DOI:
10.1039/C9RA05139B
(Paper)
RSC Adv., 2019,
9, 23045-23052
Synthesis and antibacterial and antiviral activities of myricetin derivatives containing a 1,2,4-triazole Schiff base†
Received
6th July 2019
, Accepted 22nd July 2019
First published on 25th July 2019
Abstract
A series of novel myricetin derivatives containing a 1,2,4-triazole Schiff base were designed and synthesized. Their structures were systematically characterized using 1H NMR, 13C NMR, and HRMS. During antibacterial bioassays, 6f, 6i, and 6q demonstrated a good inhibitory effect against Xanthomonas axonopodis pv. citri (Xac), with half-maximal effective concentration (EC50) values of 10.0, 9.4, and 8.8 μg mL−1, respectively, which were better than those of bismerthiazol (54.9 μg mL−1) and thiodiazole copper (61.1 μg mL−1). Note that 6w demonstrated a good inhibitory effect against Ralstonia solanacearum (Rs) with and EC50 value of 15.5 μg mL−1, which was better than those of bismerthiazol (55.2 μg mL−1) and thiodiazole copper (127.9 μg mL−1). Similarly, 6a, 6d, and 6e demonstrated a good inhibitory effect against Xanthomonas oryzae pv. oryzae (Xoo) with EC50 values of 47.1, 61.2, and 61.0 μg mL−1, respectively, which were better than those of bismerthiazol (148.2 μg mL−1) and thiodiazole copper (175.5 μg mL−1). Furthermore, we used scanning electron microscopy (SEM) to study the possible sterilization process of the target compound 6q against Xac. The results indicated the possibility of destroying the bacterial cell membrane structure, resulting in an incomplete bacterial structure, and thus achieving inhibition. Furthermore, antiviral bioassays revealed that most compounds exhibited excellent antiviral activity against tobacco mosaic virus (TMV) at a concentration of 500 μg mL−1. The results of the molecular docking studies for 6g with TMV-CP (PDB code: 1EI7) showed that compound 6g had partially interacted with TMV-CP. Therefore, mechanistic studies of the action of compound 6g could be further studied based on that.
1. Introduction
Plant diseases are one of the natural disasters that can seriously threaten agricultural production. According to statistics released by the Food and Agriculture Organization of the United Nations (FAO), the annual loss in yield because of plant diseases is about 10% of the total production.1 Note that, based on their pathogens, plant diseases can be classified into two categories: invasive diseases and non-invasive diseases. Infectious pathogens primarily include bacteria, viruses, actinomycetes, parasitic plants, fungi, nematodes, viroids, and mycoplasma. For example, Xanthomonas axonopodis pv. citri (Xac) is a citrus canker disease that has significantly impacted production of citrus worldwide. Moreover, Xanthomonas oryzae pv. oryzae (Xoo) is a rod-shaped Gram-negative bacterium causing an average annual loss of 10–50% in many rice-growing countries.2 Therefore, the development of new and highly effective pesticide fungicides and antiviral agents is very important. Flavonoids, or bioflavonoids, which were previously referred to as the basic nucleus structure of 2-phenyl-chromones, and currently referred to as two phenols are a series of compounds in which benzene rings (A and B) are linked to each other via a central three-carbon chain (Fig. 1a and b).3,4
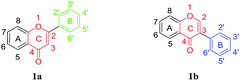 |
| Fig. 1 Core structures of flavones and isoflavones. | |
After the initial discovery of flavonoid glycosides in the 1930s, they have been extensively studied by researchers. In fact, in Chinese herbal medicines, flavonoids are very important active substances. Flavonoids have antibacterial,5,6 antiviral,7,8 antitumor,9 and antioxidant10 activities. Myricetin (3′,4′,5′,3,5,7-hexahydroxyflavonol, Myr), similar to bayanone, is an important polyphenol flavonoid that is widely found in many plants. Modern pharmacological studies have confirmed that myricetin has antibacterial,11,12 antiviral,13,14 antitumor,15 antioxidation,16 and anti-inflammatory17 activities. Furthermore, Ruan et al. reported that a series of myricetin derivatives could be used to inhibit plant pathogens.18 In our previous study, we demonstrated the synthesis of a series of 3-((5-((4-chlorobenzyl)thio)-1,3,4-thiadiazol-2-yl)methoxy)-5,7-dimethoxy-2-(3,4,5-trimethoxyphenyl)-4H-chromen-4-one and N-benzyl-2-((5,7-dimethoxy-4-oxo-2-(3,4,5-trimethoxyphenyl)-4H-chromen-3-yl)oxy) acetamide (Fig. 2a and b), both of which exhibited good antibacterial activities.19,20
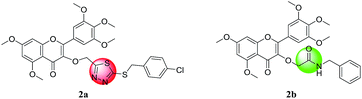 |
| Fig. 2 Compounds previously reported against pathogen. | |
Although there have been a number of studies on myricetin, they are primarily focused on myricetin and its medical applications. Moreover, there have been very few studies on the derivatives of myricetin and their application in pesticides.
In recent decades, heterocyclic compounds have played a critical role for the development of pesticides. In particular, 1,2,4-triazole-based heterocyclic derivatives have become very popular because of their high-efficiency and broad-spectrum biological activity. Similarly, Schiff base compounds have attracted considerable attention because of their unique biological activities, e.g., 1,2,4-triazole Schiff base compounds have both a triazole ring and an imine active fragment of the Schiff base. Therefore, these compounds have a broad spectrum of biological activity such as antibacterial,21,22 antitumor,23 antiviral,24,25 insecticidal,26 and weeding.27 Jin et al. reported that 1,2,4-triazole Schiff bases could be used to inhibit plant pathogens.28
To develop new, highly efficient, environmentally friendly pesticide compounds, myricetin was used as a lead compound to introduce triazole Schiff base structure, a series of myricetin derivatives containing 1,2,4-triazole Schiff base were synthesized and characterized using 1H NMR, 13C NMR, and HRMS. These compounds were then evaluated for their antibacterial and antiviral activities.
