DOI:
10.1039/C9RA08062G
(Paper)
RSC Adv., 2019,
9, 40348-40356
Retracted Article: High-performance sono/nano-catalytic system: CTSN/Fe3O4–Cu nanocomposite, a promising heterogeneous catalyst for the synthesis of N-arylimidazoles†
Received
4th October 2019
, Accepted 29th November 2019
First published on 5th December 2019
Abstract
Herein, a promising heterogeneous nanoscale catalytic system constructed of chitosan (CTSN, as a polymeric basis), iron oxide nanoparticles (Fe3O4 NPs, as the magnetic agent), and copper oxide nanoparticles (CuO NPs, as the main catalytic active site) is presented. Firstly, a convenient synthetic route for preparation of this novel nanocatalyst (CTSN/Fe3O4–Cu) is presented. Further, the synergistic catalytic effect between the novel-designed catalyst and ultrasound waves (USW) in N-arylation coupling reactions of the imidazole derivatives (using various aryl halides) is precisely discussed. Concisely, high reaction yields (98%) have been obtained in short reaction time (10 min) through applying a partial amount (0.01 g) of this nanocatalyst. As the main reason for high catalytic activity of CTSN/Fe3O4–Cu, nanosized cluster-shaped morphology, which provides a wide surface active area, can be expressed. However, as the most distinguished properties of CTSN/Fe3O4–Cu catalytic system, high convenience in separation and excellent reusability could be mentioned. CTSN/Fe3O4–Cu nanocomposite can be easily recovered by using an external magnet and reused at least for eight times with no significant decline in the catalytic activity. Structural characterizations of this novel system have been done by various analytical methods and the obtained results have been well interpreted in the context.
1. Introduction
Recently, science and technology are shifting more toward design and development of the novel safe energy resources to create a healthier world. Thus, using clean and nonhazardous materials and executing the green methods have attracted so much attentions in different research fields. In chemical catalysis scope, numerous efforts have been made to design and fabricate efficient catalytic systems that include nontoxic materials in their structures and do not need any hazardous reagent for running the catalytic process.1,2 In recent decade, ultrasound wave (USW) irradiation has been introduced as one of the most effective strategies that can also be considered as a great co-catalyst agent for chemical reactions.3,4 From both physical and chemical aspects, USW irradiation activates the catalytic sites of the chemicals to enhance the performance of the catalytic systems. Also, USW irradiation leads to obtain high reaction yields in short reaction times, with the least amount of the wasted materials. Moreover, the structure of the heterogeneous catalytic systems will not be damaged under USW irradiation. Concisely, USW irradiation is more convenient, fast, safe and simple than traditional heating sources.5–7 Therefore, diverse organic reactions can efficiently be carried out under USW irradiation. As the main reasons, acoustic cavitation and cage effect through microject bubbles (microbubbles) that create high temperatures and microscopic pressures within few seconds lead to acceleration of the chemical reactions.8 As a result, USW irradiation is classified as a green, powerful, economical and environmentally-friendly approach in chemical reactions. In this study, we try to show the synergistic catalytic effect between USW (using an US cleaner bath) with a novel nanoscale catalytic system for facilitating the chemical synthesis reactions.
Proportional to the impressive and fast progress in nanotechnology, several nanoscale heterogeneous catalytic systems have been introduced by researchers to perform the complex chemical reactions with more convenience.9–12 Among various species of the reported heterogeneous catalytic systems, magnetic nanocatalysts have attracted more attentions due to including high convenience in separation and recycling processes. For instance, we recently reported some novel magnetic nanoparticles (NPs) that have been functionalized and applied for different catalytic aims such as facilitating peptide couplings and multicomponent synthesis reactions of pharmaceutical compounds.13,14 However, wide surface active area, great functionalization capability, nontoxicity, excellent stability and thermal resistance, and etc. could also be mentioned as the additional important reasons for employing the magnetic NPs for catalytic purposes. Among all of the different categories of magnetic NPs, iron oxide NPs species (Fe3O4, γ-Fe2O3, and etc.) are extremely used due to their easy preparation and super-paramagnetic behavior.15–17 In this work, we report a novel magnetic nanocatalyst that is constructed of copper nanoparticles (Cu NPs) as the main catalytic active sites, iron oxide nanoparticles (Fe3O4 NPs) as the magnetic agent, and chitosan (CTSN) as the natural polymeric matrix for composition and stabilization of the nanoscale ingredients (CTSN/Fe3O4–Cu).
