DOI:
10.1039/C9RA05933D
(Paper)
RSC Adv., 2019,
9, 35473-35482
Retracted Article: Astragaloside IV (AS-IV) alleviates the malignant biological behavior of hepatocellular carcinoma via Wnt/β-catenin signaling pathway
Received
31st July 2019
, Accepted 26th October 2019
First published on 1st November 2019
Abstract
Astragaloside IV (AS-IV) is an active substance isolated from Astragalus membranaceus (Fisch.) Bungede, which has been shown to have pharmacological effects in a variety of cancers. However, the effects of AS-IV in hepatocellular carcinoma (HCC) and its related mechanisms have been poorly understood. In this study, we explored the roles of AS-IV on HCC and the underlying signaling pathway. We reported that the appropriate concentrations of AS-IV (25, 50, 100 nmol l−1) significantly suppressed the proliferation and cell cycle of HepG2 and Hep3B cell lines whilst promoting apoptosis. Besides, a trans-well and wound healing assay showed that AS-IV could markedly inhibit the migration and invasion of HepG2 and Hep3B cells, the expression of E-cadherin was up-regulation but the expression of N-cadherin and vimentin was down-regulation, and the protein levels of cleaved-caspase-3, 9 were increased markedly compared with the corresponding control. Furthermore, animal model treatment revealed that AS-IV could effectively reduce tumor formation. Moreover, AS-IV also significantly weakened the expression of Wnt, β-catenin and TCF-4 in vitro and in vivo. Taken together, these results suggested that AS-IV inhibited the biological processes of HCC via regulating of the Wnt/β-catenin pathway.
1 Introduction
Currently, hepatocellular carcinoma (HCC), one of the most widespread human cancers, is the fundamental factor of cancer-related deaths and is the second major cause of cancer-related deaths around the world.1,2 The known aetiologies for HCC include chronic virus infection (B and C), liver cirrhosis, alcohol abuse, hepatic steatosis, nonalcoholic steatohepatitis (NASH) and/or aflatoxin exposure.3,4 Yet, a very great many therapies including liver resection, liver transplantation, microwave ablation, and systemic treatment have been used in HCC patients. The high mortality rate of HCC is due to lack of available diagnostic tools and effective treatment methods, especially for the HCC patients in the advanced stages. At the present time, surgical resection is still the best treatment for HCC.5,6 However, the 10 year survival rate at all stages of cancer is less than 30%, which is attributed to recurrence after surgery.7 Although there has been extensive research, the underlying mechanisms during HCC progression remain unclear.
Generally, Astragalus membranaceus is a diffusely used traditional Chinese medicine that is famous for tonifying, diuresis, skin reinforcing, tissue generative actions and drainage abscess.8 Astragaloside IV (AS-IV) is a natural non-synthetic active component purified from Astragalus membranaceus, which has been widespreadly used in conventional Chinese medicine to therapy diseases. In addition, previous studies have also demonstrated that AS-IV possess phylactic effects against angiocardiopathy,9 liver fibrosis,10 lung cancer,11 breast cancer,12 allergic inflammation,13 etc. Furthermore, the pharmacological mechanism also mainly included anti-oxidative, anti-fibrotic, anti-inflammatory, anti-diabetes and immune regulation.10,14
In the last years, it has been demonstrated that HCC was associated with various pathways,15 such as MAPK/ERK,16 PI3K/Akt,17 TGF-β/EMT and Wnt/β-catenin signaling pathway,18 and so on. Besides, among these signaling cascades, the classical Wnt/β-catenin signaling pathway plays a vital function in the initiation, development and progression,19 and involving cell growth, cytoskeleton remodeling, survival, cell fate, stem cells maintenance and embryonic development.20,21 Yet, the Wnt/β-catenin pathway is relatively conservative, which regulates the key processes involving in tissue and organ formation, cellular homeostasis and disease process.22 The potential molecular mechanism of Wnt/β-catenin signaling pathway has been extensively researched, but the reason for high activation in HCC still remain unknown.
But so far, how AS-IV regulates Wnt/β-catenin pathway in HCC is rarely reported. In the present study, we investigate the potential biological mechanism of AS-IV in vitro and vivo, and then to better understand the key signaling pathways involved in HCC.
2 Materials and methods
2.1 Main materials
The AS-IV powder (C41H68O14, molecular weight: 784.970, purity ≥ 99%) was purchased from Shanghai Winherb Medical Technology Co., Ltd. (Shanghai, China). HepG2 and Hep3B cell lines were purchased from Shanghai Cell Bank, Chinese Academy of Sciences (Shanghai, China). Specific pathogen-free (SPF) male BALB/c (6 weeks old, 20 ± 2 g) nude mice were purchased from Beijing Vital River Lab Animal Technology Co., Ltd. (Beijing, China).
2.2 Cell culture
HepG2 and Hep3B cell lines were cultured in dulbecco's modified eagle medium (DMEM, high glucose, Gibco™, Thermo Fisher Scientific, USA) which were supplemented with 10% fetal bovine serum (FBS, Gibco™, Thermo Fisher Scientific, USA), penicillin (100 U ml−1) and streptomycin (0.1 mg ml−1) at 37 °C incubator with 5% CO2.
2.3 Clone formation assay
Three different concentrations of AS-IV (25, 50 and 100 nmol l−1) were treated for 48 hours with 1 × 102 HepG2 and Hep3B cells, respectively. Then they were inoculated into 60 mm culture dish and cultured for 2 weeks. The two cells were washed with PBS twice and fixed in 4% paraformaldehyde (Sigma-Aldrich, St. Louis, USA) for 15 min, followed by incubation with Wright–Giemsa stain (Solarbio Life Science, Beijing, China) for 10 min. Finally, the pictures were collected and colonies were counted. Clone formation rate = (number of clones/number of inoculated cells) × 100%.