2. Experimental
2.1. Methods and materials
Scheme 1 shows the synthetic route for developing myricetin derivatives containing 1,2,4-triazole Schiff bases. The intermediates 1, 2, 3, 4, and 5 were prepared according to previously reported methods.29–31
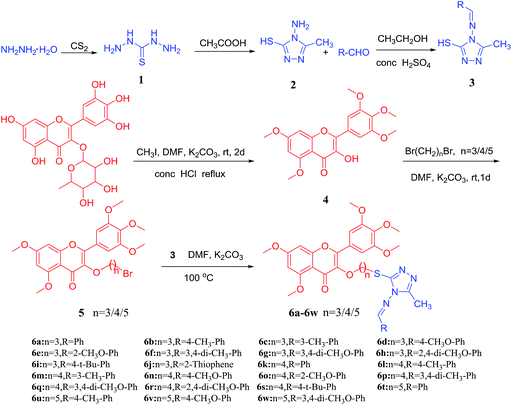 |
| Scheme 1 The synthetic route of the target compounds. | |
2.2. General procedure for preparing the intermediates 1, 2, 3, 4, and 5
Hydrazine hydrate was dissolved in water at 50 °C for 0.5 h, followed by the addition of carbon disulfide at 90 °C for 1 h. The solution was then cooled, followed by crystallization to obtain the intermediate 1. Intermediate 1 and glacial acetic acid were then refluxed for 4 h, and then cooled to room temperature. Then, the unreacted acetic acid was distilled off and the precipitate washed with water to obtain intermediate 2. Subsequently, intermediate 2 was heated till it completely dissolved in ethanol, and then substituted aldehyde was added for 6 h to obtain intermediate 3. Myricetin and methyl iodide were stirred at room temperature for 48 h, and then concentrated hydrochloric acid deglucoside was added to obtain intermediate 4. Finally, the intermediate 4 was stirred in DMF at room temperature for 0.5–1 h and dibromoalkane was added; this reaction was allowed to continue for 12 h to obtain the intermediate 5.
2.3. General procedure for preparing the target compounds 6a–6w
The target compounds 6a–6w were synthesized according to previously reported methods,32 although certain modifications were made to the preparation process. Intermediate 3 (1.2 mmol), K2CO3 (3 mmol), and DMF (20 mL) were added to a 50 mL 3-neck round bottom flask equipped with a magnetic stirrer. The mixture was stirred at room temperature for 0.5 h, and then intermediate 5 (1.0 mmol) was added to the reaction solution with stirring at 100–105 °C for 6 h. The progress of the reaction was monitored using TLC. Finally, after cooling the reaction mixture to room temperature, 100 mL of water was added, followed by extraction with dichloromethane (3 × 30 mL). The combined organic phases were washed twice with 5% NaOH, distilled water, and saturated aqueous NaCl. Finally, the solvent was dried, evaporated, and purified (petroleum ether
:
ethyl acetate = 1
:
3, V/V), and the product was obtained with 40–75% yield.
3. Results and discussion
3.1. Spectral properties
1H NMR, 13C NMR and HRMS spectra of all the target compounds are provided in the ESI.† For example, the analysis of the spectral data of 6a is given below. In the 1H NMR spectrum, 9H in the δ 8.83–7.60 ppm and δ 2.44 ppm can be attributed to the hydrogen on the molecular fragment of the 1,2,4-triazole Schiff base, whereas 4H appearing in the δ 7.34–6.48 ppm can be attributed to myricetin. Hydrogens on the benzene ring, 6H of δ 3.99 ppm, δ 3.22 ppm, and δ 2.08–2.02 ppm, can be attributed to the methylene hydrogen on the carbon chain, whereas 15H of δ 3.90–3.74 ppm can be attributed to the 5 methoxy group of myricetin's benzene ring. In the HRMS spectrum of the title compound, the characteristic absorption signals of [M + H]+ ions were obtained, which were consistent with their molecular weights.
3.2. Antibacterial activity in vitro
For determining bioactivity, the target compounds were tested against Xac, Rs, and Xoo using the turbidity method with bismerthiazol, thiadiazole copper, and myricetin being used as control agents. Table 1 lists the antibacterial activities of 6j, 6k, 6l, 6n, 6q, and 6t against Xac at 100 μg mL−1 with the percentage inhibition of 79.6%, 79.8%, 81.2%, 81.8%, 84.5%, and 85.8%, respectively, which were better than those of myricetin (49.4%), bismerthiazol (59.5%), and thiadiazole copper (56.9%). Note that the percentage inhibition of 6l, 6q, and 6t against Xac at 50 μg mL−1 were 74.9%, 75.3%, and 74.9%, respectively, which were better than those of myricetin (40.4%), bismerthiazol (49.5%), and thiadiazole copper (47.9%). Furthermore, 6w showed antibacterial activity against Rs at 100 μg mL−1 with the percentage inhibition of 83.2%, which was better than those of myricetin (56.4%), bismerthiazol (61.1%), and thiadiazole copper (37.1%). Similarly, the percentage inhibition of 6w against Rs at 50 μg mL−1 was 70.3%, which was better than those of myricetin (43.3%), bismerthiazol (49.5%), and thiadiazole copper (17.4%). Moreover, 6a, 6e, 6h, and 6w showed antibacterial activities against Xoo at 100 μg mL−1 with the percentage inhibition of 59.1%, 57.1%, 57.3%, and 56.5%, respectively, which were better than those of myricetin (41.4%), bismerthiazol (47.0%), and thiadiazole copper (43.2%). Similarly, the percentage inhibition of compounds 6a, 6h, and 6w against Xoo at 50 μg mL−1 were 51.6%, 49.5%, and 47.2%, respectively, which were better than those of myricetin (32.3%), bismerthiazol (39.6%), and thiadiazole copper (38.2%).