In recent decades, N-arylated azoles e.g., arylpyrroles, arylpyrazoles, arylimidazoles, aryltriazoles, arylindoles, arylcarbazoles, and etc. have attracted so much attentions because of their important applications in medicinal and materials fields. Some of these scaffolds are also found in many bioactive natural products.18 Among all of the heterocyclic compounds, study on the N-aryl imidazoles is very intensive because of its famous biomedical properties such as receptor antagonists and thromboxane synthase inhibitors.19,20 In this regard, some ligand-free copper-catalyzed N-arylation reactions have been reported. For instance, Son et al. have prepared coated-CuO nanoparticles by thermal decomposition using copper acetylacetonate.21 Choudary et al. have designed a catalyst for N-arylation of imidazoles via the cation exchange of fluorapatite and tert-butoxyapatite with copper.22 Thus, designing a simple and efficient route for the synthesis of N-aryl or heteroarylamines remains highly desirable.
In this work, we try to facilitate the N-arylation of imidazoles and its derivatives by using a novel designed CTSN/Fe3O4–Cu nanocomposite, as an inexpensive magnetic nanocatalyst, under ‘ligand-free’ conditions. Firstly, a convenient route is introduced for preparation of this novel catalytic system. Then, as the distinguished advantages, high magnetic behavior that leads to easily separation after completion of the synthetic reactions with no need to some complex purification processes. Moreover, high stability of this heterogeneous catalytic system provides a great opportunity to reuse this system for several times without any decline in the catalytic performance. High performance of this catalytic system origins from a synergistic catalytic effect between the fabricated nanocomposite (CTSN/Fe3O4–Cu) and ultrasound waves, which is considered as a faster and safer method in comparison with other methods.23 For this purpose, all of the synthetic reactions have been carried out in an ultrasound cleaner bath with 50 kHz frequency and 200 W L−1 power density. From physical aspect, well-known cluster shape of the composite provide an extreme surface active area for catalytic aims. In addition of the mentioned excellences, substantial biocompatibility and biodegradability is obtained for this catalytic system, through applying a natural basis (CTSN) in the structure of the catalyst. As a result, a fast and safe strategy for the synthesis of the N-arylated imidazole derivatives is presented in this study, through which high reaction yields (98%) are obtained in short reaction time (10 min) with high safety and convenience.
2. Experimental
2.1. Materials and equipment
All the solvents, chemicals and reagents were purchased from Merck, Sigma and Aldrich. Melting points were measured on an Electrothermal 9100 apparatus and are uncorrected. FT-IR spectra were recorded on a Shimadzu IR-470 spectrometer by the method of KBr pellet. 1H and 13C Nuclear Magnetic Resonance (NMR) spectra were recorded on a Bruker DRX-500 Avance spectrometer at 500, 300, 75 and 125 MHz, respectively. Thermal analysis (TGA) was also done by using of Bahr-STA 504 instrument under argon atmosphere. FESEM images were taken by Sigma-Zeiss microscope with attached camera. Magnetic measurements of the solid samples were performed using Lakeshore 7407 and Meghnatis Kavir Kashan Co., Iran vibrating sample magnetometers (VSMs). Elemental analysis of the nanocatalyst was carried out by energy-dispersive X-ray (EDX) analysis which was recorded on a Numerix DXP-X10P. XRD measurements were carried out by using a DRON-8 X-ray diffractometer.
2.2. Practical methods
2.2.1. Synthesis of CTSN/Fe3O4 nanocomposite. In a round-bottom flask (100 mL), CTSN (high purity, 180 kDa) (0.5 g) was dissolved in a mix of dilute acidic deionized water (4.0 mL, pH = 5.5 by HCl) and ethanol (4.0 mL), at 80 °C under N2 atmosphere. Then, two aqueous solutions (0.1 M) of FeCl2·4H2O and FeCl3·6H2O were simultaneously added to the flask. Next, concentrate NH3 was slowly added to the mixture, until pH = 12 was obtained. The dark particles of the Fe3O4 were produced via co-deposition method and composited with CTSN during an in situ process. After completion of the addition, the magnetized CTSN was collected by an external magnet and washed with distilled water and ethanol, and dried in a vacuum oven.
2.2.2. Synthesis of CTSN/Fe3O4–Cu nanocomposite. In a round-bottom flask (50 mL), CTSN/Fe3O4 (0.1 g) was placed and well dispersed in deionized water (3.0 mL), via ultrasonication (10 min). Next, Cu(OAc)2 aqueous solution (5% wt, 2.0 mL) was added drop by drop to the aqueous mixture of CTSN/Fe3O4, and the mixture were ultrasonicated into an US bath (50 kHz, 200 W L−1) for 1 h, at 80 °C. Finally, the fabricated CTSN/Fe3O4–Cu nanocomposite were magnetically separated and washed with deionized water and ethanol and dried at room temperature.
