DOI:
10.1039/C9RA04776J
(Paper)
RSC Adv., 2019,
9, 36825-36830
A fluorescence polarization immunoassay for detection of thiacloprid in environmental and agricultural samples†
Received
25th June 2019
, Accepted 1st November 2019
First published on 12th November 2019
Abstract
As a widely used neonicotinoid insecticide, thiacloprid has been observed to pose a risk to honeybees and the endocrine system of mammals. So a detection method with high sensitivity, simple operation and high throughput is required. Based on this consideration, we prepared an anti-thiacloprid monoclonal antibody (mAb, C9) and developed a fluorescence polarization immunoassay (FPIA) for the detection of thiacloprid. After optimizing the length of spacer and reaction conditions, the 50% inhibition concentration (IC50), limit of detection (LOD) and linear range (IC20 ∼ IC80) of the FPIA are 15.34 ng mL−1, 2.43 ng mL−1 and 3.10–65.7 ng mL−1, respectively. Meanwhile, FPIA just requires 12 min to detect the pesticide with simple operation. Then the FPIA was used to detect the thiacloprid in spiked rice, soil, cucumber and tomato samples, and recoveries were in the range of 79.1%–105.3% with 3.7%–12.3% standard deviation. The FPIA also shows good correlation with high-performance liquid chromatography for the detection of thiacloprid in tomato samples.
1. Introduction
Thiacloprid, a neonicotinoid insecticide, acts on the acetylcholine receptor of insects and causes large accumulation of acetylcholine, and thus leads to the death of insects.1 It can effectively control sucking and chewing pests, and has been widely used with vegetables, fruit and alimentary crops. Some neonicotinoid insecticides containing a nitro group (thiamethoxam, imidacloprid and clothianidin) have been temporarily prohibited by the European Commission because of the high toxicity to honeybees.2 Although thiacloprid (containing a cyano group that shows lower toxicity) can still be used in flowering crops, it also affects the behavior of honeybees and has been observed to pose a risk to the endocrine system of mammals.3–5 So, it is necessary to detect and monitor thiacloprid residues in environmental and agricultural products.
Many instrument-based methods have been established to detect thiacloprid in various samples, such as high-performance liquid chromatography (HPLC), HPLC-tandem mass spectrometry, supercritical fluid chromatographic-tandem mass spectrometry and so on.6–8 However, immunoassay, with the advantages of simplicity, high sensitivity and low cost, shows greater advantage and potential than instrument-based methods in analysis of large number of samples,9 which has been used for detection of thiacloprid. Liu et al. prepared the anti-thiacloprid polyclonal antibody to develop enzyme-linked immunosorbent assay (ELISA),10 and used various tracers to enhanced the sensitivity, such as enhanced chemiluminescent enzyme immunoassay and time-resolved fluoroimmunoassay.11,12 Yin et al. prepared monoclonal antibody (mAb) and phage-displayed peptide competitor of thiacloprid to develop phage ELISA.13 It is noticed that these assays all depend on the solid phase platform, which require the steps of “coating-blocking-binding-washing-detection”. The immunoassay procedures may be further simplified for high-throughput and rapid detection.
Fluorescence polarization (FP) was applied to immunoassay in 1960s and has been widely used for detection of small molecules recent years.16,17 When the temperature and viscosity of the solution remain constant, the FP value is only related to the size of the fluorescent molecule. Smaller size of fluorescent molecule causes faster rotation speed, which means lower FP value. In fluorescence polarization immunoassay (FPIA), with the increase of the concentration of analyte, the decrease of fluorescent tracer-antibody complex would cause the decrease in FP value of the system, which can be detect directly without washing and separation. Compared with solid phase-based immunoassay, FPIA offers simpler analysis procedures and shorter analysis time. Furthermore, due to the one-step detection and ratiometric technique, the FP value is less prone to interference from operational errors and inner-filter effects so that the result is stable and shows good reproducibility.18–20 Besides, the development of portable fluorescence polarization detector makes FPIA more potential in pesticide residue detection.21–23
In this work, we prepared an anti-thiacloprid mAb (mAb, C9) with high sensitivity, and labeled thiacloprid hapten with fluorescein isothiocyanate (FITC) to develop FPIA for detection of thiacloprid. Under the optimal condition, the standard curve of FPIA for detection of thiacloprid was established. The performances of FPIA to detect thiacloprid in agricultural and environmental samples were evaluated, and the accuracy of FPIA was validated by HPLC.
