DOI:
10.1039/C9RA07948C
(Paper)
RSC Adv., 2019,
9, 37384-37390
Sensitive detection of ascorbic acid using screen-printed electrodes modified by electroactive melanin-like nanoparticles
Received
30th September 2019
, Accepted 4th November 2019
First published on 15th November 2019
Abstract
In this work, we report on the design of an enzyme-less sensitive and selective electrochemical electrode for ascorbic acid (AA) detection using a modified screen-printed electrode of melanin-like nanoparticles (Mel-NPs). Cyclic voltammetry shows that the melanin-modified electrode exhibits high electrocatalytic activity for ascorbic acid. The melanin-like nanoparticles serve as a shuttle to transport electrons from ascorbic acid to the electrode surface. The modified electrode is endowed with a large dynamic window ranging from 5 to 500 ppb. The detection and quantification limits were estimated to be 0.07 and 0.23 ppb, respectively. The modified electrode was successfully used to determine AA in human blood serum, urine and saliva with satisfactory recovery levels.
1. Introduction
Ascorbic acid, vitamin C, is a widely used antioxidant in food, animal feed, beverages, and pharmaceutical formulations.1,2 It takes part in many important human life processes as it is necessary for promoting the generation of antibodies and the absorption of iron.3 Therefore, developing accurate, reliable, rapid and easy to implement methods for measuring low levels of ascorbic acid in real samples is of great importance. Despite the fact that vitamin C is unstable in aqueous solution since it is easily oxidized reversibly to dehydroascorbic acid and subsequently irreversibly to 2,3-diketo-L-gulonic acid,4 which make it difficult to be quantified, many methods have been developed for its determination. In addition to titrimetry,5 fluorimetry,6,7 flow injection analysis,8,9 spectrophotometry,10,11 solid-phase iodine method,12 and chromatography,13,14 electrochemical methods have attracted much interest because they are less sensitive towards the matrix effects than other analytical techniques and there is no need for derivatization of the analytes.15
Although ascorbic acid is electroactive, its direct detection using conventional electrodes such as glassy carbon, graphite and platinum is challenging because of its high overpotential resulting in electrode fouling, and poor selectivity and reproducibility.16 To overcome these challenges, increased investigations have been carried out with nanomaterials modified electrodes for AA sensing. Typical examples included carbon-supported NiCoO2 nanoparticles,17 branch-trunk Ag hierarchical nanostructure,18 NiFe2O4 nanoparticles,19 MoS2 nanosheets,20 CuS/Prussian blue core–shell nanohybrid,21 4-aminobenzoic acid functionalized herringbone carbon nanotubes,22 etc. Despite the enhanced performances of AA electrochemical sensors when using nanomaterials, they have not yet been successfully validated into marketed products which make it of paramount importance to construct portable, fast and highly sensitive real-time system for accurate point-of-care AA detection.
Great interests have been recently shown to melanin-like nanoparticles (Mel-NPs) because of their low-cost of production, low toxicity, high dispersibility, stability and biodegradability.23 More recently, they have been used to modify electrode to build an electrochemical sensor for a simultaneous detection of ascorbic acid, dopamine (DA) and uric acid (UA) using facile gold nanoplates and reduced graphene oxide modified glassy carbon electrode.24 The redox activity of melanin has been linked to pro-/antioxidant behavior, which makes it an excellent material for biomedical purposes. For instance, certain ‘black bacteria’ are protected against phagocytic mechanisms by a layer of melanin within their cell walls.25 Moreover, melanins' redox features in the solid state exhibit a fast charge time at the proper static dielectric constant which make it exploitable in electrical energy storage devices.26 Melanins have been also proposed for optoelectronic and photovoltaic applications,27 which make them interesting material with a wide range of possible technological applications.
In this work, we designed a sensitive and selective electrochemical ascorbic acid sensor using a modified screen-printed electrode by melanin-like nanoparticles, which were synthesized through neutralization of dopamine hydrochloride salt with NaOH followed by spontaneous air oxidation of dopamine at 50 °C. Screen-printed electrodes, enabling measurements in a minimum sample volume, were chosen to allow the future application of the developed electrochemical sensor in the elaboration of portable point-of-care testing system with a sample-in answer-out approach that enables their use by non-specialized personnel. Voltamperometric investigations showed that the melanin-modified electrode exhibits a high electrocatalytic activity for ascorbic acid. The voltammetric curves demonstrated excellent analytical performances with high selectivity towards ascorbic acid. The method for generating nanoparticles and electrode modification are depicted in Fig. 1.