Table 1 In vitro antibacterial activity of the target compounds against Xac, Rs and Xoo
Compd |
Percentage inhibitiona/% |
Xac |
Rs |
Xoo |
100 μg mL−1 |
50 μg mL−1 |
100 μg mL−1 |
50 μg mL−1 |
100 μg mL−1 |
50 μg mL−1 |
Average of three replicates (temperature: 28 ± 1 °C, time: 24–48 h). Myr (myricetin). BT (commercial fungicides, bismerthiazol) and TC (thiediazole copper). |
6a |
78.2 ± 0.2 |
69.7 ± 1.0 |
16.9 ± 3.7 |
6.6 ± 0.2 |
59.1 ± 8.7 |
51.6 ± 7.8 |
6b |
69.5 ± 4.8 |
65.1 ± 9.2 |
38.1 ± 7.5 |
21.4 ± 7.0 |
53.1 ± 6.9 |
45.0 ± 5.6 |
6c |
73.6 ± 7.9 |
66.0 ± 6.2 |
25.9 ± 7.2 |
18.7 ± 2.7 |
45.3 ± 1.5 |
33.9 ± 2.8 |
6d |
74.5 ± 2.9 |
66.9 ± 3.8 |
35.1 ± 5.1 |
24.6 ± 7.7 |
53.6 ± 3.5 |
46.5 ± 1.2 |
6e |
56.0 ± 0.7 |
50.2 ± 3.9 |
33.2 ± 6.8 |
11.5 ± 1.6 |
57.1 ± 4.8 |
46.1 ± 6.4 |
6f |
79.2 ± 0.2 |
72.4 ± 1.6 |
41.1 ± 1.5 |
33.9 ± 0.6 |
43.3 ± 8.2 |
35.6 ± 8.0 |
6g |
76.6 ± 8.3 |
62.9 ± 6.6 |
18.5 ± 7.4 |
13.8 ± 1.4 |
53.6 ± 4.4 |
47.0 ± 9.6 |
6h |
67.3 ± 6.1 |
56.4 ± 6.0 |
27.2 ± 4.6 |
25.3 ± 4.1 |
57.3 ± 3.4 |
49.5 ± 3.6 |
6i |
78.3 ± 1.7 |
72.1 ± 5.3 |
29.6 ± 1.2 |
19.7 ± 2.8 |
54.3 ± 2.9 |
41.3 ± 5.1 |
6j |
79.6 ± 2.0 |
67.7 ± 4.5 |
35.1 ± 1.9 |
31.3 ± 8.9 |
58.6 ± 5.2 |
44.7 ± 3.7 |
6k |
79.8 ± 3.5 |
63.2 ± 2.7 |
25.4 ± 5.1 |
13.1 ± 7.3 |
53.5 ± 9.9 |
45.1 ± 6.0 |
6l |
81.2 ± 6.1 |
74.9 ± 6.7 |
36.0 ± 3.6 |
32.7 ± 4.0 |
53.3 ± 3.0 |
46.3 ± 9.7 |
6m |
73.5 ± 4.4 |
61.1 ± 3.3 |
22.8 ± 0.6 |
11.9 ± 4.6 |
47.1 ± 4.9 |
40.4 ± 6.7 |
6n |
81.8 ± 5.4 |
68.6 ± 4.5 |
39.7 ± 2.6 |
35.1 ± 9.4 |
53.7 ± 3.2 |
44.2 ± 5.3 |
6o |
71.3 ± 4.2 |
62.9 ± 2.5 |
43.9 ± 2.9 |
32.0 ± 9.3 |
49.3 ± 0.8 |
37.7 ± 5.8 |
6p |
78.7 ± 2.0 |
63.1 ± 8.0 |
49.7 ± 6.1 |
45.7 ± 7.7 |
51.9 ± 4.0 |
45.4 ± 7.6 |
6q |
84.5 ± 2.2 |
75.3 ± 4.8 |
52.1 ± 5.6 |
36.3 ± 7.0 |
50.7 ± 0.4 |
42.4 ± 9.4 |
6r |
65.3 ± 1.4 |
54.3 ± 2.5 |
43.7 ± 2.4 |
20.8 ± 3.5 |
48.0 ± 0.9 |
33.8 ± 5.2 |
6s |
73.1 ± 6.2 |
65.6 ± 3.8 |
46.0 ± 0.4 |
26.0 ± 5.8 |
53.8 ± 3.9 |
38.2 ± 7.3 |
6t |
85.8 ± 3.2 |
74.9 ± 6.8 |
51.2 ± 3.3 |
33.0 ± 0.9 |
53.4 ± 2.6 |
45.7 ± 8.1 |
6u |
70.8 ± 4.9 |
62.7 ± 5.0 |
17.8 ± 2.7 |
11.0 ± 3.7 |
48.0 ± 9.6 |
39.7 ± 3.6 |
6v |
69.4 ± 0.2 |
61.0 ± 5.4 |
17.4 ± 4.7 |
6.1 ± 1.0 |
56.3 ± 1.8 |
45.7 ± 8.5 |
6w |
72.0 ± 7.1 |
64.1 ± 2.8 |
83.2 ± 2.0 |
70.3 ± 3.2 |
56.5 ± 2.1 |
47.2 ± 3.2 |
Myrb |
49.4 ± 4.1 |
40.4 ± 0.4 |
56.4 ± 2.0 |
43.3 ± 4.6 |
41.4 ± 5.9 |
32.3 ± 2.9 |
BTc |
59.5 ± 7.7 |
49.5 ± 5.7 |
61.1 ± 6.2 |
49.5 ± 5.3 |
47.0 ± 3.1 |
39.6 ± 6.1 |
TCc |
56.9 ± 1.5 |
47.9 ± 8.5 |
37.1 ± 3.6 |
17.4 ± 6.6 |
43.2 ± 3.0 |
38.2 ± 6.3 |
Based on these preliminary results of bioassays, EC50 values of some of the compounds showed good antibacterial activities against Xac, Rs and Xoo as indicated in Tables 2 and 3. As can be seen from Tables 2 and 3, compounds 6f, 6i, and 6q showed good inhibitory effects against Xac with the EC50 values of 10.0, 9.4, and 8.8 μg mL−1, which were better than those of myricetin (107.2 μg mL−1), bismerthiazol (54.9 μg mL−1), and thiodiazole copper (61.1 μg mL−1). Similarly, 6w demonstrated a good inhibitory effect against Rs with an EC50 value of 15.5 μg mL−1, which was better than those of myricetin (82.6 μg mL−1), bismerthiazol (55.2 μg mL−1), and thiodiazole copper (127.9 μg mL−1). Moreover, 6a, 6d, and 6e demonstrated good inhibitory effects against Xoo with EC50 values of 47.1, 61.2, and 61.0 μg mL−1, which were better than those of myricetin (222.4 μg mL−1), bismerthiazol (148.2 μg mL−1), and thiodiazole copper (175.5 μg mL−1).