2. Materials and methods
2.1 Reagents
The hapten of thiacloprid, immunogen and coating antigen were prepared as described previously.10 Thiacloprid and other pesticides for cross-reactivity were purchased from Jiangsu Pesticide Research Institute Co., Ltd (Nanjing, China). The reagents for cell culture were purchased from Invitrogen (Carlsbad, USA). Horseradish peroxidase (HRP)-labeled goat anti-mouse IgG was product of Boster Biological Technology Co., Ltd (Pleasanton, USA). Ethanediamine (EDA) and 1,6-hexanediamine (HMD) were purchased from Sinopharm Chemical Reagent Co., Ltd (Shanghai, China). Other reagents were purchased from Sigma-Aldrich Chemical Co., Ltd (St. Louis, USA). GF-254 thin-layer chromatography silica gel was purchased from Qingdao Ocean Chemical Co., Ltd (Qingdao, China). The protein A HP column for antibody purification was purchased from GE Healthcare (Piscataway, USA). The microtiter plates were product of Corning Incorporated (Corning, USA). The BALB/c mice were purchased from Yangzhou University (Yangzhou, China). All animals used in this study and animal experiments were approved by Department of Science and Technology of Jiangsu Province. The license number was SYXK (SU) 2017-0007.
2.2 Preparation of mAb
The immune procedure of six-week-old female BALB/c mice was based on previous report.13 Briefly, the mice were firstly immunized by immunogen (100 μg per mouse) mixed with equal volume Freund's complete adjuvant. After three weeks, the mice were subsequently immunized by immunogen (100 μg per mouse) mixed with equal volume Freund's incomplete adjuvant for four times at 2 week intervals. One week after the last immunization, the mice tail blood were collected to determine whether anti-thiacloprid antibodies were produced using indirect competitive ELISA (ic-ELISA), and the mouse with the best performances (titer and sensitivity) was injected with 100 μg immunogen in phosphate-buffered saline (PBS). After three days, the mouse spleen lymphocytes cells were fused with SP2/0 myeloma cells. Fourteen days after cell fusion, the cell culture supernatants were collect to screen the positive wells that can specifically recognize thiacloprid using ic-ELISA. The positive wells were cloned by the limited dilution method until the monoclonal cell line was obtained that can stably secret anti-thiacloprid antibody. Six-week-old female BALB/c mice were injected with sterilized liquid wax (0.5 mL per mouse). One week after injection, the monoclonal cells (2 × 106 cells per mouse) were injected to abdominal cavity of mouse to produce ascites containing anti-thiacloprid mAb. The mAb was purified by the protein A column.
2.3 Evaluation of mAb
The anti-thiacloprid antibody was evaluated by ic-ELISA. The microtiter plate were coated with coating antigen in buffer bicarbonate (CBS) at 0.5 μg mL−1 (100 μL per well) by incubation for overnight at 4 °C. After 5 times washing by PBS containing 0.05% Tween 20 (PBST), the plate were blocked with 3% skimmed milk (300 μL per well) in PBS for 1 h at 37 °C. After washing, 50 μL thiacloprid standard solutions mixed with equal volumn mAb (5 μg mL−1) in PBS were added to the wells and incubated for 1 h at 37 °C. After 5 times washing, 100 μL of HRP-labeled goat anti-mouse IgG antibody (1:20000 dilution in PBST) was added to bind the anti-thiacloprid mAb on the plate for 1 h at 37 °C. After another washing, 100 μL of HRP substrate was added, and stopped by addition of 2 mol L−1 H2SO4 (50 μL per well) after incubation of 15 min. The absorbance at 450 nm was measured by a Spectra-Max M5 reader (Molecular Devices).
2.4 Synthesis of fluorescent tracer-labeled hapten
The synthetic route was showed in Fig. S1.† The spacer compound (EDA or HMD, 1.5 μmol) was dissolved in 5 mL methanol solution with 1% triethylamine. The FITC was slowly dropped to the solution under magnetic stirring and reacted for 1 h. After the incubation in dark for overnight, the precipitated fluorescent tracer (EDF/HMDF) was dry at room temperature. Then, 80 μmol NHS and 80 μmol DCC were added to 0.5 mL DMF containing 40 μmol thiacloprid hapten with stirring. After the reaction for overnight under stirring, the supernatant was collected and reacted with 10 μmol fluorescent tracer for 4 h. The reaction product was obtained after centrifugation.
