.
Considering substantial changes in the presence of Fe3+ and Hg2+ as well as to have deep insight about probe-cation binding, UV/vis titration studies were performed (Fig. 3). Gradual addition of Fe3+(1.0 μL, 0.1 equiv.) to a solution of 5 leads to the noteworthy ratiometric change in the absorption bands through hyperchromic shift in the LE bands and hypochromic shift in the HE band (at 285 nm; ε, 22
769 M−1 cm−1) (Fig. 3). Further, introducing more concentration of Fe3+(2.0–15.0 μL) to the solution of 5 caused incessant hyperchromic shift in both the LE bands whereas HE bands become poorly structured with hyperchromic shifts at 244 nm and hypochromic shifts at 285 nm. Likewise, aliquot addition of Hg2+(1.0 μL, 0.1 equiv.) to a solution of 5 induced significant ratiometric changes via hyperchromic shift at both the LE bands and hypochromic shift at 285 nm (ε, 31
686 M−1 cm−1) whereas HE band at 244 nm is blue shifted to appear at 236 nm (Fig. 3). Further additions of Hg2+ (2.0–15.0 μL) lead to the similar ratiometric changes along with clear cut isosbestic points at 392, 306 and 245 nm, suggesting presence of more than two species in the medium. In addition, selectivity of 5 toward Fe3+ and Hg2+ was examined by introduction of Fe3+ (20 μL) to the solution of 5 followed by addition of Hg2+ (20 μL) to the same 5 + Fe3+ solution and the resultant 5 + Fe3+ + Hg2+ solution was left as such for an hour. Notably, spectral pattern of 5 + Fe3+ was almost completely altered to 5 + Hg2+ which strongly suggests better selectivity of 5 toward Hg2+ over Fe3+.
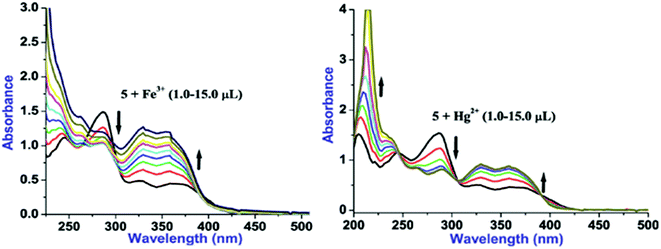 |
| Fig. 3 UV/vis titration plots of 5 (10 μM, H2O–EtOH, 8 : 2, v/v) with Fe3+ (left) and Hg2+ (right) ions. | |
Upon excitation at its LE band (λex, 368 nm), 5 exhibits moderate fluorescence at 464 nm and the quantum yield (ΦF) determined to be 0.37 (Fig. 2b).8 The moderate ΦF for 5 may be ascribed to the combined effect of intense LE absorption band and photoinduced electron transfer (PET).8 Cation interaction behavior of 5 was also investigated through fluorescence spectral technique by individual and excessive addition of various cations (10 equiv.; 100 mM) to the solution of 5. Notably, among introduced cations, only Fe3+ and Hg2+ could cause significant fluorescence change whereas presence of other cations was futile in terms of the spectral change (Fig. 2b) which can be seen in the images taken both under natural conditions and UV-light (Fig. S8 ESI†).
