DOI:
10.1039/C9RA08555F
(Paper)
RSC Adv., 2019,
9, 41431-41437
Near-infrared turn-on fluorescent probe for discriminative detection of Cys and application in in vivo imaging†
Received
19th October 2019
, Accepted 7th December 2019
First published on 16th December 2019
Abstract
Near-infrared (NIR) fluorescent probes are widely employed in biological detection because of their lower damage to biological samples, low background interference, and high signal-to-noise ratio. Herein, a highly water-soluble NIR probe (NIRHA) based on a hemicyanine skeleton and bearing an acrylate moiety was synthesized. The probe showed high selectivity toward cysteine (Cys) over homocysteine (Hcy) and glutathione (GSH). The probe also had low cytotoxicity and was successfully applied in HeLa cells and mouse experiments. Results of bioimaging experiments indicated that the probe was effective for visualizing endogenous Cys in vitro and in vivo.
Introduction
Cysteine, glutathione and homocysteine are the most common biological thiols in the body. These sulfur-containing amino acids are essential for maintaining the biological redox balance.1,2 Cys binds to enzymes and proteins in their functional state and is always inextricably linked to various diseases such as neurotoxicity, liver damage, and hair depigmentation, as evidenced from abnormal changes in concentration.3–5 The concentration of sulfur-containing enzymes and proteins can be indirectly monitored by detecting biological thiols in the body; this method has great potential application value of clinical diagnosis.6 Therefore, developing highly accurate, selective, and convenient method for detection of biological thiols in living organisms is important.
In recent years, various methods (including high-performance liquid chromatography,7,8 mass spectrometry,9,10 capillary electrophoresis,11 optical sensing,12–15 and electrochemical methods16) for detecting Cys have been proposed on the basis of the close relationship between endogenous Cys and health of living organisms. However, traditional methods have inevitable disadvantages, such as high detection cost, long detection time, complicated pretreatment, and limited applications in vivo. Recently, fluorescent probes have been widely investigated for detection of Cys due to their high efficiency, rapidity, low cost, and high sensitivity.17–20 A number of sensors cannot distinguish Hcy, Cys, and GSH due to their similar reactivity and structure. Some probes for discriminately detecting Cys have been reported. Strongin et al.21 reported a fluorescent probe with acrylate as recognition group for specific detection of Cys.
Fluorescent probes have gained increasing attention in the biological field; various types of fluorophore-based probes reported in literature include spiropyran,22 fluorescein,23 terthiophene,24 rhodamine,25 and so on.26–28 A large number of these fluorescent probes cannot be applied to investigate deep tissue layers, which have high background interference in cells because of short emission spectrum characteristic.29 However, near-infrared fluorescent probes have been the focus because of their advantages, such as less damage to biological samples, low background interference, and high signal-to-noise ratio.30–32
Several NIR Cys probes have been reported recently.33–38 For example, McCarley's group39 reported dicyanomethylene-4H-pyrans (DCM dyes)-based with attractive photophysical properties NIR probe for detecting of Cys over Hcy, and GSH. Yang's group40 has presented a ratiometric two-photon fluorescent probe for detection Cys based on intramolecular charge transfer (ICT) mediated two-photon-FRET integration mechanism. Other NIR probes based on spirocyclization of benzopyrylium,41 naphthofluorescein42 and cyanine43 were also reported recently. However, some defects still exist in these probes, such as, the organic solvent was largely utilized; low efficiency in the detection process (response time > 30 min); complex synthesis; and scarcely probes were employed in endogenous Cys detection. In this case, a fluorescent probe that has excellent water solubility and NIR emission spectrum that can distinguish detect endogenous Cys must be synthesized.
Herein, a highly water-soluble NIR probe (NIRHA) based on a hemicyanine skeleton and bearing an acrylate moiety was designed and synthesized. The probe exhibits excellent selectivity toward Cys, and the sensor mechanism is shown in Scheme 1. Cys can selectivity recognize the acrylate moiety and release NIR fluorescence signal. The sensor mechanism was verified by direct mass spectroscopy. NIRHA can be applied in almost all aqueous solutions because sulfonate in the probe has excellent water solubility. Probe proved rapid (15 min) for determination of Cys. NIRHA was successfully applied in cells and in vivo due to its advantage of low cytotoxicity. In addition, NIRHA was successfully applied to the detection of endogenous Cys in vivo.