2.4 Flow cytometry
In brief, HepG2 and Hep3B cells were fixed for 24 hours, and then the fixed cells were stained with Cell Cycle and Apoptosis Analysis Kit (Beyotime, Shanghai, China) according to the manufacturer's procedures. Finally, red fluorescence was detected by flow cytometry at 488 nm wavelength, and light scattering was detected at the same time by a flow cytometer (Thermo Fisher Scientific, Waltham, USA).
2.5 Trans-well analysis
Trans-well assay was analyzed with polycarbonate membrane Transwell cell chamers inserts (Corning, New York, USA). The cells in serum-free medium was starved for 24 h, then the AS-IV-treated HepG2 and Hep3B cells suspension were seeded to the upper chambers (200 μl per chamber), respectively. Similarly, the lower chambers were supplemented with 10% FBS complete medium (600 μl), and the chamber was incubated for 24 h (37 °C and 5% CO2). Next, the cells on the upper side of the membrane were wiped with cotton swabs, yet, the invaded cells were fixed with 95% alcohol and following stained with 0.1% crystal violet (Sigma-Aldrich, St. Louis, USA) for 15 min. Finally, images were captured in at least three independent fields under an optical microscope to cell count (Leica, Germany).
2.6 Wound healing assay
The HepG2 and Hep3B cells were cultivated in 6-well plates with a density of 1 × 106, respectively, and cultured in complete medium until formed a confluent monolayer cell, and then the 100% confluent monolayer cell was scratched with a sterile 200 μl micropipettor. Subsequently, the cells suspension were cleaned in normal medium. Images of the monolayer wound were acquired at 0 and 24 h using an inverted microscope in three randomly selected visual areas (×200 magnification). The migratory ability was calculated as the ratio of the open area at 24 h to 0 h, and all experiments were performed at least three times.
2.7 Immunofluorescence (IF) assay
At room temperature, the AS-IV-treated HepG2 and Hep3B cells were immobilized with 4% formaldehyde for 20 min, permeated with 0.5% Triton X-100 for 15 min, and closed by 10% goat serum working liquid for 2 h. Then, the samples were incubated with anti-vimentin antibody (1:300; ab92547, Abcam, USA) overnight at 4 °C. The following day, after cleaning with PBS, the cells were incubated with Horseradish Peroxidase-Conjugated goat anti-rabbit secondary antibody (1:200, ab150077, Abcam, USA) for 1 h at 37 °C. After cleaning with PBS, staining with DAPI (Beyotime, Shanghai, China) for 10 min. Finally, the redundant DAPI was washed with PBS and the slides of cells were sealed with anti-fluorescence quenching agent. Images were captured using a fluorescence microscope, and all experiments were performed at least three times.
2.8 Animal model and treatment
HepG2 cells were injected subcutaneously with PBS 1 × 107 into each male BALB/c nude mice for the sake of producing implanted tumors. When the tumors reached a volume of 100 mm3, and were injected intraperitoneally for 7 consecutive days (50 mg kg−1 of AS-IV). Similarly, the control group was injected with 0.9% NaCl. After 30 days later, the tumors were weighed, then a part of tissues were fixed in 10% formalin for histopathological assay, and another part of tissues were employed for western blotting assay. The mice were kept with controlled environment (temperature 24 °C, humidity 60%, and 12 h light/dark cycles), had free access to food and water. All animal procedures were approved by the ethics committee of Gansu provincial hospital (SYXK-Gan-20190021).
2.9 Immunohistochemistry (IHC) assay
Paraffin was used to embedding the tissue of nude mice which had been fixed with 10% formalin, and the paraffin blocks were sliced to a thickness of 4 μm. Next, the tissue sections were rehydrated after dewaxing. The slides were incubated on PBS containing 30% H2O2 for 30 min towards blocking endogenous peroxidase activity, and antigen was repaired in heated citrate buffer for 10 minutes. In addition, the samples were incubated with following primary antibodies: anti-caspase-3 antibody, ab13847, 1:500; anti-VEGF antibody, ab32152, 1:300 (Abcam, USA), holding overnight in a 4 °C refrigerator. Subsequently, the matching secondary antibody were incubated for 1 h at 37 °C. Finally, the antigen–antibody binding was visualized with DAB (ZSGB-BIO Co., Ltd., Beijing, China), and samples sections were redyed with hematoxylin. The images were observed using a light microscopy, and all experiments were performed at least three times.
2.10 Western blot (WB) assay
Briefly, the total protein of tissues and cells were extracted with RIPA lysis buffer (P0013B, Beyotime Biotechnology Co., Ltd., Shanghai, China) according to the original manufacturer's instructions. The concentrations of protein was tested by BCA Protein Assay Kit (Thermo Scientific™, MA, USA). The proteins were separated by sodium dodecyl sulfate-polyacrylamide gel electrophoresis (SDS-PAGE, Millipore, MA, USA) and then transferred to polyvinylidene fluoride (PVDF) membrane. Next, placed the membrane in 5% nonfat milk blocking solution at 4 °C overnight. The target proteins were incubated with primary antibodies (Abcam, UK), holding overnight in a 4 °C refrigerator, and then incubated with the matching secondary antibody 1 h at 37 °C, respectively. Finally, the bands were revealed using enhanced chemiluminescence (ECL) substrate (Thermo Scientific™, MA, USA). β-Actin acted as the internal reference, all experiments were performed at least three times.
2.11 Statistical analysis
Statistics were analyzed and processed by SPSS 19.0 (SPSS, Inc., Chicago, USA). All data were showed as means ± standard deviation (S.D.), statistical comparison was evaluated by Student's t-test. p < 0.05 was considered to have statistically significant. The significant differences among experimental groups was determined by ANOVA analysis.