The fluorescent tracer-labeled haptens (THI-EDF and THI-HMDF) were purified by thin layer chromatography (TLC). The yellow bands with different rate of flow (Rf) were collected and crushed into powder. The compounds in the silica gel were eluted with methanol and concentrated at room temperature by nitrogen blowing.
2.5 Development of FPIA
One hundred milliliter thiacloprid standard solutions or sample solutions mixed with 50 μL fluorescent tracer-labeled hapten (50-fold dilution) in borate saline buffer (BB) were added to non-binding black microtiter plate. Then, 50 μL mAb in BB (200 μg mL−1) was added and reacted for 12 min. The FP value was measured by SpectraMax M5 with the 492 nm for excitation wavelength and 526 nm for emission wavelength.
2.6 Cross-reactivity (CR)
A series of thiacloprid analogues standard solutions were prepared and analyzed by FPIA. The FPIA standard curves for different analogues were established to obtain 50% inhibition concentration (IC50) and calculate the CR using the following formula:
CR (%) = [IC50 (thiacloprid)/IC50 (analogue)] ×100. |
2.7 Analysis of spiked samples
The soil, rice, tomato and cucumber samples, which were verified by HPLC to be thiacloprid-free, were collected from the local farm in Nanjing, China. The thiacloprid standard solutions were spiked to these blank samples (10 g) with final concentrations of 80, 400, 2000 ng g−1. The spiked samples were placed at dark for overnight. Then, thiacloprid in these samples were extracted by 20 mL of 50% methanol-BB. The mixtures were vortexed for 5 min and sonicated for 15 min. The supernatants containing thiacloprid were collected by vacuum filtering and adjusted to 25 mL. After appropriate dilution, the supernatants were test by FPIA for determining the concentrations of thiacloprid.
2.8 HPLC validation
Eight tomato samples, collected from local farm in Nanjing, China, were parallelly analyzed by FPIA and HPLC. The pretreatment of tomato samples for FPIA were same with spiked sample. The tomato samples for HPLC were prepared as follow: 10 g homogenized sample was extracted by 30 mL methanol. The mixture was vortexed for 5 min and sonicated for 10 min. After centrifugation, half supernatant (15 mL) was filtered through anhydrous sodium sulfate and evaporated to dryness. The extractions were dissolved by 2 mL acetonitrile/water (30:70, v/v) for HPLC (Agilent 1260) analysis. The chromatographic column was Eclipse plus-C18 column (250 mm × 4.6 mm, 5 μm) and the detection parameters were set as follow: injection volumn was 20 μL; mobile phase was acetonitrile–water (30:70, v/v) at a flow rate of 1 mL min−1 at 30 °C; the detection wavelength was 245 nm.
3. Results and discussion
3.1 The characterization of mAb
The cell line that can stably produce the mAb against thiacloprid was named C9, which antibody subtype was measured as IgG2b. MAb C9 shows good performance on thiacloprid detection in ic-ELISA, which IC50 value is 2.31 ng mL−1 and limit of detection (LOD) is 0.44 ng mL−1 (Fig. 1). Compared with the reported antibodies, Yin et al. prepared the anti-thiacloprid monoclonal antibody with IC50 of 26.30 ng mL−1,13 Liu et al.10 and Li et al.15 prepared the anti-thiacloprid polyclonal antibodies with IC50 values of 10.00 ng mL−1 and 182.62 ng mL−1 respectively, the mAb C9 presented in this study shows the best sensitivity.