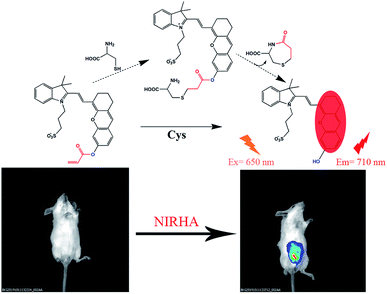 |
| Scheme 1 Reaction between probe NIRHA and Cys. | |
Experimental section
Synthesis
As shown in Fig. 1, the probe NIRHA synthesis route is as follows.
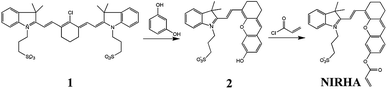 |
| Fig. 1 Synthesis of the NIRHA probe. | |
Synthesis of compound 2
Resorcinol (0.44 g, 4 mmol) and K2CO3 (0.552 g, 4 mmol) were placed in a 100 ml round bottom flask with 20 ml methanol under a nitrogen atmosphere, and the mixture was stirred at room temperature for 20 min. Compound 1 (ref. 44) (1.396 g, 2 mmol) was dissolved in 15 ml of methanol and slowly added dropwise to the above. The reaction was carried out at 50 °C for 4 h, and the solvent was removed under reduced pressure. Crude product was purified by column chromatography eluted with dichloromethane/methanol (10/1, v/v) and a pure blue-green solid was obtained. Yield (0.63 g, 64.1%). 1H NMR (400 MHz, DMSO) δ 8.55 (dd, J = 14.2, 1.4 Hz, 1H), 7.73 (dd, J = 7.0, 4.6 Hz, 2H), 7.46 (dq, J = 15.8, 7.3 Hz, 4H), 6.90 (s, 1H), 6.83 (dd, J = 8.4, 1.8 Hz, 1H), 6.74 (dd, J = 14.4, 1.9 Hz, 1H), 5.03 (s, 1H), 4.59–4.47 (m, 2H), 2.69 (s, 4H), 2.60 (s, 2H), 2.12–2.03 (m, 2H), 1.80 (d, J = 4.3 Hz, 2H), 1.74 (s, 6H). ESI-MS (m/z): [M + H]+ calcd for [C28H30NO5S]+, 492.1820; found, 492.1839.
Synthesis of NIRHA
Compound 2 (491 mg, 1 mmol) was dissolved in a 50 ml dry round bottom flask with 20 ml absolutes dichloromethane. Then, triethylamine (280 μL, 2 mmol) was slowly added to the above solution under ice water bath and stir for 10 min. Subsequently, acryloyl chloride (160 μL, 2 mmol) diluted with dichloromethane was added dropwise to the system, and reacted for 5 h at room temperature. Quenching reaction, the solvent was removed under reduced pressure and the crude mixture was purified by column chromatography (eluted with dichloromethane/methanol = 15
:
1) on silica gel. Get a blue-green solid NIRHA. Yield (435 mg, 79.7%). 1H NMR (400 MHz, MeOD) δ 8.80 (d, J = 15.0 Hz, 1H), 7.71 (d, J = 7.9 Hz, 1H), 7.67 (d, J = 6.7 Hz, 1H), 7.53 (dd, J = 14.6, 6.3 Hz, 2H), 7.34 (d, J = 2.0 Hz, 1H), 7.31 (s, 1H), 7.12 (dd, J = 8.4, 2.2 Hz, 1H), 6.85 (d, J = 15.0 Hz, 1H), 6.64 (dd, J = 17.3, 1.2 Hz, 1H), 6.42 (dd, J = 17.3, 10.4 Hz, 1H), 6.15 (dd, J = 10.5, 1.2 Hz, 1H), 6.12–6.02 (m, 1H), 4.67–4.62 (m, 2H), 3.02–2.98 (m, 2H), 2.79 (s, 4H), 2.35–2.29 (m, 2H), 1.98–1.92 (m, 2H), 1.83 (s, 6H). 13C NMR (101 MHz, MeOD) δ 164.74, 160.77, 153.81, 153.53, 147.51, 143.30, 141.97, 133.61, 131.81, 130.87, 129.74, 128.82, 128.34, 127.94, 123.17, 120.58, 119.56, 116.08, 113.82, 110.00, 106.44, 51.77, 49.00, 48.79, 48.57, 48.36, 48.15, 47.94, 47.72, 47.28, 44.58, 29.73, 27.54, 24.48, 24.07, 20.87. ESI-MS (m/z): [M + H]+ calcd for [C31H32NO6S]+, 546.1950; found, 546.1945.