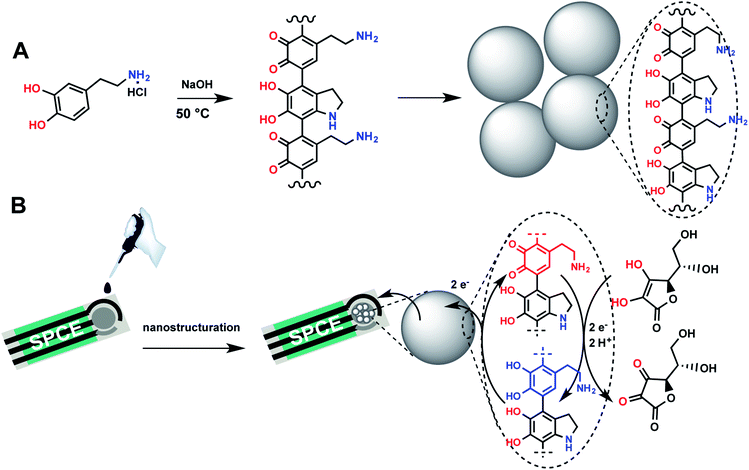 |
| Fig. 1 Principles of the preparation and sensing of ascorbic acid using a melanin-like-modified screen-printed carbon electrode. | |
2. Methods
2.1. Materials and apparatuses
Dopamine hydrochloride (≥98%), sodium hydroxide (≥97%), L-ascorbic acid (≥99%), uric acid (≥98%), sulfuric acid (96%) were purchased from Sigma-Aldrich (https://www.sigmaaldrich.com) and used without further purification. All solutions were prepared either in deionized water or in a 0.1 M phosphate-buffered saline (PBS) solution (pH 7.4) unless otherwise stated.
Melanin-like nanoparticles were prepared according to the procedure of Ju et al.28 In short, 760 μL of 1 M NaOH solution was added to a dopamine hydrochloride solution (2 mg mL−1), prepared by dissolving 180 mg in 90 mL of deionized water, and the suspension was heated to 50 °C under continuous stirring for 5 h. As soon as NaOH was added, the solution color turned to pale yellow and gradually changed to dark brown. The final product (i.e. melanin-like nanoparticles) was isolated by successive centrifugation and deionized water washing.
A Metrohm PGSTAT M204 electrochemical workstation equipped with an FRA32 impedance module controlled by Nova® software was used to record all cyclic and differential pulse voltammetric experiments (CV and DPV) and electrochemical impedance (EIS) measurements. Screen-printed carbon electrodes (SPCEs), incorporating a carbon working electrode, a carbon counter-electrode and a silver pseudo-reference electrode, were obtained from Orion Hi-Tech S. L. (Madrid, Spain) and were employed to perform all the electrochemical experiments. SEM micrographs and Energy-Dispersive X-ray Spectroscopy (EDX) analysis were performed using a FEI Quanta FEG250 ESEM scanning electron microscope.
2.2. Preparation of the modified electrode
First, the screen-printed carbon electrodes were cleaned and activated using cyclic voltammetry by scanning the potential for 5 cycles in the range −1.0 V to +1.0 V in 0.5 M H2SO4 solution. For the electrode preparation, a drop of melanin-like nanoparticles black homogenous suspension (1 mg mL−1), resulting from dispersed melanin-like nanoparticles in deionized water, was deposited on the cleaned electrode surface and was dried for 1 h at room temperature before use. These devices are designated to be MelNPs/SPCE.