Table 2 In vitro antibacterial EC50 activity value of the target compounds against Xac
Compd |
n |
R |
Toxic regression equationa |
r2 |
EC50/(μg mL−1) |
pEC50/(μM) |
Tested and calculated at the drug test concentrations of 100, 50, 25, 12.5, and 6.25 μg mL−1. Myr (myricetin). BT (commercial fungicides, bismerthiazol) and TC (thiediazole copper). |
6a |
3 |
Ph |
y = 0.8044x + 4.1554 |
0.9961 |
11.2 ± 3.4 |
4.8 |
6b |
3 |
4-CH3-Ph |
y = 0.6949x + 4.1397 |
0.9697 |
17.3 ± 2.2 |
4.6 |
6c |
3 |
3-CH3-Ph |
y = 0.8528x + 3.9474 |
0.9972 |
17.2 ± 1.4 |
4.6 |
6d |
3 |
4-CH3O-Ph |
y = 1.0517x + 3.5664 |
0.9650 |
23.1 ± 2.8 |
4.5 |
6e |
3 |
2-CH3O-Ph |
y = 0.6924x + 3.7991 |
0.9822 |
54.3 ± 2.4 |
4.1 |
6f |
3 |
3,4-Di-CH3-Ph |
y = 0.8130x + 4.1875 |
0.9977 |
10.0 ± 3.9 |
4.8 |
6g |
3 |
3,4-Di-CH3O-Ph |
y = 0.9169x + 3.8298 |
0.9762 |
18.9 ± 2.6 |
4.6 |
6h |
3 |
2,4-Di-CH3O-Ph |
y = 0.6912x + 3.8298 |
0.9690 |
28.1 ± 1.5 |
4.4 |
6i |
3 |
4-t-Bu-Ph |
y = 0.7819x + 4.2398 |
0.9917 |
9.4 ± 1.9 |
4.9 |
6j |
3 |
2-Thiophene |
y = 0.9335x + 3.8960 |
0.9642 |
15.2 ± 2.9 |
4.6 |
6k |
4 |
Ph |
y = 0.9655x + 3.7731 |
0.9334 |
18.7 ± 0.8 |
4.6 |
6l |
4 |
4-CH3-Ph |
y = 0.9335x + 4.0630 |
0.9877 |
10.1 ± 1.1 |
4.8 |
6m |
4 |
3-CH3-Ph |
y = 0.7733x + 4.0122 |
0.9599 |
18.9 ± 0.9 |
4.6 |
6n |
4 |
4-CH3O-Ph |
y = 0.9324x + 3.9636 |
0.9716 |
12.9 ± 1.9 |
4.7 |
6o |
4 |
2-CH3O-Ph |
y = 0.6712x + 4.1730 |
0.9624 |
17.1 ± 2.5 |
4.6 |
6p |
4 |
3,4-Di-CH3-Ph |
y = 0.9633x + 3.8184 |
0.9729 |
16.9 ± 2.6 |
4.6 |
6q |
4 |
3,4-Di-CH3O-Ph |
y = 0.9311x + 4.1191 |
0.9819 |
8.8 ± 1.9 |
4.9 |
6r |
4 |
2,4-Di-CH3O-Ph |
y = 0.5861x + 4.1558 |
0.9706 |
27.6 ± 3.1 |
4.4 |
6s |
4 |
4-t-Bu-Ph |
y = 0.7906x + 4.0584 |
0.9903 |
15.5 ± 2.0 |
4.7 |
6t |
5 |
Ph |
y = 1.1883x + 3.6956 |
0.9958 |
12.5 ± 2.9 |
4.7 |
6u |
5 |
4-CH3-Ph |
y = 0.7831x + 3.9652 |
0.9780 |
21.0 ± 1.0 |
4.5 |
6v |
5 |
4-CH3O-Ph |
y = 0.7105x + 4.0749 |
0.9998 |
20.5 ± 2.7 |
4.5 |
6w |
5 |
3,4-Di-CH3O-Ph |
y = 0.7706x + 4.0642 |
0.9814 |
16.4 ± 1.9 |
4.7 |
Myrb |
— |
— |
y = 0.6520x + 3.6763 |
0.9838 |
107.2 ± 1.6 |
— |
BTc |
— |
— |
y = 0.8357x + 3.5466 |
0.9876 |
54.9 ± 1.4 |
— |
TCc |
— |
— |
y = 0.8874x + 3.4149 |
0.9984 |
61.1 ± 3.4 |
— |
Table 3 In vitro antibacterial EC50 activity value of the target compounds against Rs and Xoo
Bacteria |
Compd |
n |
R |
Toxic regression equationa |
r2 |
EC50/(μg mL−1) |
pEC50/(μM) |
Tested and calculated at the drug test concentrations of 100, 50, 25, 12.5, and 6.25 μg mL−1. Myr (myricetin). BT (commercial fungicides, bismerthiazol) and TC (thiediazole copper). |
Rs |
6w |
5 |
3,4-Di-CH3O-Ph |
y = 1.0988x + 3.6922 |
0.9829 |
15.5 ± 1.3 |
4.7 |
Myrb |
— |
— |
y = 0.8815x + 3.3101 |
0.9399 |
82.6 ± 1.0 |
— |
BTc |
— |
— |
y = 0.9991x + 3.2599 |
0.9930 |
55.2 ± 3.5 |
— |
TCc |
— |
— |
y = 1.3627x + 2.1291 |
0.9184 |
127.9 ± 2.5 |
— |
Xoo |
6a |
3 |
Ph |
y = 0.8103x + 3.6447 |
0.9958 |
47.1 ± 2.6 |
4.1 |
6b |
3 |
4-CH3-Ph |
y = 0.7806x + 3.5304 |
0.9988 |
76.3 ± 1.0 |
3.9 |
6d |
3 |
4-CH3O-Ph |
y = 0.7023x + 3.7451 |
0.9841 |
61.2 ± 2.1 |
|
6e |
3 |
2-CH3O-Ph |
y = 0.8457x + 3.4899 |
0.9871 |
61.0 ± 2.9 |
4.0 |
6g |
3 |
3,4-Di-CH3O-Ph |
y = 0.8448x + 3.4411 |
0.9947 |
70.0 ± 3.1 |
4.0 |
6k |
4 |
Ph |