3 Results
3.1 AS-IV regulated hepatoma cells proliferation and cell cycle in a concentration-dependent manner
In order to investigate the effects of different concentrations of (25, 50 and 100 nmol l−1) AS-IV on the proliferation of hepatocellular carcinoma cell, clone formation assay was used to determine the proliferation of HepG2 and Hep3B cell lines. We found that the proliferation abilities were markedly decreased (Fig. 1B) when compared with the corresponding control. In addition, as shown as Fig. 1C, the proportion of G2/M phase cells in different concentrations of AS-IV treatment group was significantly increased in HepG2 cell line, compared with the control group. In contrast, the proportion of the G0/G1 phase cell was notably elevated in Hep3B cell line, indicated that AS-IV promoted G2/M arrest of HepG2 cells and G0/G1 arrest of Hep3B cells in a concentration-dependent manner. Consequently, these findings suggested that AS-IV had stress effects for inhibiting hepatoma cancer cell growth and cell survival.
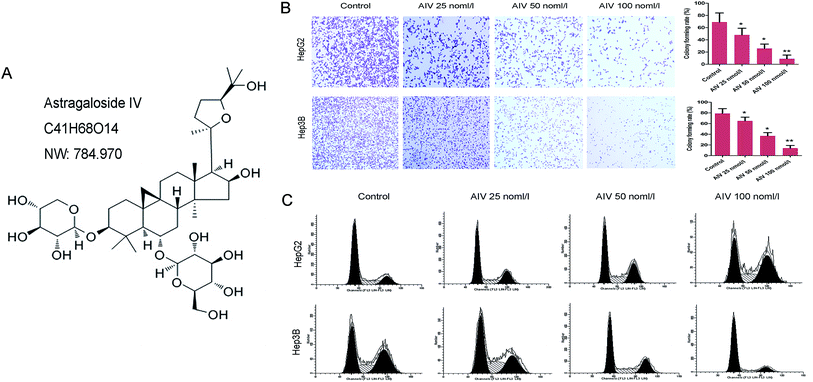 |
| Fig. 1 Effect of AS-IV on cell proliferation and cell cycle. The HepG2 and Hep3B cell lines were conducted with AS-IV in a range of concentrations (25, 50 and 100 nmol l−1), respectively. (A) The chemical structural formula of AS-IV. (B) Cell proliferation viability was performed by cloning formation assay, visual areas were magnified at ×100. (C) Cells cycle were analyzed by flow cytometry. Data are showed as mean ± S.D, all individual experiments were presented at least three times. *p < 0.05 and **p < 0.01 compared with the control group. | |
3.2 AS-IV induced hepatoma cells apoptosis in a concentration-dependent manner
For better realizing the effect of AS-IV on cell growth, we detected cell apoptosis or necrosis using flow cytometry. The data showed that the apoptosis rate of HepG2 and Hep3B cells markedly increased after AS-IV treatment (25, 50 and 100 nmol l−1), compared with control group (Fig. 2A). Besides, the label protein levels (caspase-3 and caspase-9) of apoptosis were confirmed employing western blotting assay, and we found that protein levels of cleaved-caspase-3 and cleaved-caspase-9 increased significantly (Fig. 2B and C) after AS-IV-treatment.
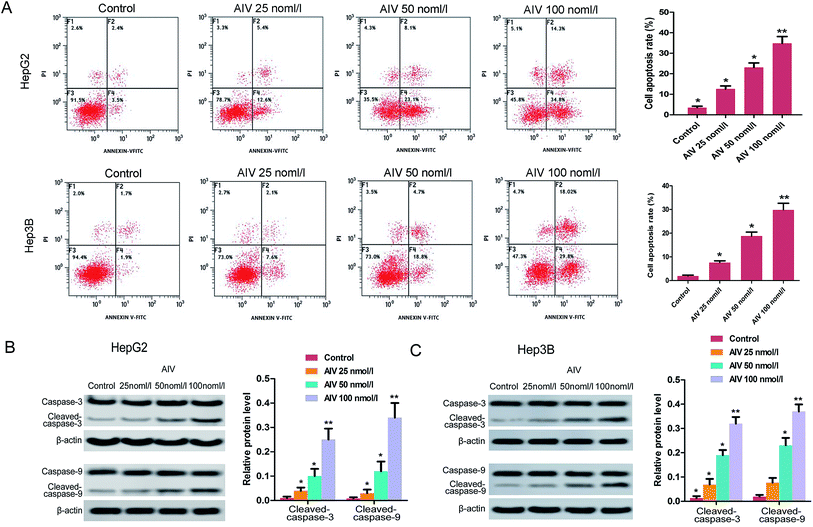 |
| Fig. 2 Effect of AS-IV on cell apoptosis. The HepG2 and Hep3B cell lines were conducted with AS-IV in different concentrations (25, 50 and 100 nmol l−1), respectively. (A) Cell apoptosis was detected by flow cytometry. (B and C) The expression levels of cleaved-caspase-3 and cleaved-caspase-9 protein and semi-quantitative analysis of the relative values normalized to β-actin. Data are showed as mean ± S.D, all individual experiments were presented at least three times. *p < 0.05 and **p < 0.01 compared with the control group. | |
3.3 AS-IV suppressed the invasion and migration of human hepatoma cells through a concentration-dependent manner
Objective to investigate the HCC metastasis, the wound healing assay showed that different concentrations (25, 50 and 100 nmol l−1) of AS-IV inhibited the cell migration of HepG2 and Hep3B, the migration capacities were decreased significantly (Fig. 3A). Moreover, the trans-well assay revealed that different concentrations of AS-IV also damaged the cell invasion ability of HepG2 and Hep3B, and the invasion abilities were weakened significantly (Fig. 3B). To further evaluate the pharmacological importance of AS-IV to invasion and migration, we then analyzed the expression of representative maker proteins VEGF and MMP-14 in AS-IV-treated HepG2 and Hep3B cell lines. As demonstrated in Fig. 4A, compared with the corresponding control, the expression of VEGF and MMP-14 protein were decreased markedly. Taken together, our results might speculate that AS-IV effectively suppressed invasion and migration capabilities of the human hepatoma cells.