Materials and instrumentation
The chemicals and solvents were sourced from purchase and were used directly unless otherwise stated. The solvents were dried according to standard procedures, aqueous solutions were prepared using deionized water in the test. 1H NMR and 13C NMR spectra were recorded on a Bruker AV-400 MHz spectrometer, and HR-MS spectra were measured using an Agilent 1290-micrOTOF Q II instrument in the ESI mode. Fluorescence measurement was performed on a Hitachi F-4500 spectrometer with a quartz cell (1 cm × 1 cm) and UV-vis absorption spectra were obtained on a Hitachi U-3010©. Fluorescence microscopy (Carl Zeiss, Axio Observer A1) was used to image the cells. Column chromatography was performed by using silica gel (200–300 mesh, QingdaoHaiyang Chemical Co.) and silica gel 60F254 plates were used as the solid phases for thin-layer chromatography (TLC), TLC plates were viewed with UV light. All tests were performed at 298.0 ± 0.2 K.
Sample preparation and measurements
Stock solutions of probe NIRHA were prepared in DMF with a concentration of 1 × 10−3 M and stored in the refrigerator for later. Then, measure out 40 μL stock solutions and dilute with HEPES buffer solution (10 mM, pH = 7.4) during the test. The concentration of probe was 1 × 10−5 M for all fluorescence emission and UV-vis absorption experiment. During the Cys detection, excitation wavelength was 650 nm, excitation slit/emission width was set to 5 nm/5 nm. All other interfering substance (Gly, Ala, Asn, Asp, Gln, Arg, Glu, Phe, Trp, Tyr, Lys, Ser, Hcy, GSH, Thr, Val, Pro, Gly, Met, H2S, PhSH, Na2SO3 and K2S2) employed in the interference and competition experiments were prepared as 1 × 10−2 M in aqueous solution and stored in the refrigerator.
Cell cytotoxicity evaluated by MTT assay
Standard MTT (3-(4,5-dimethyl-2-thiazolyl)-2,5-diphenyl-tetrazolium bromide) assay was applied in cytotoxicity of NIRHA in HeLa cells (1 × 104 cells per well). Cells were seeded in a 96-well cell culture cluster and incubated at 37 °C containing 5% CO2 for 24 h in a humidified incubator. Cells were treated with various concentration of NIRHA (0, 5, 10, 15, 20, 25 and 30 μM) for 4, 6, 12 and 24 h (37 °C, 5% CO2). Then cells were washed thrice with HEPES (10 nM, pH = 7.4). MTT solution (5 mg mL−1) prepared in HEPES was added to each well and incubator (37 °C, 5% CO2) for another 4 h. Excess MTT was carefully removed and then DMSO (150 μL) was added to dissolve the purple formazan crystals. The optical density was taken by ELx800 universal microplate reader (BIO-TAK). Cell viability rate was calculated according to the equation:
Cell viability (%) = OD490 (sample)/OD490 (control) × 100% |
Cell culture and cell imaging
HeLa cells were prepared in 24 well cell culture plate with circle microscope cover glasses (2 × 105 cells per well) in Dulbecco's modified Eagle medium (DMEM) with 10% fetal bovine serum (FBS) and treated with 5% CO2 at 37 °C in a humidity incubator for 24 h. A total of four control experiments were performed: only cells; the free probe; cells incubated with N-ethylmaleimide (5 mM, NEM) for 30 min; cells incubated with NEM for 30 min and treated with Cys. Parts of cells were pretreated with 5 mM NEM for 30 min in HEPES buffer at 37 °C for 3 h. Then HeLa cells was washed three times with HEPES buffer in order to remove the residual NEM. Next, HeLa cells were incubated with 10 μM probe for 30 min and washed with HEPES for thrice. The fluorescent image was recorded by the excitation at 633 nm and observed by laser confocal microscopy (LSM710).