2.2.3. General procedure for N-arylation of imidazoles by CTSN/Fe3O4–Cu nanocatalyst via USW irradiation. In a glass tube (Threaded Test Tube with Phenolic Cap, 13 × 100 mm), aryl halide (1.0 mmol), imidazole (2.0 mmol), CTSN/Fe3O4–Cu (0.01 mg), CTAB (0.5 mmol) and K2CO3 (1.5 mmol) were added in deionized water (4.0 mL). The mixture was sonicated for 10 min, at room temperature in an ultrasonic bath (50 kHz, 200 W). After completion of the reaction, the mixture was diluted with H2O (5.0 mL) and extracted with EtOAc (4 × 10 mL). The combined organic phases was washed with water and brine, dried over anhydrous Na2SO4, and concentrated in vacuum. The residue was purified by flash column chromatograph on silica gel (ethyl acetate/n-hexane, 2
:
1) to afford the target pure product. As same as excellent results were obtained in gram scale of the reaction by starting these ratio of raw materials and reagents.
2.2.4. Spectral data for selected products.
1-Phenyl-1H-imidazole 3a. 1H NMR (DMSO-d6, 300 MHz), δ (ppm): 7.14 (s, 1H), 7.37 (t, 1H, J = 7.2 Hz), 7.53 (t, 2H, J = 7.4 Hz), 7.67–7.78 (m, 3H), 8.3 (s, 1H). 13C NMR (DMSO-d6, 75 MHz): δ (ppm): 118.5, 121.5, 127.8, 130.1, 130.8, 135.7, 137.7.
1-p-Tolyl-1H-imidazole 3b. 1H NMR (CDCl3, 500 MHz), δ (ppm): 7.79 (s, 1H), 7.22 (m, 5H), 7.17 (s, 1H), 2.37 (s, 3H). 13C NMR (CDCl3, 125 MHz): δ (ppm): 137.7, 135.8, 135.4, 130.7, 130.4, 121.6, 118.6, 21.2.
3. Results and discussion
3.1. Preparation of CTSN/Fe3O4–Cu nanocatalyst
Initially, CTSN was dissolved in a mix of dilute acidic water (pH = 5.5) and ethanol with equal ratios, at 80 °C under N2 atmosphere. When a clear and homogeneous mixture was obtained, two aqueous solutions of FeCl2·4H2O and FeCl3·6H2O were added drop by drop to into the flask. Next, ammonia was slowly added to the mixture, until pH = 12 was obtained. The dark particles of the Fe3O4 were synthesized via an in situ co-deposition method and composited with CTSN. After completion of the addition, the magnetized CTSN was collected by an external magnet and washed with distilled water and ethanol, and dried in a vacuum oven. Afterward, copper(II) acetate aqueous solution (5% wt) was added drop by drop to a dispersed aqueous mixture of CTSN/Fe3O4, and the mixture were ultrasonicated into an US bath (with frequency: 50 kHz and power density: 200 W L−1) for 1 h, at 80 °C. Finally, the CTSN/Fe3O4–Cu nanocomposite was magnetically separated and washed with deionized water and ethanol and dried at room temperature (Scheme 1).
 |
| Scheme 1 Preparation route of CTSN/Fe3O4–Cu nanocomposite. | |
3.2. Characterization of CTSN/Fe3O4–Cu nanocatalyst
After preparation of the nanocomposite, various conventional instrumental techniques were used for the characterization of the structure and morphology. The comparative FT-IR spectra of the synthesized CTSN/Fe3O4–Cu nanocomposite and its individual components are shown in Fig. 1. In the spectrum of CTSN, The absorption band at 3443 cm−1 is related to the vibrational stretching of the N–H and O–H groups. The absorption band that is appeared at 2922 cm−1 coming from the stretching vibrations of aliphatic C–H groups, and the appeared peak at 1155 cm−1 origins from the stretching vibrations of C–O–C bond. The absorption band at 1602 cm−1 indicates the presence of N–H of amide groups. The absorption bands appeared at 1423 and 1384 cm−1 refer to C–N vibrations. The absorption bands at ca. 598 cm−1 can be seen in the spectrum of Fe3O4 NPs, corresponds to the stretching vibrations of Fe–O band. The intensive broad absorption at 3425 cm−1 represented the stretching mode of H2O molecules and –OH groups. In the spectrum of CTSN/Fe3O4–Cu nanocomposite, absorption band at 3431 cm−1 refers to the stretching vibrations of O–H and N–H groups of chitosan that bonded to the copper ferrite nano particles and shift to lower frequency. It also refers to O–H groups of nanoparticles. The absorption band at ca. 1059 cm−1 shows the stretch vibrations of C–O bond and the 598 cm−1 band represented the Fe–O group of Fe3O4 NPs. Therefore, the FT-IR analysis confirmed the supporting of Fe3O4 and Cu NPs on the surface and matrix of chitosan. EDX analysis was also performed for determination of the elements constitutes catalyst.24 EDX spectra shows that there are C, Fe, Cu and O atoms in the structure of the fabricated nanocomposite. The EDX spectrum of the fabricated CTSN/Fe3O4–Cu nanocomposite and also the quantitative results have been exhibited in Fig. 2(c).