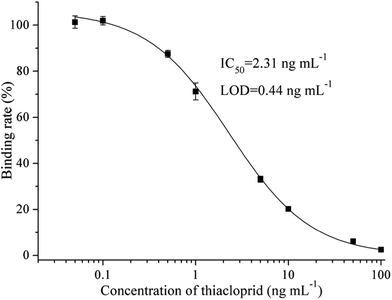 |
| Fig. 1 Standard curve for thiacloprid by ic-ELISA with mAb C9. Serial dilutions of thiacloprid standard were mixed with mAb C9. Then 100 μL of the mixtures were added to the coating antigen-coated wells. Each point represents the mean value of three replicates. | |
3.2 Selection of fluorescent tracers
Two fluorescent tracers with different length spacers were synthesized and conjugated with the hapten of thiacloprid. The fluorescent tracer-labeled haptens (THI-EDF and THI-HMDF) were purified by TLC (Fig. S2†). There were three bands at Rf = 0.4, 0.6 and 0.8 for THI-EDF, while Rf = 0.5, 0.6 and 0.8 for THI-HMDF. The fluorescent tracer-labeled haptens could bind mAb C9 to raise the FP value. The compounds in yellow bands were collected and tested separately. After reacting with mAb C9, the compounds showed maximum increase in FP value were considered the target compounds, they were the compounds at Rf = 0.4 for THI-EDF and Rf = 0.5 for THI-HMDF (Fig. S3†). Mass spectrometry and nuclear magnetic resonance were used to confirm the presence of fluorescent tracer-labeled haptens among reaction product: HRMS (ESI, +ve) calculated for C36H31N7O6S3+ (THI-EDF) 754.1576, found 754.1572, HRMS (ESI, +ve) calculated for C40H39N7O6S3+ (THI-HMDF) 810.2202, found 810.2213 (Fig. S4†); THI-EDF: 1H NMR (400 MHz, CD3OD) δ 8.38 (s, 1H), 8.14 (s, 1H), 7.76 (d, J = 8.6 Hz, 1H), 7.62–7.55 (m, 1H), 7.31–7.25 (m, 3H), 7.22 (d, J = 8.3 Hz, 1H), 6.74–6.52 (m, 4H), 4.67 (s, 2H), 4.07 (t, J = 6.6 Hz, 2H), 3.90 (t, J = 7.6 Hz, 2H), 3.52–3.46 (m, 2H), 3.42 (t, J = 7.1 Hz, 2H), 2.86 (t, J = 7.3 Hz, 2H), 2.67 (t, J = 7.0 Hz, 2H), THI-HMDF: 1H NMR (400 MHz, CD3OD) δ 8.18 (d, J = 1.9 Hz, 1H), 8.15 (s, 1H), 7.76 (d, J = 2.4 Hz, 1H), 7.68–7.64 (m, 2H), 7.49 (d, J = 2.5 Hz, 1H), 7.44 (d, J = 2.4 Hz, 2H), 7.28 (d, J = 2.1 Hz, 1H), 7.22 (d, J = 2.0 Hz, 2H), 7.16 (d, J = 4.9 Hz, 1H), 4.67 (s, 2H), 4.36–4.31 (m, 4H), 4.07 (t, J = 6.6 Hz, 2H), 3.73–3.66 (m, 2H), 2.86 (t, J = 7.3 Hz, 2H), 2.60 (t, J = 7.3 Hz, 2H), 1.80–1.73 (m, 4H), 1.56–1.50 (m, 4H).
The FP values gradually increased with the processing of incubation, and reached the maximum after 12 minutes (Fig. S5†). So the optimal incubation time of FPIA were determined as 12 min. Then the sensitivities of FPIA with different fluorescent tracers were tested. The dilution times were 400 for THI-EDF and 200 for THI-HMDF to get the fluorescence values of 1000. The optimal concentrations of mAb C9 were determined when the FP values reach 50–80% of mPmax values after binding with THI-EDF/THI-HMDF, which were 100 μg mL−1 for THI-EDF and 50 μg mL−1 for THI-HMDF, respectively. Under the optimal case, the IC50 of FPIA using THI-EDF was 59.64 ng mL−1, while 22.31 ng mL−1 for the FPIA using THI-HMDF (Fig. 2). Therefore, THI-HMDF was chosen to develop the assay.
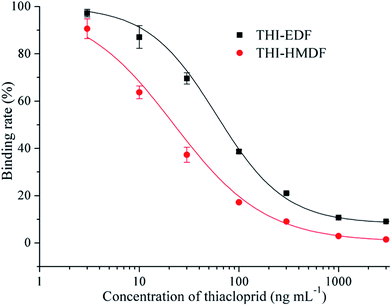 |
| Fig. 2 FPIAs for thiacloprid with different fluorescent tracers. Serial dilutions of thiacloprid standard were mixed with different fluorescent tracers respectively in black microtiter plate and analyzed by FPIA. | |
3.3 Optimization of FPIA
The concentrations of Na+ and pH value in the reaction system were optimized to enhanced sensitivity of the assay. In the pH 5.4, BB containing 0.1 mol L−1 Na+, the assay showed lowest IC50 and highest mPmax/IC50 (Tables S1 and S2†). The organic solvent is essential reagent in extraction and dissolution of pesticide, which usually shows great influence on immunoassay. Methanol is commonly used in immunoassay because of its relatively weak effect. The maximum tolerance to methanol of FPIA was 5% in reaction system (Table S3†).