y = 0.6866x + 3.7132 |
0.9990 |
74.8 ± 2.6 |
3.9 |
6l |
4 |
4-CH3-Ph |
y = 0.8236x + 3.4626 |
0.9798 |
73.6 ± 3.9 |
4.0 |
6m |
4 |
3-CH3-Ph |
y = 0.9248x + 3.1185 |
0.9728 |
108.3 ± 2.0 |
3.8 |
6n |
4 |
4-CH3O-Ph |
y = 0.9574x + 3.2088 |
0.9960 |
74.3 ± 2.5 |
4.0 |
6p |
4 |
3,4-Di-CH3-Ph |
y = 0.1077x + 2.8916 |
0.9494 |
90.8 ± 1.8 |
3.9 |
6t |
5 |
Ph |
y = 0.7280x + 3.6251 |
0.9920 |
77.4 ± 3.3 |
3.9 |
6u |
5 |
4-CH3-Ph |
y = 0.7541x + 3.4240 |
0.9929 |
123.0 ± 3.0 |
3.7 |
Myrb |
— |
— |
y = 0.6512x + 3.4715 |
0.9862 |
222.4 ± 2.4 |
— |
BTc |
— |
— |
y = 0.5525x + 3.8806 |
0.9947 |
148.2 ± 2.4 |
— |
TCc |
— |
— |
y = 0.5876x + 3.6813 |
0.9950 |
175.5 ± 2.1 |
— |
3.3. Structure–activity relationships (SAR) of antibacterial activities
According to the results in Tables 2 and 3, combined with the activity data analysis of the parent structure of myricetin, the myricetin derivative can be obtained after introducing the 1,2,4-triazole Schiff base, which can inhibit biological activity. For example, 6f (n = 3, R = 3,4-di-CH3-Ph), 6i (n = 3, R = 4-t-Bu-Ph), and 6q (n = 4, 3,4-di-CH3O-Ph) demonstrated a good inhibitory effect against Xac with the EC50 values of 10.0, 9.4, and 8.8 μg mL−1, which were better than those of myricetin (107.2 μg mL−1), bismerthiazol (54.9 μg mL−1), and thiodiazole copper (61.1 μg mL−1). Similarly, 6w (n = 5, R = 3,4-di-CH3O-Ph) demonstrated a good inhibitory effect against Rs with EC50 value of 15.5 μg mL−1, which was better than those of myricetin (82.6 μg mL−1), bismerthiazol (55.2 μg mL−1), and thiodiazole copper (127.9 μg mL−1). Moreover, 6a (n = 3, R = Ph), 6d (n = 3, R = 4-CH3O-Ph), and 6e (n = 3, R = 2-CH3O-Ph) demonstrated good inhibitory effect against Xoo with EC50 values of 47.1, 61.2, and 61.0 μg mL−1, which were better than those of myricetin (222.4 μg mL−1), bismerthiazol (148.2 μg mL−1), and thiodiazole copper (175.5 μg mL−1). According to the results in Tables 2 and 3, together with the activity data analytical of the parent structure of myricetin, the myricetin derivatives can be obtained after introducing the 1,2,4-triazole Schiff base, which can inhibit biological activity. For example, when n = 3, R is Ph, 4-CH3O-Ph, 2-CH3O-Ph, 3,4-di-CH3O-Ph, and 2,4-di-CH3O-Ph, the activity of substituted benzene is higher than that of substituted phenyl-containing methoxy compounds. When n = 4, R is Ph, 4-CH3O-Ph, 2-CH3O-Ph, and 3,4-di-CH3O-Ph, the activity of substituted phenyl is higher than that of substituted benzene compounds.
To summarize, the derivative obtained by introducing the 1,2,4-triazole Schiff base into the myricetin structure as a lead compound has a good inhibitory activity against plant pathogens and can be used as a potential plant-inhibiting drug.
3.4. SEM study
According to the results in Fig. 3A–C, the surface morphology of Xac was observed by scanning electron microscopy (SEM).33,34 Bacterial cells in group (A) were rhabditiform, homogeneous in size, and unbroken in morphology. After treatment with 6q (12.5 μg mL−1), cell wall invagination appeared at both ends of the bacterial cells. Moreover, after treatment with 6q (25 μg mL−1), the bacterial cell wall showed different degrees of rupture and the cell contents leaked.