Additions of both Fe3+ and Hg2+ lead to the subtle blue shift (820 cm−1 for Fe3+ and 1124 cm−1 for Hg2+) however, Fe3+ induces ‘turn-on’ fluorescence and Hg2+ causes ‘turn-off’ fluorescence. To get profound idea into probe-cation binding, fluorescence titration studies were performed by gradual additions of Fe3+ and Hg2+ separately to the solution of 5 (Fig. 4). Aliquot additions of Fe3+ (1.0–15.0 μL) exhibited ‘turn-on’ fluorescence (∼33%) with blue shifted emission maxima to appear at 447 nm. Likewise, gradual introduction of Hg2+(1.0–15.0 μL) displayed ‘turn-off’ emission (∼59%) with hypsochromically shifted fluorescence maxima to emerge at 441 nm. Blue shifts in case of both Fe3+ and Hg2+ suggest that probe 5 may be interacting with these cations through same binding site. Further, the ‘turn-on’ fluorescence of 5 upon binding with Fe3+ may be attributed to an increase in charge transfer from imidazo nitrogen of 5 to Fe3+ and/or due to chelation enhanced fluorescence (CHEF).8 In contrast, ‘turn-off’ signaling with Hg2+ may be ascribed to the spin orbit coupling and/or the chelation-enhanced quenching (CHEQ) effect.9
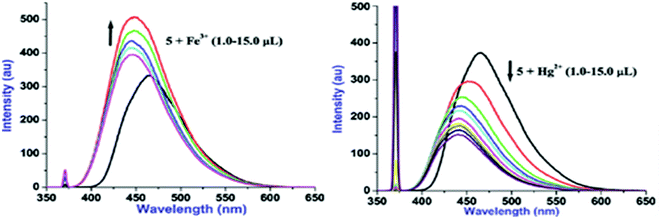 |
| Fig. 4 Fluorescence titration plots of 5 (10 μM, H2O–EtOH, 8 : 2, v/v) with Fe3+ (a) and Hg2+ (b) ions. | |
The Job's plot analysis revealed 1
:
1 probe-cation stoichiometry for Fe3+ whereas 2
:
1 for Hg2+ (Fig. S9; ESI†). The quenching constant (Ksv) for 5 + Hg2+ has been determined to be 1.52 × 104 M−1 derived from the Stern–Volmer plot (Fig. S10; ESI†). Using Benesi–Hildebrand method, the association constants (Ka) for 5 + Fe3+ and 5 + Hg2+ were calculated to be 1.09 × 105 M−1 and 1.31 × 105 M−1, respectively (Fig. S11; ESI†).19 Moreover, the fluorescent detection of cations can only be claimed if competitive analytes induce insignificant interference to the selectivity assured by probe, therefore, we examined two sequences of interference: (1) various cations were successively added to the solution containing complex 5 + Fe3+ and/or 5 + Hg2+; (2) Fe3+ and/or Hg2+ were added separately into the solution containing 5 + Mn+ (M = various tested cations; (Fig. 5). Notably, none of the other metal ions could interfere to the selectivity of 5 toward Fe3+ and Hg2+. Moreover, 5 offer greater selectivity for Hg2+ over Fe3+. Other trivalent cations like Cr3+ and Al3+ could also not affect the selectivity assay of 5.
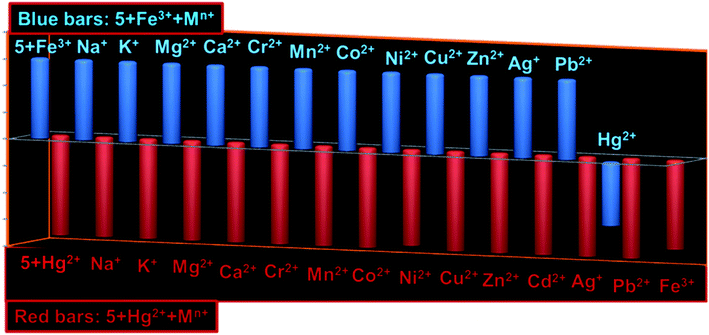 |
| Fig. 5 Bar diagrams showing interference of various competing cations with fluorescence of 5 + Fe3+ (blue bars) and 5 + Hg2+ (brown bars). | |
The sensitivity of 5 (1.0 μM) toward Fe3+ and Hg2+ was examined in the concentration range of 10−12 M to 10−6 M wherein both the cations perturbed the fluorescence intensity of 5 at a concentration of 10−9 M. To further get better accuracy, 10−9 M (1.0 × 10−9 − 9.0 × 10−9 M) concentration of Fe3+ and/or Hg2+ were introduced to the solution of 5 (1.0 μM) and displayed good plot linearity (R2 = 0.99) with limit of detection (LOD) of 4.0 ppb (Fe3+) and 1.0 ppb (Hg2+) (Fig. S12; ESI†). It suggests that 5 can potentially be used as probe for practical detection of Fe3+ and Hg2+. Further, 5 exhibited almost complete fluorescence revival upon excessive addition of EDTA (5.0 equivalent) to the solution of 5 + Fe3+/Hg2+ suggesting that probe 5 can serve as reversible probe for said cations.