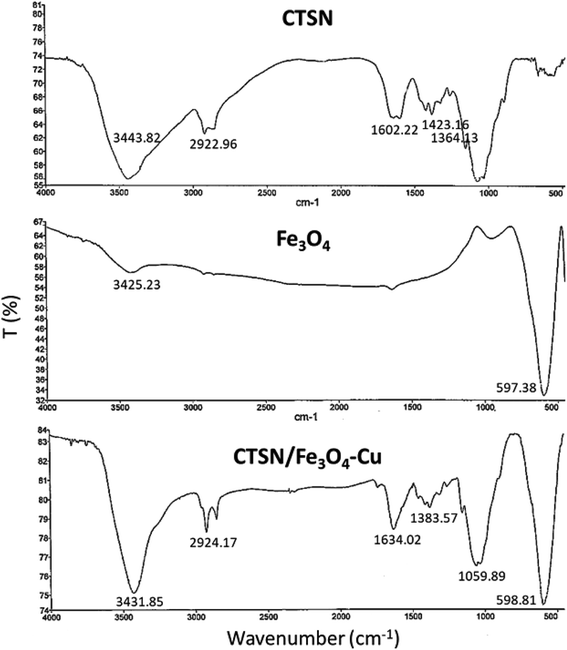 |
| Fig. 1 FT-IR spectra of CTSN, Fe3O4, and CTSN/Fe3O4–Cu nanocomposite. | |
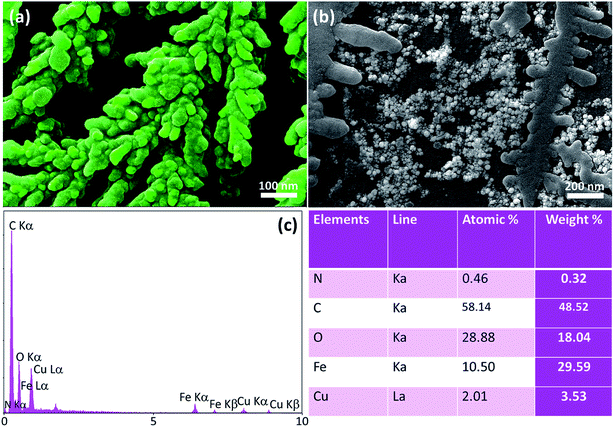 |
| Fig. 2 (a) FE-SEM image of CTSN/Fe3O4, and (b) the prepared CTSN/Fe3O4–Cu nanocomposite. (c) EDX analysis results of the prepared CTSN/Fe3O4–Cu nanocomposite [Y-axis: count, X-axis: energy (keV)]. | |
Field-emission scanning electron microscopy (FE-SEM) images were used to investigate shape and size of the prepared nanocomposite (Fig. 2). As seen in Fig. 2(a), suitable distribution of the spherical Fe3O4 NPs on the chitosan surfaces has formed a cluster-shaped nanocomposite. This morphology provides more extreme active areas for catalytic applications. Moreover, the size of the spherical Fe3O4 NPs is in a range of 40–60 nm diameter. Most likely, these regular cluster-shaped structures are produced via the in situ co-deposition process (described in preparation section). Then, the uniform spherical Cu NPs have been incorporated into the CTSN polymeric network, as can be observed in Fig. 2(b). Overall, this is clearly seen that an extreme catalytic active area is provided by the nanosized CTSN/Fe3O4–Cu nanocomposite.