2.3. Electrochemical measurements
All assays were run in triplicate by casting 25 μL of AA dissolved in a PBS solution on the working electrode. For all the CV and DPV measurements, the scan rate was fixed at 50 mV s−1and the potential range was limited to [–0.6 V, 1.0 V] prior and after successive additions of 2 μL from stock solutions to achieve 0.1, 0.2, 1, 2, 3, 5, 10, 15, 20, 50, 100, 200, 300 or 500 ng mL−1 of AA. For the selectivity tests, 25 μL (i.e. 50 ng) of dopamine or uric acid were casted onto the surface of the biosensor, the DPV current was recorded and compared to that obtained for 10 ng of AA. For real samples analysis, human serum, urine and saliva were provided by Prof. Besma Yacoubi-Loueslati (University of Tunis El Manar) and were part of the samples collected for her recent study.29 The study was conducted as per Helsinki II declaration and was approved by the Research & Ethic Committee of Salah Azaïz Institute in Tunis (registration number: ISA/2018/19, granted on 25 June 2017). Informed consent was obtained from all individual participants included in the study. The samples were diluted and no other treatment was carried out.
The impedance spectra were recorded in PBS solution containing 5 mM of [Fe(CN)6]4/3– at 0.2 V, in a frequency ranging from 100 kHz to 0.1 mHz, with an amplitude modulation of ±20 mV.
3. Results and discussion
3.1. Characterization and electrochemical properties of Mel-NPs
The morphology of the Mel-NPs was investigated by scanning electron microscopy and energy dispersive X-ray spectroscopy. As shown in Fig. 2A, the Mel-NPs are highly dispersed with an average size of approximately 200 nm. EDX analysis (Fig. 2B) reveals that they are composed by ca. 64% of carbon, 24% of oxygen and 12% of nitrogen, which is approximately the same composition as 2,3-dihydro-1H-indole-5,6-dione, supposedly the molecular unit forming melanin biopolymer. Fig. 2D depicts the stepwise design of the modified Mel-NPs/SPCE using [Fe(CN)6]4/3– as a redox probe. In fact, a noticeable electron-transfer improvement (i.e. ipc-to-ipa current ratio and peak-to-peak potential separation) can be observed after the nanostructuration step, which means that Mel-NPs probably served as a shuttle to transport electrons from and to the redox probe towards the electrode surface. Moreover, calculation, using Randles–Sevcik equation,30 of the real electrochemical surface area of SPCE before and after modification revealed an enhanced surface area of Mel-NPs/SPCE found to be 1.5 × 10−4 cm2 when compared to unmodified SPCE estimated to be 0.96 × 10−4 cm2, which is in great agreement with the observed improved electrical response. Furthermore, electrochemical impedance spectroscopy, used to probe the changes of electrode surface, shows that the charge-transfer resistance (RCT) of the electrode decreased after the deposition of Mel-NPs (Fig. 2E) indicating a better electrical conductivity and hence confirming the surface modification.
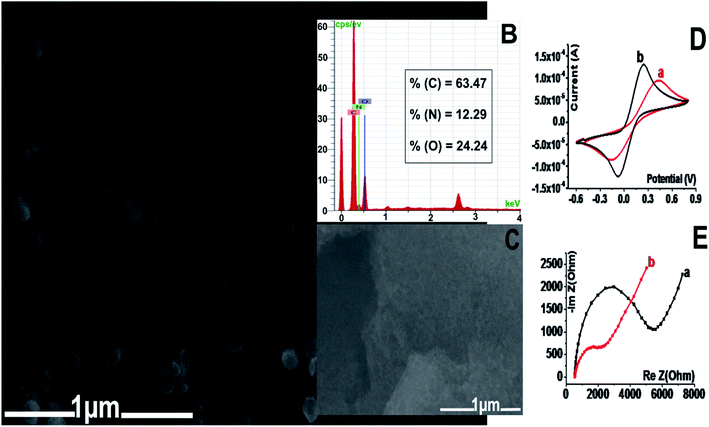 |
| Fig. 2 Characterization of the nanostructured SPCE electrode: (A) SEM image of the modified Mel-NPs/SPCE. (B) EDX analysis of the modified Mel-NPs/SPCE. (C) SEM image of unmodified SPCE. (D) CV and (E) EIS characterization of the SPCE electrode surface: before (a) and after (b) Mel-NPs deposition. | |
Compared to the bare SPCE electrode in a PBS solution, cyclic voltammetry of the Mel-NPs-modified electrode shows a quasi-reversible CV feature (curve b, Fig. 3A) related to the oxidation and reduction of melanin respectively at +0.4 V and −0.1 V while the former do not exchange any electrons (curve a, Fig. 3A). The exchanged electrons are due to the oxidation of the melanin hydroquinonoid moiety to their corresponding quinonoid form and their reduction to the former state. Furthermore, the ipc-to-ipa current ratio close to unity suggests that Mel-NPs keep its reversibility even when adsorbed onto the carbon surface. The oxidation and reduction peak currents increase linearly with scan rates in the range from 10 to 500 mV s−1, suggesting a surface-confined electron transfer process and thus confirming the immobilization of the Mel-NPs on the electrode surface (Fig. 3B). So, we sought to take advantage of the electrochemical features of these Mel-NPs to detect ascorbic acid without using an external redox mediator. In our case, the Mel-NPs will play at once the role of a nanometeric platform modifier and a redox transducer with which we can monitor any surface modification.
