DOI:
10.1039/C9RA05059K
(Paper)
RSC Adv., 2019,
9, 29273-29292
Design, synthesis and biological evaluation of 1,2,3-triazole based 2-aminobenzimidazoles as novel inhibitors of LasR dependent quorum sensing in Pseudomonas aeruginosa†
Received
4th July 2019
, Accepted 6th September 2019
First published on 17th September 2019
Abstract
Bacteria regulate their phenotype, growth and population via a signalling pathway known as quorum sensing. In this process, bacteria produce signalling molecules (autoinducers) to recognize their population density. Inhibiting this quorum sensing signalling pathway is one of the potential methods to treat bacterial infection. 2-Aminobenimdazoles are reported to be the strongest inhibitors of quorum sensing against wild-type P. aeruginosa. 1,2,3-Triazole based acyl homoserine lactones are found to be good inhibitors of the quorum sensing LasR receptor. Hence, in our current study, forty 1,2,3-triazole based 2-aminobenzimdazoles were synthesized and characterized using IR, NMR, MS and elemental analysis. A single crystal was developed for N-(1H-benzo[d]imidazol-2-yl)-2-(4-nonyl-1H-1,2,3-triazol-1-yl)acetamide (6d). All final compounds were screened for in vitro quorum sensing inhibitory activity against Pseudomonas aeruginosa. The quorum sensing inhibitory activity was determined in the LasR expressing P. aeruginosa MH602 reporter strain by measuring green fluorescent protein production. Among the title compounds, N-(1H-benzo[d]imidazol-2-yl)-2-(4-(4-chlorophenyl)-1H-1,2,3-triazol-1-yl)acetamide (6i) exhibited good quorum sensing inhibitory activity of 64.99% at 250 μM. N-(1H-Benzo[d]imidazol-2-yl)-2-(4-(4-nitrophenyl)-1H-1,2,3-triazol-1-yl)acetamide (6p) exhibited the most promising quorum sensing inhibitory activity with 68.23, 67.10 and 63.67% inhibition at 250, 125 and 62.5 μM, respectively. N-(1H-Benzo[d]imidazol-2-yl)-2-(4-(4-(trifluoromethyl)phenyl)-1H-1,2,3-triazol-1-yl)acetamide (6o) and N-(5,6-dimethyl-1H-benzo[d]imidazol-2-yl)-2-(4-(4-(trifluoromethyl)phenyl)-1H-1,2,3-triazol-1-yl)acetamide (7l) also exhibited 64.25% and 65.80% quorum sensing inhibition at 250 μM. Compound 6p, the most active quorum sensing inhibitor, also displayed low cytotoxicity at the tested concentrations (25, 50 and 100 μM) against normal human embryonic kidney cell lines. Finally, a docking study using Schrodinger Glide elucidated the possible putative binding mode of the significantly active compound 6p at the active site of the target LasR receptor (PDB ID: 2UV0).
1. Introduction
Many pathogenic bacteria use signaling strategies to multiply their number in a process known as Quorum sensing (QS). In this process, bacteria produce and release chemical signaling molecules, known as auto-inducers (AIs). These signaling chemical molecules play a vital role in both bacterial virulence and symbiosis and have a noteworthy impact on human health, cultivation and the environment.1–3 At high bacterial cell density, bacteria alter gene expression levels to coordinate assorted behaviors, including biofilm formation, virulence factor production, bioluminescence, conjugation, sporulation, etc.4–7 Gram-negative bacteria cause several nosocomial infections including bloodstream infections, pneumonia, wound or surgical site infections.8,9 P. aeruginosa, an opportunistic pathogen and second leading cause of ventilator associated pneumonia is responsible for several serious infections in immune compromised patients, chronic lung infection (cystic fibrosis) and for the most common fatal communicable disease.10–13 As P. aeruginosa develops resistant to available carbapenem and other antibiotics, it is listed as critical by the World Health Organization.14 Bacterial resistance may cause prolonged hospitalization, expensive medical costs and increase in mortality and hence, there is an urgent need to look out for non-antibiotic solutions to manage invasive bacterial infections and QS could be a potential solution. QSIs are attractive alternatives to replace the conventional antibiotics to minimize the development of bacterial resistance.15,16 So far, many QSIs have been reported but only very few entered clinical trials.17 Many proteobacteria which includes P. aeruginosa use N-acylated-L-homoserine lactones (AHLs/AIs) as QS signaling molecules.18 (Fig. 1).
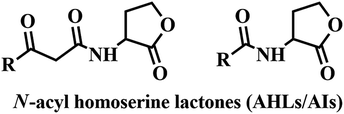 |
| Fig. 1 Autoinducers in proteobacteria. | |
P. aeruginosa uses LasI/LasR, RhlI/RhlR and PQS/MvfR QS systems to alter gene expression and is responsible for prolonged hospitalization and high mortality in hospital patients.19–21 N-(3-Oxododecanoyl)-L-homoserine lactone (OdDHL) and N-butanoyl-L-homoserine lactone (BHL) are the AIs of P. aeruginosa (Fig. 2).22
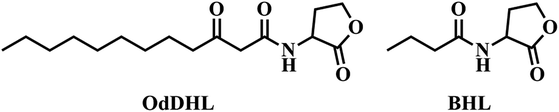 |
| Fig. 2 Auto-inducers in P. aeruginosa. | |
Design
Several AHL and non-AHL based QSIs against P. aeruginosa are reported till date.23,24 Biofilm formation of pathogenic bacteria occurs at high cell-density and higher cell density is responsible for QS.25–27 Based on this, Blackwell group identified two most active biofilm inhibitors, 2-aminobenzimidazole (I) and 5,6-dimethyl-2-aminobenzimidazole (II) and evaluated for QSI activity against wild-type P. aeruginosa QS reporter strains. Among these, compound II possesses higher inhibition against Las and Rhl QS systems with IC50 values 37.5 μM and 12.5 μM respectively.28,29 Furthermore, the same group reported that compound II is a potential inhibitor of LasR activity with IC50 2.3 μM against P. aeruginosa PAO-JP2 and 1.4 μM against P. aeruginosa PAO-JG21 (Fig. 3).29
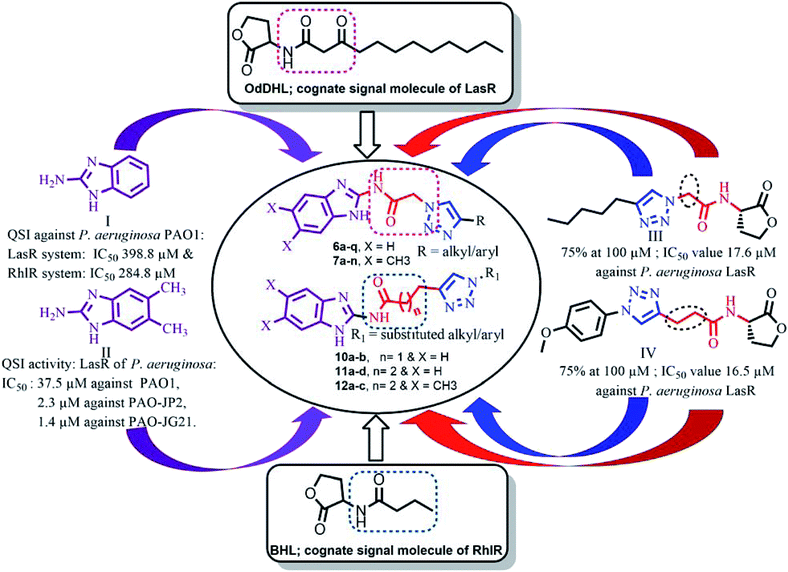 |
| Fig. 3 Design strategy for the synthesis of titled quorum sensing inhibitors. | |
1,2,3-Triazoles are reported as QSIs, antitubercular agents, fluorinated hydrogels and their applications in radiochemistry was also studied previously.30–35 In 2013, Stacy et al. reported AHL based 1,2,3-triazoles as QSIs, in which, they retained the lactone ring of AHL and its amide bond and modified the side chain with various substituted 1,2,3-triazoles and reported effective QSIs against P. aeruginosa. Further, they have clearly highlighted that inclusion of triazoles led to superior activity than their counter parts against LasR.31 Compounds III and IV are the most active QS inhibitors (Fig. 3), with 75% QS inhibition at 100 μM. In conclusion, this group noticed that compounds with methylene group between N-acyl group and 1,2,3-triazole nucleus is indispensable for QSI activity,31 while compounds with absence of methylene group and N-acyl group exhibited poor QSI activity (compounds V and VI).32,33 Compounds VII and VIII are active QSIs with 50.7% and 49.7% inhibition at 250 μM against P. aeruginosa MH602 respectively.34 In another recent work, two methylene linkers retained between triazole nucleus and N-acyl-2-phenyl indole gave the most active QSI with 60.8% inhibition at 250 μM against P. aeruginosa MH602 (compound IX).24 Compound X, with one methylene linker, is an another active QSI with 74.2% inhibition at 250 μM.35 Based on these results, we retained at least one methylene linker between 1,2,3-triazole and N-acyl cycloalkane and designed the compounds of the current scheme (Fig. 3). 1,2,3-Triazole based QSIs are summarized in Table 1.
Table 1 1,2,3-Triazole based QSIs against P. aeruginosa reported from literature
Entry |
Compound number |
Structures of QSIs |
% Inhibition against P. aeruginosa |
Reference no. |
1 |
III |
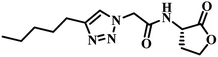 |
75% at 100 μM |
31 |
2 |
IV |
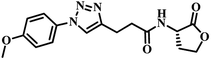 |
75% at 100 μM |
31 |
3 |
V |
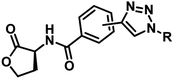 |
Ranged from 0 to 49.9% at 1 mM concentration |
32 |
4 |
VI |
 |
Ranged from 1 to 22% at 100 μM |
33 |
5 |
VII & VIII |
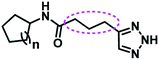 |
n = 1; VI |
50.7% at 250 μM |
34 |
n = 2; VII |
49.7% at 250 μM |
34 |
6 |
IX |
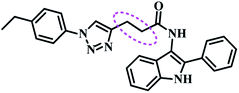 |
60.8% at 250 μM |
24 |
7 |
X |
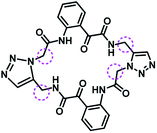 |
74.2% at 250 μM |
35 |
Enthused by the QSI active molecules of 2-aminonobenzimdazoles and 1,2,3-triazole analogues, we incorporated these active pharmacophores into one framework, and synthesized non-AHL based QSIs, as shown in Fig. 3. In this work, we synthesized various substituted 1,2,3-triazoles of N-(1H-benzo[d]imidazol-2-yl) and N-(5,6-dimethyl-1H-benzo[d]imidazol-2-yl)acetamide, propanamide and butamides and screened them for their QSI activity against P. aeruginosa MH602 strain. Several N-acyl analogues of cyclopentyl, cyclohexyl and amino pyridines also exhibited promising QSI activity against P. aeruginosa.34 Hence, as a part of this work, we also introduced triazole based N-acyl cyclopentane, cyclohexane and pyridine as the tail groups of aminobenzimdazole to gauge their importance in exhibiting QS inhibition.
2. Results and discussion
Chemistry
As shown in Scheme 1, first compounds 2a and 2b were prepared according to literature procedure from starting materials 1a and 1b respectively.36 2a and 2b upon treatment with chloroacetyl chloride yielded N-acyl compounds 3a and 3b respectively. To obtain fourth intermediate, compounds 5a and 5b from second 3a and 3b, we initially performed several reactions by varying the reaction conditions, which includes the use of different solvents (acetonitrile, tetrahydrofuran, dimethylformamide etc.) and temperatures. All these conditions required high temperatures and seal tube/microwave irradiation with long time. Furthermore, KI was used as the catalyst (Finkelstein reaction) and the reaction was carried out in an aprotic solvent in seal tube at 120 °C. It took 72 h for the reaction to proceed but only gave 35% yield. Since satisfactory yields were not obtained even after varying the conditions, we decided to synthesize 5a and 5b in two steps. 3a and 3b were dissolved in acetone and water to give a clear solution, followed by the addition of 1.2 equivalent of KI and the reaction mixture was heated up to 55 °C for 12 h. On completion of reaction, as indicated by TLC, 4a and 4b were isolated. Then pre-final azides 5a and 5b were prepared from 4a and 4b using NaN3. Final compounds 6a–q from 5a and 7a–n from 5b were obtained on treatment with various acetylenes in 45–91% yield (Scheme 1).
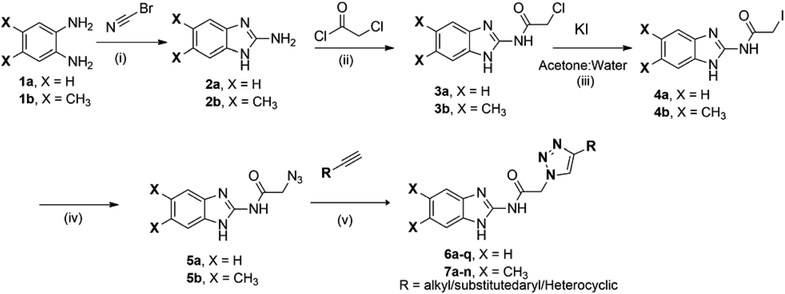 |
| Scheme 1 General synthetic route for compounds 6a–q and 7a–n. Reagents and conditions: (i) cyanogen bromide (1.1 eq.), methanol, reflux, 5 min; (ii) chloroacetyl chloride (1.0 eq.), pyridine (2.5 eq.), CH2Cl2, 0 °C-rt, 12 h; (iii) potassium iodide (1.2 eq.), acetone, 55 °C, 12 h; (iv) NaN3 (1.5 eq.), DMF : water (8 : 2), 0 °C; (v) substituted terminal alkynes (1.4 eq.), CuSO4·5H2O, (5 mol%), sodium ascorbate (10 mol%), DMF : water (8 : 2), rt, 6–16 h. | |
As shown in Scheme 2, in order to get amides 9a–c, we performed acid-amine coupling reaction using EDC·HCl and HOBt as coupling reagents. Compound 9a was prepared from 1H-benzo[d]imidazol-2-amine (2a) and pent-4-ynoic acid (8a). Similarly, 2a and 2b on treatment with hex-5-ynoic acid (8b) yielded compounds 9b and 9c respectively. Finally, the 9a, 9b and 9c on treatment with various substituted azides using copper sulphate pentahydrate as catalyst gave 10a–b, 11a–d and 12a–c in 45–68% yield.