The XRD pattern of the CTSN/Fe3O4–Cu nanocomposite has been demonstrated in Fig. 3, and the average size of the particles is calculated by the Scherrer equation; D = kλ/β
cos
θ. According to the figure, the resulted diffraction pattern has been precisely compared with the reference patterns of the Fe3O4 and CuO NPs and it was strongly confirmed that they have been thoroughly produced and distributed onto the textures of CTSN matrix. Accordingly, the peaks at the dispersion angles 2θ = 19.11°, 31.64°, 33.91°, 36.56°, 38.42°, 45.15°, 58.65°, 63.25°, 64.25° and 78.65°, which have been marked with miller indices, are attributed to the formed Fe3O4 and CuO NPs. There are strong correlations between the obtained XRD pattern and the reported references.13,25
 |
| Fig. 3 XRD pattern of the fabricated CTSN/Fe3O4–Cu nanocomposite. | |
Thermal resistance of the fabricated CTSN/Fe3O4–Cu nanocomposite has also been studied by thermogravimetric analysis (TGA). As shown in Fig. 4(a), initially a partial increase in the weight was observed that is attributed to the physical adsorption of the moisture of the air onto the hot surfaces of NPs. Then, the weight percentage was decreased to ca. 98% due to removal of the water molecules. In continue, by increasing the temperature to around 420 °C, the first important shoulder was appeared that coming from the weight loss of CTSN. According to the literature, decomposition of the CTSN is started above 420 °C.26 Further, the second shoulder is observed at 620 °C, which is ascribed to the decomposition of the NPs. Totally, from the TGA, this is well proven that the degradation and decomposition of CTSN polysaccharide is greatly inhibited by the composited Cu and Fe3O4 NPs. Magnetic property of the fabricated CTSN/Fe3O4–Cu nanocomposite has also been investigated by vibrating-sample magnetometer (VSM) analysis and related spectra are shown in Fig. 4(b). As it can be seen in the spectra, the magnetic feature of the neat Fe3O4 NPs is reduced proportional to more core coating by various layers. Graph (i) that belongs to the neat Fe3O4 NPs exhibits a typical super-paramagnetic behavior (55 emu g−1) through applying the magnetic field, as presented in the figure. This property was ∼20 emu g−1 decreased through subsequent coating by CTSN (graph ii). However, this is clearly seen that super-paramagnetic behavior of the produced CTSN/Fe3O4–Cu nanocomposite is adequate for easily separation of the catalyst from the reaction mixture.
 |
| Fig. 4 (a) TGA of the fabricated CTSN/Fe3O4–Cu nanocomposite, and (b) VSM analysis of (i) Fe3O4 NPs and (ii) CTSN/Fe3O4–Cu nanocomposite. | |
3.3. Catalytic activity of CTSN/Fe3O4–Cu nanocatalyst
3.3.1. Optimization of the catalyzed-reaction conditions & catalytic performance screening. In order to obtain the optimum conditions for the synthesis reactions by novel prepared heterogeneous CTSN/Fe3O4–Cu nanocatalyst, control reaction was carried out using imidazole 1 (2.0 mmol), bromobenzene 2 (1.0 mmol), cetyltrimethylammonium bromide (CTAB, 0.5 mmol), and different amounts of CTSN/Fe3O4–Cu in various conditions (Scheme 2 and Table 1). Accordingly, the same reaction conditions were applied for all of the control reactions. Different amounts of the magnetic nanocatalyst, different reaction times, and the synergistic effect between the magnetic nanocomposite and USW were precisely investigated. As reported in Table 1, the best result was observed when 0.01 g of CTSN/Fe3O4–Cu was used in deionized water, under 10 min ultrasonication (50 kHz frequency and 200 W power density), at room temperature. The reaction progress was screened using thin-layer chromatography (TLC). After completion of the reaction, the heterogeneous nanocatalyst were conveniently collected using an external magnet, then resulted aryl imidazole was immediately separated and purified via flash column chromatography and identified with 1H-NMR and 13C-NMR (see the ESI section†). However, in order to evaluate the scope and generality of this approach, the optimized reaction condition was applied for the synthesis reactions of the diverse aryl imidazole derivatives, as summarized in Table 2. The N-arylation of imidazoles with aryl iodides and aryl bromides bearing both electron-donating and electron-withdrawing groups was done effectively and gave good yields. As same as excellent results were obtained in gram scale of the reaction by starting these ratio of raw materials and reagents.