Living animal imaging
All animal experiments were performed in accordance with the Regulations for the Administration of Affairs Concerning Experimental Animals of the People's Republic of China and approved by the Animal Ethics Committee of Jilin University. Naked mice (weight 18–20 g) were employed in vivo experiment. Two sets of experiments were conducted. As a control experiment, in the first group, free probe NIRHA (50 μL, 10 μM) was injected into the peritoneal cavity of mice and detected immediately. Furthermore, in the second group of experiment, the NIRHA (50 μL, 10 μM) was injected into the peritoneal cavity of mice. After the mice were gas anaesthesia at 20 min and 40 min, fluorescence distributions images were obtained by a small animal living imaging system (λex = 640 nm, λem = 700–720 nm).
Results and discussion
Naked eye identification and UV-vis absorption spectrum
Prior to spectroscopy experiment, the response of the probe toward GSH, Cys, and Hcy was compared because of the molecular structure similarity of these amino and their constant mutual interference during detection. A simple colorimetric experiment indicated color change only after adding Cys to the probe solution. Strong fluorescence intensity enhancement was observed by a fluorescence spectrometer. Scarce fluorescence change was observed after adding GSH and Hcy to the solution under the same test conditions.
The effect of Cys on the absorption spectra was investigated in aqueous solution (HEPES/DMF, v/v = 99/1, 10 nM, pH = 7.4). In the absorption titration, the color of the solution changed from blue to light green upon the addition of Cys. In addition, the absorption peak was gradually decreased at 585 nm, and a new absorption peak arose at 680 nm with a distinct isosbestic point at 615 nm (Fig. 2), indicated that the nucleophilic attack occurred between Cys and NIRHA. The absorption spectrum exhibits excellent linear relationship to the concentration of Cys.
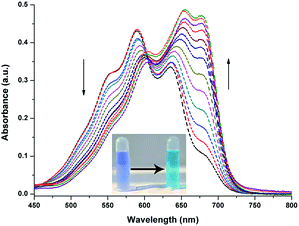 |
| Fig. 2 UV-vis absorption spectra of NIRHA (10 μM) for Cys in HEPES/DMF = 99/1 (10 nM, pH = 7.4). Inset: colorimetric responses of probe to Cys in naked eyes. | |
Fluorescence titration of probe NIRHA
In the following experiments, fluorescence titration of NIRHA was emphasized. As shown in Fig. 3, the fluorescence signal scarcely changed under the excitation wavelength at 650 nm. Upon addition of increasing amount of Cys, an escalation emission peak appeared at 710 nm. The fluorescence signal remained stable until the concentration of the analyte reached 4.8 eq. This finding implied that the acrylate of the probe was attacked by Cys and the original hydroxyl group was released. The regression equation was y = 0.77482 + 0.5411x based on the fluorescence titration data, and a good linear relationship (R2 = 0.99628) was observed with increasing Cys concentration. In addition, the detection limit was calculated as 7.76 × 10−8 M based on 3σ/k, which is better than recently reported probes (Table S1†).
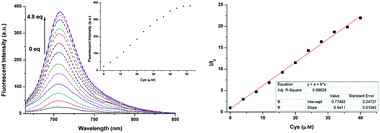 |
| Fig. 3 Fluorescence titration of NIRHA (10 μM) for Cys in HEPES/DMF = 99/1 (10 nM, pH = 7.4). Inset: the fluorescence intensity increased upon gradual addition of Cys at 710 nm (left). Fluorescence intensity ratio (I/I0) of NIRHA varies almost linearly vs. the concentration of Cys in the range of 0–40.0 μM (right). | |
Reaction time and pH effect
An excellent probe should exhibit quick response. Several Cys probes are limited by response time and are difficult to use for real-time monitoring. The probe NIRHA was designed and fully reacted with Cys within 15 min to avoid the problem (Fig. 4a). Therefore, the ability of the probe for real-time monitoring was significantly improved. Next, the reaction time among Cys, Hcy (48 eq.) and GSH (480 eq.) was compared, and no valid signal was observed when Hcy or GSH was added in 30 min. The effect of pH on NIRHA was also investigated. As shown in Fig. 4b, in the absence of Cys, the fluorescence intensity of the probe remained constant under neutral or weakly acidic conditions. By contrast, the fluorescence intensity of the probe was gradually enhanced with increasing solution alkalinity. After the addition of Cys, the fluorescence intensity rapidly enhanced to the maximum in the pH region from 6 to 8. However, in the alkaline environment, the fluorescence signal showed maintained stability with a slight downward trend. pH 7.4 was selected as the detecting condition considering the characteristic of biological thiols.