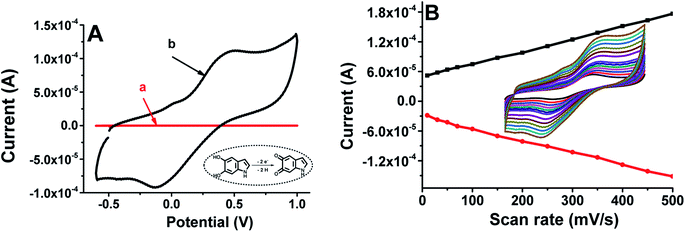 |
| Fig. 3 (A) Cyclic voltammetry curves of the electrode before (a) and after its modification with Mel-NPs (b) in PBS at 50 mV s−1. (B) Plots of anodic and cathodic peak currents vs. scan rates with inset corresponding to CVs of the modified electrode recorded at different scan rates (from inner to outer: 10–500 mV s−1). | |
3.2. Electrocatalytic activity of Mel-NPs in the presence of ascorbic acid
The electrochemical properties of the bare screen-printed electrode and Mel-NPs/SPCE were examined by cyclic voltammetry in a 0.1 M phosphate-buffered solution PBS containing 1, 5 and 10 mM of ascorbic acid at a scan rate of 50 mV s−1. Upon the addition of AA, the shape of the cyclic voltammograms of the Mel-NPs/SPCE electrode changes significantly to show only a large anodic peak at ca. +0.30 V (Fig. 4A) meanwhile, the cathodic peak disappears completely. Increasing the ascorbic acid concentration leads to the anodic current increase. This behavior can be explained by the electrocatalytic reactions that can be schematized using the following equations: |
AA → dehydro-AA + 2H+ + 2e−
| (1) |
|
Mel-NPs + 2H+ + 2e− → H2-Mel-NPs
| (2) |
|
H2-Mel-NPs (electrode) → Mel-NPs (electrode) + 2H+ + 2e−
| (3) |
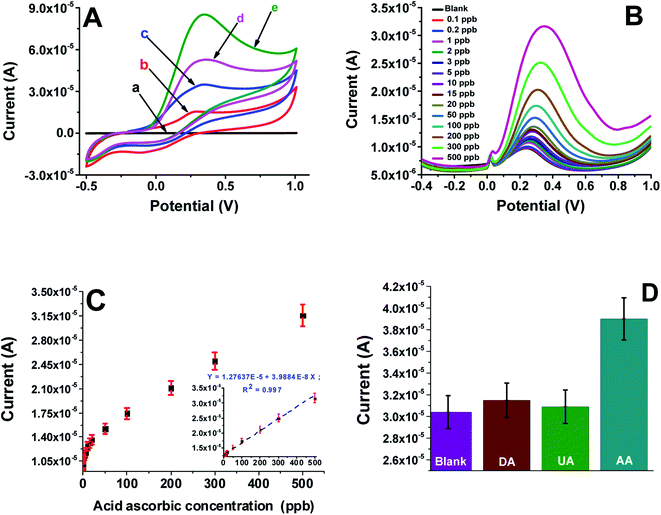 |
| Fig. 4 (A) Cyclic voltammograms of the SPCE (a) and Mel-NPs/SPCE (b) in PBS (0.1 M, pH = 7.4) in the absence of ascorbic acid, in PBS containing 1 mM (c), 5 mM (d) and 10 mM (e) ascorbic acid. Scan rate: 50 mV s−1. (B) DPV voltammograms obtained from different concentrations of ascorbic acid in PBS (0.1 M, pH = 7.4). (C) Calibration curve for ascorbic acid detection (the inset shows the linear concentration range). (D) DPV response of the Mel-NPs/SPCE to 10 ppb ascorbic acid and 50 ppb of uric acid and dopamine in PBS (0.1 M, pH = 7.4). | |
3.3. Sensor performances
The differential pulse voltammetry shows that oxidation current increased steadily with the increase of ascorbic acid concentration. Plotting the current variation versus AA concentration gives a straight with a wide dynamic window ranging from 5 to 500 ppb, with a slope of 0.38 nA ppb−1 and r > 0.997 indicating, respectively, a high sensitivity of the modified electrode and an excellent repeatability of the measurements after three runs. The limits of detection (LoD) and quantification (LoQ) were calculated using the formulae LoD = (3.3 × sb)/S and LoQ = (10 × sb)/S, respectively, where sb represents the standard deviation of the blank signal and S represents the sensitivity of the calibration curve. These limits were estimated to be ca. 0.07 ppb and ca. 0.23 ppb, respectively.