 |
| Scheme 2 Schematic of the synthesis of aryl imidazole derivatives by CTSN/Fe3O4–Cu nanocatalyst, in US bath. | |
Table 1 Optimization of the reaction conditions of the synthesis of phenyl imidazole as the pilot experiment under USW irradiation
Entry |
Solvent |
Cat. (g) |
Temp. (°C) |
Time (min) |
Yielda (%) |
Isolated yield for product 3a, via coupling reaction between bromobenzene (1.0 mmol) and imidazole (2.0 mmol), CTAB (0.5 mmol), in the presence of K2CO3 (1.5 mmol), and the solvent (4.0 mL), in the USW bath (50 kHz, 200 W). Optimum conditions. |
1 |
DMF |
0.01 |
r.t. |
10 |
88 |
2 |
DCM |
0.01 |
r.t. |
10 |
85 |
3 |
Water |
0.01 |
r.t. |
10 |
76 |
4 |
EtOH |
0.01 |
r.t. |
10 |
96 |
5 |
MeOH |
0.01 |
r.t. |
10 |
91 |
6 |
Water |
0.01 |
r.t. |
10 |
98b |
7 |
Water/EtOH |
0.05 |
r.t. |
10 |
98 |
8 |
Water/EtOH |
0.01 |
50 |
10 |
97 |
9 |
Water/EtOH |
0.01 |
80 |
10 |
97 |
10 |
Water/EtOH |
0.01 |
r.t. |
30 |
98 |
Table 2 Synthesis of various aryl imidazole derivatives using CTSN/Fe3O4–Cu nanocatalyst
Entry |
R–Ar–X |
Product structure |
Product no. |
Yielda (%) |
R |
X |
Isolated yield. |
1 |
H |
Br |
 |
3a |
98 |
2 |
Me |
Br |
 |
3b |
92 |
3 |
Me |
I |
 |
3c |
90 |
4 |
OMe |
I |
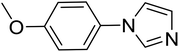 |
3d |
85 |
5 |
OH |
Br |
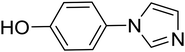 |
3e |
82 |
6 |
Cl |
I |
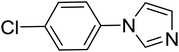 |
3f |
93 |
7 |
COMe |
Br |
 |
3g |
92 |
8 |
CN |
I |
 |
3h |
98 |
9 |
NO2 |
Br |
 |
3i |
96 |
10 |
NO2 |
I |
 |
3j |
97 |
3.3.2. Catalyst reusability. Another important advantage of this active, non-toxic, and environmentally-benign heterogeneous catalyst is its well recyclability. In this regard, the nanocatalyst performance was carefully monitored in the model reaction (product 3a) after eight times recycling. For this purpose, after completion of the reaction, the CTSN/Fe3O4–Cu nanocomposite were magnetically isolated from the mixture and washed with ethanol, then dried and reused in subsequent reactions. It is observed that the nanocatalyst can be reused at least for eight successive times without any significant reduction in coupling reaction yield (Fig. 5). The observed reduction in catalytic activity probably origins from the separation of Cu NPs from the total structure of the nanocatalyst and deformation of the cluster-shaped structure of CTSN/Fe3O4–Cu nanocomposite during the catalytic process. These claims have been proven by SEM imaging and EDX spectroscopy of the recovered nanocatalyst after eight times usages (Fig. S5 and S6, in the ESI section†).
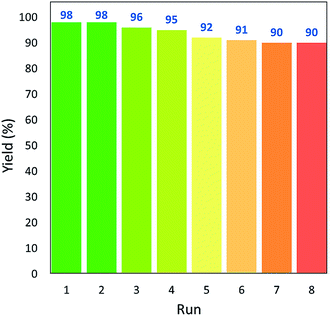 |
| Fig. 5 Reusability diagram of CTSN/Fe3O4–Cu nanocomposite. | |
3.4. Suggested mechanism
A plausible mechanism for this catalytic reaction has been depicted in Fig. 6. As can be seen, at the first stage, an oxidative addition is occurred through which Cu inserts into the Ar–X bond. Then, the resulted complex reacts with imidazole that was activated by potassium carbonate, at stage 2. In the following step (stage 3), a reductive elimination is occurred and the desirable product is obtained.27
 |
| Fig. 6 The plausible mechanism for N-arylation of imidazole derivatives assisted by CTSN/Fe3O4–Cu nanocomposite. | |
4. Conclusion
In summary, a novel catalytic system constructed of chitosan, iron oxide NPs, and copper NPs has been designed and presented as an instrumental tool for facilitating the synthesis of N-arylimidazole derivatives. There are several advantages for applying this nanoscale system. The first and foremost excellence, well execution of synthesis reactions under mild conditions (98% yielded in 10 min at room temperature) through using a partial amount (0.01 g) of this heterogeneous catalytic system can be referred. This high catalytic performance is due to a synergistic catalytic effect between the fabricated nanocomposite (CTSN/Fe3O4–Cu) and the ultrasound waves with 50 kHz frequency and 200 W L−1 power density. In addition, the nanocatalyst is conveniently separated from the reaction mixture and recovered by an external magnetic field and reused efficiently for additional seven times without significant decrease in its catalytic activity. Moreover, there are several advantages for the presented catalytic system such as high heterogeneity, great biocompatibility and biodegradability (through applying CTSN as a natural basis), economic advantages, excellent structural stability due to assisting by ultrasound waves, and no need to another chemical additive or applying intense reaction conditions to provide the required driving force. Thus, this catalytic system could be suggested for scaling up and using in industry.
Conflicts of interest
There are no conflicts to declare.
Acknowledgements
The authors gratefully acknowledge the partial support from the Research Council of the Iran University of Science and Technology and Iran National Science Foundation (INSF).