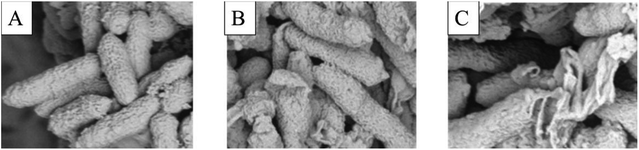 |
| Fig. 3 SEM images for Xac after incubated in different concentration of compound 6q. (A) 0 μg mL−1, (B) 12.5 μg mL−1, and (C) 25 μg mL−1. Scale bar for (A, B, and C) are 2 mm. | |
3.5. Antiviral activity of the title compounds against TMV
As can be seen in Table 4, at a test concentration of 500 μg mL−1, the curative, protective, and inactivation activities of the target compounds against tobacco mosaic virus (TMV) were tested. The results showed that both 6g and 6j showed a good therapeutic effect on TMV, whereas the percentage inhibition were 52.5% and 52.4%, respectively, which exceeded those of myricetin (31.6%) and ribavirin (39.9%) but close to that of ningnanmycin (52.7%). Similarly, 6f, 6p, and 6w show a good protective effect on TMV, and their percentage inhibition were 58.7%, 57.8%, and 58.0%, respectively, which exceeded those of myricetin (42.1%) and ribavirin (51.8%) but close to that of ningnanmycin (65.7%). Furthermore, 6g showed a good passivation effect on TMV, and its percentage inhibition was 88.6%, which exceeded those of myricetin (50.9%) and ribavirin (73.3%) but close to that of ningnanmycin (90.4%).
Table 4 Antiviral activities of the target compounds against TMV in vivo at 500 μg mL−1
Compd |
n |
R |
Percentage inhibitiona/% |
Curative |
Protection |
Inactivation |
Average of three replicates. Lead compound myricetin. Commercial antiviral agents ribavirin and ningnanmycin. |
6a |
3 |
Ph |
51.5 ± 3.1 |
52.6 ± 5.2 |
67.5 ± 8.1 |
6b |
3 |
4-CH3-Ph |
41.6 ± 2.9 |
48.9 ± 2.8 |
70.4 ± 4.4 |
6c |
3 |
3-CH3-Ph |
40.8 ± 5.3 |
42.9 ± 3.7 |
47.6 ± 8.5 |
6d |
3 |
4-CH3O-Ph |
47.5 ± 4.8 |
50.0 ± 1.2 |
57.3 ± 9.2 |
6e |
3 |
2-CH3O-Ph |
45.6 ± 7.0 |
27.8 ± 4.9 |
44.5 ± 6.6 |
6f |
3 |
3,4-Di-CH3-Ph |
50.4 ± 7.6 |
58.7 ± 5.9 |
41.4 ± 2.8 |
6g |
3 |
3,4-Di-CH3O-Ph |
52.5 ± 1.9 |
55.4 ± 6.5 |
88.6 ± 4.5 |
6h |
3 |
2,4-Di-CH3O-Ph |
46.1 ± 5.4 |
50.7 ± 6.2 |
73.2 ± 5.7 |
6i |
3 |
4-t-Bu-Ph |
44.3 ± 3.7 |
49.3 ± 7.4 |
77.5 ± 8.9 |
6j |
3 |
2-Thiophene |
52.4 ± 6.8 |
54.6 ± 7.5 |
67.5 ± 8.5 |
6k |
4 |
Ph |
50.8 ± 3.3 |
57.2 ± 7.2 |
58.9 ± 8.3 |
6l |
4 |
4-CH3-Ph |
42.0 ± 4.5 |
45.8 ± 2.4 |
60.9 ± 5.3 |
6m |
4 |
3-CH3-Ph |
39.5 ± 7.0 |
46.1 ± 5.6 |
49.5 ± 2.9 |
6n |
4 |
4-CH3O-Ph |
50.2 ± 4.7 |
48.0 ± 3.6 |
71.4 ± 1.0 |
6o |
4 |
2-CH3O-Ph |
39.0 ± 7.3 |
53.6 ± 6.5 |
67.5 ± 7.1 |
6p |
4 |
3,4-Di-CH3-Ph |
49.9 ± 3.4 |
57.8 ± 8.5 |
52.3 ± 2.4 |
6q |
4 |
3,4-Di-CH3O-Ph |
50.4 ± 7.3 |
52.6 ± 5.8 |
68.9 ± 5.7 |
6r |
4 |
2,4-Di-CH3O-Ph |
45.1 ± 0.2 |
36.8 ± 4.3 |
55.4 ± 3.5 |
6s |
4 |
4-t-Bu-Ph |
44.8 ± 2.6 |
45.5 ± 3.0 |
37.3 ± 7.1 |
6t |
5 |
Ph |
51.3 ± 5.5 |
56.0 ± 7.1 |
56.4 ± 2.8 |
6u |
5 |
4-CH3-Ph |
49.6 ± 6.9 |
50.8 ± 4.5 |
51.7 ± 4.6 |
6v |
5 |
4-CH3O-Ph |
46.2 ± 0.8 |
45.3 ± 6.4 |
74.6 ± 8.3 |
6w |
5 |
3,4-Di-CH3O-Ph |
51.1 ± 7.4 |
58.0 ± 3.1 |
42.8 ± 2.0 |
Myrb |
— |
— |
31.6 ± 7.2 |
42.1 ± 6.4 |
50.9 ± 6.3 |
Ribavirinc |
— |
— |
39.9 ± 3.7 |
51.8 ± 6.0 |
73.3 ± 2.9 |
Ningnanmycinc |
— |
— |
52.7 ± 1.3 |
65.7 ± 1.9 |
90.4 ± 3.1 |
3.6. Structure–activity relationship (SAR) of the title compounds against TMV
In summary, the introduction of the 1,2,4-triazole Schiff base into the myricetin structure showed a good inhibitory activity against the plant virus. According to the anti-TMV activity data of 1,2,4-triazole Schiff base myricetin derivatives, when R = Ph, 3,4-di-CH3-Ph, 3,4-di-CH3O-Ph, and 2-thiophene, the compounds have good curative and protective activities. For example, 6a, 6g, 6j, 6t, and 6w have a good therapeutic effect on TMV with percentage inhibition of 51.5%, 52.5%, 52.4%, 51.3%, and 51.1%, respectively, which are higher than those of myricetin (31.6%) and ribavirin (39.9%) but close to that of ningnanmycin (52.7%). Similarly, 6f, 6g, 6k, 6p, 6t, and 6w have a good protective effect on TMV with percentage inhibition of 58.7%, 55.4%, 57.2%, 57.8%, 56.0%, and 58.0%, respectively, which are higher than those of myricetin (42.1%) and ribavirin (51.8%) but close to that of ningnanmycin (65.7%). When R = 3,4-di-CH3O-Ph, the compound showed better passivation activity, e.g., 6g had a good passivation effect on TMV with an percentage inhibition of 88.6%, which exceeded those of myricetin (50.9%) and ribavirin (73.3%) but was close to that of ningnanmycin (90.4%). In summary, when n = 3, and n = 4, the R group is Ph, 3,4-di-CH3-Ph, 3,4-di-CH3O-Ph, and 2-thiophene, the therapeutic and protective activity of the compounds against tobacco flower leaf virus is superior to the R group being the other substituent. When n = 3, and n = 4, and the R group is 3,4-di-CH3O-Ph, the compounds has a relatively good passivating activity against tobacco mosaic virus.