Comparison of the sensor performances in terms of limit of detection and linear range with previously reported sensors for AA were summarized in Table 1. As shown, the developed electrode exhibited lower detection limit than that of carbon-supported NiCoO2 nanoparticles,17 branch-trunk Ag hierarchical nanostructure,18 NiFe2O4 nanoparticles,19 MoS2 nanosheets,20 CuS/Prussian blue core–shell nanohybrid,21 and 4-aminobenzoic acid functionalized herringbone carbon nanotubes22 modified electrodes. Whereas, the obtained linear range was smaller than that obtained for others systems. Even known that the AA concentration levels for healthy people in serum are between 30 and 90 μM,22 that the average value of the AA excreted by urine has been established in 132 μM (based on a 1.5 L total urine volume in 24 hours),22 and that salivary vitamin C concentration shown in nonsmokers is in the range of 0.2–19 μM with a median of 0.6 μM,31 determination of AA in real human serum, urine and saliva samples will be achieved in scarcely diluted samples.
Table 1 Comparison of analytical performances of the as-developed Mel-NPs/SPCE sensor with other sensors reported in literature
Electrode materials |
Linear range (M) |
Detection limit (nM) |
Reference |
NiCoO2/C modified GCE |
10 × 10−6 to 2.63 ×10−3 |
500.0 |
17 |
Branch-trunk Ag hierarchical nanostructures/GCE |
0.017 ×10−6 to 1.8 ×10−3 |
60.0 |
18 |
NiFe2O4/SPGE |
5.0 × 10−7 to 1.0 × 10−4 |
10 000.0 |
19 |
MoS2–OCu/GCE |
0.015 × 10−3 to 11.75 × 10−3 |
22.2 |
20 |
CuS@PB/GCE |
5 × 10−6 to 3875 × 10−6 |
240.0 |
21 |
hCNTs-4ABA/Au-IDA electrode |
0 to 600 × 10−6 |
15 000.0 |
22 |
Mel-NPs/SPCE |
29 × 10−9 to 286 × 10−9 |
0.4 |
This work |
3.4. Interferences and stability of the modified electrode
The effect of naturally occurring substances such as dopamine and uric acid that can interfere with the Mel-NPs/SPCE electrode performances in the determination of ascorbic acid in complex media such as human body fluids was evaluated using cyclic voltammetry. The response of the Mel-NPs/SPCE to successive additions of 10 ppb ascorbic acid and five-folds (50 ppb) of uric acid or dopamine are displayed in the histogram of the Fig. 4D. Only small increases in the current can be observed with the two substances comparatively to the higher current obtained with 10 ppb of AA. The Mel-NPs/SPCE exhibits an excellent selectivity towards the examined substances especially considering the relative natural abundances in corporal fluid samples such as human serum or saliva.