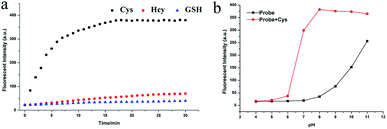 |
| Fig. 4 (a) Time dependent-fluorescence changes with the addition of Cys, Hcy and GSH. (b) Fluorescence intensity of NIRHA with or without Cys in solutions with different pH. | |
Selectivity and interference
Specificity is one of the most important property of fluorescent probes. The selectivity and anti-interference of NIRHA toward Cys over other interfering substance (Gly, Ala, Asn, Asp, Gln, Arg, Glu, Phe, Trp, Tyr, Lys, Ser, Hcy, GSH, Thr, Val, Pro, Gly, Met, H2S, PhSH, Na2SO3 and K2S2) were investigated. As shown in Fig. 5, upon treatment with other interfering substance, scarce fluorescence emission was observed even at 48 eq. Negligible fluorescence response was detected with Hcy and GSH. After the addition of Cys, the fluorescence intensity in the emission at 710 nm showed a large enhancement even when 48 eq. interference was present in the solution. Hence, the probe NIRHA did not react with interfering amino acids and did not affect the detection of Cys in a solution containing other interferences. Therefore, the probe NIRHA can be employed in specific detection of Cys.
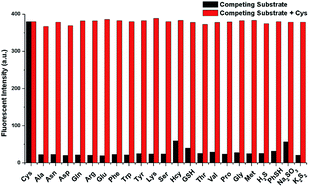 |
| Fig. 5 Fluorescence of NIRHA in the presence of various amino acids: 4.8 eq. Cys, 480 eq. GSH and 48 eq. other interfering substance (black bar). Fluorescence of NIRHA in the presence of 4.8 eq. Cys, 480 eq. GSH and 48 eq. other competitive analytes. (Red bar). | |
Reaction mechanism
The reaction mechanism of the probe NIRHA toward Cys was also investigated. The speculated reaction mechanism is that thiol of Cys attacks the vinyl of acrylate to form an intramolecular seven-membered ring and release the hydroxyl group in the probe. The fluorescent signal is then emitted. However, the cyclization of Hcy forms an intramolecular eight-membered ring in the process. The structure of the seven-membered ring is quite easier than that of the eight-membered ring, as determined by the efficiency of intramolecular cyclization kinetics rate (Fig. 6).
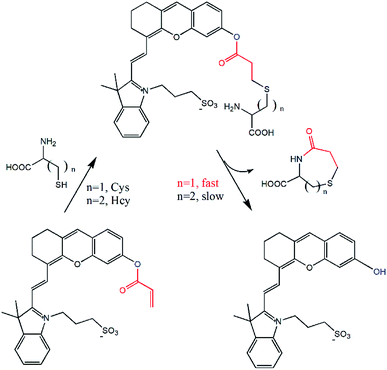 |
| Fig. 6 The proposed Cys sensing mechanism of the NIRHA. | |
The fluorescence signal of compound 2 was measured to confirm our assumption (Fig. S4†). A distinct emission peak appeared at 710 nm upon excitation at 650 nm, which was perfectly matched with the experimental results. In addition, the HR-MS spectra showed that compound 2 (m/z 492.1839) was released after NIRHA reacted with Cys. The seven-membered ring compound (176.0376) was also monitored (Fig. S5 and S6†).
Cell toxicity and fluorescence imaging
The cytotoxicity of NIRHA was first examined by standard MTT assay to verify the application potential of NIRHA in living cells. As shown in Fig. S3,† the cells were incubated with variable concentrations of NIRHA for 24 h. The cell viability was maintained above 90%, indicating that the probe NIRHA had low cytotoxicity and excellent biocompatibility.
The ability of the probe NIRHA to monitor Cys in HeLa cells was evaluated by confocal fluorescence imaging (CLSM). As shown in Fig. 7A, blank cells showed scarce fluorescence change under light excitation. Upon treatment with 10 μM NIRHA, the fluorescence was significantly enhanced in to HeLa cells (Fig. 7B), indicating the direct reaction of endogenous Cys to the probe. The cells were pretreated with NEM added with NIRHA to prove that the reaction between endogenous Cys and the probe increases the fluorescence. No fluorescence signal enhancement was observed (Fig. 7C). Immediately, after the addition of 48 μM Cys, the cells exhibited extraordinary fluorescent signals (Fig. 7D). Therefore, the probe NIRHA was capable of monitoring endogenous and exogenous Cys in living cells.