1H NMR titration studies
The information about binding site of 5 for Fe3+ and Hg2+ cations was obtained though 1H NMR titration studies (Fig. 6a) which show significant downfield shift in the protons adjacent to imidazo-nitrogen both in presence of Fe3+ and Hg2+. Addition of Fe3+ (2.0 μL) to the solution of 5 led to the downfield shift in the aromatic protons H2 and H1 to appear at 8.11 ppm (Δδ = 0.27 ppm) and 7.94 ppm (Δδ = 0.10 ppm), respectively (Fig. 6a). Further addition of Fe3+ (4.0 μL) caused incessant downfield shift in H2 and H1 protons to resonate at 8.15 ppm (Δδ = 0.31 ppm) and Fe3+ and 8.07 ppm (Δδ = 0.23 ppm), respectively. Increasing the concentration of Fe3+ (4.0 μL) to the solution induced insignificant downfield shift to the resonances of 5. Likewise, addition of Hg2+ (2.0 μL) to the solution of 5 resulted in the downfield shift to H2 and H1 aromatic protons to resonate at 8.02 ppm (Δδ = 0.18 ppm) and 7.89 ppm (Δδ = 0.05 ppm), respectively (Fig. 6a). Further additions of Hg2+ (4.0 μL) induced large downfield shift in H2 proton to appear at 8.29 ppm (Δδ = 0.45 ppm) whereas H1 proton resonates at 8.00 ppm (Δδ = 0.16 ppm). It is noteworthy to mention that –OCH3 proton exhibits insignificant downfield shift in presence of Fe3+/Hg2+, which rules out the binding of cations through methoxy oxygen site. Overall, 1H NMR titration spectra clearly displayed larger downfield shift for H2 proton (adjacent to the nitrogen donor site) relative to other aromatic protons and thereby strongly suggests imidazo-nitrogen as m downfield shift in presence of Fe3+/Hg2+, which rules out the binding of cations through methoxy oxygen site. Overall, 1H NMR titration spectra clearly displayed larger downfield shift for H2 proton (adjacent to the nitrogen donor site) relative to other aromatic protons and thereby strongly suggests imidazo-nitrogen as most probable binding site for both the metal ions.
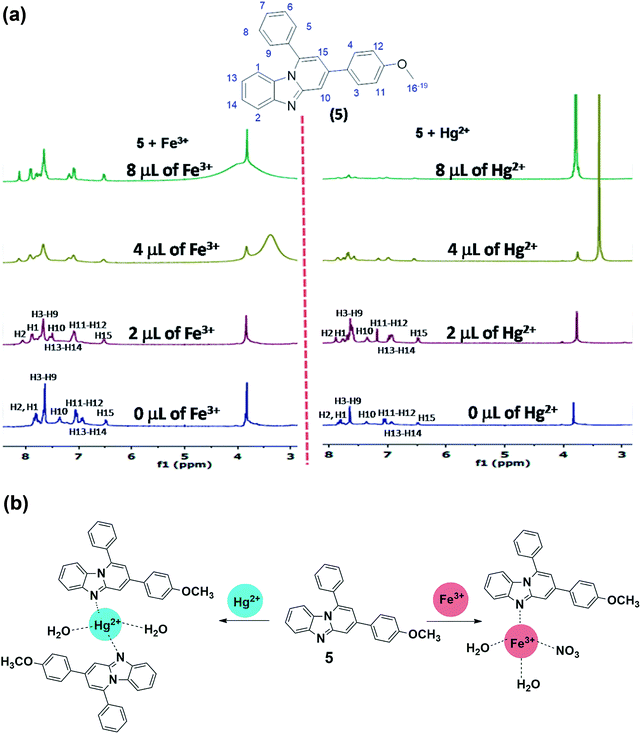 |
| Fig. 6 (a) 1H NMR titration plot of 5 with Fe3+ and Hg2+; (b) Binding of probe 5 with Fe3+ and Hg2+ in aqueous-ethanol media. | |
ESI-mass spectral studies
Further to get better idea about the complex ensued from probe-cation binding, ESI-MS spectra of compound obtained upon treatment of 5 with Hg2+ and Fe3+ salts, were acquired (Fig. S13; ESI†). The 5 + Hg2+ complex exhibits a peak at m/z 469 which corresponds to the half of the [Hg(5)2(H2O)2]2+ entity having at molecular mass of 938. As the charge (z) in Hg(II)-complex is +2, it displays [M]+/2 peak at m/z 469 (938/2). Although, the base peak of Hg(II) complex is in accordance with the [M + 1]+ peak of probe 5 (m/z,350). Likewise, 5 + Fe3+ complex shows moderately abundant [M]+/2 peak at m/z 252 which is in accordance with half of the formula [Fe(5)(NO3)(H2O)2]2+ which have molecular mass of 504. Overall, the ESI-MS spectra also supported the probe-cation binding and stoichiometry (Fig. 6b).