3.4 Sensitivity of FPIA
Thiacloprid was detected by FPIA with the competitive format. With the increase of concentration of thiacloprid, the THI-HMDF bound to mAb C9 would decrease, which resulted in the decrease of mP value. Under the optimal condition, the standard curve of FPIA was fitted by Origin 8.0 with the concentration of thiacloprid as abscissa and the binding rate (%) as ordinate (Fig. 3). The IC50 value, LOD and linear range (IC20∼IC80) are 15.34 ng mL−1, 2.43 ng mL−1 and 3.10–65.7 ng mL−1. Compared with the sensitivities of the immunoassays had been developed (Table 1), the sensitivity of FPIA was not the best, just lower than phage ELISA, time-resolved fluoroimmunoassay (TRFIA) and enhanced chemiluminescence enzyme-linked immunosorbent assay (CLEIA).11–13 However, as the homogeneous system, the advantages of high throughput, short assay time and simple operation of the FPIA were attractive.
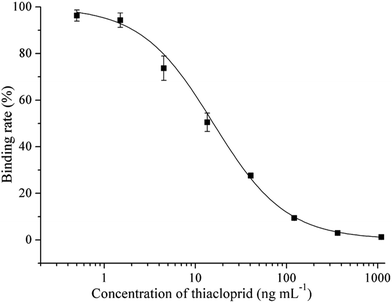 |
| Fig. 3 Standard curve of FPIA for thiacloprid. Serial dilutions of thiacloprid standard mixed with THI-HMDF in optimized buffer were added to black microtiter plate. Then mAb C9 were added to the wells. Each point represents the mean value of three replicates. | |
Table 1 An overview on the immunoassays reported for determination of thiacloprid
Method |
Steps |
Assay timea (h) |
IC50 (ng mL−1) |
Reference |
The step of coating over night was calculated as 2 h. |
ELISA |
6 |
4.8, 4.1, 5.7 |
10.00, 182.62, 26.30 |
10, 13 and 15 |
Phage-ELISA |
6 |
5.7 |
8.30 |
13 |
CLEIA |
6 |
4.1 |
30.90 |
24 |
Enhanced CLEIA |
6 |
4.0 |
1.80 |
11 |
TRFIA |
6 |
5.1 |
1.90 |
12 |
Immunochip assay |
3 |
0.7 |
46.87 |
14 |
FPIA |
1 |
0.2 |
15.34 |
This work |
3.5 Specificity of FPIA
Specificity of the assay was expressed by CR value. A series of thiacloprid analogues were tested by FPIA to calculate CR (%) values. Table 2 showed there were no significant CR (≤0.4%) except for acetamiprid with slight CR (CR = 2.6%). The results shows that the FPIA is specific for detection of thiacloprid.
Table 2 IC50 values and cross-reactivity of a set of analogues structurally related to thiacloprid by FPIA
Compound |
Structure |
IC50 (ng mL−1) |
CR (%) |
Thiacloprid |
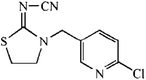 |
15.34 |
100.0 |
Acetamiprid |
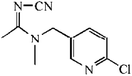 |
604.36 |
2.5 |
Imidacloprid |
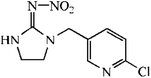 |
18 232.87 |
0.1 |
Imidaclothiz |
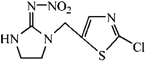 |
6090.29 |
0.3 |
Clothianidin |
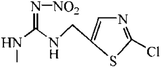 |
3704.99 |
0.4 |
Nitenpyram |
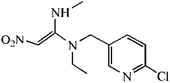 |
>100 000 |
<0.02 |
Thiamethoxam |
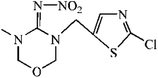 |
>100 000 |
<0.02 |
3.6 Average recovery
The standard curve ran in sample matrix is usually different from the standard curve ran in optimal buffer because of matrix effects. So, the matrix effects are evaluated and eliminated at first in sample analysis by diluting the extracts with the optimal buffer. The matrixes standard curves of rice, soil, tomato and cucumber are similar with standard curve in 5% methanol-BB after a total 30-fold dilution (containing 2-fold dilution in immunoassay procedures, 2.5-fold dilution in extraction procedures and 6-fold dilution of extract), which represents the matrix effect have been eliminated (Fig. S6†). Using the optimal dilution factors, the average recoveries of thiacloprid in spiked samples were in the range of 79.1–105.3% with the RSDs of 3.7–12.3% (Table 3). Although dilution would decrease the LOD of the immunoassay, it is a simple way to remove the matrix effects. In the analysis of spiked samples, the lowest spiked concentration was 0.08 mg kg−1, which is lower than the maximum residue limits of thiacloprid in China (0.2, 0.5 and 1 mg kg−1 in brown rice, tomato and cucumber, GB 2763-2016). Therefore, the FPIA can meet the requirement of thiacloprid detection.