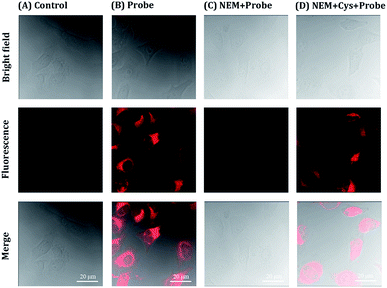 |
| Fig. 7 Confocal microscope images of HeLa cells. (A) Control; (B) HeLa cells incubated with NIRHA (10 μM) for 30 min; (C) HeLa cells pretreated with NEM (5 mM) for 30 min and then incubated with NIRHA (10 μM) for another 30 min; (D) HeLa cells pretreated with NEM (5 mM) for 30 min and treated with Cys (48 μM) for 30 min, then incubated with NIRHA (10 μM) for another 30 min. Scale bar = 20 μm. | |
Application to living animals
The prominent features of NIRHA, such as low detection limit, excellent selectivity, fast response time, low cytotoxicity, and remarkable performance in cell images, inspired us to test its potential application for detecting Cys in living mice. Two experiments were conducted. Imaging was immediately performed after the free probe was injected into the peritoneal cavity of mice as control experiment. As shown in Fig. 8a, the fluorescent emission was not detected. In another set of experiment, the mice injected with the probe for 20 min showed distinct fluorescence. Hence, NIRHA completely reacted with endogenous Cys (Fig. 8b). After the mice were injected with the probe for 40 min (Fig. 8c), the probe was diffused in the abdominal cavity. The fluorescence intensity remained constant.
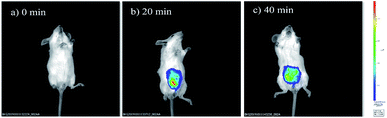 |
| Fig. 8 Fluorescence images of mice injected with NIRHA (50 μL, 10 μM). (a) Recorded at 0 min. (b) Recorded at 20 min. (c) Recorded at 40 min. | |
In addition, a group of animal experiments was also added to further confirm the sensitively of probe to monitor Cys in mice. As shown in Fig. 9a (control group), a strong fluorescent signal was detected after nude mouse injected intraperitoneally with NIRHA (50 μL, 10 μM) in 20 minutes. In another group, the mouse was preinjected with NEM for 30 min and intraperitoneal injection with NIRHA (50 μL, 10 μM), the weakened emission was exhibited in 20 minutes (Fig. 9b). Therefore, the probe successfully interacted with endogenous Cys in vivo and has a large prospect for biological detection.
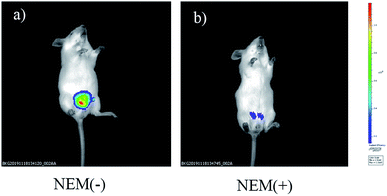 |
| Fig. 9 Fluorescence images of mice injected NIRHA (50 μL, 10 μM) recorded at 20 minutes without NEM (a) or with NEM (b). | |
In vivo studies, all animal procedures were performed in accordance with the Regulations for the Administration of Affairs Concerning Experimental Animals of the People's Republic of China and approved by the Animal Ethics Committee of Jilin University.
Conclusions
A probe based on the hemicyanine skeleton and bearing an acrylate moiety was synthesized and applied to discriminative detection of Cys over other biothiols (Hcy and GSH). In addition, the performance of the probe was not affected by interfering amino acids (Gly, Ala, Asn, Asp, Gln, Arg, Glu, Phe, Trp, Tyr, Lys, Ser, Hcy, GSH, Thr, Val, Pro, Gly, Met, H2S, PhSH, Na2SO3 and K2S2). Only 1% organic solvent was employed in the test, and the limits of detection reached 7.76 × 10−8 M. The reaction mechanism was investigated by HR-MS. Furthermore, the probe exhibited low cytotoxicity and excellent biocompatibility. The cell viability was maintained above 90% after incubation with variable concentrations of NIRHA for 24 h. In HeLa cells and mouse experiments, the probe successfully combined with endogenous and extracorporeal Cys. Hence, the probe has a large application prospect for biological detection.