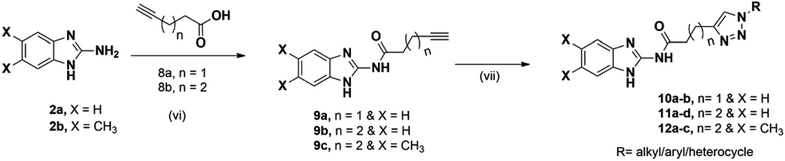 |
| Scheme 2 General synthetic route for compounds 10a–b, 11a–d and 12a–c. Reagents and conditions: (vi) 2a or 2b (1.0 eq.), EDC·HCl (1.5 eq.) HOBt, (1.5 eq.) diisopropyl ethylamine (2.5 eq.), DMF, rt, 16 h; (vii) 9a/9b/9c and substituted azides (1.5 eq.), CuSO4·5H2O, (10 mol%), sodium ascorbate (10 mol%), DMF : water (8 : 2), rt, 6–16 h. | |
Most of the azides required for triazole preparation were procured commercially. However, azides A, B and C required for the preparation of the final derivatives of Series-III were accomplished via Scheme 3.
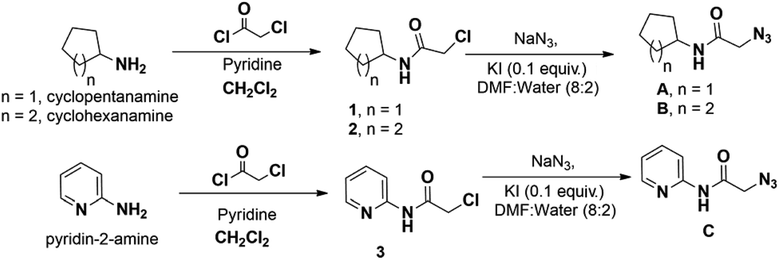 |
| Scheme 3 General synthetic route for intermediates A, B and C. | |
The purity of the synthesized compounds was assessed by LC-MS and elemental analyses. Structures of the compounds were confirmed by spectral data. In 1H NMR and 13C NMR, the signals of the corresponding protons and carbon atoms were verified based on their chemical shift, multiplicities and coupling constant values. The results of elemental analysis were within ±0.05 of the theoretical values.
1H NMR spectra of compounds 6a–q and 7a–n from Scheme 1 showed singlet which integrates to two protons at 5–6 ppm due to the active methylene CH2, whereas the methylene protons for compounds 10–12 from Scheme 2 were observed at more up field (at around 1.5 to 3.5 ppm) due to a larger separation of methylene protons from N-acyl group and triazole nucleus. All final compounds from Schemes 1 and 2 have triazole nucleus, benzimidazole N–H and amide N–H, in which the proton of triazole nucleus was observed at around 8.2–9.2 ppm as singlet, and the N–H protons of amide and imidazole were observed as broad singlets at 11–14 ppm. Furthermore, single crystal was developed for compound 6d.
Biological activity
Series of triazole based benzimidazole derivatives (6a–q, 7a–n, 10a–b, 11a–d and 12a–c) were analyzed for their QS-inhibition activity at various concentrations (62.5, 125 & 250 μM) against P. aeruginosa MH602 lasB reporter strain [PlasB: gfp(ASV)] following the protocol developed by Hentzer et al.37 The production of AHL signals by this reporter strain leads to an increase in the production of unstable green fluorescent protein (GFP-ASV) as a function of an active QS system. Considering this fact, synthesis of benzimidazole triazole analogues has been prioritized to counteract the QS system. The results are compiled in Table 2.
Table 2 Percentage inhibition of GFP fluorescence by the synthesized compounds against P. aeruginosa MH602
Entry |
R |
R1 |
X |
n |
Concentrationa (μM) |
250 |
125 |
62.5 |
Growth inhibition, ±standard deviation of the mean from at least two independent experiments. In each independent experiment, compounds were tested in triplicate. NA: no activity. |
Series-I |
6a |
 |
— |
H |
— |
50.50 ± 11.81 |
35.28 ± 20.43 |
25.10 ± 0.37 |
6b |
 |
— |
H |
— |
43.47 ± 6.23 |
38.77 ± 11.47 |
27.37 ± 2.36 |
6c |
 |
— |
H |
— |
42.76 ± 14.58 |
34.69 ± 11.60 |
25.68 ± 7.62 |
6d |
 |
— |
H |
— |
48.55 ± 12.66 |
41.93 ± 4.50 |
30.61 ± 7.62 |
6e |
 |
— |
H |
— |
41.31 ± 7.90 |
37.20 ± 6.71 |
24.87 ± 6.69 |
6f |
 |
— |
H |
— |
58.84 ± 5.09 |
52.70 ± 11.86 |
36.07 ± 10.70 |
6g |
 |
— |
H |
— |
45.95 ± 10.15 |
17.43 ± 21.96 |
15.10 ± 5.83 |
6h |
 |
— |
H |
— |
56.14 ± 1.77 |
54.85 ± 2.42 |
48.95 ± 12.32 |
6i |
 |
— |
H |
— |
64.99 ± 8.25 |
60.55 ± 6.16 |
52.86 ± 8.86 |
6j |
 |
|
H |
— |
50.74 ± 10.91 |
46.25 ± 8.52 |
45.68 ± 5.44 |
6k |
 |
— |
H |
— |
35.85 ± 13.83 |
32.32 ± 22.23 |
36.82 ± 6.63 |
6l |
 |
— |
H |
— |
35.22 ± 8.78 |
NA |
NA |
6m |
 |
— |
H |
— |
58.44 ± 7.75 |
45.66 ± 6.21 |
34.37 ± 4.97 |
6n |
 |
— |
H |
— |
20.03 ± 14.00 |
5.16 ± 16.63 |
NA |
6o |
 |
— |
H |
— |
64.25 ± 7.30 |
59.85 ± 7.99 |
57.55 ± 7.73 |
6p |
 |
— |
H |
— |
68.23 ± 3.87 |
67.10 ± 5.45 |
63.67 ± 7.51 |
6q |
 |
— |
H |
— |
39.37 ± 5.14 |
36.04 ± 6.13 |
28.21 ± 10.23 |
![[thin space (1/6-em)]](https://www.rsc.org/images/entities/char_2009.gif) |
Series-II |
7a |
 |
— |
CH3 |
— |
31.76 ± 10.99 |
22.05 ± 14.66 |
19.85 ± 3.80 |
7b |
 |
— |
CH3 |
— |
26.59 ± 20.80 |
20.22 ± 11.52 |
16.92 ± 5.74 |
7c |
 |
— |
CH3 |
— |
NA |
NA |
NA |
7d |
 |
— |
CH3 |
— |
44.57 ± 3.87 |
41.65 ± 5.08 |
37.11 ± 7.75 |
7e |
 |
— |
CH3 |
— |
16.00 ± 26.10 |
NA |
15.13 ± 9.82 |
7f |
 |
|
CH3 |
— |
36.80 ± 7.90 |
29.03 ± 13.57 |
26.08 ± 7.69 |
7g |
 |
— |
CH3 |
— |
47.56 ± 14.43 |
29.85 ± 17.40 |
28.51 ± 8.41 |
7h |
 |
— |
CH3 |
— |
57.60 ± 7.43 |
44.67 ± 14.27 |
31.23 ± 5.02 |
7i |
 |
— |
CH3 |
— |
50.74 ± 12.31 |
14.91 ± 8.82 |
NA |
7j |
 |
— |
CH3 |
— |
44.73 ± 13.15 |
24.16 ± 14.52 |
17.62 ± 6.21 |
7k |
 |
— |
CH3 |
— |
40.82 ± 23.41 |
24.30 ± 23.26 |
20.38 ± 2.59 |
7l |
 |
— |
CH3 |
— |
65.80 ± 1.91 |
56.44 ± 5.85 |
36.13 ± 7.67 |
7m |
 |
— |
CH3 |
— |
53.85 ± 9.78 |
31.46 ± 16.18 |
21.02 ± 10.29 |
7n |
 |
— |
CH3 |
— |
46.73 ± 4.87 |
34.42 ± 14.10 |
34.20 ± 7.43 |
![[thin space (1/6-em)]](https://www.rsc.org/images/entities/char_2009.gif) |
Series-III |
10a |
— |
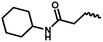 |
H |
1 |
47.40 ± 2.69 |
42.35 ± 8.46 |
38.43 ± 7.72 |
10b |
— |
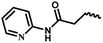 |
H |
1 |
28.46 ± 17.54 |
15.64 ± 7.17 |
4.40 ± 2.93 |
11a |
— |
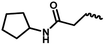 |
H |
2 |
51.23 ± 7.10 |
45.93 ± 8.18 |
42.27 ± 10.29 |
11b |
— |
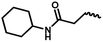 |
H |
2 |
12.87 ± 20.18 |
NA |
NA |
11c |
— |
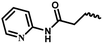 |
H |
2 |
19.41 ± 8.16 |
15.69 ± 8.81 |
3.69 ± 11.15 |
11d |
— |
 |
H |
2 |
39.38 ± 4.60 |
21.37 ± 2.62 |
21.17 ± 1.76 |
12a |
— |
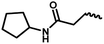 |
CH3 |
2 |
48.48 ± 5.25 |
34.14 ± 12.60 |
28.54 ± 7.69 |
12b |
— |
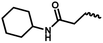 |
CH3 |
2 |
30.83 ± 26.38 |
9.74 ± 20.67 |
5.35 ± 10.20 |
12c |
— |
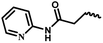 |
CH3 |
2 |
48.26 ± 13.42 |
7.07 ± 11.80 |
NA |
Furanone 30 |
— |
— |
|
— |
86.86 ± 1.18 |
83.02 ± 3.15 |
77.33 ± 2.00 |
Predictive structure–activity relationship study (SAR)
In the first series (Series-I in Table 2), benzimidazoles 6a–q with no substitution on the benzene ring were undertaken. The effect of alkyl chain length on the triazole partner of the molecule was first studied. It was found that changing the lipophilicity by altering the length of the alkyl chain on triazole ring has no significant effect on the QSI activities of the compound as compounds 6a–d possess similar percentage of QS inhibition within different tested concentrations. The effect of attaching a terminal heterocyclic group on the triazole partner of the molecule was then studied. While attaching a 2-methylthio-benzimidazole onto the triazole ring (6e) gave a similar QSI activity (41.31% inhibition at 250 μM) as compounds 6a–d (42.76–50.50% inhibition at 250 μM), it was found that attaching 5-chloro-pyridone on the triazole nucleus (6f) enhanced the QSI activity of benzimidazole to 58.84% at 250 μM. The QSI activities of benzimidazoles having various substituted aryl groups on triazole nucleus (6g–q) were also investigated. Halo-substitution at the para-position of aryls on the triazole nucleus (6h–j) showed promising QSI activity even at low concentration (>45.00% inhibition at 62.5 μM) when compared to its un-substituted parent molecule 6g, with only 15.10% inhibition at 62.5 μM. Furthermore, among these halo-aryl derivatives, chloro-derivative (6i) exhibited the highest QSI activity at all concentrations. Alkyl groups substituted at the para-position of aryl ring (6k–l) diminished the QSI activity of the benzimidazoles. In fact, when a tert-butyl group was placed on the aryl ring of the triazole nucleus, the corresponding benzimidazole (6l) showed no QSI activity at lower concentrations. To study the effect of aryl rings carrying electron-releasing groups on the QSI activity, benzimidazoles 6m and 6n bearing a phenol and anisole group, respectively, were tested. Benzimidazoles 6m with a phenol group present on the triazole nucleus possesses moderate QSI activity at low concentrations (34.37% at 62.5 μM), however, when the phenol group was substituted by a different electron-releasing anisole group (6n), the QSI activity of the molecule was completely abolished. This suggested that QSI activity of a compound could not be correlated by attaching an electron-releasing group at the para-position of the phenyl ring on the triazole nucleus. Following this finding, the effect of substituting electron withdrawing groups on the aryl ring towards QS inhibition was studied. Benzimidazole compound 6o bearing a trifluoromethyl group on the aryl ring exhibited a significantly higher QSI activity (57.55% inhibition at 62.5 μM) when compared to its parent compound 6g (with only 15.10% inhibition at 62.5 μM) and the QSI activity is further enhanced by substituting more electron-withdrawing nitro group at para-position of the phenyl ring, with compound 6p showed 63.67% inhibition at 62.5 μM. However, when the nitro group was moved to the meta-position (6q), QSI activity was diminished (28.21% at 62.5 μM). From the QSI data of this series, it can be concluded that, QSI activity of the benzimidazole molecule is favored by the presence of aryl ring with a para-substituted electron-withdrawing group on the triazole nucleus.
In the second part of the series (Series-II), benzimidazoles 7a–n bearing di-methyl substitution on the benzene ring was considered as basic moiety and the effect of substitution on the triazole nucleus towards the QSI activity was investigated. When alkyl groups of different lengths were placed on the triazole nucleus, di-methylbenzimidazole (compound 7d) with the longest carbon chain exhibited moderate activity (44.57% at 250 μM). The QSI activity of the compounds reduced while decreasing the alkyl chain length. When introducing heterocyclic groups such as 2-methylthio-benzimidazole (7e) and 5-chloro-pyridone (7f) onto the triazole nucleus, the analogues only showed mild inhibitory activity even at the highest tested concentration. In another attempt, various aryl groups bearing different substituents on triazole nucleus were investigated. The presence of an electron-donating methyl group at the 4-position of the aryl ring (7i) did not impact the inhibitory activity at higher doses (50.74% at 250 μM) when compared to its un-substituted parent molecule 7g, in fact, it hampered the QSI inhibition at lower concentrations. The presence of other electron-donating groups such as hydroxyl (7j) and alkoxy (7k) at the 4-position of the phenyl ring were also found to be effective only at higher concentrations (>40% at 250 μM). In case of aryl rings possessing electron withdrawing groups, trifluoromethyl group at para-position enhanced the QSI activity of the dimethylbenzimidazole (65.80% at 250 μM), while other nitro substituted aryl partners were effective only at higher concentrations (7m & 7n). It can be concluded that aryl substituted triazole nucleus based dimethylbenzimidazole derivatives displayed better QS inhibition activity when electron withdrawing groups are present on the aryl ring.