Table 3 Average recoveries of samples spiked with thiacloprid by FPIA (n = 3)
Sample |
Spiked concentration (ng g−1) |
Recovery ± SD (%) |
RSD (%) |
Rice |
80 |
90.7 ± 5.5 |
6.0 |
400 |
84.3 ± 3.1 |
3.7 |
2000 |
88.6 ± 6.1 |
6.9 |
Soil |
80 |
105.3 ± 9.7 |
9.2 |
400 |
93.7 ± 6.6 |
7.1 |
2000 |
80.8 ± 4.1 |
5.1 |
Tomato |
80 |
88.4 ± 7.2 |
8.2 |
400 |
79.1 ± 5.6 |
7.0 |
2000 |
82.9 ± 10.2 |
12.3 |
Cucumber |
80 |
93.5 ± 8.6 |
9.2 |
400 |
89.1 ± 7.4 |
8.3 |
2000 |
85.7 ± 5.1 |
6.0 |
3.7 HPLC validation
Eight tomato samples were parallelly analyzed by FPIA and HPLC. The concentrations of thiacloprid in these samples detected by FPIA and HPLC were 121.0–1409 ng g−1 and 95.14–1610 ng g−1, respectively. The Student's t test between the detection results of HPLC and FPIA (P = 0.4937) showed that there was no significantly difference. In addition, the slope of linear regression equation and correlation coefficient (R2) between FPIA and HPLC were both close to 1 (y = 0.9401x + 14.09, R2 = 0.9579), which indicates the good correlation between FPIA and HPLC detection (Fig. 4).
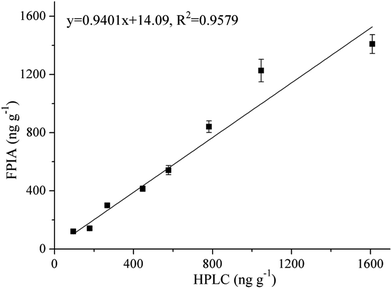 |
| Fig. 4 Correlation between the FPIA and HPLC. Eight representative tomato samples were analyzed by FPIA and HPLC. The line equation and correlation coefficient were obtained from the linear regression are shown. | |
4. Conclusions
In this work, we prepared an anti-thiacloprid mAb with high sensitivity. Then hapten of thiacloprid was conjugated with two fluorescent tracers with different length spacers. By comparing the sensitivities, the THI-HMDF (containing six carbon length spacer) was used to develop FPIA for detection of thiacloprid because of the higher sensitivity. Compared with other assays that had been reported, FPIA just requires 12 min for detection of pesticide, and the homogeneous system make the operation simpler. The FPIA shows high sensitivity and good specificity for detection of thiacloprid, while performs good recoveries and shows good correlation with HPLC in analysis of authentic samples. This assay would be a good addition to detection of thiacloprid. Except microplate reader, the FP value also can be measured by portable fluorescence polarizer, which is potential for on-site detection.
Conflicts of interest
There are no conflicts to declare.
Acknowledgements
This work was supported by the National Key Research and Development Program of China (2017YFF0210200) and the National Natural Science Foundation of China (31772194).
References
- K. Matsuda, M. Shimomura, M. Ihara, M. Akamatsu and D. B. Sattelle, Biosci., Biotechnol., Biochem., 2005, 69, 1442–1452 CrossRef CAS.
- A. Brandt, A. Gorenflo, R. Siede, M. Meixner and R. Büchler, J. Insect Physiol., 2016, 86, 40–47 CrossRef CAS.