3.7. Molecular docking of 6g and TMV-CP
Molecular docking studies for 6g with TMV-CP (PDB code: 1EI7)35,36 indicated that the compound was well embedded in the pocket (including residues of TYR139, PRO254, LYS268, ARG134, ASN140, VAL75, etc.) between the two subunits of TMV-CP (Fig. 4). It has been reported that these residues played important roles in the self-assembly of TMV particles.33,35,36 For example, LYS268 and TYR139 play continuous hydrophobic interactions in the ring.35 In addition, VAL75, VAL260, LYS253, GLY135, ASN140, ASP266 and other subunits of TMV-CP.33,35 Moreover, there are also π-alkyl, alkyl, amide-π stacking effects, carbon hydrogen bond between the molecules and the above residues in TMV-CP as depicted in Fig. 4. For example, TYR139, PRO254, LYS268, and ARG134 had strong hydrogen bonds with 6g (2.40 Å, 2.47 Å, 2.11 Å, and 2.41 Å). These interactions between small molecules and TMV-CP are likely to inhibit the interaction of two subunit of TMV-CP, thereby preventing the self-assembly of TMV particle. The results showed that compound 6g had partially interacted with TMV-CP, and mechanistic studies of the action of compound 6g could be further studied.
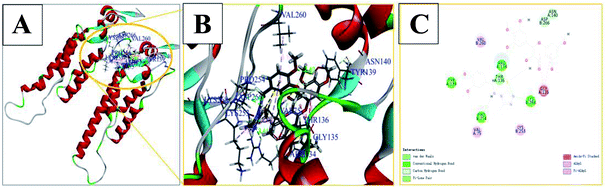 |
| Fig. 4 The binding mode of compound 6g docked with TMV-CP. | |
4. Conclusions
In this study, using active splicing, a reasonable structural modification of myricetin was undertaken. To design and synthesize a series of myricetin derivatives, an active small molecular group of 1,2,4-triazole Schiff base was introduced into the myricetin structure, and then their biological activities were tested. 6f, 6i, and 6q showed a good inhibitory effect against Xac with EC50 values of 10.0, 9.4, and 8.8 μg mL−1, which were better than those of bismerthiazol (54.9 μg mL−1) and thiodiazole copper (61.1 μg mL−1). Moreover, using SEM on the target compound, the antibacterial mechanism against Xac was identified. It was found from the experiment that the cell membrane structure of the bacteria was destroyed, and the bacterial structure changed from the intact form of the blank control (A) to the incomplete form after dosing (B and C). Therefore, this may be the bacteriostatic action of the target compound. Furthermore, antiviral bioassays revealed that most compounds exhibited excellent antiviral activity against TMV at a concentration of 500 μg mL−1. The results of the molecular docking studies for 6g with TMV-CP (PDB code: 1EI7) showed that compound 6g had partially interacted with TMV-CP. Therefore, mechanistic studies of the action of compound 6g could be further studied based on that. The above biological activity study results demonstrate that the myricetin derivative with the 1,2,4-triazole Schiff base has better biocontrol activity against plant diseases and plant viruses.
Conflicts of interest
The authors declare that they have no competing interests.
Acknowledgements
The authors gratefully acknowledge the financial support of the National Nature Science Foundation of China (No. 21867003) and Science Foundation of Guizhou Province (No. 20185781, 20191105).
References
- Z. S. Feng and S. J. Gao, Mod. Agric., 2013, 3, 5–6 Search PubMed.
- X. Lv, L. Yang, Z. Fan and X. P. Bao, J. Saudi Chem. Soc., 2018, 22, 101–109 CrossRef CAS.
- A. L. Simons, M. Renouf, S. Hendrich and P. A. Murphy, J. Agric. Food Chem., 2005, 53, 4258–4263 CrossRef CAS PubMed.
- D. Kim, J. Park, J. Kim, C. Han and C. Lee, J. Agric. Food Chem., 2006, 54, 935–941 CrossRef CAS PubMed.
- I. Oh, W. Y. Yang, S. C. Chung, T. Y. Kim, K. B. Oh and J. Shin, Arch. Pharmacal Res., 2011, 34, 217–222 CrossRef CAS PubMed.
- M. G. Huang, X. H. Ruan, J. P. Zhang, Q. Li, Y. H. Wang, L. J. Chen, C. Zhang, P. Li and W. Xue, Chin. J. Org. Chem., 2017, 37, 2145–2152 CrossRef CAS.
- W. H. Huang, G. X. Zhou, G. C. Wang, H. Y. Chung, W. C. Ye and Y. L. Li, J. Asian Nat. Prod. Res., 2012, 14, 401–406 CrossRef CAS PubMed.
- J. S. Dong, B. J. Su, H. Oh, B. H. Lee, S. Y. Lee, S. H. Oh, J. Y. Jung and C. S. Choi, Food Control, 2016, 60, 25–30 CrossRef.