Apart from the above moieties studied so far, few more 2-aminobenzimidazole derivatives were designed and synthesized by altering the length of carbon chain between the amido functional group and the triazole moiety as well as placing different functionalities on triazole nucleus (Series-III). All these moieties were explored for their QSI at reported concentrations. In the first part of this series, the chain length between the 2-amido benzimidazole and the triazole nucleus was fixed at two and two analogues 10a and 10b were synthesized by retaining different substitutions on the triazole nucleus. Among these, N-cyclohexyl acetamido substitution at the triazole (10a) showed good QSI activity regardless of the concentration (38–47% at 62.5–250 μM). In the later series, the length of the carbon chain between the 2-amido benzimidazole and the triazole nucleus was increased to three. Four benzimidazoles (11a–d) with the three carbon chain length were synthesized and tested for their QSI activity. It was found that triazole carrying N-cyclopentyl acetamido group (11a) was identified to be the most potent compound in this series and its potency was retained even at lower concentration (42.27% at 62.5 μM). On the other end, compound 11d with 4-nitrophenyl group on triazole nucleus was active only at high doses (39.38% at 250 μM). The remaining analogues 11b–c were less potent even at highest tested concentration. Finally, by maintaining three carbon chain length, various dimethylbenzimidazoles were also investigated. Dimethylbenzimidazoles possessing N-(2-pyridyl)-acetamido group on triazole nucleus (12c) showed moderate inhibition activity only at high concentration (48.26% at 250 μM) and its inhibition activity was diminished when its concentration was halved. The same trend was observed for compound 12b with a N-cyclohexyl acetamido group placed at the triazole nucleus. On the other hand, compound 12a exhibited moderate inhibition activity at 250 μM. While its inhibition activity reduces with the decrease in concentration, the magnitude of the reduction was smaller than that of compounds 12b–c. It can be concluded that N-(2-pyridyl), N-cyclopentyl and N-cyclohexyl acetamido substituted triazoles exhibited moderate QSI activity at high concentration within this series.
Overall, it can be concluded that, most of the scaffolds in this study possess moderate to high inhibition activity at high concentration and compounds 6i, 6o, 6p and 7l exhibited significant QSI activity at all the reported concentrations and were identified to be the most active compounds in this study. Most of the compounds reported in the current work exhibited comparable activity to the previously reported triazoles as shown in Table 2. The most active compound from this study, 6p is better than the previously reported compounds (V–IX of Table 1).
Cytotoxicity studies
On the basis of QS inhibitory activity, the significantly active compounds (6a, 6f, 6h, 6i, 6m, 6o, 6p, 7h, 7i, 7l, 7m and 10a) were evaluated for their cytotoxicity against Human Embryonic Kidney cell lines (HEK-293) at 25, 50 and 100 μM concentrations using BG45 as a positive control. Most of the compounds have low cytotoxicity at all tested concentrations using MTT assay (Fig. 4).38
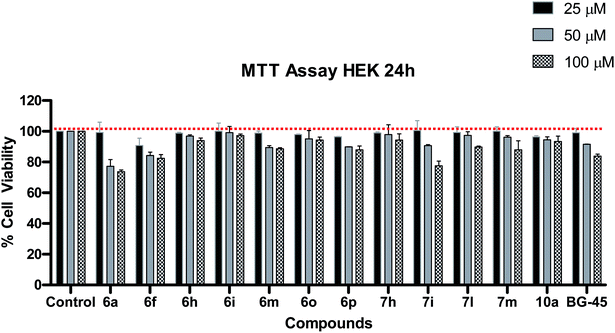 |
| Fig. 4 Cytotoxicity study of the most active QSIs in HEK-293 cells by MTT assay after 24 h compound treatment. BG45 was used as a positive control. Data represents mean ± SD (n = 3). | |
In silico prediction of drug likeness properties
Physicochemical parameters of the designed compounds were in silico predicted using Qik-prop module of Schrödinger.39,40 The in silico predicted values of physico-chemical parameters for the titled compounds (6a–q, 7a–n, 10a–b, 11a–d, 12a–c) were shown in Table 3. Furthermore, ranges of these parameters followed by 95% of market approved drugs are given as footnotes of Table 3 (ref. 41 and 42) for comparison.
Table 3 In silico predicted physico-chemical and pharmacokinetic parameters of the titled compounds
Entry |
Mol. wta |
SASAb |
HBDc |
HBAd |
log Po/we |
PCacof |
log BBg |
log Sh |
Rot. bondsi |
Mol. wt – molecular weight (130–725). SASA – solvent accessible surface area (300–1000). HBD – no. of hydrogen bond donors (0–6). HBA – no. of hydrogen bond acceptors (2 to 20). log Po/w – predicted octanol/water partition coefficient (−2.0–6.5). Caco – 2 cell permeability in nm s−1 (<25 poor, >500 great). log BB – predicted brain/blood partition coefficient (−3.0–1.2). log S – aqueous solubility of a compound (−6.5–0.5 mol dm−3). Rot – no. of rotatable bonds (0 to 15). |
6a |
284.32 |
567.436 |
0 |
3.5 |
3.038 |
343.769 |
−1.286 |
−4.161 |
6 |
6b |
312.374 |
636.971 |
0 |
3.5 |
3.92 |
429.085 |
−1.412 |
−5.153 |
8 |
6c |
340.427 |
711.635 |
0 |
3.5 |
4.822 |
498.442 |
−1.569 |
−6.242 |
10 |
6d |
368.481 |
743.435 |
0 |
3.5 |
5.268 |
305.705 |
−1.907 |
−6.519 |
12 |
6e |
404.448 |
584.577 |
1 |
5.0 |
3.838 |
605.209 |
−0.839 |
−4.174 |
7 |
6f |
383.796 |
530.836 |
0 |
6.5 |
2.171 |
267.446 |
−0.934 |
−2.154 |
6 |
6g |
318.337 |
564.382 |
0 |
3.5 |
3.461 |
357.559 |
−1.068 |
−4.428 |
4 |
6h |
336.328 |
567.032 |
0 |
3.5 |
3.647 |
353.871 |
−0.952 |
−4.683 |
4 |
6i |
352.782 |
586.704 |
0 |
3.5 |
3.94 |
351.095 |
−0.933 |
−5.163 |
4 |
6j |
397.233 |
589.559 |
0 |
3.5 |
4.025 |
354.108 |
−0.911 |
−5.242 |
4 |
6k |
332.364 |
594.968 |
0 |
3.5 |
3.794 |
375.045 |
−1.088 |
−5.008 |
4 |
6l |
374.444 |
636.388 |
0 |
3.5 |
4.559 |
358.01 |
−1.138 |
−5.63 |
5 |
6m |
334.337 |
570.734 |
1 |
4.25 |
2.794 |
106.664 |
−1.634 |
−4.336 |
5 |
6n |
348.363 |
629.418 |
0 |
4.25 |
3.834 |
519.109 |
−1.094 |
−5.105 |
5 |
6o |
386.336 |
598.845 |
0 |
3.5 |
4.344 |
358.282 |
−0.799 |
−5.59 |
4 |
6p |
363.335 |
610.671 |
0 |
4.5 |
2.762 |
42.587 |
−2.146 |
−4.619 |
5 |
6q |
363.335 |
630.124 |
0 |
4.5 |
3.014 |
61.546 |
−2.088 |
−4.988 |
5 |
7a |
312.374 |
631.212 |
0 |
3.5 |
3.818 |
475.547 |
−1.228 |
−5.369 |
6 |
7b |
340.427 |
671.361 |
0 |
3.5 |
4.387 |
413.083 |
−1.414 |
−5.715 |
8 |
7c |
368.481 |
753.598 |
0 |
3.5 |
5.352 |
506.822 |
−1.573 |
−7.037 |
10 |
7d |
396.534 |
826.137 |
0 |
3.5 |
6.173 |
496.042 |
−1.803 |
−8.086 |
12 |
7e |
432.502 |
774.439 |
1 |
5.0 |
4.777 |
146.42 |
−1.994 |
−7.549 |
7 |
7f |
411.85 |
546.498 |
0 |
6.5 |
2.611 |
358.622 |
−0.82 |
−2.398 |
6 |
7g |
346.391 |
593.336 |
0 |
3.5 |
3.9 |
361.81 |
−1.048 |
−4.977 |
4 |
7h |
425.287 |
623.302 |
0 |
3.5 |
4.458 |
341.091 |
−0.935 |
−5.879 |
4 |
7i |
360.418 |
620.804 |
0 |
3.5 |
4.176 |
339.567 |
−1.108 |
−5.498 |
4 |
7j |
362.39 |
591.857 |
1 |
4.25 |
3.165 |
104.014 |
−1.59 |
−4.71 |
5 |
7k |
376.417 |
617.631 |
0 |
4.25 |
4.029 |
420.983 |
−1.026 |
−4.882 |
5 |
7l |
414.389 |
700.508 |
0 |
3.5 |
5.396 |
514.904 |
−0.833 |
−7.521 |
4 |
7m |
391.388 |
639.666 |
0 |
4.5 |
3.217 |
43.007 |
−2.11 |
−5.169 |
5 |
7n |
391.388 |
559.1 |
0 |
4.5 |
2.89 |
93.403 |
−1.454 |
−3.642 |
5 |
10a |
395.463 |
752.156 |
2 |
8.0 |
2.322 |
85.992 |
−2.006 |
−4.778 |
7 |
10b |
390.404 |
576.151 |
2 |
9.0 |
1.653 |
229.916 |
−1.278 |
−2.688 |
7 |
11a |
395.463 |
765.567 |
2 |
8.0 |
2.482 |
90.546 |
−2.062 |
−4.838 |
8 |
11b |
409.49 |
800.267 |
2 |
8.0 |
2.778 |
87.077 |
−2.169 |
−5.447 |
8 |
11c |
404.43 |
778.651 |
2 |
9.0 |
2.694 |
104.37 |
−2.408 |
−5.789 |
8 |
11d |
391.388 |
720.921 |
1 |
6.0 |
3.206 |
49.823 |
−2.531 |
−6.191 |
6 |
12a |
423.517 |
841.741 |
2 |
8.0 |
3.099 |
110.476 |
−2.224 |
−6.492 |
8 |
12b |
437.544 |
842.109 |
2 |
8.0 |
3.317 |
87.762 |
−2.177 |
−6.169 |
8 |
12c |
432.484 |
835.341 |
2 |
9.0 |
3.272 |
107.993 |
−2.472 |
−6.752 |
8 |
The predicted values of these parameters revealed that all the parameters like mol. wt., hydrogen bond donors (HBD), hydrogen bond acceptors (HBA), solvent accessible surface area (SASA), partition co-efficient (log
P), predicted apparent Caco-2 cell permeability (PCaco), predicted brain/blood partition coefficient (log
BB), aqueous solubility (log
S) and number of rotatable bonds (Rot) were found within acceptable range and followed Lipinski rule of five. So overall, based upon the predicted values of these physico-chemical parameters, all the titled compounds possessed the drug-likeness behavior.
Docking studies
We performed molecular docking to investigate the binding mode of most active QSI 6p with LasR receptor by the X-ray structure (PDB ID: 2UV0). For the docking study, we selected co-crystal structure as the source of the target taken is from the organism P. aeruginosa LasR and its resolution is better compared to other available PDB structures. Moreover, all the titled compounds were screened against the strain of P. aeruginosa and hence we took the same species for docking to get better correlation.
Docking studies of the significantly active compound (6p) and least active compound (11b) was performed using Glide 5.9 (ref. 43) running on maestro version 9.4, to investigate their putative binding mode with the target protein. Enzyme used for the docking study was LasR receptor protein (PDB: 2UV0) of P. aeruginosa, retrieved from RCSB, Protein Data Bank.44 Protein preparation wizard of Schrödinger suite was used for preparation of target protein.
In order to predict the nature of binding mode of significant and least active compounds (6p and 11b respectively) with the target protein, docking studies were carried out. The value of RMSD obtained between X-ray pose and re-docked pose (Fig. 5) for co-crystallized ligand in target protein was found to be 1.55 Å, suggesting that docking protocol could be relied on for the docking studies. The best docked pose (docking score −8.62) view of the co-crystallized ligand inside the active site of the target protein (PDB ID: 2UV0) is shown in Fig. 6. The figure revealed that, the co-crystallized ligand exhibited four hydrogen bond interactions with TYR 56 (2.29 Å), TRP 60 (2.12 Å), ASP 73 (1.94 Å) and SER 129 (2.22 Å) amino acid residues of the protein and two pi–pi stacking interactions (3.39 and 3.36 Å) respectively.
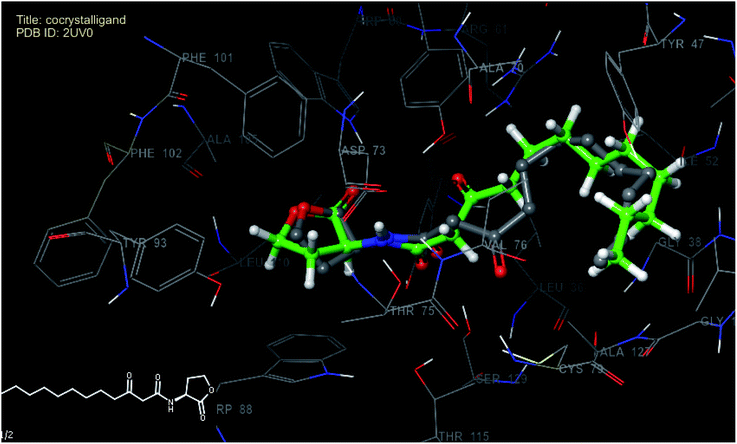 |
| Fig. 5 Superimposed view of best scoring native pose of co-crystallized (grey) ligand with its X-ray pose (green) inside the active site of 2UV0. | |
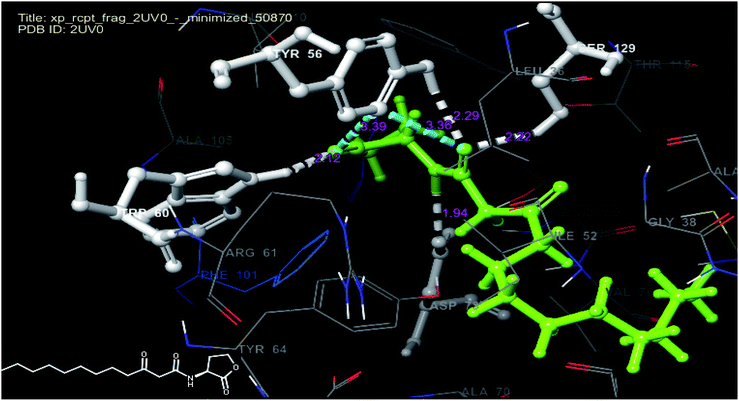 |
| Fig. 6 Docked pose view of co-crystallized ligand with interactions and distances (color interpretation: white-hydrogen bond, blue pi–pi stacking) inside the active site of 2UV0. | |
The analysis of best docked pose (Fig. 7) of compound 6p (docking score −8.40) revealed that, the compound showed hydrogen bond interaction with TYR 56 (2.08 Å) and ASP 73 (2.90 Å) as that of co-crystallized ligand and also formed hydrogen bond interactions with ARG 61 (2.25 Å), TYR-93 (3.76 Å), LEU-125 (3.25 Å) and TRP-88 (2.80 Å) residues of the target. The compound also exhibited three pi–pi stacking interactions with TYR-47 (2.25 Å), TRP-88 (2.80 Å) and PHE-101 (3.22 Å) residues of the target protein and hence might stabilize its binding affinity with the target receptor, consequently may be responsible for its significant in vitro activity. The best docked pose (Fig. 8) of compound 11b (docking score −9.06) displayed weak hydrogen bond interactions between TYR-64 (2.96 Å), TYR-93 (1.90 Å) and ASP 73 (3.37 Å) residues of target protein. The compound also exhibited pi–pi stacking interactions with Trp-88 (3.16 Å) residues. This weak binding affinity of compound 11b may be responsible for its lower activity in the in vitro assay.