Conflicts of interest
There are no conflicts to declare.
Acknowledgements
We thank Jilin Province Science and Technology Research Plan (No. 20170204039GX and 20180201085GX), the National Natural Science Foundation of China (No. 21174052), the Natural Science Foundation of Jilin Province of China (No. 20170101105JC), and the Provincial Industrial Innovation Special Fund Project of jilin province (No. 2018C052-5) for their generous financial support.
Notes and references
- Z. A. Wood, E. Schröder, J. Robin Harris and L. B. Poole, Trends Biochem. Sci., 2003, 28, 32–40 CrossRef CAS PubMed.
- T. P. Dalton, H. G. Shertzer and A. Puga, Annu. Rev. Pharmacol. Toxicol., 1999, 39, 67–101 CrossRef CAS PubMed.
- S. Shahrokhian, Anal. Chem., 2001, 73, 5972–5978 CrossRef CAS PubMed.
- Y. Wang, J. Zheng, Z. Zhang, C. Yuan and D. Fu, Colloids Surf., A, 2009, 342, 102–106 CrossRef CAS.
- N. Zhang, F. Qu, H. Q. Luo and N. B. Li, Biosens. Bioelectron., 2013, 42, 214–218 CrossRef CAS PubMed.
- D. M. Townsend, K. D. Tew and H. Tapiero, Biomed. Pharmacother., 2003, 57, 145–155 CrossRef CAS.
- Y. V. Tcherkas and A. D. Denisenko, J. Chromatogr. A, 2001, 913, 309–313 CrossRef CAS PubMed.
- A. R. Ivanov, I. V. Nazimov and L. A. Baratova, J. Chromatogr. A, 2000, 870, 433–442 CrossRef CAS PubMed.
- M. J. MacCoss, N. K. Fukagawa and D. E. Matthews, Anal. Chem., 1999, 71, 4527–4533 CrossRef CAS PubMed.
- A. P. Vellasco, R. Haddad, M. N. Eberlin and N. F. Höehr, Analyst, 2002, 127, 1050–1053 RSC.
- D. Diaz-Diestra, B. Thapa, J. Beltran-Huarac, B. R. Weiner and G. Morell, Biosens. Bioelectron., 2017, 87, 693–700 CrossRef CAS PubMed.
- J. Zhang, J. Wang, J. Liu, L. Ning, X. Zhu, B. Yu, X. Liu, X. Yao and H. Zhang, Anal. Chem., 2015, 87, 4856–4863 CrossRef CAS PubMed.
- H. Zhang, L. Xu, W. Chen, J. Huang, C. Huang, J. Sheng and X. Song, ACS Sens., 2018, 3, 2513–2517 CrossRef CAS PubMed.
- Q. Yang, C. Jia, Q. Chen, W. Du, Y. Wang and Q. Zhang, J. Mater. Chem. B, 2017, 5, 2002–2009 RSC.
- H. Wang, G. Zhou, H. Gai and X. Chen, Chem. Commun., 2012, 48, 8341–8343 RSC.
- P. T. Lee, J. E. Thomson, A. Karina, C. Salter, C. Johnston, S. G. Davies and R. G. Compton, Analyst, 2015, 140, 236–242 RSC.
- Y. Zhou and J. Yoon, Chem. Soc. Rev., 2012, 41, 52–67 RSC.
- H. Wang, G. Zhou and X. Chen, Sens. Actuators, B, 2013, 176, 698–703 CrossRef CAS.
- Y. Li, Z. Zhao, Y. Xiao, X. Wang, X. Jiao, X. Xie, J. Zhang and B. Tang, Anal. Chem., 2019, 91, 6097–6102 CrossRef CAS PubMed.
- H. Maeda, H. Matsuno, M. Ushida, K. Katayama, K. Saeki and N. Itoh, Angew. Chem., Int. Ed., 2005, 44, 2922–2925 CrossRef CAS PubMed.
- X. Yang, Y. Guo and R. M. Strongin, Angew. Chem., Int. Ed., 2011, 50, 10690–10693 CrossRef CAS PubMed.
- Y. Shiraishi, S. Sumiya and T. Hirai, Chem. Commun., 2011, 47, 4953–4955 RSC.