The repeatability and reproducibility of the developed sensor were evaluated by DPV in a solution containing 10 ppb of AA in a 0.1 M PBS. Using the same modified electrode, the RSD for 5 successive measurements was 5.4%, revealing a good repeatability. Moreover, a RSD of ca. 4.3%, calculated from the responses of 5 independently prepared Mel-NPs/SPCE, is a clear evidence of an acceptable reproducibility. The long-term stability of the Mel-NPs/SPCE electrode was assessed by storing the electrode at RT for 2 weeks in PBS (0.1 M, pH 7.4) and checking its performance daily with the same concentration of AA. The sensor retained 92.7% of its initial response, indicating good long-term stability.
3.5. Detection of ascorbic acid in human serum, urine and saliva
The feasibility of the developed ascorbic acid sensor for practical application was evaluated by detecting the AA concentration in human blood serum, urine and saliva. Standard addition method was adopted according to previous reports.32 Different concentrations of AA standard solutions were added to diluted samples of serum (×1000), urine (×1000) and saliva (×10) in order to meet the dynamic range of the sensor. As listed in Table 2, the recovery values were found to be respectively in the range of 101.3–102.1%, 103.7–100.8% and 107.0–104.8% with standard derivations of less than 3.4%, 3.2% and 4.1% in human serum, urine and saliva respectively, indicating the great potential of the Mel-NPs/SPCE electrode for the practical and reliable determination of AA in real samples. Moreover, the recovery values obtained in all samples were in agreement with those obtained with hCNTs-4ABA/Au-IDA modified electrodes.22
Table 2 Detection of AA in real samples of human blood serum, urine and saliva
Samples |
Detected P |
Added Q |
Detected after addition R |
% recovery = 100×(R − P)/Q (±standard deviation) |
Serum |
12.45 |
20 |
32.70 |
101.30 ± 2.80 |
12.45 |
40 |
53.30 |
102.10 ± 3.40 |
Urine |
17.50 |
20 |
38.24 |
103.70 ± 3.20 |
17.50 |
40 |
57.83 |
100.83 ± 2.60 |
Saliva |
140.30 |
20 |
161.7 |
107.00 ± 4.10 |
140.30 |
40 |
182.20 |
104.80 ± 3.60 |
4. Conclusion
A melanin-like nanoparticles-modified screen-printed carbon electrode was prepared by a simple drop casting procedure and employed to construct an ascorbic acid sensor. Mel-NPs play at once the role of a nanometeric platform modifier and a redox transducer that shows high electrochemical performances for the electrocatalytic oxidation of AA. The developed sensor was successfully employed to detect AA in human body fluids with satisfactory results, revealing its promising practical applicability. Particularly, the prepared electrode may have promising potential applications for AA detection in clinical and biochemical analysis.
Conflicts of interest
On behalf of all authors, the corresponding authors state that there is no conflict of interest.
Acknowledgements
The authors acknowledge the help of Prof. Besma Yacoubi-Loueslati for providing the biological samples collected from consent informed patients and thank PRF NanoFastResponse (ref. PRF2017-D4P1) for financial support and the University of Tunis El Manar for the mobility grants awarded to W. Argoubi and A. Rabti.
References
- K. Pallauf, J. Bendall, C. Scheiermann, K. Watschinger, J. Hoffmann, T. Roeder and G. Rimbach, Vitamin C and lifespan in model organisms, Food Chem. Toxicol., 2013, 58, 255–263 CrossRef CAS PubMed.
- G. Maret and F. Jan, Vitamins C and E: beneficial effects from a mechanistic perspective, Free Radical Biol. Med., 2011, 51, 1000–1013 CrossRef PubMed.
- L. Yu, J. Zhao, S. Tricard, Q. Wang and J. Fang, Efficient detection of ascorbic acid utilizing molybdenum oxide@Prussian Blue/graphite felt composite electrodes, Electrochim. Acta, 2019, 322, 134712 CrossRef CAS.
- S. Yilmaz, M. Sadikoglu, S. Yagmur and G. Askin, Determination of Ascorbic Acid in Tablet Dosage Forms and Some Fruit Juices by DPV, Int. J. Electrochem. Sci., 2008, 3, 1534–1542 CAS.