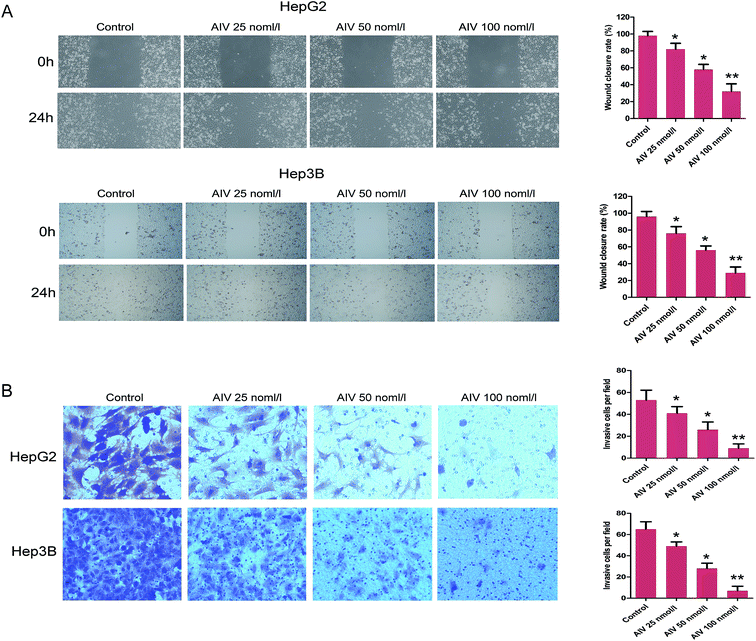 |
| Fig. 3 Effect of AS-IV on the migration and invasion in HepG2 and Hep3B cell lines. HepG2 and Hep3B cells were treated with AS-IV of different concentrations (25, 50 and 100 nmol l−1) for 24 h, respectively. (A) The effect of cell migration was tested by wound healing, visual areas were magnified at ×100. (B) The effect of cell invasion was examined by trans-well assay, visual areas were magnified at ×200. Data are showed as mean ± S.D, all individual experiments were presented at least three times. *p < 0.05 and **p < 0.01 compared with the control group. | |
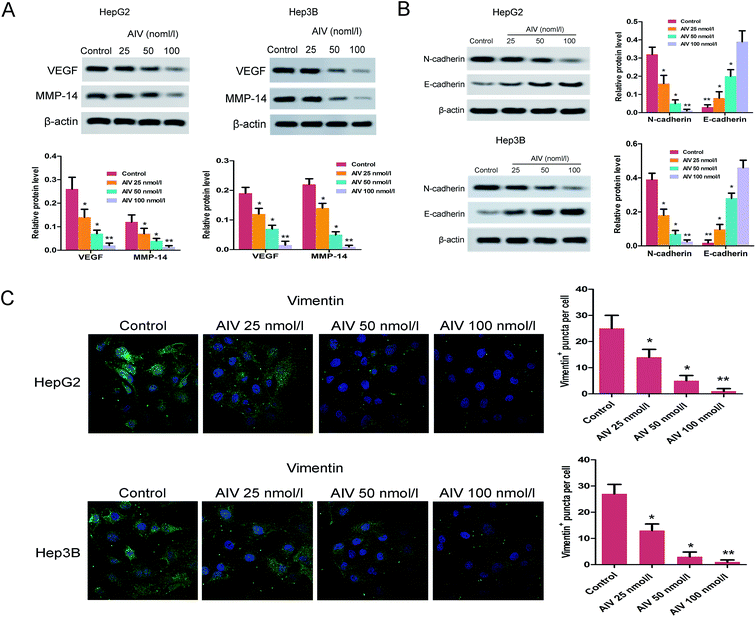 |
| Fig. 4 Effect of AS-IV on the levels of N-cadherin, E-cadherin and vimentin in HepG2 and Hep3B cell lines. (A) The protein levels of VEGF and MMP-14 were examined by WB assay (B) WB analysis the protein levels of E-cadherin and N-cadherin of AS-IV-treated in different concentrations (25, 50 and 100 nmol l−1). (C) Immunocytochemical analysis the protein level of vimentin, visual areas were magnified at ×200. Data are showed as mean ± S.D, all individual experiments were presented at least three times. *p < 0.05 and **p < 0.01 compared with the control group. | |
3.4 AS-IV inhibited the levels of N-cadherin, E-cadherin and vimentin in HepG2 and Hep3B cell lines
Based on the previous study, it had been proved that the invasion and migration of tumor cells were attributed to their phenotypic modulation, which involved a trans-differentiation channel known as epithelial–mesenchymal transition (EMT).23 To further confirmed whether AS-IV-treated HepG2 and Hep3B cell are involved in the regulation of invasion and migration and the possible mechanism, western blot and immunofluorescence assays were respectively conducted to evaluate the levels of the correlative proteins. As for the EMT associated maker proteins, the western blot (Fig. 4B) and immunofluorescence (Fig. 4C) showed that the expression of E-cadherin was increased, but the expression levels of N-cadherin and vimentin were decreased in the AS-IV-treated cell lines, compared to the matching control. These results suggested that AS-IV inhibited the initiation of tumor invasion and migration via EMT.