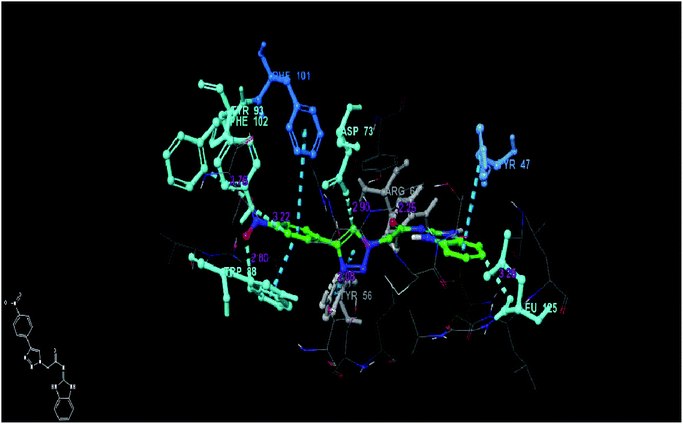 |
| Fig. 7 Docked pose view of compound 6p inside the 2UV0, showing interactions and distances (color interpretation: white-hydrogen bond, blue pi–pi stacking). | |
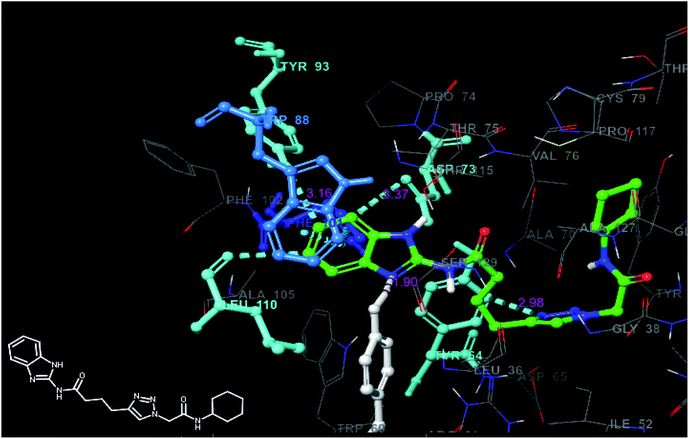 |
| Fig. 8 Docked pose view of compound 11b inside the 2UV0, showing interactions and distances (color interpretation: white-hydrogen bond, blue pi–pi stacking). | |
Single crystal X-ray crystallographic structure of compound 6d
The suitable crystals of the compound 6d for X-ray crystallographic study were grown from the mixture of methanol and dichloromethane (1
:
1) solution. The single crystal X-ray diffraction measurement of the molecule (C20H28N6O) was on Rigaku XtaLAB P200 diffract meter using graphite monochromated Cu-Ka radiation (λ = 1.54184 Å) on 0.5 mm × 0.02 mm × 0.01 mm colorless crystal. Data were collected and processed using CrysAlisPro (Rigaku Oxford Diffraction). The data were collected at a temperature of 293 K to a maximum 2q value of 159.794°. Of the 10
329 reflections were collected, where 4308 were unique (Rint = 0.0482) and equivalent reflections were merged. The crystal was kept at 293(2) K during data collection. Using Olex2, the structure was solved with the ShelX structure solution program using Direct Methods and refined with the ShelXL refinement package using least squares minimization. The basic crystallographic data are shown in Table 4. The molecular structure of the compound with methanol and dichloromethane solvents of crystallization is given as an ORTEP diagram in Fig. 9. The chain of nine carbons with C12 is directly bonded to C11 with more rigidity. As we move from C12 to C20, since the molecule is continuously undergoing vibration the terminal C20 with more flexibility experiences higher degree of vibrations as compare to C12. Therefore, the thermal parameters increase as we go from C12 to C20 and it is highest for C20. Crystallographic data for the compound 6d have been deposited to the Cambridge Crystallographic Data Center and corresponding deposition number is CCDC 1904042.
Table 4 Crystal data and structure refinement for 6d
Identification code |
6d (exp_399-AB-4R) |
Empirical formula |
C20H28N6O |
Formula weight |
368.48 |
Temperature/K |
293(2) |
Crystal system |
Monoclinic |
Space group |
P21/c |
a/Å |
18.8646(7) |
b/Å |
5.4019(2) |
c/Å |
20.0357(6) |
α/° |
90 |
β/° |
90.614(3) |
γ/° |
90 |
Volume/Å3 |
2041.61(12) |
Z |
4 |
ρcalcg/cm3 |
1.199 |
μ/mm−1 |
0.619 |
F(000) |
792.0 |
Crystal size/mm3 |
0.5 × 0.02 × 0.01 |
Radiation |
CuKα (λ = 1.54184) |
2Θ range for data collection/° |
8.828 to 159.794 |
Index ranges |
−23 ≤ h ≤ 23, −6 ≤ k ≤ 3, −23 ≤ l ≤ 25 |
Reflections collected |
10 329 |
Independent reflections |
4308 [Rint = 0.0482, Rsigma = 0.0635] |
Data/restraints/parameters |
4308/0/245 |
Goodness-of-fit on F2 |
1.063 |
Final R indexes [I ≥ 2σ (I)] |
R1 = 0.0671, wR2 = 0.1867 |
Final R indexes [all data] |
R1 = 0.0834, wR2 = 0.2036 |
Largest diff. peak/hole/e Å−3 |
0.42/−0.27 |
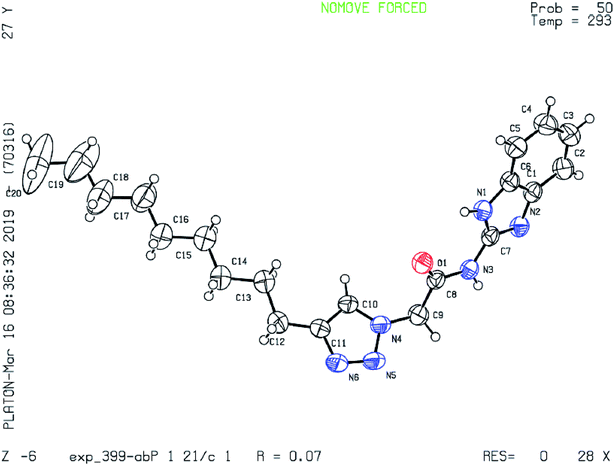 |
| Fig. 9 ORTEP crystal structure diagram of compound. | |
3. Conclusion
In conclusion, series of novel 1,2,3-triazole derivatives of 2-aminobenzimidazole and 2-amino-5,6-dimethylbenzimidazole were designed and synthesized. All synthesized compounds were characterised using 1H NMR, 13C NMR, mass spectrometry and elemental analyses. The QSI activity of all 40 final compounds was evaluated against P. aeruginosa MH602. Among all derivatives, three compounds, 6i, 6o, 6p and 7l exhibited promising QSI activity of 64.99, 64.25, 68.23 and 65.80% against P. aeruginosa MH602 at 250 μM, respectively. These three compounds also exhibited significant activity at low concentrations (125 and 62.5 μM). Like, previously reported triazole based AHL analogues (III and IV), 6p exhibited greatest QSI activity at low concentrations with 63.67% inhibition at 62.5 μM. These compounds were not found to be toxic when treated in normal cell line (HEK cell line) at 25, 50 and 100 μM. Hence, these compounds may become good starting point for further refinement in order to emerge as potent molecules with maximum therapeutic effect.
4. Experimental section
Materials and methods
All chemical reagents and solvents are purchased from Aldrich, Alfa Aesar, Finar. The solvents and reagents were of LR grade. All the solvents were dried and distilled before use. Thin-layer chromatography (TLC) was carried out on aluminium-supported silica gel plates (Merck 60 F254) with visualization of components by UV light (254 nm). Column chromatography was carried out on silica gel (Merck 100–200 mesh). 1H NMR and 13C NMR spectra were recorded at 400 MHz and 101 MHz respectively using a Bruker AV 400 spectrometer (Bruker CO., Switzerland) in CDCl3 and DMSO-d6 solution with tetramethylsilane as the internal standard and chemical shift values (δ) were given in ppm. 1H NMR spectra were recorded in CDCl3 or DMSO-d6. The following abbreviations are used to designate multiplicities: s = singlet, d = doublet, t = triplet, m = multiplet, br = broad. Melting points were determined on an electro thermal melting point apparatus (Stuart-SMP30) in open capillary tubes and are uncorrected. Elemental analyses were performed by Elementar Analysensysteme GmbH vario MICRO cube CHN Analyzer. Mass spectra (ESI-MS) were recorded on Schimadzu MS/ESI mass spectrometer.
General synthetic procedure for compounds 6a–q and 7a–n
A solution of azide (5a) (0.5 g, 2.3 mmol) in DMF
:
H2O (8
:
2) was reacted with various substituted acetylenes (0.24 g, 3.5 mmol) in the presence of sodium ascorbate (0.023 g, 5 mol%), CuSO4·5H2O (0.006 g, 1 mol%). The reaction mixture was stirred at rt for 6–24 h. The reaction mixture was monitored by TLC. Upon completion of the reaction, as indicated by TLC, the reaction was diluted with ethyl acetate and washed with water. Organic layer was dried over anhydrous sodium sulphate, concentrated under reduced pressure and the crude residue was purified by column chromatography using 60–90% ethyl acetate in hexane as eluent to give 0.57 g of the title compound N-(1H-benzo[d]imidazol-2-yl)-2-(4-propyl-1H-1,2,3-triazol-1-yl)acetamide (6a) (yield: 88%). Similar procedure was followed to get the compounds 6b–q and 7a–n from 5a and 5b respectively.
General synthetic procedure for 9a–c
To a solution of compound (2a) (0.5 g, 3.776 mmol) in DMF (8.0 mL) at 0 °C was added EDC–HCl (1.08 g, 5.64 mmol), diisopropyl ethylamine (1.33 mL, 9.4 mmol), and HOBt (0.76 g, 5.664 mmol). The reaction mixture was stirred at rt for 16 h. Upon completion of the reaction, as indicated by TLC, DCM was added to the reaction mixture and washed with 10% bicarbonate and brine solution. The organic layer was dried over anhydrous Na2SO4 and concentrated in vacuo. The crude product was purified by column chromatography using 50–70% ethyl acetate in hexane as eluent to yield 0.66 g of N-(1H-benzo[d]imidazol-2-yl)pent-4-ynamide (9a). Similar procedure was followed for preparation of 9a–c from 2a and 2b. Yield: 9a: 82%; 9b: 78% and 9c: 80% (pale brown solids).
General synthetic procedure for 10a–c, 11a–d and 12a–c
A solution of alkyne (9a) (0.5 g, 2.3 mmol) in DMF
:
H2O (8
:
2) was reacted with 2-azido-N-cyclohexylacetamide (B) (0.24 g, 2.34 mmol) in the presence of sodium ascorbate (0.023 g, 5 mol%), CuSO4·5H2O (0.006 g, 1 mol%). The reaction mixture was stirred at rt for 12 h. Upon completion of the reaction, as indicated by TLC, the reaction was diluted with ethyl acetate and washed with water. Organic layer was dried over anhydrous sodium sulphate, concentrated under reduced pressure and the crude residue was purified by column chromatography using 60–90% ethyl acetate in hexane as eluent to give 0.42 g of N-(1H-benzo[d]imidazol-2-yl)-3-(1-(2-(cyclohexylamino)-2-oxoethyl)-1H-1,2,3-triazol-4-yl)propanamide (10a; yield 46%). Similar procedure was followed in the synthesis of 10b, 11a–d and 12a–c from 9a, 9b and 9c respectively.
Analytical data for the final compounds
N-(1H-Benzo[d]imidazol-2-yl)-2-(4-propyl-1H-1,2,3-triazol-1-yl)acetamide (6a). Pale green; (88%); m.p. 238–240 °C; IR νmax (KBr, cm−1) 3392, 3124, 2959, 1637, 1598; 1H NMR (400 MHz, DMSO) δ 12.05 (s, 2H), 7.81 (s, 1H), 7.33 (dd, J = 5.9, 3.2 Hz, 2H), 6.98 (dd, J = 5.9, 3.2 Hz, 2H), 5.27 (s, 2H), 2.54 (t, 2H), 1.54 (sextet, 2H), 0.84 (t, 3H). 13C NMR (101 MHz, DMSO) δ 168.17
147.83, 147.04, 135.44, 124.02, 121.95, 114.13, 52.77, 27.47, 22.77, 14.0. ESI-MS: (m/z) calcd for C14H16N6O: 284.32, found 283.00 (M–H)+; anal. calcd for C14H16N6O: (%) C, 59.14; H, 5.67; N, 29.56; O, 5.63 found: C, 59.18; H, 5.63; N, 29.60; O, 5.59.
N-(1H-Benzo[d]imidazol-2-yl)-2-(4-pentyl-1H-1,2,3-triazol-1-yl)acetamide (6b). Off white; (76%); m.p. 224–226 °C; IR νmax (KBr, cm−1) 3386, 3141, 2957, 1639, 1592, 1403; 1H NMR (400 MHz, DMSO) δ 12.13 (s, 2H), 7.89 (s, 1H), 7.43 (dd, J = 5.9, 3.2 Hz, 2H), 7.12 (dd, J = 5.9, 3.2 Hz, 2H), 5.36 (s, 2H), 2.64 (t, 2H), 1.72–1.52 (m, 2H), 1.41–1.25 (m, 4H), 0.88 (t, 3H). 13C NMR (101 MHz, DMSO) δ 168.15, 147.81 (s), 147.19, 135.31, 123.89, 121.96, 114.12, 52.77, 31.22, 29.20, 25.39, 22.33, 14.36. ESI-MS: (m/z) calcd for C16H20N6O: 312.37, found 311.00 (M–H)+; anal. calcd for C16H20N6O: (%) C, 61.52; H, 6.45; N, 26.90; O, 5.12 found: C, 61.54; H, 6.43; N, 26.92; O, 5.10.