- A. R. Mayers and C. G. Taylor, Determination of ascorbic acid in multivitamin tablets by thermometric titrimetry with cerium (IV), Analyst, 1987, 112, 507–509 RSC.
- T. Pérez-Ruiz, C. Martínez-Lozano, A. Sanz and E. Bravo, Simultaneous determination of doxorubicin, daunorubicin, and idarubicin by capillary electrophoresis with laser-induced fluorescence detection, Electrophoresis, 2001, 22, 134–138 CrossRef.
- X. Wu, Y. Diao, C. Sun, J. Yang, Y. Wang and S. Sun, Fluorimetric determination of ascorbic acid with o-phenylenediamine, Talanta, 2003, 59, 95–99 CrossRef CAS PubMed.
- T. Paixão and M. Bertotti, FIA determination of ascorbic acid at low potential using a ruthenium oxide hexacyanoferrate modified carbon electrode, J. Pharm. Biomed. Anal., 2008, 46, 528–533 CrossRef PubMed.
- T. Maki, N. Soh, K. Nakano and T. Imato, Flow injection fluorometric determination of ascorbic acid using perylenebisimide-linked nitroxide, Talanta, 2011, 85, 1730–1733 CrossRef CAS PubMed.
- P. Barrales, M. Fernández de Córdova and A. M. Díaz, Indirect determination of ascorbic acid by solid-phase spectrophotometry, Anal. Chim. Acta, 1998, 360, 143–152 CrossRef.
- C. Garnero and M. Longhi, Development of HPLC and UV spectrophotometric methods for the determination of ascorbic acid using hydroxypropyl-β-cyclodextrin and triethanolamine as photostabilizing agents, Anal. Chim. Acta, 2010, 659, 159–166 CrossRef CAS PubMed.
- M. Noroozifar and M. Khorasani-Motlagh, Solid-phase iodine as an oxidant in flow injection analysis: determination of ascorbic acid in pharmaceuticals and foods by background correction, Talanta, 2003, 61, 173–179 CrossRef CAS PubMed.
- H. Iwase and I. Ono, Determination of Ascorbic Acid in Soups by High-Performance Liquid Chromatography with Electrochemical Detection, J. Agric. Food Chem., 1997, 45, 4664–4667 CrossRef CAS.
- O. Heudi, T. Kilinç, P. Fontannaz and E. Marley, Determination of vitamin B12 in food products and in premixes by reversed-phase high performance liquid chromatography and immunoaffinity extraction, J. Chromatogr. A, 2006, 1101, 63–68 CrossRef CAS PubMed.
- A. M. Pisoschi, A. Pop, A. I. Serban and C. Fafaneata, Electrochemical methods for ascorbic acid determination, Electrochim. Acta, 2014, 121, 443–460 CrossRef CAS.
- Y. P. Dong, L. Huang, J. Zhang, X. F. Chu and Q. F. Zhang, Electro-oxidation of ascorbic acid at bismuth sulfide nanorod modified glassy carbon electrode, Electrochim. Acta, 2012, 74, 189–193 CrossRef CAS.
- X. Zhang, S. Yu, W. He, H. Uyama, Q. Xie, L. Zhang and F. Yang, Electrochemical sensor based on carbon-supported NiCoO2 nanoparticles for selective detection of ascorbic acid, Biosens. Bioelectron., 2014, 55, 446–451 CrossRef CAS PubMed.
- Y. Zhang, Z. Cai, M. Chen, M. Zhang, P. Liu and F. Cheng, A novel electrochemical ascorbic acid sensor based on branch-trunk Ag hierarchical nanostructures, J. Electroanal. Chem., 2018, 818, 250–256 CrossRef CAS.
- S. Jahani, Evaluation of the Usefulness of
an Electrochemical Sensor in Detecting Ascorbic Acid using a Graphite Screen-printed Electrode Modified with NiFe2O4 Nanoparticles, Anal. Bioanal. Electrochem., 2018, 10(6), 739–750 CAS.