3.5 AS-IV inhibited expression of the Wnt/β-catenin/TCF-4 in vitro
Previous researches had shown that the Wnt/β-catenin/TCF-4 axis acted a vital role in the regulation of different biological processes, such as cell proliferation, survival, migration and polarization.24 Therefore, for purpose of investigating the underlying mechanisms by which AS-IV was utilizing its cytotoxic effects, we tested the expression of components of Wnt/β-catenin/TCF-4 axis by western blot. As showed in Fig. 5A and B, the expression levels of Wnt, β-catenin and TCF-4 in the hepatoma cell lines were significantly decreased compared with the corresponding control group, following treatment with different doses of AS-IV (25, 50 and 100 nmol l−1). These findings indicated that AS-IV inhibited hepatocellular carcinoma progression by suppressing excitement of the Wnt/β-catenin/TCF-4 axis in vitro.
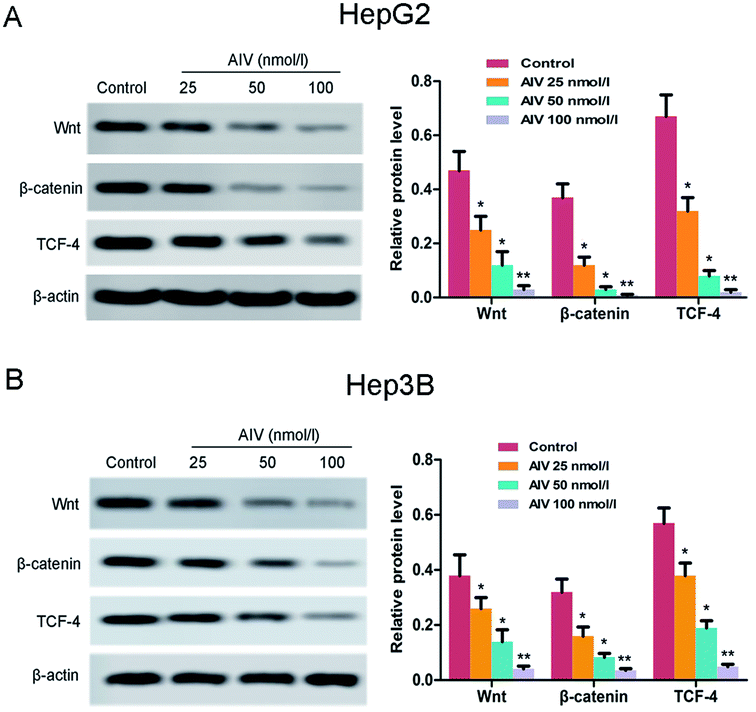 |
| Fig. 5 Effect of AS-IV on the Wnt/β-catenin/TCF-4 pathway in vitro. (A and B) The proteins levels of Wnt, β-catenin and TCF-4 in HepG2 and Hep3B cell lines treated with different concentrations of AS-IV (25, 50 and 100 nmol l−1), and semi-quantitative analysis of the relative values of Wnt, β-catenin and TCF-4 protein normalized with β-actin. Data are showed as mean ± S.D, all individual experiments were presented at least three times. *p < 0.05 and **p < 0.01 compared with the control group. | |
3.6 AS-IV suppressed the growth of xenograft tumors in mice and induced tumor cell apoptosis via suppressing excitement of the Wnt/β-catenin/TCF-4 pathway in vivo
We had simply investigated the effect of AS-IV on tumors in vitro, for further confirming the anti-tumor effect of AS-IV in vivo, we formed a HepG2 xenograft tumor model. When the mice tumors reached a volume of 100 mm3, and were injected intraperitoneally equivalent to 50 mg kg−1 of AS-IV lasting one week. After only 30 days of injection, the tumor weights of AS-IV-treated nude mice were remarkably lost compared to corresponding control (Fig. 6A and B). Next, we also performed immumohistochemical staining to assess the levels of caspase-3 and VEGF, the results demonstrated that AS-IV could promote apoptosis of tumor cells and inhibit the protein expression of VEGF in tumor tissues (Fig. 6C and D). Finally, we tested the expression of components of Wnt/β-catenin/TCF-4 axis through western blot assay. As demonstrated in Fig. 6E and F, the protein levels of Wnt, β-catenin and TCF-4 in the hepatoma tissue was remarkable down-regulation compared with corresponding non-AS-IV-treated group. These data, taken together, further approved that AS-IV suppressed tumor growth and progression, also induced tumor cells apoptosis via inhibiting excitement the Wnt/β-catenin/TCF-4 pathway.