N-(1H-Benzo[d]imidazol-2-yl)-2-(4-heptyl-1H-1,2,3-triazol-1-yl)acetamide (6c). White; (63%); m.p. 218–220 °C; IR νmax (KBr, cm−1) 3373, 3144, 2948, 1632, 1594, 1401; 1H NMR (400 MHz, DMSO) δ 12.11 (s, 2H), 7.84 (s, 1H), 7.41 (dd, J = 5.9, 3.2 Hz, 2H), 7.032 (dd, J = 5.9, 3.2 Hz, 2H), 5.35 (s, 2H), 2.53 (t, 2H), 1.61–1.49 (m, 4H), 1.39–1.22 (m, 6H), 0.87 (t, 3H). 13C NMR (101 MHz, DMSO) δ 168.03, 147.69, 147.24, 135.08, 123.45, 121.74, 114.11, 52.18, 31.34, 29.20, 28.12, 25.39, 24.46, 22.33, 14.36. ESI-MS: (m/z) calcd for C18H24N6O: 340.42, found 339.00 (M–H)+; anal. calcd for C18H24N6O: (%) C, 63.51; H, 7.11; N, 24.69; O, 4.70 found: C, 63.54; H, 7.17; N, 24.66; O, 4.64.
N-(1H-Benzo[d]imidazol-2-yl)-2-(4-nonyl-1H-1,2,3-triazol-1-yl)acetamide (6d). White; (55%); m.p. 215–217 °C; IR νmax (KBr, cm−1) 3339, 3142, 2962, 1634, 1591, 1402; 1H NMR (400 MHz, DMSO) δ 12.13 (s, 2H), 7.89 (s, 1H), 7.45–7.40 (dd, J = 5.9, 3.2 Hz, 2H), 7.12 (dd, J = 5.9, 3.2 Hz, 2H), 5.35 (s, 2H), 2.66 (t, 2H), 1.59 (m, 2H), 1.36–1.23 (m, 12H), 0.86 (t, 3H). 13C NMR (101 MHz, DMSO) δ 168.01, 147.62, 147.13, 134.19, 122.46, 121.21, 113.08, 52.47, 31.34, 29.81, 29.74, 29.11, 28.12, 25.39, 24.46, 22.33, 14.36. ESI-MS: (m/z) calcd for C20H28N6O: 368.48, found 367.00 (M–H)+; anal. calcd for C20H28N6O: (%) C, 65.19; H, 7.66; N, 22.81; O, 4.34 found: C, 65.20; H, 7.69; N, 22.80; O, 4.31.
N-(1H-Benzo[d]imidazol-2-yl)-2-(4-nonyl-1H-1,2,3-triazol-1-yl)acetamide (6e). White; (92%); m.p. 201–203 °C; IR νmax (KBr, cm−1) 3391, 3136, 1641, 1583, 1399; 1H NMR (400 MHz, DMSO) δ 12.62 (s, 1H), 12.14 (s, 2H), 8.13 (s, 1H), 7.52 (m, 1H), 7.41 (dd, J = 5.9, 3.1 Hz, 3H), 7.24–6.99 (m, 4H), 5.38 (s, 2H), 4.68 (s, 2H). 13C NMR (101 MHz, DMSO) δ 168.27, 150.05, 147.99, 144.11, 143.56, 125.67, 122.03, 121.65, 117.94, 114.01, 110.87, 53.04, 28.28. ESI-MS: (m/z) calcd for C19H16N8OS: 404.45, found 403.00 (M–H)+; anal. calcd for C19H16N8OS: (%) C, 56.42; H, 3.99; N, 27.71; O, 3.96; S, 7.93 found: C, 56.38; H, 4.12; N, 27.69; O, 3.97; S, 7.97.
N-(1H-Benzo[d]imidazol-2-yl)-2-(4-((5-chloro-2-oxopyridin-1(2H)-yl)methyl)-1H-1,2,3-triazol-1-yl)acetamide (6f). White; (86%); m.p. 276–278 °C; IR νmax (KBr, cm−1) 3395, 3135, 1648, 1583, 1398; 1H NMR (400 MHz, DMSO) δ 12.15 (s, 2H), 8.14 (s, 1H), 8.10 (dd, J = 7.5, 3.0 Hz, 1H), 7.50 (dt, J = 8.9, 4.4 Hz, 1H), 7.45–7.35 (m, 2H), 7.19–7.06 (m, 2H), 6.46 (t, J = 8.9 Hz, 1H), 5.40 (s, 2H), 5.16 (s, 2H). 13C NMR (101 MHz, DMSO) δ 168.21, 160.26, 147.99, 142.43, 141.19, 137.22, 134.97, 126.12, 122.02, 121.37, 114.02, 110.86, 53.02, 44.02. ESI-MS: (m/z) calcd for C17H14ClN7O2: 383.79, found 382.00 (M–H)+; anal. calcd for C17H14ClN7O2: (%) C, 53.20; H, 3.68; Cl, 9.24; N, 25.55; O, 8.34 found: C, 53.25; H, 3.65; Cl, 9.28; N, 25.53; O, 8.30.
N-(1H-Benzo[d]imidazol-2-yl)-2-(4-phenyl-1H-1,2,3-triazol-1-yl)acetamide (6g). Brown; (45%); m.p. 272–274 °C; IR νmax (KBr, cm−1) 3385, 3101, 1646, 1595, 1401; 1H NMR (400 MHz, DMSO) δ 12.15 (s, 2H), 8.62 (m, 2H), 8.13 (s, 1H), 7.79–7.40 (m, 4H), 7.31–6.83 (m, 3H), 5.46 (s, 2H). 13C NMR (101 MHz, DMSO) δ 168.51, 147.23, 142.71, 141.38, 136.48, 135.82, 125.21, 124.98, 122.02, 121.37, 114.02, 54.01. ESI-MS: (m/z) calcd for C17H14N6O: 318.33, found 317.00 (M–H)+; anal. calcd for C17H14N6O: (%) C, 64.14; H, 4.43; N, 26.40; O, 5.03 found: C, 64.19; H, 4.42; N, 26.37; O, 5.01.
N-(1H-Benzo[d]imidazol-2-yl)-2-(4-(4-fluorophenyl)-1H-1,2,3-triazol-1-yl)acetamide (6h). White; (54%); m.p. 283–285 °C; IR νmax (KBr, cm−1) 3381, 3135, 1632, 1589, 1406. 1H NMR (400 MHz, DMSO) δ 12.09 (s, 2H), 8.36 (s, 1H), 7.87 (d, J = 8.5 Hz, 2H), 7.64 (m, 2H), 7.43 (dd, J = 6.7, 3.3 Hz, 2H), 7.12 (dd, J = 6.7, 3.3 Hz, 2H), 5.45 (s, 2H). 13C NMR (101 MHz, DMSO) δ 168.63, 157.42, 148.37, 145.71, 134.89, 131.87, 130.16, 128.56, 124.14, 122.10, 121.26, 113.91, 54.45. ESI-MS: (m/z) calcd for C17H13FN6O: 336.32, found 335.00 (M–1)+; anal. calcd for C17H13FN6O: (%) C, 60.71; H, 3.90; F, 5.65; N, 24.99; O, 4.76 found: C, 60.74; H, 3.92; F, 5.66; N, 24.96; O, 4.73.
N-(1H-Benzo[d]imidazol-2-yl)-2-(4-(4-chlorophenyl)-1H-1,2,3-triazol-1-yl)acetamide (6i). White; (52%); m.p. 269–271 °C; IR νmax (KBr, cm−1) 3348, 3137, 1644, 1597, 1403; 1H NMR (400 MHz, DMSO) δ 12.08 (s, 2H), 8.37 (s, 1H), 7.72 (d, J = 8.5 Hz, 2H), 7.54 (d, J = 8.5 Hz, 2H), 7.41 (dd, J = 6.7, 3.3 Hz, 2H), 7.16–7.11 (m, 2H), 5.47 (s, 2H). 13C NMR (101 MHz, DMSO) δ 168.65, 148.24, 146.52, 135.51, 132.45, 131.24, 130.86, 127.42, 123.87, 122.26, 121.27, 113.83, 53.41. ESI-MS: (m/z) calcd for C17H13ClN6O: 397.23, found 395.00 (M–2)+; anal. calcd for C17H13ClN6O: (%) C, 51.40; H, 3.30; Br, 20.12; N, 21.16; O, 4.03 found: C, 51.43; H, 3.32; Br, 20.10; N, 21.15; O, 4.04.
N-(1H-Benzo[d]imidazol-2-yl)-2-(4-(4-bromophenyl)-1H-1,2,3-triazol-1-yl)acetamide (6j). Brown; (82%); m.p. 292–294 °C; IR νmax (KBr, cm−1) 3354, 3112, 1629, 1594, 1403; 1H NMR (400 MHz, DMSO) δ 12.20 (s, 2H), 8.38 (s, 1H), 7.85 (d, J = 8.5 Hz, 2H), 7.67 (d, J = 8.5 Hz, 2H), 7.42 (dd, J = 6.7, 3.3 Hz, 2H), 7.18–7.08 (m, 2H), 5.46 (s, 2H). 13C NMR (101 MHz, DMSO) δ 168.63, 148.38, 145.63, 134.63, 132.40, 131.85, 130.52, 127.58, 123.86, 122.13, 121.28, 113.90, 53.41. ESI-MS: (m/z) calcd for C17H13BrN6O: 397.23, found 395.00 (M–2)+; anal. calcd for C17H13BrN6O: (%) C, 51.40; H, 3.30; Br, 20.12; N, 21.16; O, 4.03 found: C, 51.43; H, 3.32; Br, 20.10; N, 21.15; O, 4.04.
N-(1H-Benzo[d]imidazol-2-yl)-2-(4-(p-tolyl)-1H-1,2,3-triazol-1-yl)acetamide (6k). Off white; (70%); m.p. 281–283 °C; IR νmax (KBr, cm−1) 3376, 3132, 1645, 1597, 1405; 1H NMR (400 MHz, DMSO) δ 12.19 (s, 2H), 8.56 (s, 1H), 7.77 (d, J = 8.0 Hz, 2H), 7.42 (dd, J = 6.6, 3.3 Hz, 2H), 7.28 (d, J = 8.0 Hz, 2H), 7.17–7.09 (m, 2H), 5.44 (s, 2H), 2.34 (s, 3H). 13C NMR (101 MHz, DMSO) δ 168.51, 148.23, 146.73, 137.60, 129.73, 129.50, 128.50, 125.54, 123.06, 122.08, 113.95, 53.26, 21.30. ESI-MS: (m/z) calcd for C18H16N6O: 332.36, found 331.00 (M–H)+; anal. calcd for C18H16N6O: (%) C, 65.05; H, 4.85; N, 25.29; O, 4.81 found: C, 65.11; H, 4.82; N, 25.30; O, 4.83.
N-(1H-Benzo[d]imidazol-2-yl)-2-(4-(4-(tert-butyl)phenyl)-1H-1,2,3-triazol-1-yl)acetamide (6l). Off white; (67%); m.p. 265–267 °C; IR νmax (KBr, cm−1) 3362, 3123, 1642, 1599, 1404; 1H NMR (400 MHz, DMSO) δ 12.07 (s, 2H), 8.51 (s, 1H), 7.76 (d, J = 8.0 Hz, 2H), 7.41 (dd, J = 6.6, 3.3 Hz, 2H), 7.18 (d, J = 8.0 Hz, 2H), 7.15–7.05 (m, 2H), 5.42 (s, 2H), 1.33 (s, 9H). 13C NMR (101 MHz, DMSO) δ 168.43, 148.24, 146.71, 137.41, 129.01, 129.67, 128.61, 125.55, 123.09, 121.22, 114.05, 53.22, 35.79, 32.41. ESI-MS: (m/z) calcd for C21H22N6O: 374.44, found 373.00 (M–H)+; anal. calcd for C21H22N6O: (%) C, 67.36; H, 5.92; N, 22.44; O, 4.27 found: C, 67.39; H, 5.90; N, 22.41; O, 4.29.
N-(1H-Benzo[d]imidazol-2-yl)-2-(4-(4-hydroxyphenyl)-1H-1,2,3-triazol-1-yl)acetamide (6m). Brown gummy solid; (46%); IR νmax (KBr, cm−1) 3365, 3142, 1634, 1596, 1403; 1H NMR (400 MHz, DMSO) δ 12.13 (s, 2H), 8.38 (s, 1H), 7.72 (d, J = 8.5 Hz, 2H), 7.41 (dd, J = 6.7, 3.3 Hz, 2H), 7.16–7.11 (m, 2H), 6.91 (d, J = 8.5 Hz, 2H), 6.7 (bs, 1H), 5.57 (s, 2H). 13C NMR (101 MHz, DMSO) δ 168.43, 156.75, 148.23, 146.61, 135.42, 131.09, 130.81, 126.44, 123.88, 122.12, 121.23, 113.49, 54.98. ESI-MS: (m/z) calcd for C17H14N6O2: 334.33, found 333.00 (M–2)+; anal. calcd for C17H14N6O2: (%) C, 61.07; H, 4.22; N, 25.14; O, 9.57 found: C, 61.10; H, 4.21; N, 25.15; O, 9.54.
N-(1H-Benzo[d]imidazol-2-yl)-2-(4-(4-methoxyphenyl)-1H-1,2,3-triazol-1-yl)acetamide (6n). Pale yellow; (48%); m.p. 242–243 °C; IR νmax (KBr, cm−1) 3343, 3107, 1646, 1598, 1405; 1H NMR (400 MHz, DMSO) δ 12.16 (s, 2H), 8.36 (s, 1H), 7.75 (d, J = 8.4 Hz, 2H), 7.62 (d, J = 8.4 Hz, 2H), 7.40 (dd, J = 6.7, 3.3 Hz, 2H), 7.16–7.07 (m, 2H), 5.46 (s, 2H), 3.78 (s, 3H). 13C NMR (101 MHz, DMSO) δ 168.73, 159.41, 147.63, 135.13, 132.42, 131.87, 130.47, 127.64, 123.26, 122.14, 121.38, 113.91, 53.42, 54.47. ESI-MS: (m/z) calcd for C18H16N6O2: 348.36, found 347.00 (M–2)+; anal. calcd for C18H16N6O2: (%) C, 62.06; H, 4.63; N, 24.12; O, 9.19 found: C, 62.11; H, 4.61; N, 24.11; O, 9.17.