Imaging in HeLa cells
To evaluate the feasibility of Fe3+ and Hg2+ sensing by 5 inside the cells, the HeLa cells were incubated with different concentration of the respective ions for 10 min. The phase contrast and blue channel images of the fixed cells after probing with sample is shown in Fig. 7. Overlay images showed that the blue fluorescence coincides with the cells. The blue channel displayed an increase in fluorescence with increasing concentration of both Fe3+ and Hg2+ and the same has been illustrated in the mean fluorescence intensity plot (Fig. S14 and S15; ESI†). Additionally, the cell morphology also indicated cell survival after 10 min of incubation with the respective ions. The sharp contrast in the confocal study indicates the efficient cellular uptake and micromolar sensing of Fe3+ and Hg2+ by 5.
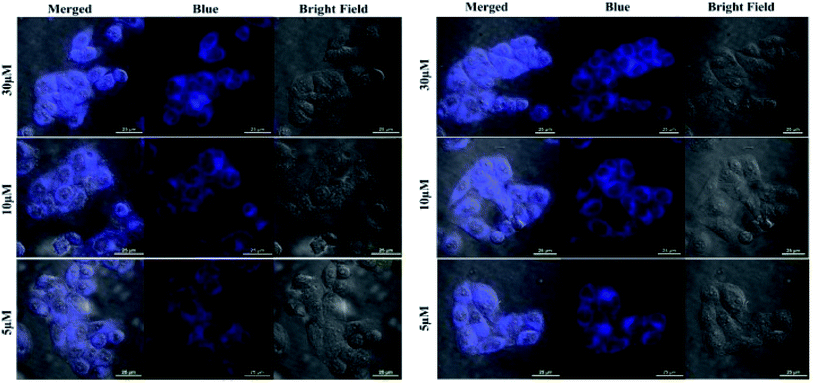 |
| Fig. 7 Confocal images showing Fe3+ (left) and Hg2+ (right) sensing by 5 inside HeLa cells. The cells were incubated with increasing concentration of the respective ions. | |
In summary, a fused imidazopyridine has been synthesized, thoroughly characterized and used as sensitive and highly selective fluorescent sensor for biologically and environmentally vital Fe3+ and Hg2+ through distinct fluorescence readout. Probe 5 was designed with the view point of having single open imidazolyl nitrogen as unique interaction site for cations so that none of the competing cation can interfere with its selectivity assay. The cation binding has been well examined and supported by UV/vis, fluorescence, 1H NMR titration techniques and mass spectral studies. The ppb level sensitivity and quite high selectivity of 5 toward Fe3+ and Hg2+ strongly claimed its practical application for determination of Fe3+ and Hg2+ in biological/environmental samples. Eventually, imaging in HeLa cells incubated with 5 has revealed enhanced fluorescence for Fe3+ and Hg2+ ions which strongly supports the claim that 5 can potentially be used as fluorescent probe for these cations under cellular conditions.
Conflicts of interest
There are no conflicts to declare.
Acknowledgements
SS and RP acknowledge the Department of Science and Technology (DST) for financial support through Inspire Faculty Award No IFA-13-CH-123 and IFA12-CH-66 respectively. NG acknowledges Ramanujan Fellowship from SERB, India (SB/S2/RJN-072/2015) and BioX center and AMRC, IIT Mandi for providing facilities.