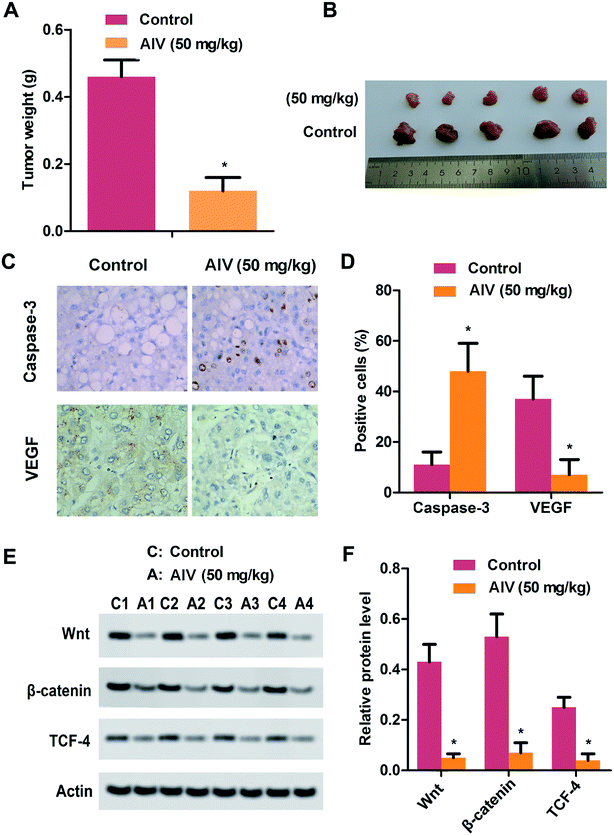 |
| Fig. 6 Effect of AS-IV on the growth of xenograft tumors in mice. The nude-mouse were injected with AS-IV (50 mg kg−1, lasting a week) at 30 day in vivo. (A and B) The weights of isolated tissues were measured. (C and D) The effects of AS-IV on the levels of caspase-3 and VEGF via IHC, visual areas were magnified ×400 (E and F). The proteins levels of Wnt, β-catenin and TCF-4 were examined by WB. Data are showed as mean ± S.D, all individual experiments were presented at least three times. *p < 0.05 compared with the control group. | |
4 Discussion
AS-IV is a cycloartane-type triterpene glycosides extracted from traditional Chinese medicine (white or pale yellow powder). Molecular formula is C41H68O14, molecular weight is 784.97, melting point is 295.0–296.0 °C, and it is easily soluble in organic solvents such as methanol, which has been widely used in the treatment of various cancers. AS-IV is a conventional Chinese herbal medicine, which is extensively applied to conflict with various types of cancers. A previous research showed that AS-IV remarkably transformed the loss of neurocyte activity and also inhibited the Bax/Bcl-2-mediated apoptosis.25 Sun et al. reported that AS-IV suppressed the proliferation of colorectal cancer (CRC) cells and also suppressed the neoplasm growth in CRC xenograft mouse models via a dose-dependent manner.26 Similarly, our experiments revealed that AS-IV effectively suppressed hepatoma cancer cell proliferation in a concentrations-dependent manner, and did prevented weight gain in tumor tissue. Besides, AS-IV significantly suppressed HK-2 cell apoptosis induced by high glucose, which may be involved in inhibiting the expression of Bcl-2 linked X protein and cleaved-caspase-3, 9, yet promoting the expression of Bcl-2.27 Liu et al. found that AS-IV decreased the apoptotic ratio of human neurocytes caused by hydrogen peroxide (H2O2).28 On the other side, as well known, caspases is a family of genes, which regulate cell death and inflammations to maintain homeostasis.29 Previously, it has been demonstrated that the internal and external leading causes of apoptosis are associated with caspases gene family, such as caspase-3 and/or caspase-9.30 Accordingly, caspase-3 and caspase-9 played key role in apoptosis and were closely related to the progression and prognosis of carcinomatosis, also could be used as bio-markers for oncogenesis in oral tongue squamous cell carcinoma (OTSCC).31 In this study, our results showed that the AS-IV treatment significantly reduced the cell proliferation and induced apoptosis, and the levels of cleaved-caspase-3 and cleaved-caspase-9 increased significantly in vitro. Therefore, these results, as well as others, indicated that AS-IV had stress effects for inhibiting HCC cell growth and promoting HCC cell apoptosis through initiating functional caspases.
Furthermore, angiogenesis is an important physiological process involving various of pathological situations, including endothelial cell proliferation, wound healing, tumor growth, and metastasis.32 In addition, vascular endothelial growth factor (VEGF), as an important regulator of angiogenesis, which could induce angiogenesis via stimulating endothelial cell proliferation, endothelial cell migration, and lumen formation.33 Subsequently, the matrix metalloproteinases (MMPs) also have been presented to be associated with angiogenesis,34 and MMP14 (MT1-MMP), the first identified transmembrane-containing MMP, is a collagenase involving in varieties of pathological processes, including cancer invasion and metastasis.35 In the current study, our results showed that VEGF level was decreased markedly in vitro and vivo and MMP-14 protein level was decreased significantly in vivo. Furthermore, the wound healing and trans-well assay also revealed that the ability of cell invasion and migration were significantly suppressed. Therefore, these data indicated that AS-IV inhibited hepatoma cancer cell migration and invasion via regulating the expression of VEGR and MMP-14 protein.
Previous reports demonstrated that metastasis is an important and complicated multistep in human cancers, including cell invasion, cell migration and the epithelial–mesenchymal transition (EMT).36 At the same time, EMT process played a excellent role in the progression and metastasis through cancer cells move from mesenchymal to the epithelial site.37 Wang et al. reported that ursolic acid (UA) promoted caspase-3 mediated apoptosis, meanwhile the expression of E-cadherin was notably increased and the expression of vimentin was significantly decreased, consequently inhibiting or weakening EMT.38 Xu et al. found that overexpression of nerve guidance factor 4 (NTN4) weakened cell migration and invasion, and caused down-regulation of N-cadherin and vimentin expression,39 so that the cancer cells enhanced the capabilities of migration and invasion.40,41 Moreover, there was another evidence revealed that AS-IV might also have effectively inhibit function on EMT.42 In our study, for further realizing the underlying mechanisms of AS-IV affecting the migration and invasion in hepatoma cells, we tested the expression of E-cadherin, N-cadherin and vimentin in AS-IV-treated HepG2 cells line, and found that the expression level of E-cadherin was obviously increased, then the levels of N-cadherin and vimentin were remarkable declined. In summary, all above results, suggested that AS-IV inhibited HCC invasion and metastasis via regulating EMT process. Therefore, combined with the results of the wound healing and trans-well assay, it had been further confirmed that AV could inhibit the migration and invasion of HCC cells, which might be another key drugs to control the deterioration of tumors.