N-(1H-Benzo[d]imidazol-2-yl)-2-(4-(4-(trifluoromethyl)phenyl)-1H-1,2,3-triazol-1-yl)acetamide (6o). White; (72%); m.p. 286–288 °C; IR νmax (KBr, cm−1) 3383, 3107, 1654, 1599, 1404; 1H NMR (400 MHz, DMSO) δ 12.06 (s, 2H), 8.66 (s, 1H), 7.96 (d, J = 8.1 Hz, 2H), 7.69 (d, J = 8.3 Hz, 2H), 7.31–7.24 (m, 2H), 7.02–6.95 (m, 2H), 5.33 (s, 2H). 13C NMR (101 MHz, DMSO) δ 168.69, 148.44, 145.30, 135.23, 134.59, 128.62, 128.31, 126.44, 124.78, 123.38, 122.15, 113.87, 53.50. ESI-MS: (m/z) calcd for C18H13F3N6O: 386.33, found 385.00 (M–H)+; anal. calcd for C18H13F3N6O: (%) C, 55.96; H, 3.39; F, 14.75; N, 21.75; O, 4.14 found: C, 60.01; H, 3.40; F, 14.72; N, 21.71; O, 4.15.
N-(1H-Benzo[d]imidazol-2-yl)-2-(4-(4-nitrophenyl)-1H-1,2,3-triazol-1-yl)acetamide (6p). Pale yellow; (68%); m.p. 283–285 °C; IR νmax (KBr, cm−1) 3358, 3104, 1638, 1595, 1403; 1H NMR (400 MHz, DMSO) δ 12.28 (s, 2H), 8.96 (s, 1H), 8.40 (d, J = 8.9 Hz, 2H), 8.23 (d, J = 8.8 Hz, 2H), 7.48 (dd, J = 5.9, 3.2 Hz, 2H), 7.25–7.12 (m, 2H), 5.56 (s, 2H). 13C NMR (101 MHz, DMSO) δ 168.79, 148.54, 147.07, 147.78, 137.66, 126.37, 125.63, 124.93, 124.36, 122.19, 113.81, 53.62. ESI-MS: (m/z) calcd for C17H13N7O3: 363.33, found 362.00 (M–H)+; anal. calcd for C17H13N7O3: (%) C, 56.20; H, 3.61; N, 26.99; O, 13.21 found: C, C, 56.24; H, 3.59; N, 26.96; O, 13.22.
N-(1H-Benzo[d]imidazol-2-yl)-2-(4-(3-nitrophenyl)-1H-1,2,3-triazol-1-yl)acetamide (6q). Yellow; (64%); m.p. 240–242 °C; IR νmax (KBr, cm−1) 3357, 3120, 1642, 1596, 1403; 1H NMR (400 MHz, DMSO) 12.26 (s, 2H), 8.91 (s, 1H), 8.32 (m, 2H), 8.21 (d, J = 6.8 Hz, 1H), 7.79 (t, J = 7.8 Hz, 1H), 7.42 (dd, J = 5.5, 3.1 Hz, 2H), 7.13 (dd, J = 5.4, 3.2 Hz, 2H), 5.49 (s, 2H). 13C NMR (101 MHz, DMSO) δ 168.25, 148.44, 148.18, 147.13, 137.52, 1345.41, 133.46, 126.77, 125.47, 124.82, 124.53, 122.67, 113.22, 53.41. ESI-MS: (m/z) calcd for C17H13N7O3: 363.33, found 362.00 (M–H)+; anal. calcd for C17H13N7O3: (%) C, 56.20; H, 3.61; N, 26.99; O, 13.21 found: C, 56.22; H, 3.57; N, 26.97; O, 13.21.
N-(5,6-Dimethyl-1H-benzo[d]imidazol-2-yl)-2-(4-propyl-1H-1,2,3-triazol-1-yl)acetamide (7a). White; (73%); m.p. 241–243 °C; IR νmax (KBr, cm−1) 3367, 3134, 1692, 1598, 1401; 1H NMR (400 MHz, DMSO) δ 11.99 (s, 2H), 7.88 (s, 1H), 7.18 (s, 2H), 5.30 (s, 2H), 2.62 (t, 2H), 2.26 (s, 6H), 1.69–1.56 (m, 2H), 0.93 (t, 3H). 13C NMR (101 MHz, DMSO) δ 168.95, 148.07, 146.92, 132.79, 130.27, 123.90, 114.12, 53.13, 27.51, 22.79, 20.30, 14.05. ESI-MS: (m/z) calcd for C16H20N6O: 312.37, found 300.00 (M–H)+; anal. calcd for C16H20N6O: (%) C, 61.52; H, 6.45; N, 26.90; O, 5.12 found: C, 61.56; H, 6.43; N, 26.86; O, 5.14.
N-(5,6-Dimethyl-1H-benzo[d]imidazol-2-yl)-2-(4-pentyl-1H-1,2,3-triazol-1-yl)acetamide (7b). Off white; (66%); m.p. 200–202 °C; IR νmax (KBr, cm−1) 3374, 3132, 1682, 1584, 1407; 1H NMR (400 MHz, DMSO) δ 12.09 (s, 2H), 7.87 (s, 1H), 7.18 (s, 2H), 5.29 (s, 2H), 2.64 (t, 2H), 2.26 (s, 6H), 1.64–1.56 (m, 2H), 1.32 (m, 4H), 0.87 (t, 3H). 13C NMR (101 MHz, DMSO) δ 168.92, 147.98, 147.16, 132.91, 130.26, 123.85, 114.12, 53.14, 31.23, 29.19, 25.40, 22.33, 20.29, 14.35. ESI-MS: (m/z) calcd for C18H24N6O: 340.42, found 339.00 (M–H)+; anal. calcd for C18H24N6O: (%) C, 63.51; H, 7.11; N, 24.69; O, 4.70 found: C, 63.54; H, 7.13; N, 24.67; O, 4.67.
N-(5,6-Dimethyl-1H-benzo[d]imidazol-2-yl)-2-(4-heptyl-1H-1,2,3-triazol-1-yl)acetamide (7c). Grey colour; (57%); m.p. 209–211 °C; IR νmax (KBr, cm−1) 3369, 3124, 1691, 1594, 1403; 1H NMR (400 MHz, DMSO) δ 12.12 (s, 2H), 7.87 (s, 1H), 7.18 (s, 2H), 5.29 (s, 2H), 2.36 (t, 2H), 2.26 (s, 2H), 1.62–1.50 (m, 6H), 1.38–1.23 (m, 8H), 0.84 (t, 3H). 13C NMR (101 MHz, DMSO) δ 168.90, 147.96, 147.11, 132.91, 130.22, 123.81, 114.15, 53.16, 31.24, 29.13, 28.95, 25.41, 22.23, 20.29, 19.18, 14.36. ESI-MS: (m/z) calcd for C20H28N6O: 368.48, found 367.00 (M–H)+; anal. calcd for C20H28N6O: (%) C, 65.19; H, 7.66; N, 22.81; O, 4.34 found: C, 65.23; H, 7.64; N, 22.77; O, 4.36.
N-(5,6-Dimethyl-1H-benzo[d]imidazol-2-yl)-2-(4-nonyl-1H-1,2,3-triazol-1-yl)acetamide (7d). Off white; (55%); m.p. 194–196 °C; IR νmax (KBr, cm−1) 3358, 3112, 1684, 1598, 1406; 1H NMR (400 MHz, DMSO) δ 12.03 (s, 2H), 7.87 (s, 1H), 7.18 (s, 2H), 5.29 (s, 2H), 2.63 (t, 2H), 2.25 (s, 6H), 1.60 (m, 2H), 1.31–1.28 (m, 4H), 1.27–1.23 (s, 8H), 0.85 (t, 3H). 13C NMR (101 MHz, DMSO) δ 168.72, 148.47, 147.50, 133.55, 130.26, 123.66, 114.06, 53.13, 31.77, 29.54, 29.44, 29.29, 29.19, 29.05, 25.45, 22.57, 20.30, 14.42. ESI-MS: (m/z) calcd for C22H32N6O: 396.53, found 395.00 (M–H)+; anal. calcd for C22H32N6O: (%) C, 66.64; H, 8.13; N, 21.19; O, 4.03 found: C, 66.66; H, 8.11; N, 21.17; O, 4.05.
2-(4-(((1H-Benzo[d]imidazol-2-yl)thio)methyl)-1H-1,2,3-triazol-1-yl)-N-(5,6-dimethyl-1H-benzo[d]imidazol-2-yl)acetamide (7e). Off white; (84%); m.p. 194–196 °C; IR νmax (KBr, cm−1) 3371, 3132, 1691, 1594, 1405; 1H NMR (400 MHz, DMSO) δ 12.62 (s, 2H), 11.19 (s, 1H), 8.16 (s, 1H), 7.60 (m, 2H), 7.43 (dd, J = 5.9, 3.1 Hz, 2H), 7.19 (s, 2H), 5.37 (s, 2H), 4.43 (s, 2H), 2.31 (s, 6H). 13C NMR (101 MHz, DMSO) δ 168.26, 150.24, 148.01, 144.08, 143.53, 125.67, 122.04, 121.68, 117.91, 114.00, 110.67, 53.06, 28.48, 20.18. ESI-MS: (m/z) calcd for C21H20N8OS: 432.50, found 431.00 (M–H)+; anal. calcd for C21H20N8OS: (%) C, 58.32; H, 4.66; N, 25.91; O, 3.70; S, 7.41 found: C, 58.35; H, 4.68; N, 25.89; O, 3.69; S, 7.40.
2-(4-((5-Chloro-2-oxopyridin-1(2H)-yl)methyl)-1H-1,2,3-triazol-1-yl)-N-(5,6-dimethyl-1H-benzo[d]imidazol-2-yl)acetamide (7f). White; (83%); m.p. 281–283 °C; IR νmax (KBr, cm−1) 3369, 3131, 1694, 1593, 1409; 1H NMR (400 MHz, DMSO) δ 12.01 (s, 2H), 8.11 (m, 2H), 7.51 (dd, J = 9.7, 2.9 Hz, 1H), 7.17 (s, 2H), 6.47 (d, J = 9.7 Hz, 1H), 5.33 (s, 2H), 5.17 (s, 2H), 2.26 (s, 6H). 13C NMR (101 MHz, DMSO) δ 160.16, 142.36, 141.51, 141.51, 137.22, 136.84, 126.05, 121.37, 121.23, 113.99, 110.86, 53.41, 44.00, 20.29. ESI-MS: (m/z) calcd for C19H18ClN7O2: 411.84, found 410.00 (M–H)+; anal. calcd for C19H18ClN7O2: (%) C, 55.41; H, 4.41; Cl, 8.61; N, 23.81; O, 7.77 found: C, 55.44; H, 4.43; Cl, 8.60; N, 23.80; O, 7.73.
N-(5,6-Dimethyl-1H-benzo[d]imidazol-2-yl)-2-(4-phenyl-1H-1,2,3-triazol-1-yl)acetamide (7g). Dark brown gummy solid; (47%); IR νmax (KBr, cm−1) 3358, 3137, 1671, 1584, 1404; 1H NMR (400 MHz, DMSO) δ 12.03 (s, 2H), 8.37 (s, 1H), 7.73 (d, J = 7.8 Hz, 2H), 7.41–7.32 (m, 3H), 7.17 (s, 2H), 5.34 (s, 2H), 2.23 (s, 6H). 13C NMR (101 MHz, DMSO) δ 168.43, 147.18, 146.45, 136.89, 129.84, 1298.40, 127.51, 125.59, 122.34, 113.91, 53.25, 21.30. ESI-MS: (m/z) calcd for C19H18N6O: 346.39, found 345.00 (M–H)+; anal. calcd for C19H18N6O: (%) C, 65.88; H, 5.24; N, 24.26; O, 4.62 found: C, 65.90; H, 5.28; N, 24.24; O, 4.58.
2-(4-(4-Bromophenyl)-1H-1,2,3-triazol-1-yl)-N-(5,6-dimethyl-1H-benzo[d]imidazol-2-yl)acetamide (7h). White; (76%); m.p. 273–275 °C; IR νmax (KBr, cm−1) 3349, 3137, 1691, 1588, 1407; 1H NMR (400 MHz, DMSO) δ 12.04 (s, 2H), 8.55 (s, 1H), 7.41 (dd, J = 6.7, 3.3 Hz, 2H), 7.12–7.09 (m, 2H), 7.18 (s, 2H), 5.37 (s, 2H), 2.26 (s, 6H). 13C NMR (101 MHz, DMSO) δ 168.51, 148.17, 146.25, 137.11, 129.69, 129.41, 128.22, 125.69, 123.63, 122.34, 114.12, 53.24, 21.29. ESI-MS: (m/z) calcd for C19H17BrN6O: 425.28, found 424.00 (M–H)+; anal. calcd for C19H17BrN6O: (%) C, 53.66; H, 4.03; Br, 18.79; N, 19.76; O, 3.76, found: C, 53.71; H, 4.00; Br, 18.77; N, 19.78; O, 3.74.
N-(5,6-Dimethyl-1H-benzo[d]imidazol-2-yl)-2-(4-(p-tolyl)-1H-1,2,3-triazol-1-yl)acetamide (7i). Pale yellow; (55%); m.p. 258–260 °C; IR νmax (KBr, cm−1) 3386, 3127, 1639, 1592, 1404; 1H NMR (400 MHz, DMSO) δ 12.06 (s, 2H), 8.54 (s, 1H), 7.76 (d, J = 7.8 Hz, 2H), 7.27 (d, J = 7.8 Hz, 2H), 7.18 (s, 2H), 5.37 (s, 2H), 2.34 (s, 3H), 2.26 (s, 6H). 13C NMR (101 MHz, DMSO) δ 168.53, 148.17, 146.45, 137.89, 129.86, 129.40, 128.52, 125.59, 123.67, 122.33, 113.94, 53.25, 21.30, 20.24. ESI-MS: (m/z) calcd for C20H20N6O: 360.41, found 359.00 (M–H)+; anal. calcd for C20H20N6O: (%) C, 66.65; H, 5.59; N, 23.32; O, 4.44 found: C, 66.68; H, 5.56; N, 23.34; O, 4.42.