References
- Z. Wang, S. Yao, S. Pan, J. Su, C. Fang, X. Houa and M. Zhan, Synthesis of silver particles stabilized by a bifunctional SiHx–NHy–PMHS oligomer as recyclable nanocatalysts for the catalytic reduction of 4-nitrophenol, RSC Adv., 2019, 9, 31013–31020 RSC.
- R. Eisavi and A. Karimi, CoFe2O4/Cu(OH)2 magnetic nanocomposite: an efficient and reusable heterogeneous catalyst for one-pot synthesis of β-hydroxy-1,4-disubstituted-1,2,3-triazoles from epoxides, RSC Adv., 2019, 9, 29873–29887 RSC.
- P. Bai, S. Wei, X. Loua and L. Xu, An ultrasound-assisted approach to bio-derived nanoporous carbons: disclosing a linear relationship between effective micropores and capacitance, RSC Adv., 2019, 9, 31447–31459 RSC.
- Y. Yang, M. Chen, Y. Wu, P. Wang, Y. Zhao, W. Zhu, Z. Song and X. B. Zhang, Ultrasound assisted one-step synthesis of Au@Pt dendritic nanoparticles with enhanced NIR absorption for photothermal cancer therapy, RSC Adv., 2019, 9, 28541–28547 RSC.
- A. Maleki, H. Movahed, P. Ravaghi and T. Kari, Facile in situ synthesis and characterization of a novel PANI/Fe3O4/Ag nanocomposite and investigation of catalytic applications, RSC Adv., 2016, 6, 98777–98787 RSC.
- A. Maleki, Green oxidation protocol: Selective conversions of alcohols and alkenes to aldehydes, ketones and epoxides by using a new multiwall carbon nanotubebased hybrid nanocatalyst via ultrasound irradiation, Ultrason. Sonochem., 2018, 40, 460–464 CrossRef CAS PubMed.
- R. Taheri-Ledari, J. Rahimi and A. Maleki, Synergistic catalytic effect between ultrasound waves and pyrimidine-2,4-diamine-functionalized magnetic nanoparticles: Applied for synthesis of 1,4-dihydropyridine pharmaceutical derivatives, Ultrason. Sonochem., 2019, 59, 104737 CrossRef PubMed.
- A. H. Liao, Y. J. Lu, C. R. Hung and M. Y. Yang, Efficacy of transdermal magnesium ascorbyl phosphate delivery after ultrasound treatment with microbubbles in gel-type surrounding medium in mice, Mater. Sci. Eng., C, 2016, 61, 591–598 CrossRef CAS PubMed.
- J. Rahimi, R. Taheri-Ledari, M. Niksefat and A. Maleki, Enhanced reduction of nitrobenzene derivatives: Effective strategy executed by Fe3O4/PVA-10% Ag as a versatile hybrid nanocatalyst, Catal. Commun., 2020, 134, 105850 CrossRef.
- A. Maleki, R. Rahimi, S. Maleki and N. Hamidi, Synthesis and characterization of magnetic bromochromate hybrid nanomaterials with triphenylphosphine surface-modified iron oxide nanoparticles and their catalytic application in multicomponent reactions, RSC Adv., 2014, 4, 29765–29771 RSC.
- A. Maleki, R. Taheri-Ledari, R. Ghalavand and R. Firouzi-Haji, Palladium-decorated o-phenylenediamine-functionalized Fe3O4/SiO2 magnetic nanoparticles: A promising solid-state catalytic system used for Suzuki–Miyaura coupling reactions, J. Phys. Chem. Solids, 2020, 136, 109200 CrossRef CAS.
- A. Maleki, P. Zand and Z. Mohseni, Fe3O4@PEG-SO3H rod-like morphology along with the spherical nanoparticles: novel green nanocomposite design, preparation, characterization and catalytic application, RSC Adv., 2016, 6, 110928–110934 RSC.
- A. Maleki, R. Taheri-Ledari, J. Rahimi, M. Soroushnejad and Z. Hajizadeh, Facile peptide bond formation: effective interplay between isothiazolone rings and silanol groups at silver/iron oxide nanocomposite surfaces, ACS Omega, 2019, 4, 10629–10639 CrossRef CAS PubMed.
- A. Maleki, M. Niksefat, J. Rahimi and R. Taheri-Ledari, Multicomponent synthesis of pyrano[2,3-d]pyrimidine derivatives via a direct one-pot strategy executed by novel designed copperated Fe3O4@polyvinyl alcohol magnetic nanoparticles, Mater. Today Chem., 2019, 13, 110–120 CrossRef CAS.