Currently, the Wnt/β-catenin signaling pathway has been known to be involved in development of various cancers, and also contributed to manage the expression levels of genes related to cellular proliferation and apoptosis, thus promoting the response and progression of cancer.43,44 In the conventional Wnt/β-catenin signaling pathway, the presence of Wnt ligands facilitated continuing accumulation of β-catenin in the cytoplasm and nucleus, which bound to T cell factor/lymphoid enhancer factor (TCF/LEF).45 In the previous study, the AS-IV could impact on renal interstitial fibrosis via Wnt/β-catenin signaling pathway,46 Wang et al. reported that AS-IV inhibited Wnt4 expression in a concentration-dependent pathway, and the expression of β-catenin was also depressed by AS-IV in the cytoplasm or nucleus. Another, AS-IV could regulate the expression of Wnt and its receptors, as well as alter the levels of CK1, APC, and TCF/LEF in different degrees.47 Furthermore, the result of genome-wide RNA-mediated interference (RNAi) screening revealed that TCF4 was a transcriptional inhibitor which decreased the Wnt signaling pathway export and restricted CRC cell growth.48 Similarly, our study revealed the expression of Wnt, β-catenin and TCF-4 was dramatically down-regulated in vitro and in vivo through a concentration-dependent manner.
5 Conclusions
In conclusion, the results in our study presented the inhibition of AS-IV about the cell proliferation, cell cycle, apoptosis, migration and invasion in vitro, and suppressed the growth of xenograft tumors in vivo. In addition, the data were also revealed that the effect of AS-IV on Wnt/β-catenin signaling pathway, indicating that AS-IV regulated HCC progression and metastasis via Wnt/β-catenin signaling pathway and provided a novel approach and strategy for the treatment of HCC.
Ethical approval
All of the animal experiments were performed according to the Animal Care and Use guide of Laboratory Animals (NIH, published 2011), and were authorized by the Gansu Provincial Hospital Ethics Committee.
Authors' contributions
ZY participated in the experiment design, contributed to the cell and the animal experiments, performed the data analysis and statistics, and drafted and compiled the manuscript. MZ participated in the experiment design, independently performed the immunofluorescence and the immunohistochemistry experiments, yet drafted and compiled the manuscript. Both ZY and MZ have checked and agreed the final manuscript for publication.
Conflicts of interest
All authors have declared that there are no competitive interest.
References
- T. Akinyemiju, S. Abera, M. Ahmed, N. Alam, M. A. Alemayohu, C. Allen, R. Al-Raddadi, N. Alvis-Guzman, Y. Amoako, A. Artaman, T. A. Ayele, A. Barac, I. Bensenor, A. Berhane, Z. Bhutta, J. Castillo-Rivas, A. Chitheer, J. Y. Choi, B. Cowie, L. Dandona, R. Dandona, S. Dey, D. Dicker, H. Phuc, D. U. Ekwueme, M. S. Zaki, F. Fischer, T. Fürst, J. Hancock, S. I. Hay, P. Hotez, S. H. Jee, A. Kasaeian, Y. Khader, Y. H. Khang, A. Kumar, M. Ku, H. Larson, A. Lopez, R. Lunevicius, R. Malekzadeh, C. McAlinden, T. Meier, W. Mendoza, A. Mokdad, M. Moradi-Lakeh, G. Nagel, Q. Nguyen, G. Nguyen, F. Ogbo, G. Patton, D. M. Pereira, F. Pourmalek, M. Qorbani, A. Radfar, G. Roshandel, J. A. Salomon, J. Sanabria, B. Sartorius, M. Satpathy, M. Sawhney, S. Sepanlou, K. Shackelford, H. Shore, J. Sun, D. T. Mengistu, R. Topór-Mądry, B. Tran, N. Kingsley, V. Vlassov, S. E. Vollset, T. Vos, T. Wakayo, E. Weiderpass, A. Werdecker, N. Yonemoto, M. Younis, C. Yu, Z. Zaidi, L. Zhu, C. J. L. Murray, M. Naghavi and C. Fitzmaurice, JAMA Oncology, 2017, 3(12), 1683–1691 CrossRef.
- R. L. Siegel, K. D. Miller and A. Jemal, Ca-Cancer J. Clin., 2016, 62(1), 7–30 CrossRef.
- G. Baffy, E. M. Brunt and S. H. Caldwell, J. Hepatol., 2012, 56, 1384–1391 CrossRef.
- M. Maluccio and A. Covey, Ca-Cancer J. Clin., 2012, 62, 394–399 CrossRef.
- L. Rossi, F. Zoratto, A. Papa, F. Iodice, M. Minozzi, L. Frati and S. Tomao, World J. Gastrointest. Oncol., 2010, 2, 348–359 CrossRef PubMed.
- M. Allaire and J. C. Nault, Curr. Opin. Oncol., 2017, 29(4), 288–295 CrossRef CAS PubMed.
- B. C. Chapman, A. Paniccia, P. W. Hosokawa, W. G. Henderson, D. M. Overbey, W. Messersmith, M. D. McCarter, A. Gleisner, B. H. Edil, R. D. Schulick and C. Gajdos, J. Am. Coll. Surg., 2017, 224, 362–372 CrossRef.
- W. C. Cho and K. N. Leung, J. Ethnopharmacol., 2007, 113(1), 132–141 CrossRef PubMed.
- P. Yang, Y. Zhou, Q. Xia, L. Yao and X. Chang, Biol. Pharm. Bull., 2019, 42, 721–727 CrossRef CAS.
- L. Li, X. Hou, R. Xu, C. Liu and M. Tu, Fundam. Clin. Pharmacol., 2017, 31, 17–36 CrossRef CAS.
- L. Jia, D. Lv, S. Zhang, Z. Wang and B. Zhou, Oncol. Res., 2019, 27, 503–508 CrossRef.
- K. Jiang, Q. Lu, Q. Li, Y. Ji, W. Chen and X. Xue, Int. Immunopharmacol., 2017, 42, 195–202 CrossRef CAS.
- X. Huang, L. Tang, F. Wang and G. Song, Immunobiology, 2014, 219, 565–571 CrossRef CAS.
- Z. Cai, J. Liu, H. Bian and J. Cai, Exp. Ther. Med., 2016, 12(4), 2702–2708 CrossRef CAS.