N-(5,6-Dimethyl-1H-benzo[d]imidazol-2-yl)-2-(4-(4-hydroxyphenyl)-1H-1,2,3-triazol-1-yl)acetamide (7j). Brown; (65%); m.p. 326–328 °C; IR νmax (KBr, cm−1) 3378, 3136, 1684, 1594, 1409; 1H NMR (400 MHz, DMSO) δ 11.85 (s, 2H), 8.49 (s, 1H), 7.83 (d, J = 8.1 Hz, 2H), 7.66 (d, J = 8.2 Hz, 2H), 7.19 (s, 2H), 6.83 (bs, 1H) 5.22 (s, 2H), 2.23 (s, 6H). 13C NMR (101 MHz, DMSO) δ 168.41, 148.34, 136.21, 131.69, 128.53, 128.24, 126.19, 126.37, 123.82, 122.39, 113.77, 53.58, 20.35. ESI-MS: (m/z) calcd for C19H18N6O2: 362.39, found 361.00 (M–H)+; anal. calcd for C19H18N6O2: (%) C, 62.97; H, 5.01; N, 23.19; O, 8.83 found: C, 62.99; H, 5.04; N, 23.16; O, 8.81.
N-(5,6-Dimethyl-1H-benzo[d]imidazol-2-yl)-2-(4-(4-methoxyphenyl)-1H-1,2,3-triazol-1-yl)acetamide (7k). Pale yellow; (58%); m.p. 283–285 °C; IR νmax (KBr, cm−1) 3338, 3127, 1672, 1598, 1402; 1H NMR (400 MHz, DMSO) δ 12.03 (s, 2H), 8.49 (s, 1H), 7.96 (d, J = 8.1 Hz, 2H), 7.81 (d, J = 8.2 Hz, 2H), 7.00 (s, 2H), 5.36 (s, 2H), 3.78 (s, 3H) 2.23 (s, 6H). 13C NMR (101 MHz, DMSO) δ 168.33, 148.42, 136.93, 131.17, 128.73, 126.81, 126.73, 123.64, 121.82, 113.37, 53.71, 51.47, 20.32. ESI-MS: (m/z) calcd for C20H20N6O2: 376.41, found 375.00 (M–H)+; anal. calcd for C20H20N6O2: (%) C, 63.82; H, 5.36; N, 22.33; O, 8.50 found: C, 63.87; H, 5.34; N, 22.32; O, 8.49.
N-(5,6-Dimethyl-1H-benzo[d]imidazol-2-yl)-2-(4-(4-(trifluoromethyl)phenyl)-1H-1,2,3-triazol-1-yl)acetamide (7l). Pale yellow; (66%); m.p. 273–275 °C; IR νmax (KBr, cm−1) 3385, 3142, 1694, 1527, 1402; 1H NMR (400 MHz, DMSO) δ 11.93 (s, 2H), 8.64 (s, 1H), 7.96 (d, J = 8.1 Hz, 2H), 7.68 (d, J = 8.2 Hz, 2H), 7.03 (s, 2H), 5.22 (s, 2H), 2.10 (s, 6H). 13C NMR (101 MHz, DMSO) δ 169.69, 145.24, 135.29, 130.54, 128.56, 128.24, 126.45, 126.09, 124.74, 123.39, 113.78, 53.91, 20.28. ESI-MS: (m/z) calcd for C20H17F3N6O: 314.38, found 313.00 (M–H)+; anal. calcd for C20H17F3N6O: (%) C, 57.97; H, 4.14; F, 13.75; N, 20.28; O, 3.86 ffound: C, 57.99; H, 4.18; F, 13.74; N, 20.26; O, 3.83.
N-(5,6-Dimethyl-1H-benzo[d]imidazol-2-yl)-2-(4-(4-nitrophenyl)-1H-1,2,3-triazol-1-yl)acetamide (7m). Pale yellow; (71%); m.p. 288–289 °C; IR νmax (KBr, cm−1) 3346, 3148, 1686, 1585, 1406; 1H NMR (400 MHz, DMSO) δ 12.09 (s, 2H), 8.89 (s, 1H), 8.31 (d, J = 7.9 Hz, 2H), 8.16 (d, J = 7.9 Hz, 2H), 7.18 (s, 2H), 5.42 (s, 2H), 2.26 (s, 6H). 13C NMR (101 MHz, DMSO) δ 169.68, 145.28, 135.45, 131.07, 128.67, 128.38, 127.02, 126.01, 124.75, 123.43, 113.76, 53.92, 20.29. ESI-MS: (m/z) calcd for C19H17N7O3: 391.38, found 390.00 (M–H)+; anal. calcd for C19H17N7O3: (%) C, 58.31; H, 4.38; N, 25.05; O, 12.26 found: C, 58.33; H, 4.42; N, 25.01; O, 12.24.
N-(5,6-Dimethyl-1H-benzo[d]imidazol-2-yl)-2-(4-(3-nitrophenyl)-1H-1,2,3-triazol-1-yl)acetamide (7n). Yellow; (67%); m.p. 277–279 °C; IR νmax (KBr, cm−1) 3328, 3137, 1649, 1598, 1389; 1H NMR (400 MHz, DMSO) δ 12.08 (s, 2H), 8.89 (s, 1H), 8.73–8.63 (m, 1H), 8.33 (d, J = 7.9 Hz, 1H), 8.21 (d, J = 7.8, 1H), 7.78 (t, J = 8.0 Hz, 1H), 7.18 (s, 2H), 5.41 (s, 2H), 2.26 (s, 6H). 13C NMR (101 MHz, DMSO) δ 169.67, 145.21, 135.34, 134.81, 131.18, 130.72, 128.69, 128.38, 127.13, 126.11, 124.74, 123.23, 113.77, 53.82, 20.27. ESI-MS: (m/z) calcd for C19H17N7O3: 391.38, found 390.00 (M–H)+; anal. calcd for C19H17N7O3: (%) C, 58.31; H, 4.38; N, 25.05; O, 12.26 found: C, 58.32; H, 4.43; N, 25.02; O, 12.23.
N-(1H-Benzo[d]imidazol-2-yl)-3-(1-(2-(cyclohexylamino)-2-oxoethyl)-1H-1,2,3-triazol-4-yl)propanamide (10a). White; (46%); m.p. 328–330 °C; IR νmax (KBr, cm−1) 3384, 3137, 1648, 1579, 1408; 1H NMR (400 MHz, DMSO) 12.04 (s, 1H), 11.54 (s, 1H), 8.32 (d, 1H), 7.81 (s, 1H), 7.48 (dd, J = 5.9, 3.2 Hz, 2H), 7.05 (dd, J = 5.9, 3.2 Hz, 2H), 4.98 (s, 2H), 3.84 (p, 1H), 2.76 (t, 2H) 2.61 (t, 2H) 2.14–1.18 (m, 10H). 13C NMR (101 MHz, DMSO) δ 172.75, 165.22, 146.48, 124.96, 124.49, 121.61, 121.67, 121.44, 51.96, 50.83, 32.64, 31.11, 25.06, 24.38, 23.86. ESI-MS: (m/z) calcd for C20H25N7O2: 395.46, found 394.00 (M–H)+; anal. calcd for C20H25N7O2: (%) C, 60.74; H, 6.37; N, 24.79; O, 8.09 found: C, 60.78; H, 6.39; N, 24.77; O, 8.05.
N-(1H-Benzo[d]imidazol-2-yl)-3-(1-(2-oxo-2-(pyridin-2-ylamino)ethyl)-1H-1,2,3-triazol-4-yl)propanamide (10b). White; (63%); m.p. 286–288 °C; IR νmax (KBr, cm−1) 3378, 3131, 1643, 1569, 1405; 1H NMR (400 MHz, DMSO) δ 12.14 (s, 1H), 11.30 (s, 1H), 10.96 (s, 1H), 8.36 (s, 1H), 7.95–7.79 (m, 3H), 7.68–7.57 (m, 1H), 7.53–7.11 (m, 4H), 5.38 (s, 2H), 3.04–2.60 (m, 4H). 13C NMR (101 MHz, DMSO) δ 171.92, 165.73, 151.89, 148.61, 145.99, 145.84, 139.15, 138.87, 124.38, 124.30, 121.69, 120.34, 113.96, 52.55, 31.13, 21.07. ESI-MS: (m/z) calcd for C19H18N8O2: 390.40, found 389.00 (M–H)+; anal. calcd for C19H18N8O2: (%) C, 58.45; H, 4.65; N, 28.70; O, 8.20 found: C, 58.49; H, 4.62; N, 28.66; O, 8.23.
N-(1H-Benzo[d]imidazol-2-yl)-4-(1-(2-(cyclopentylamino)-2-oxoethyl)-1H-1,2,3-triazol-4-yl)butanamide (11a). White; (62%); m.p. 261–263 °C; IR νmax (KBr, cm−1) 3372, 3131, 1660, 1577, 1420; 1H NMR (400 MHz, DMSO) 12.07 (s, 1H), 11.50 (s, 1H), 8.30 (d, 1H), 7.84 (s, 1H), 7.47 (dd, J = 5.9, 3.2 Hz, 2H), 7.07 (dd, J = 5.9, 3.2 Hz, 2H), 4.99 (s, 2H), 4.00 (p, 1H), 2.81 (t, 2H) 2.79 (m, 1H), 2.08–1.29 (m, 11H). 13C NMR (101 MHz, DMSO) 172.70, 165.23, 146.47, 124.16, 124.00, 121.61, 121.52, 121.39, 51.98, 51.02, 32.69, 31.14, 25.06, 24.91, 23.85. ESI-MS: (m/z) calcd for C20H25N7O2: 396.46, found 395.00 (M–H)+; anal. calcd for C20H25N7O2: (%) C, 60.74; H, 6.37; N, 24.79; O, 8.09 found: C, 60.79; H, 6.34; N, 24.78; O, 8.08.
N-(1H-Benzo[d]imidazol-2-yl)-4-(1-(2-(cyclohexylamino)-2-oxoethyl)-1H-1,2,3-triazol-4-yl)butanamide (11b). White; (58%); m.p. 199–201 °C; IR νmax (KBr, cm−1) 3383, 3133, 1637, 1566, 1402; 1H NMR (400 MHz, DMSO) 12.09 (s, 1H), 11.57 (s, 1H), 8.31 (d, 1H), 7.86 (s, 1H), 7.51 (dd, J = 5.9, 3.2 Hz, 2H), 7.14 (dd, J = 5.9, 3.2 Hz, 2H), 4.97 (s, 2H), 3.89 (p, 1H), 2.69 (t, 2H) 2.62 (t, 2H), 2.14–1.18 (m, 12H). 13C NMR (101 MHz, DMSO) δ 172.71, 165.82, 146.73, 124.83, 124.47, 121.61, 121.66, 121.49, 51.95, 50.82, 32.66, 31.17, 27.53, 25.26, 24.40, 23.83. ESI-MS: (m/z) calcd for C21H27N7O2: 409.48, found 394.00 (M–H)+; anal. calcd for C21H27N7O2: (%) C, 61.60; H, 6.65; N, 23.94; O, 7.81 found: C, 61.64; H, 6.61; N, 23.92; O, 7.83.
N-(1H-Benzo[d]imidazol-2-yl)-4-(1-(2-oxo-2-(pyridin-2-ylamino)ethyl)-1H-1,2,3-triazol-4-yl)butanamide (11c). White; (68%); m.p. 258–259 °C; IR νmax (KBr, cm−1) 3394, 3125, 1652, 1563, 1404; 1H NMR (400 MHz, DMSO) δ 12.24 (s, 1H), 11.54 (s, 1H), 10.97 (s, 1H), 8.37 (s, 1H), 7.97 (m, 2H), 7.74 (dd, J = 5.8, 3.5 Hz, 3H), 7.15 (m, 1H), 7.08 (m, 2H), 5.37 (s, 2H), 2.73 (t, 2H), 2.59 (t, 2H), 1.93 (p, 2H). 13C NMR (101 MHz, DMSO) δ 176.80, 165.75, 151.90, 148.61, 146.79, 133.93, 124.47, 124.29, 121.65, 121.45, 120.37, 116.09, 113.98, 52.51, 33.55, 31.27, 24.93. ESI-MS: (m/z) calcd for C20H20N8O2: 304.43, found 403.00 (M–H)+; anal. calcd for C20H20N8O2: (%) C, 59.40; H, 4.98; N, 27.71; O, 7.91 found: C, 59.43; H, 4.96; N, 27.68; O, 7.93.
N-(1H-Benzo[d]imidazol-2-yl)-4-(1-(4-nitrophenyl)-1H-1,2,3-triazol-4-yl)butanamide (11d). Yellow; (59%); m.p. 263–265 °C; IR νmax (KBr, cm−1) 3396, 3143, 1654, 1566, 1405; 1H NMR (400 MHz, DMSO) δ 12.08 (s, 1H), 11.61 (s, 1H), 8.93 (s, 1H), 8.51 (d, J = 8.9 Hz, 2H), 8.32 (d, J = 8.8 Hz, 2H), 7.43 (dd, J = 5.9, 3.2 Hz, 2H), 7.25–7.12 (m, 2H), 2.83 (t, 2H), 2.61 (t, 2H), 1.98 (p, 2H). 13C NMR (101 MHz, DMSO) δ 168.72, 148.57, 147.37, 147.71, 137.26, 126.57, 125.63, 124.98, 124.31, 122.14, 113.88, 33.69, 31.73, 24.28. ESI-MS: (m/z) calcd for C19H17N7O3: 391.38, found 390.00 (M–H)+; anal. calcd for C19H17N7O3: (%) C, 58.31; H, 4.38; N, 25.05; O, 12.26 found: C, 58.35; H, 4.41; N, 25.02; O, 12.22.
4-(1-(2-(Cyclopentylamino)-2-oxoethyl)-1H-1,2,3-triazol-4-yl)-N-(5,6-dimethyl-1H-benzo[d]imidazol-2-yl)butanamide (12a). White; (47%); m.p. 252–254 °C; IR νmax (KBr, cm−1) 3377, 3148, 1647, 1582, 1404; 1H NMR (400 MHz, DMSO) δ 12.08 (s, 1H), 11.57 (s, 1H), 10.91 (s, 1H), 8.34 (s, 1H), 7.44 (s, 2H), 4.94 (s, 2H), 3.61 (m, 1H), 2.65 (t, 2H) 2.4–1.86 (m, 12H), 1.67–1.33 (m, 6H). 13C NMR (101 MHz, DMSO) δ 172.74, 165.37, 146.38, 124.91, 124.80, 121.49, 121.24, 121.45, 51.96, 51.02, 35.64, 34.45, 32.57, 32.29, 25.05, 24.83. ESI-MS: (m/z) calcd for C22H29N7O2: 423.51, found 422.00 (M–H)+; anal. calcd for C22H29N7O2: (%) C, 62.39; H, 6.90; N, 23.15; O, 7.56 found: C, 62.37; H, 6.88; N, 23.17; O, 7.58.