Notes and references
-
(a) K. P. Carter, A. M. Young and A. E. Palmer, Chem. Rev., 2014, 114(8), 4564–4601 CrossRef CAS PubMed
;
(b) N. D. Acha, C. Elosúa, J. M. Corres and F. J. Arregui, Sensors, 2019, 19(3), 599–633 CrossRef PubMed
;
(c) B. Kaur, N. Kaur and S. Kumar, Coord. Chem. Rev., 2018, 358, 13–69 CrossRef CAS
. -
(a) R. Meneghini, Free Radical Biol. Med., 1997, 23, 783–792 CrossRef CAS
;
(b) P. Aisen, M. Wessling-Resnick and E. A. Leibold, Curr. Opin. Chem. Biol., 1999, 3, 200–206 CrossRef CAS PubMed
;
(c) R. S. Eisenstein, Annu. Rev. Nutr., 2000, 20, 627–662 CrossRef CAS PubMed
;
(d) T. A. Rouault, Nat. Chem. Biol., 2006, 2, 406–414 CrossRef CAS PubMed
. -
(a) F. D. Natale, A. Lancia, A. Molino, M. D. Natale, D. Karatza and D. Musmarra, J. Hazard. Mater., 2006, 132, 220–225 CrossRef PubMed
;
(b) C. M. L. Carvalho, E.-H. Chew, S. I. Hashemy, J. Lu and A. Holmgren, J. Biol. Chem., 2008, 283, 11913–11923 CrossRef CAS PubMed
;
(c) W. F. Fitzgerald, C. H. Lamborg and C. R. Hammerschmidt, Chem. Rev., 2007, 107, 641–662 CrossRef CAS PubMed
. -
(a) L. Zhang, J. Wang, J. Fan, K. Guo and X. Peng, Bioorg. Med. Chem. Lett., 2011, 21, 5413–5416 CrossRef CAS PubMed
;
(b) J. Yao, W. Dou, W. Qin and W. Liu, Inorg. Chem. Commun., 2009, 12, 116–118 CrossRef CAS
;
(c) Z.-X. Li, L.-F. Zhang, W.-Y. Zhao, X.-Y. Li, Y.-K. Guo, M.-M. Yu and J.-X. Liu, Inorg. Chem. Commun., 2011, 14, 1656–1658 CrossRef CAS
;
(d) L.-J. Fan and W. E. Jones Jr, J. Am. Chem. Soc., 2006, 128, 6784–6785 CrossRef CAS PubMed
;
(e) N. C. Lim, S. V. Pavlova and C. Bruckner, Inorg. Chem., 2009, 48, 1173–1182 CrossRef CAS PubMed
;
(f) Y. Xiang and A. Tong, Org. Lett., 2006, 8, 1549–1552 CrossRef CAS PubMed
. -
(a) H. N. Kim, W. X. Ren, J. S. Kim and J. Yoon, Chem. Soc. Rev., 2012, 41, 3210–3244 RSC
and references therein;
(b) X. Chen, T. Pradhan, F. Wang, J. S. Kim and J. Yoon, Chem. Rev., 2012, 112, 1910–1956 CrossRef CAS PubMed
and references therein;
(c) H. N. Kim, M. H. Lee, H. J. Kim, J. S. Kim and J. Yoon, Chem. Soc. Rev., 2008, 37, 1465–1472 RSC
and references therein. -
(a) J. Du, J. Fan, X. Peng, P. Sun, J. Wang, H. Li and S. Sun, Org. Lett., 2010, 12, 476–479 CrossRef CAS PubMed
;
(b) S. Madhu, D. K. Sharma, S. K. Basu, S. Jadhav, A. Chowdhury and M. Ravikanth, Inorg. Chem., 2013, 52, 11136–11145 CrossRef CAS PubMed
;
(c) X. Zhang, Y. Shiraishi and T. Hirai, Tetrahedron Lett., 2008, 49, 4178–4181 CrossRef CAS
. -
(a) R. Pandey, G. Méhes, A. Kumar, R. K. Gupta, C. Adachi and D. S. Pandey, Chem. Commun., 2014, 50, 8032–8035 RSC
;
(b) R. Pandey, M. Yadav, M. Shahid, A. Misra and D. S. Pandey, Tetrahedron Lett., 2012, 53, 3550–3555 CrossRef CAS
. -
(a) T. Nandhini, P. Kaleeswaran and K. Pitchumani, Sens. Actuators, B, 2016, 230, 199–205 CrossRef CAS
;
(b) R. Pandey, P. Kumar, A. K. Singh, M. Shahid, P.-z. Li, S. K. Singh, Q. Xu, A. Misra and D. S. Pandey, Inorg. Chem., 2011, 50, 3189–3197 CrossRef CAS PubMed
;
(c) S. A. de Silva, A. Zavaleta, D. E. Baron, O. Allam, E. V. Isidor, N. Kashimura and J. M. Percarpio, Tetrahedron Lett., 1997, 38, 2237–2240 CrossRef CAS
. -