- S. Hittaker, R. Marais and A. X. Zhu, Oncogene, 2010, 29, 4989–5005 CrossRef PubMed.
- M. Dimri, A. Humphries, A. Laknaur, S. Elattar, T. J. Lee, A. Sharma, R. Kolhe and S. Ande, Hepatology, 2019, 31215069 Search PubMed.
- D. W. Ren, H. D. Liu, R. Chen, Y. J. Sheng and T. Liu, Exp. Ther. Med., 2019, 17, 393–397 Search PubMed.
- Z.-H. Liu, Y.-F. Zhang and Z.-D. Xu, JBUON, 2018, 23(6), 1717–1724 Search PubMed.
- J. Chen, M. Rajasekaran, H. Xia, X. Zhang, S. N. Kong, K. Sekar, V. P. Seshachalam, A. Deivasigamani, B. K. Goh, L. L. Ooi, W. Hong and K. M. Hui, Gut, 2016, 65, 1522–1534 CrossRef CAS.
- R. Nusse and H. Clevers, Cell, 2017, 169(6), 985–999 CrossRef CAS.
- J. Chen, M. Rajasekaran and K. M. Hui, Exp. Biol. Med., 2017, 242, 1142–1149 CrossRef CAS.
- B. T. MacDonald, K. Tamai and X. He, Dev. Cell, 2009, 17, 9–26 CrossRef CAS.
- J. Roche, Cancers, 2018, 10(2), 52 CrossRef.
- J. N. Anastas and R. T. Moon, Nat. Rev. Cancer, 2013, 13(1), 11–26 CrossRef CAS.
- Z. G. Zhang, L. Wu, J. L. Wang, J. D. Yang, J. Zhang, J. Zhang, L. H. Li, Y. Xia, L. B. Yao, H. Z. Qin and G. D. Gao, Mol. Cell. Biochem., 2012, 364, 209–216 CrossRef CAS.
- P. Sun, Y. Liu, Q. Wang and B. Zhang, Front. Biosci., 2019, 24, 597–606 CrossRef CAS.
- J. Wang and H. Guo, Exp. Ther. Med., 2019, 17(6), 4409–441628 CAS.
- X. Liu, J. Zhang, S. Wang, J. Qiu and C. Yu, Int. J. Mol. Med., 2017, 40(6), 1772–1780 CAS.
- D. R. McIlwain, T. Berger and T. W. Mak, Cold Spring Harbor Perspect. Biol., 2013,(4), a008656 Search PubMed.
- J. C. Reed, Am. J. Pathol., 2000, 157(5), 1415–1430 CrossRef CAS.
- P.-F. Liu, Y.-C. Hu and B.-H. Kang, PLoS One, 2017, 12(7), e0180620 CrossRef.
- D. Azar, Trans. Am. Ophthalmol. Soc., 2006, 104, 264–302 Search PubMed.
- K.-Y. Han, J.-H. Chang, H. Lee and D. T. Azar, Invest. Ophthalmol. Visual Sci., 2016, 57, 3313–3322 CrossRef CAS.
- J. D. Raffetto and R. A. Khalil, Biochem. Pharmacol., 2008, 75, 346–359 CrossRef CAS.
- H. Qiu, X. Xu and M. Liu, Mol. Med. Rep., 2017, 15(6), 3724–3734 CrossRef CAS.
- X. Zhang, P. Cui, B. Ding, Y. Guo, K. Han, J. Li, H. Chen and W. Zhang, Cancer Gene Ther., 2018, 25, 18–26 CrossRef CAS.
- B. De Craene and G. Berx, Nat. Rev. Cancer, 2013, 13, 97–110 CrossRef CAS.
- X. Wang, T. Wang and Y. Fan, Biol. Pharm. Bull., 2019, 42, 685–691 CrossRef CAS.
- X. P. Xu, Q. Y. Yan, Y. Wang and X. J. Dong, Oncol. Rep., 2017, 37, 449–457 CrossRef.
- Y. Zhang, X. Zhang, M. Ye, P. Jing, J. Xiong, Z. Han, J. Kong, M. Li, X. Lai, N. Chang and J. Zhang, Cancer Lett., 2018, 419, 75–78 CrossRef CAS.
- D. H. Kim, T. Xing, Z. Yang, R. Dudek, Q. Lu and Y. H. Chen, J. Clin. Med., 2017, 7(1), 29271928 Search PubMed.
- W. Qi, J. Niu, Q. Qin, Z. Qiao and Y. Gu, Cell Stress Chaperones, 2014, 19, 105–114 CrossRef CAS.
- Z. Li, L. Zhao and Q. Wang, Am. J. Transl. Res., 2016, 8, 2385–2393 CAS.
- Y. Cui, F. Zhang, C. Zhu, L. Geng, T. Tian and H. Liu, Oncotarget, 2017, 8, 17785–17794 Search PubMed.
- B. T. MacDonald, K. Tamai and X. He, Dev. Cell, 2009, 17(1), 9–26 CrossRef CAS.
- L. Wang, Y.-F. Chi and Z.-T. Yuan, Cell. Physiol. Biochem., 2014, 33, 1316–1328 CrossRef CAS.
- L. Wang, Y. F. Chi, Z. T. Yuan, W. C. Zhou, P. H. Yin, X. M. Zhang and W. Peng, Cell. Physiol. Biochem., 2014, 33, 1316–1328 CrossRef CAS.
- W. Tang, M. Dodge, D. Gundapaneni, C. Michnoff, M. Roth and L. Lum, Proc. Natl. Acad. Sci. U. S. A., 2008, 105, 9697–9702 CrossRef CAS.
|
This journal is © The Royal Society of Chemistry 2019 |
Click here to see how this site uses Cookies. View our privacy policy here.