4-(1-(2-(Cyclohexylamino)-2-oxoethyl)-1H-1,2,3-triazol-4-yl)-N-(5,6-dimethyl-1H-benzo[d]imidazol-2-yl)butanamide (12b). White; (46%); m.p. 282–285 °C; IR νmax (KBr, cm−1) 3393, 3127, 1682, 1582, 1408; 1H NMR (400 MHz, DMSO) δ 12.05 (s, 1H), 11.53 (s, 1H), 10.78 (s, 1H), 8.32 (s, 1H), 7.82 (s, 2H), 4.98 (s, 2H), 3.54 (m, 1H), 2.64 (t, 2H) 2.4–1.5 (m, 12H), 1.31–1.12 (m, 8H). 13C NMR (101 MHz, DMSO) δ 172.69, 165.37, 146.42, 124.11, 124.08, 121.19, 121.22, 121.49, 51.98, 51.02, 35.37, 34.27, 32.69, 32.14, 25.06, 24.92, 23.86. ESI-MS: (m/z) calcd for C23H31N7O2: 437.54, found 436.00 (M–H)+; anal. calcd for C23H31N7O2: (%) C, 63.14; H, 7.14; N, 22.41; O, 7.31 found: C, 63.17; H, 7.11; N, 22.43; O, 7.29.
N-(5,6-Dimethyl-1H-benzo[d]imidazol-2-yl)-4-(1-(2-oxo-2-(pyridin-2-ylamino)ethyl)-1H-1,2,3-triazol-4-yl)butanamide (12c). White; (45%); m.p. 273–275 °C; IR νmax (KBr, cm−1) 3314, 3127, 1639, 1571, 1407; 1H NMR (400 MHz, DMSO) δ 12.45 (s, 1H), 11.54 (s, 1H), 10.96 (s, 1H), 8.44 (s, 1H), 7.47 (s, 2H), 5.18 (s, 2H), 2.27 (t, 2H), 2.59 (s, 6H), 2.48 (t, 2H), 1.78 (p, 2H). 13C NMR (101 MHz, DMSO) δ 174.84, 165.71, 150.91, 148.66, 146.19, 133.43, 124.46, 123.27, 121.45, 121.43, 120.37, 116.14, 113.88, 52.54, 33.54, 31.54, 24.97. ESI-MS: (m/z) calcd for C22H24N8O2: 432.48, found 431.00 (M–H)+; anal. calcd for C22H24N8O2: (%) C, 61.10; H, 5.59; N, 25.91; O, 7.40 found: C, 61.14; H, 5.57; N, 25.87; O, 7.38.
Quorum sensing inhibition assay
Antibacterial activities of the synthesized compounds were evaluated by using P. aeruginosa MH602 lasB reporter strain (PlasB: gfp(ASV)) following the protocol developed by Hentzer et al.37 The production of AHL signals by this reporter strain leads to an increase in product of unstable green fluorescent protein (GFP-ASV) as a function of an active QS system.
AHL dependent GFP expressing P. aeruginosa MH602 PlasB-gfp(ASV) harbouring a chromosomal fusion of the lasB promoter expressing Gfp(ASV) in response to 3-oxo-dodecanoyl HSL strains were cultured overnight in LB10. Strains were diluted (1
:
100) in ABT medium supplemented with 0.25% tryptone and 0.13% yeast extract and 200 μL of aliquots were dispensed to flat bottom 96-well plate wells (Sarstedt Australia). Cultures were supplemented with varying concentrations of synthetic compounds dissolved in DMSO. Control cultures were supplemented with equal amount of DMSO (1%). Plates were sealed with self-adhesive microplate sealers (TopSeal-A, PerkinElmer) to allow air diffusion and to prevent condensation. Cultures were incubated in a plate reader (EnSight Multimode Plate Reader, PerkinElmer) at 37 °C with shaking briefly prior to each reading and fluorescence (excitation, 485 nm; emission, 535 nm) and OD600 of cultures were measured every 30 min over 20 h. Ampicillin (100 μg mL−1) and gentamicin (20 μg mL−1) were supplemented to P. aeruginosa MH602 cultures.
Cytotoxicity assay
MTT assay. Cytotoxicity of the novel promising compounds was determined using MTT assay. 45 7.5 × 103 cells were seeded in 96 well plates and incubated overnight. Cells were treated with synthesized compounds at three concentrations (50 μM, 25 μM and 10 μM) in duplicates and incubated for 24 h. 50 μL of 5 mg mL−1 3-(4,5-dimethylthiazol-2-yl)-2,5-diphenyltetrazolium bromide (MTT; Himedia Laboratories Pvt. Ltd., Mumbai, India) was added and incubated for 4 h. Formazan crystals were dissolved using DMSO and absorbance was measured using Spectramax M4 (Molecular Devices, USA).
Cell culture and assay procedure
All the compounds tested for their cytotoxicity against Human Embryonic Kidney (HEK) cell lines (procured from National Centre for Cell Science, Pune, India) were cultured in DMEM (high glucose media: AL007S, Dulbecco's modified eagle medium) with 10% fetal bovine serum (FBS) and 1% antibiotic (Pen strep: A001) incubated at 37 °C and 5% CO2 atmosphere. All reagents were purchased from Himedia Laboratories Pvt. Ltd., Mumbai, India. Cells were seeded in a 96-well plate (Eppendorf 0030730119) with 100 μL of cell suspension containing 104 cells per well and incubated overnight. The DMSO solutions of the synthesized compounds and BG45 were prepared and they were further diluted to their respective concentrations of 25 μM, 50 μM, and 100 μM with the DMEM complete media. The cells were then treated with 100 μL of the compound solutions made in media along with a blank control containing DMSO in medium and BG45 as positive control and were incubated for 24 h. The culture medium was aspirated and subsequently, 50 μL of 5 mg mL−1 concentrated solution of MTT (3-(4,5-dimethylthiazol-2-yl)-2,5-diphenyltetrazolium bromide) in phenol red free DMEM was prepared and added in each well and further incubated for 3 h for the formation of formazan crystals. Later, 100 μL DMSO was added to the culture after aspirating media in the wells to dissolve the formazan crystals and the absorbance was measured using multi-well plate reader Spectramax (Molecular Devices, USA) at two different wavelengths of 570 nm and 650 nm. The % cell viability was calculated as a fraction of absorbance obtained from the treated cells from the absorbance of untreated control cells.37
Conflicts of interest
There are no conflicts of interest to declare.
Acknowledgements
KVGCS and SM thank DBT, New Delhi [BT/IN/Spain/39/SMl2017-18] for providing financial support. The financial assistance provided by DIST FIST grant (SR/FST/CSI-240/2012), New Delhi is gratefully acknowledged. SS thanks CSIR for providing SRF fellowship. Central analytical lab facilities of BITS Pilani Hyderabad campus are gratefully acknowledged. We are also thankful to UNSW School of Biotechnology and Biomolecular Sciences and UNSW School of Medical Sciences for their support.
References
- M. Boyer and F. Wisniewski-Dyé, FEMS Microbiol. Ecol., 2009, 1, 1–19 CrossRef PubMed.
- G. D. Geske, J. C. O'Neill, D. M. Miller, M. E. Mattmann and H. E. Blackwell, J. Am. Chem. Soc., 2007, 129, 13613–13625 CrossRef CAS PubMed.
- D. McDougald, W. H. Lin, S. A. Rice and S. Kjelleberg, Biofouling, 2006, 22, 133–144 CrossRef CAS PubMed.
- M. E. Mattmann and H. E. Blackwell, J. Org. Chem., 2010, 75, 6737–6746 CrossRef CAS PubMed.
- A. Eberhard, A. L. Burlingame, C. Eberhard, G. L. Kenyon, K. H. Nealson and N. J. Oppenheimer, Biochemistry, 1981, 20, 2444–2449 CrossRef CAS PubMed.
- D. A. Higgins, M. E. Pomianek, C. M. Kraml, R. K. Taylor, M. F. Semmelhack and B. L. Bassler, Nature, 2007, 45, 883–886 CrossRef PubMed.
- M. M. Marketon, M. R. Gronquist, A. Eberhard and J. E. González, J. Bacteriol., 2002, 184, 5686–5695 CrossRef CAS PubMed.
- https://www.cdc.gov/hai/organisms/gram-negative-bacteria.html.
- H. A. Khan, F. K. Baig and R. Mehboob, Asian Pac. J. Trop. Med., 2017, 7, 478–482 CrossRef.
- N. A. Bhawsar and M. Singh, Int. J. Adv. Res., 2014, 6, 778–783 Search PubMed.
- R. Manfredi, A. Nanetti, M. Ferri and F. Chiodo, Eur. J. Clin. Microbiol. Infect. Dis., 2000, 19, 248–253 CrossRef CAS PubMed.
- A. R. Hauser and J. Rello, Severe Infections Caused by Pseudomonas Aeruginosa, 2003, http://www.springer.com/gp/book/9781402074219(book) Search PubMed.
- C. Van Delden and B. H. Iglewski, Emerging Infect. Dis., 1998, 4, 551–560 CrossRef CAS PubMed.
- http://www.who.int/medicines/publications/WHO-PPL-Short_Summary_25FebET_NM_WHO.pdf.
- http://www.who.int/news-room/fact-sheets/detail/antibiotic-resistance.
- L. Fulghesu, C. Giallorenzo and D. Savoia, J. Chemother., 2007, 19, 388–391 CrossRef CAS PubMed.
- R. Kerstin, S. Anke and H. Volkhard, Perspect. Med. Chem., 2016, 8, 1–15 Search PubMed.
- N. Ni, M. Li, J. Wang and B. Wa, Med. Res. Rev., 2009, 29, 65–124 CrossRef CAS PubMed.
- C. Y. Chang, T. Krishnan, H. Wang, Y. Chen, W. F. Yin, Y. M. Chong, L. Y. Tan, T. M. Chong and K. G. Chan, Sci. Rep., 2014, 4, 1–8 Search PubMed.
- Y. Peng, J. Bi, J. Shi, Y. Li, X. Ye, X. Chen and Z. Yao, Am. J. Infect. Control, 2014, 42, 1308–1311 CrossRef PubMed.
- L. C. Antunes, R. B. Ferreira, M. M. Buckner and B. B. Finlay, Microbiology, 2010, 156, 2271–2282 CrossRef CAS PubMed.
- P. Williams and M. Cámara, Curr. Opin. Microbiol., 2009, 12, 182–191 CrossRef CAS PubMed.
- https://www.springer.com/in/book/9788132219811.
- S. Srinivasarao, S. Nizalapur, T. T. Yu, D. S. Wenholz, P. Trivedi, B. Ghosh, K. Rangan, N. Kumar and K. V. G. Chandra Sekhar, ChemistrySelect, 2018, 3, 9170–9180 CrossRef CAS.
- J. Musk, J. Dinty and P. J. Hergenrother, Curr. Med. Chem., 2006, 13, 2163–2177 CrossRef PubMed.
- H. O. Sintim, J. A. Smith, J. Wang, S. Nakayama and L. Yan, Future Med. Chem., 2010, 2, 1005–1035 CrossRef CAS PubMed.
- J. J. Richards and C. Melander, Anti-Infect. Agents Med. Chem., 2009, 4, 295–314 CrossRef.
- R. Frei, A. S. Breitbach and H. E. Blackwell, Angew. Chem., Int. Ed., 2012, 51, 5226–5229 CrossRef CAS PubMed.
- J. D Moore, J. P. Gerdt, N. R. Eibergen and H. E. Blackwell, ChemBioChem, 2014, 3, 435–442 CrossRef PubMed.
- D. Dheer, V. Singh and R. Shankar, Bioorg. Chem., 2017, 71, 30–54 CrossRef CAS PubMed.
- D. M. Stacy, S. T. Le Quement, C. L. Hansen, J. W. Clausen, T. T. Nielsen, J. W. Brummond, M. Givskov, T. E. Nielsen and H. E. Blackwella, Org. Biomol. Chem., 2013, 11, 938–954 RSC.
- M. R. Hansen, T. H. Jakobsen, C. G. Bang, A. E. Cohrt, C. L. Hansen, J. W. Clausen, S. T. Le Quement, T. T. Nielsen, M. Givskov and T. E. Nielsen, Bioorg. Med. Chem., 2015, 23, 1638–1650 CrossRef CAS PubMed.
- G. Brackman, M. Risseeuw, S. Celen, P. Cos, L. Maes, H. J. Nelis, S. V. Calenbergh and T. Coenye, Bioorg. Med. Chem., 2012, 20, 4737–4743 CrossRef CAS PubMed.
- H. Nagesh, S. Singireddi, A. Suresh, S. Nizalapur, S. Murugesan, K. Kanneboina, N. Kumar and K. V. G. Chandra Sekhar, ChemistrySelect, 2018, 3, 7565–7571 CrossRef CAS.
- S. Nizalapur, O. Kimyon, E. Yee, M. M. Bhadbhade, M. Manefield, M. Willcox, D. S. Black and N. Kumar, Org. Biomol. Chem., 2017, 15, 5743–5755 RSC.
- https://patents.google.com/patent/WO2012174312A3/en.
- M. Hentzer, K. Riedel, T. B. Rasmussen, A. Heydorn, J. B. Andersen, M. R. Parsek, S. A. Rice, L. Eberl, S. Molin and N. Høiby, Microbiology, 2002, 148, 87–102 CrossRef CAS PubMed.
- J. Van Meerloo, G. J. Kaspers and J. Cloos, Methods Mol. Biol., 2011, 731, 237–245 CrossRef CAS PubMed.
- Qikprop, Schrödinger, LLC, New York, version 3.8, 2013 Search PubMed.
- S. Chander, A. Penta and S. Murugesan, J. Pha. Res., 2014, 8, 552–562 CAS.
- Qikprop, User Manual, Schrödinger, LLC, version 4, 2014, ch. 1, pp. 2–5 Search PubMed.
- W. L. Jorgensen and E. M. Duffy, Prediction of drug solubility from Monte Carlo simulations, Bioorg. Med. Chem. Lett., 2000, 10, 1155–1158 CrossRef CAS PubMed.
- Glide, Schrödinger, LLC, New York, version 5.9, 2013 Search PubMed.
- L. K. Williams, C. Li, S. G. Withers and G. D. Brayer, J. Med. Chem., 2012, 55, 10177–10186 CrossRef CAS PubMed.
Footnote |
† Electronic supplementary information (ESI) available: Experimental procedures for intermediates (chemistry) and spectroscopic/analytical data. CCDC 1904042. For ESI and crystallographic data in CIF or other electronic format see DOI: 10.1039/c9ra05059k |
|
This journal is © The Royal Society of Chemistry 2019 |
Click here to see how this site uses Cookies. View our privacy policy here.