- D. Li, X. Liu, R. Yi, J. Zhang, Z. Su and G. Wei, Electrochemical sensor based on novel two-dimensional nanohybrids: MoS2 nanosheets conjugated with organic copper nanowires for simultaneous detection of hydrogen peroxide and ascorbic acid, Inorg. Chem. Front., 2018, 5, 112–119 RSC.
- L. Li, P. Zhang, Z. Li, D. Li, B. Han, L. Tu, B. Li, Y. Wang, L. Ren, P. Yang, S. Ke, S. Ye and W. Shi, CuS/Prussian blue core–shell nanohybrid as an electrochemical sensor for ascorbic acid detection, Nanotechnology, 2019, 30, 325501 CrossRef PubMed.
- A. Abellán-Llobregat, C. González-Gaitán, L. Vidal, A. Canals and E. Morallón, Portable electrochemical sensor based on 4-aminobenzoic acid functionalized herringbone carbon nanotubes for the determination of ascorbic and uric acid in human fluids, Biosens. Bioelectron., 2018, 109, 123–131 CrossRef PubMed.
- Y. Liu, K. Ai, J. Liu, M. Deng, H. Yangyang and L. Lehui, Dopamine-Melanin Colloidal Nanospheres: An Efficient Near-Infrared Photothermal Therapeutic Agent for In Vivo Cancer Therapy, Adv. Mater., 2013, 25, 1353–1359 CrossRef CAS PubMed.
- C. Wang, J. Du, H. Wang, C. Zou, F. Jiang, P. Yang and Y. Du, A facile electrochemical sensor based on reduced graphene oxide and Au nanoplates modified glassy carbon electrode for simultaneous detection of ascorbic acid, dopamine and uric acid, Sens. Actuators, B, 2014, 204, 302–309 CrossRef CAS.
- D. Nosanchuk and A. Casadevall, Impact of Melanin on Microbial Virulence and Clinical Resistance to Antimicrobial Compounds, Antimicrob. Agents Chemother., 2006, 50, 3519–3528 CrossRef PubMed.
- Y. J. Kim, S. Chun, F. Whitacre and J. Bettinger, Biologically derived melanin electrodes in aqueous sodium-ion energy storage devices, Proc. Natl. Acad. Sci. U. S. A., 2013, 110, 20912–20917 CrossRef CAS PubMed.
- P. Meredith, C. Bettinger, M. Irimia-Vladu, A. Mostert and P. Schwenn, Electronic and optoelectronic materials and devices inspired by nature, Rep. Prog. Phys., 2013, 76, 36 CrossRef PubMed.
- K. Y. Ju, Y. Lee, S. Lee, S. Park and J. Lee, Bioinspired Polymerization of Dopamine to Generate Melanin-Like Nanoparticles Having an Excellent Free-Radical-Scavenging Property, Biomacromolecules, 2011, 12, 625–632 CrossRef CAS PubMed.
- M. Hadj-Ahmed, R. M. Ghali, H. Bouaziz, A. Habel, M. Stayoussef, M. Ayedi, M. Hachiche, K. Rahal, B. Yacoubi-Loueslati and W. Y. Almawi, Transforming growth factor beta 1 polymorphisms and haplotypes associated with breast cancer susceptibility: a case-control study in Tunisian women, Tumor Biol., 2019, 41(8), 1–9 Search PubMed.
- N. P. Shetti, D. S. Nayak, S. J. Malode and R. M. Kulkarni, An electrochemical sensor for clozapine at ruthenium doped TiO2 nanoparticles modified electrode, Sens. Actuators, B, 2017, 247, 858–867 CrossRef CAS.
- S. J. Padayatty and M. Levine, Vitamin C physiology: the known and the unknown and Goldilocks, Oral Dis., 2016, 22(6), 463–493 CrossRef CAS PubMed.
- W. Sun, Y. Z. Li, Y. Y. Duan and K. Jiao, Direct electrocatalytic oxidation of adenine and guanine on carbon ionic liquid electrode and the simultaneous determination, Biosens. Bioelectron., 2008, 24, 988–993 CrossRef CAS PubMed.
|
This journal is © The Royal Society of Chemistry 2019 |
Click here to see how this site uses Cookies. View our privacy policy here.