- L. W. Pisa, V. Amaralrogers, L. P. Belzunces, J. M. Bonmatin, C. A. Downs, D. Goulson, D. P. Kreutzweiser, C. Krupke, M. Liess and M. Mcfield, Environ. Sci. Pollut. Res., 2015, 22, 68–102 CrossRef CAS.
- L. Tison, M. L. Hahn, S. Holtz, A. Rößner, U. Greggers, G. Bischoff and R. Menzel, Environ. Sci. Technol., 2016, 50, 7218–7227 CrossRef CAS.
- U. Bajwa and K. S. Sandhu, J. Food Sci. Technol., 2014, 51, 201–220 CrossRef CAS.
- J. Vichapong, R. Burakham and S. Srijaranai, Food Analytical Methods, 2016, 9, 419–426 CrossRef.
- O. López-Fernández, R. Rial-Otero and J. Simal-Gándara, Anal. Bioanal. Chem., 2015, 407, 7101–7110 CrossRef.
- R. N. Li, Z. L. Chen, F. S. Dong, J. Xu, X. G. Liu, X. H. Wu, X. L. Pan, Y. Tao and Y. Q. Zheng, J. Chromatogr. B: Anal. Technol. Biomed. Life Sci., 2018, 1081, 29–36 Search PubMed.
- Y. Quan, Y. Zhang, S. Wang, N. Lee and I. R. Kennedy, Anal. Chim. Acta, 2016, 580, 1–8 CrossRef.
- Z. J. Liu, M. Li, H. Y. Shi and M. H. Wang, Food Analytical Methods, 2013, 6, 691–697 CrossRef.
- Z. J. Liu, X. Wei, H. Xu, M. Li, G. B. Zhu, Y. L. Xue, Z. Zhang, G. D. Zhao and D. L. Du, RSC Adv., 2016, 6, 29460–29465 RSC.
- Z. J. Liu, X. Yan, X. D. Hua and M. H. Wang, Anal. Methods, 2013, 5, 3572–3576 RSC.
- W. Yin, X. D. Hua, X. F. Liu, H. Y. Shi, S. J. Gee, M. H. Wang and B. D. Hammock, Anal. Biochem., 2015, 481, 27–32 CrossRef CAS.
- M. J. Lan, Y. R. Guo, Y. Zhao, Y. H. Liu, W. J. Gui and G. N. Zhu, Anal. Chim. Acta, 2016, 938, 146–155 CrossRef CAS.
- H. X. Li, X. Yan, H. Y. Shi and X. H. Yang, Food Chem., 2014, 164, 166–172 CrossRef CAS.
- W. B. Dandlike, S. P. Halbert, M. C. Florin, R. Alonso and H. C. Shapiro, J. Exp. Med., 1965, 122, 1029–1048 CrossRef.
- H. Y. Zhang, S. P. Yang, K. D. Ruyck, N. V. Beloglazova, S. A. Eremin, S. D. Saeger, S. X. Zhang, J. Z. Shen and Z. H. Wang, TrAC, Trends Anal. Chem., 2019, 114, 293–313 CrossRef CAS.
- G. S. Furzer, L. Veldhuis and J. C. Hall, J. Agric. Food Chem., 2006, 54, 688–693 CrossRef CAS.
- S. Q. Liao, J. J. Zhao, Y. F. Qin and S. L. Zhao, RSC Adv., 2017, 7, 55668–55672 RSC.
- M. Li, X. F. Liu, X. D. Hua, W. Yin, Q. K. Fang and M. H. Wang, Anal. Methods, 2014, 6, 6541–6547 RSC.
- A. Y. Boroduleva, J. J. Manclus, A. Montoya and S. A. Eremin, Anal. Bioanal. Chem., 2018, 410, 6923–6934 CrossRef CAS.
- L. Oberleitner, S. A. Eremin, A. Lehmann, L. A. Garbe and R. J. Schneider, Anal. Methods, 2015, 7, 5854–5861 RSC.
- A. Y. Boroduleva, J. Wu, Q. Q. Yang, H. Li, Q. Zhang, P. W. Li and S. A. Eremin, Anal. Methods, 2017, 9, 6814–6822 RSC.
- Z. J. Liu, X. Yan, X. Y. Xu and M. H. Wang, Analyst, 2013, 138, 3280–3286 RSC.
Footnote |
† Electronic supplementary information (ESI) available. See DOI: 10.1039/c9ra04776j |
|
This journal is © The Royal Society of Chemistry 2019 |
Click here to see how this site uses Cookies. View our privacy policy here.