- K. D. Hoang, K. C. Huynh, H. T. Do and T. D. Nguyen, Chem. Pap., 2018, 72, 1399–1406 CrossRef.
- W. Pietrzak, R. Nowak and M. Olech, Chem. Pap., 2014, 68, 976–982 CAS.
- D. Grenier, H. Chen, L. A. Ben, J. Fournierlarente and M. P. Morin, PLoS One, 2015, 10, e0131758 CrossRef PubMed.
- K. Rashed, A. Ćirić and J. Glamočlija, Ind. Crops Prod., 2014, 59, 210–215 CrossRef CAS.
- J. T. Ortega, A. I. Suárez, M. L. Serrano, J. Baptista, F. H. Pujol and H. R. Rangel, AIDS Res. Ther., 2017, 14, 57–62 CrossRef PubMed.
- S. Park, J. I. Kim, I. Lee, S. Lee, M. W. Hwang, J. Y. Bae, J. Heo, D. Kim, S. Z. Han and M. S. Park, Biochem. Biophys. Res. Commun., 2013, 440, 14–19 CrossRef CAS PubMed.
- X. H. Ruan, H. J. Zhao, C. Zhang, L. J. Chen, P. Li, Y. H. Wang, M. He and W. Xue, Chem. J. Chin. Univ., 2018, 39, 1197–1204 CAS.
- V. Chobot and F. Hadacek, Redox Rep., 2011, 16, 242–247 CrossRef CAS PubMed.
- X. Wang, Z. W. Wang, P. S. Sidhu, U. R. Desai and Q. B. Zhou, PLoS One, 2015, 10, e0116409 CrossRef PubMed.
- X. H. Ruan, C. Zhang, S. C. Jiang, T. Guo, R. J. Xia, Y. Chen, X. Tang and W. Xue, Molecules, 2018, 23, 3132–3141 CrossRef PubMed.
- X. M. Zhong, X. B. Wang, L. J. Chen, X. H. Ruan, Q. Li, J. P. Zhang, Z. Chen and W. Xue, Chem. Cent. J., 2017, 11, 106–114 CrossRef PubMed.
- W. Xiao, X. H. Ruan, Q. Li, J. P. Zhang, X. M. Zhong, Y. Xie, X. B. Wang, M. G. Huang and W. Xue, Chem. J. Chin. Univ., 2017, 38, 35–40 CAS.
- F. Zhang, Q. Wen, S. F. Wang, B. Shahla Karim, Y. S. Yang, J. J. Liu, W. M. Zhang and H. L. Zhu, Bioorg. Med. Chem. Lett., 2014, 24, 90–95 CrossRef CAS PubMed.
- K. A. Dilmaghani, F. N. Pur and M. H. Nezhad, Iran. J. Pharm. Res., 2015, 14, 693–699 CAS.
- X. Li, X. Q. Li, H. M. Liu, X. Z. Zhou and Z. H. Shao, Bioorg. Med. Chem. Lett., 2012, 2, 26–30 CrossRef PubMed.
- A. R. Maarouf, A. A. Farahat, K. B. Selim and H. M. Eisa, Med. Chem. Res., 2012, 21, 703–710 CrossRef CAS.
- Q. Z. Xiong, X. F. Lin, J. H. Liu, L. Bi and X. P. Bao, Chin. J. Org. Chem., 2012, 32, 1255–1260 CrossRef CAS.
- S. Maddila, R. Pagadala and S. B. Jonnalagadda, J. Heterocycl. Chem., 2015, 52, 487–491 CrossRef CAS.
- B. L. Wang, Y. X. Shi, Y. Ma, X. H. Liu, Y. H. Li, H. B. Song and Z. M. Li, J. Agric. Food Chem., 2010, 58, 5515–5522 CrossRef CAS PubMed.
- R. Y. Jin, C. Y. Zeng, X. H. Liang, X. H. Sun, Y. F. Liu, Y. Y. Wang and S. Zhou, Bioorg. Chem., 2018, 80, 253–260 CrossRef CAS PubMed.
- C. Y. Liu, Q. Q. Zhao and J. Li, Chem. Reagents, 2001, 23, 344–345 CAS.
- D. W. Pan, H. Du, X. Y. Lü and X. P. Bao, Chin. J. Org. Chem., 2016, 36, 818–825 CrossRef CAS.
- W. Xue, B. A. Song, H. J. Zhao, X. B. Qi, Y. J. Huang and X. H. Liu, Eur. J. Med. Chem., 2015, 97, 155–163 CrossRef CAS PubMed.
- P. Li, Y. Chen, R. J. Xia, T. Guo, M. Zhang, S. C. Jiang, X. Tang, M. He and W. Xue, Chem. J. Chin. Univ., 2019, 40, 909–917 Search PubMed.
- X. Tang, S. J. Su, M. Chen, J. He, R. J. Xia, T. Guo, Y. Chen, C. Zhang, J. Wang and W. Xue, RSC Adv., 2019, 9, 6011–6020 RSC.
- P. Y. Wang, M. W. Wang, D. Zeng, M. Xiang, J. R. Rao, Q. Q. Liu, L. W. Liu, Z. B. Wu, Z. Li, B. A. Song and S. Yang, J. Agric. Food Chem., 2019, 67, 3535–3545 CrossRef CAS PubMed.
- Y. Y. Wang, F. Z. Xu, Y. Y. Zhu, B. A. Song, D. X. Luo, Y. Gang, S. H. Chen, W. Xue and J. Wu, Bioorg. Med. Chem. Lett., 2018, 28, 2979–2984 CrossRef CAS PubMed.
- B. Bhyravbhatla, S. J. Watowich and D. L. D. Caspar, Biophys. J., 1998, 74, 604–615 CrossRef CAS PubMed.
Footnotes |
† Electronic supplementary information (ESI) available. See DOI: 10.1039/c9ra05139b |
‡ The first and the second authors contributed equally to this work. |
|
This journal is © The Royal Society of Chemistry 2019 |
Click here to see how this site uses Cookies. View our privacy policy here.