- A. Maleki, M. Aghaei, H. R. Hafizi-Atabak and M. Ferdowsi, Ultrasonic treatment of CoFe2O4@B2O3-SiO2 as a new hybrid magnetic composite nanostructure and catalytic application in the synthesis of dihydroquinazolinones, Ultrason. Sonochem., 2017, 37, 260–266 CrossRef CAS PubMed.
- A. Maleki, M. Aghaei and N. Ghamari, Synthesis of benzimidazolo[2,3-b]quinazolinone derivatives via a one-pot multicomponent reaction promoted by chitosan-based composite magnetic nanocatalyst, Chem. Lett., 2015, 44, 259–261 CrossRef CAS.
- A. Maleki, R. Taheri-Ledari and M. Soroushnejad, Surface functionalization of magnetic nanoparticles via palladium-catalyzed Diels–Alder approach, ChemistrySelect, 2018, 3, 13057–13062 CrossRef CAS.
- D. Koseki, E. Aoto, T. Shoji, K. Watanabe, Y. In, Y. Kita and T. Dohi, Efficient N-arylation of azole compounds utilizing selective aryl-transfer TMP-iodonium(III) reagents, Tetrahedron Lett., 2019, 60, 1281–1286 CrossRef CAS.
- J. Ohmori, H. Kubota, M. Shimizu-Sasamata, M. Okada and S. Sakamoto, Novel α-Amino-3-hydroxy-5-methylisoxazole-4-propionate Receptor Antagonists: Synthesis and Structure−Activity Relationships of 6-(1H-Imidazol-1-yl)-7-nitro-2,3(1H,4H)-pyrido[2,3-b]pyrazinedione and Related Compounds, J. Med. Chem., 1996, 39, 1331–1338 CrossRef CAS PubMed.
- P. Cozzi, G. Carganico, D. Fusar, M. Grossoni, M. Menichincheri, V. Pinciroli, R. Tonani, F. Vaghi and P. Salvati, Imidazol-1-yl and pyridin-3-yl derivatives of 4-phenyl-1,4-dihydropyridines combining Ca2+ antagonism and thromboxane A2 synthase inhibition, J. Med. Chem., 1993, 36, 2964–2972 CrossRef CAS PubMed.
- S. U. Son, I. K. Park, J. Park and T. Hyeon, Synthesis of Cu2O coated Cu nanoparticles and their successful applications to Ullmann-type amination coupling reactions of aryl chlorides, Chem. Commun., 2004, 7, 778–779 RSC.
- B. M. Choudary, C. Sridhar, M. L. Kantam, G. T. Venkanna and B. Sreedhar, Design and evolution of copper apatite catalysts for N-Arylation of heterocycles with chloro- and fluoroarenes, J. Am. Chem. Soc., 2005, 127, 9948–9949 CrossRef CAS PubMed.
- R. Taheri-Ledari, A. Maleki, E. Zolfaghari, M. Radmanesh, H. Rabbani, A. Salimi and R. Fazel, High-performance sono/nano-catalytic system: Fe3O4@ Pd/CaCO3-DTT core/shell nanostructures, a suitable alternative for traditional reducing agents for antibodies, Ultrason. Sonochem., 2020, 61, 104824 CrossRef CAS PubMed.
- A. Maleki, R. Taheri-Ledari, R. Eivazzadeh-Keihan, M. de la Guardia and A. Mokhtarzadeh, Preparation of Carbon-14 Labeled 2-(2-mercaptoacetamido)-3-phenylpropanoic Acid as Metallo-beta-lactamases Inhibitor (MBLI), for Coadministration with Beta-lactam Antibiotics, Curr. Org. Synth., 2019, 16, 765–771 CrossRef CAS.
- S. Suresh, S. Karthikeyan and K. Jayamoorthy, FTIR and multivariate analysis to study the effect of bulk and nano copper oxide on peanut plant leaves, Journal of Science: Advanced Materials and Devices, 2016, 1, 343–350 Search PubMed.
- G. Cardenas and S. P. Miranda, FTIR and TGA studies of chitosan composite films, J. Chil. Chem. Soc., 2004, 49, 291–295 CAS.
- A. R. Sardarian, N. Zohourian-Mashmoul and M. Esmaeilpour, Salen complex of Cu(II) supported on superparamagnetic Fe3O4@SiO2 nanoparticles: an efficient and magnetically recoverable catalyst for N-arylation of imidazole with aryl halides, Monatsh. Chem., 2018, 149, 1101–1109 CrossRef CAS.
Footnote |
† Electronic supplementary information (ESI) available: The ESI file includes the NMR (H and C) of selected N-aryimidazole products and also SEM and EDX results of recovered nanocatalyst. See DOI: 10.1039/c9ra08062g |
|
This journal is © The Royal Society of Chemistry 2019 |
Click here to see how this site uses Cookies. View our privacy policy here.