- S. Lim, J. O. Escobedo, M. Lowry, X. Xu and R. Strongin, Chem. Commun., 2010, 46, 5707–5709 RSC.
- Q. Niu, L. Lan, T. Li, Z. Guo, T. Jiang, Z. Zhao, Z. Feng and J. Xi, Sens. Actuators, B, 2018, 276, 13–22 CrossRef CAS.
- H. Li, J. Fan, J. Wang, M. Tian, J. Du, S. Sun, P. Sun and X. Peng, Chem. Commun., 2009, 5904–5906, 10.1039/B907511A.
- S.-R. Liu, C.-Y. Chang and S.-P. Wu, Anal. Chim. Acta, 2014, 849, 64–69 CrossRef CAS PubMed.
- L. Qu, C. Yin, F. Huo, J. Li, J. Chao and Y. Zhang, Sens. Actuators, B, 2014, 195, 246–251 CrossRef CAS.
- Y. Yue, Y. Guo, J. Xu and S. Shao, New J. Chem., 2011, 35, 61–64 RSC.
- H. Shang, H. Chen, Y. Tang, Y. Ma and W. Lin, Biosens. Bioelectron., 2017, 95, 81–86 CrossRef CAS PubMed.
- W. Zhu, X. Huang, Z. Guo, X. Wu, H. Yu and H. Tian, Chem. Commun., 2012, 48, 1784–1786 RSC.
- K. Chen, Q. Shu and M. Schmittel, Chem. Soc. Rev., 2015, 44, 136–160 RSC.
- M. H. Lee, J. S. Kim and J. L. Sessler, Chem. Soc. Rev., 2015, 44, 4185–4191 RSC.
- J. Sun, Z. Hu, S. Zhang and X. Zhang, ACS Sens., 2019, 4, 87–92 CrossRef CAS PubMed.
- Y. Yue, F. Huo, P. Ning, Y. Zhang, J. Chao, X. Meng and C. Yin, J. Am. Chem. Soc., 2017, 139, 3181–3185 CrossRef CAS PubMed.
- C. Han, H. Yang, M. Chen, Q. Su, W. Feng and F. Li, ACS Appl. Mater. Interfaces, 2015, 7, 27968–27975 CrossRef CAS PubMed.
- Y. Li, X. He, Y. Huang, L. Xu, L. Zhao, X. Li, Y. Sun, X. Wang, P. Ma and D. Song, Spectrochim. Acta, Part A, 2020, 226, 117544 CrossRef CAS PubMed.
- H. Hong, L. Shi, J. Huang, C. Peng, S. Yang, G. Shao and S. Gong, New J. Chem., 2019, 43, 72–76 RSC.
- Y.-L. Meng, Z.-H. Xin, Y.-J. Jia, Y.-F. Kang, L.-P. Ge, C.-H. Zhang and M.-Y. Dai, Spectrochim. Acta, Part A, 2018, 202, 301–304 CrossRef CAS PubMed.
- R. R. Nawimanage, B. Prasai, S. U. Hettiarachchi and R. L. McCarley, Anal. Chem., 2017, 89, 6886–6892 CrossRef CAS PubMed.
- S. Yang, C. Guo, Y. Li, J. Guo, J. Xiao, Z. Qing, J. Li and R. Yang, ACS Sens., 2018, 3, 2415–2422 CrossRef CAS PubMed.
- H. Lv, X.-F. Yang, Y. Zhong, Y. Guo, Z. Li and H. Li, Anal. Chem., 2014, 86, 1800–1807 CrossRef CAS PubMed.
- S. Xue, S. Ding, Q. Zhai, H. Zhang and G. Feng, Biosens. Bioelectron., 2015, 68, 316–321 CrossRef CAS PubMed.
- Y.-S. Guan, L.-Y. Niu, Y.-Z. Chen, L.-Z. Wu, C.-H. Tung and Q.-Z. Yang, RSC Adv., 2014, 4, 8360–8364 RSC.
- X. Zou, M. Xu, W. Yuan, Q. Wang, Y. Shi, W. Feng and F. Li, Chem. Commun., 2016, 52, 13389–13392 RSC.
Footnotes |
† Electronic supplementary information (ESI) available. See DOI: 10.1039/c9ra08555f |
‡ These two authors equally contribute to this paper. |
|
This journal is © The Royal Society of Chemistry 2019 |
Click here to see how this site uses Cookies. View our privacy policy here.