(a) K. M. Vengaian, C. D. Britto, K. Sekar, G. Sivaraman and S. Singaravadivel, RSC Adv., 2016, 6, 7668–7673 RSC
;
(b) N. Thakur, M. D. Pandey and R. Pandey, New J. Chem., 2018, 42, 3582–3592 RSC
;
(c) R. Pandey, R. K. Gupta, M. Shahid, B. Maiti, A. Misra and D. S. Pandey, Inorg. Chem., 2012, 51, 298–311 CrossRef CAS PubMed
. -
(a) F. Couty and G. Evano, in Comprehensive Heterocyclic Chemistry III, ed. A. R. Katritzky, C. A. Ramsden, E. F. V. Scriven and R. J. K. Taylor, Elsevier, Oxford, 2008, vol. 11, p. 409 Search PubMed
;
(b) C. Enguehard-Gueiffier and A. Gueiffier, Mini-Rev. Med. Chem., 2007, 7, 888–899 CrossRef CAS PubMed
. - N. Shao, G. X. Pang, C. X. Yan, G. F. Shi and Y. Cheng, J. Org. Chem., 2011, 76, 7458–7465 CrossRef CAS PubMed
. - A. J. Stasyuk, M. Banasiewicz, M. K. Cyrański and D. T. Gryko, J. Org. Chem., 2012, 77, 5552–5558 CrossRef CAS PubMed
. -
(a) A. K. Bagdi, S. Santra, K. Monir and A. Hajra, Chem. Commun., 2015, 51, 1555–1575 RSC
;
(b) A. K. Bagdi and A. Hajra, Chem. Rec., 2016, 16(4), 1868–1885 CrossRef CAS PubMed
. -
(a) A. Kumar, S. Srivastava and G. Gupta, Green Chem., 2012, 14, 3269–3272 RSC
;
(b) A. Kumar, S. Srivastava, G. Gupta, V. Chaturvedi, S. Sinha and R. Srivastava, ACS Comb. Sci., 2011, 13, 65–71 CrossRef CAS PubMed
;
(c) A. Kumar, S. Srivastava and G. Gupta, Tetrahedron Lett., 2010, 51, 517–520 CrossRef CAS
;
(d) A. Kumar, S. Srivastava, G. Gupta, P. Kumar and J. Sarkar, RSC Adv., 2013, 3, 3548–3552 RSC
;
(e) S. Srivastava, New J. Chem., 2019, 43, 6469–6471 RSC
;
(f) H. Sharma and S. Srivastava, RSC Adv., 2018, 8, 38974–38979 RSC
. -
(a) H. Giloh and J. W. Sedat, Science, 1982, 217, 1252–1255 CrossRef CAS PubMed
;
(b) D. Sinnecker, P. Voigt, N. Hellwig and M. Schaefer, Biochemistry, 2005, 44, 7085–7094 CrossRef CAS PubMed
;
(c) J. N. Henderson, H.-W. Ai, R. E. Campbell and S. J. Remington, Proc. Natl. Acad. Sci. U. S. A., 2007, 104, 6672–6677 CrossRef CAS PubMed
;
(d) N. Periasamy, S. Bicknese and A. S. Verkman, Photochem. Photobiol., 1996, 63, 265–271 CrossRef CAS
. -
(a) T. E. Kaiser, V. Stepanenko and F. Würthner, J. Am. Chem. Soc., 2009, 131, 6719–6732 CrossRef CAS PubMed
;
(b) K.-R. Wang, D.-S. Guo, B.-P. Jiang, Z.-H. Sun and Y. Liu, J. Phys. Chem. B, 2010, 114, 101–106 CrossRef CAS PubMed
. - P. H. J. Kouwer, W. F. Jager, W. J. Mijs and S. J. Picken, Macromolecules, 2002, 35, 4322–4329 CrossRef CAS
. -
(a) M. Gazvoda, M. Virant, B. Pinter and J. Košmrlj, Nat. Commun., 2018, 9, 4814–4822 CrossRef PubMed
;
(b) A. Chandra, B. Singh, S. Upadhyay and R. M. Singh, Tetrahedron, 2008, 64, 11680–11685 CrossRef CAS
. -
(a) K. A. Connors, Binding Constants, New York, Wiley, 1987 Search PubMed
;
(b) S. H. Mashraqui, T. Khan, S. Sundaram, R. Betkar and M. Chandiramani, Tetrahedron Lett., 2007, 48, 8487–8490 CrossRef CAS
.
Footnote |
† Electronic supplementary information (ESI) available. CCDC 1921596. For ESI and crystallographic data in CIF or other electronic format see DOI: 10.1039/c9ra04743c |
|
This journal is © The Royal Society of Chemistry 2019 |
Click here to see how this site uses Cookies. View our privacy policy here.