DOI:
10.1039/C9RA09265J
(Paper)
RSC Adv., 2019,
9, 42172-42182
Direct intramolecular double cross-dehydrogentive-coupling (CDC) cyclization of N-(2-pyridyl)amidines under metal-free conditions†
Received
8th November 2019
, Accepted 6th December 2019
First published on 19th December 2019
Abstract
A facile transition-metal-free protocol to form 2-iminoimidazo[1, 2-a]-pyridines bearing a –CHBr2 group and an aza-quaternary carbon center at the 3 position from N-(2-pyridyl)amidines substrates, in which the new heterocyclic skeletons constructed from amidines via radical reactions or nucleophilic substitution reactions are promoted only by CBr4 under mild conditions, is demonstrated. The reactions were realized by intramolecular CDC reaction involving C–N and C–C bond formation via the sequential C(sp3)–H bifunctionalization mode on the same carbon atom under mild conditions. Moreover, this work also provides an excellent and representative example for CBr4 as an efficient reagent to initiate radical reactions under initiator-free conditions or to give rise to nucleophilic substitution reactions only by base.
Introduction
C–H functionalization, especially sp3 C–H bond functionalization, to efficiently construct C–X (X = C, N, O, S, etc.) bonds has been one of the most researched topics in the field of organic chemistry because the formation of C–X (X = C, N, O, S, etc) bonds is a fundamental organic reaction.1 Previous reports on C–H functionalization to build new C–X (X = C, N, O, S, etc) bonds required the prefunctionalization of the substrates, which caused unnecessary waste, great costs, and laborious experimental handling. Moreover, some cross-coupling reactions generally needed transition-metal catalysts and special ligands,2 which would also cause heavy metal contamination for underground water and soil. Therefore, it is still highly desirable to further develop new atom- and step-economic, greener approaches to construct C–X (X = C, N, O, S, etc) bonds by direct C–H functionalization. More recently, cross-dehydrogenative-coupling (CDC) reactions,3 especially transition-metal-free CDC reaction, which can introduce a substituent through the direct cleavage of a C–H bond under redox conditions without the introduction of a leaving group,4 have emerged as a valuable tool for this transformation and have also gained significant attention because this strategy presented a non-metallic, environmentally friendly, and concise way compared to other previous available methods. For example, the formation of C–X (X = C, N, O, S, etc) bonds can be achieved by the metal-free CDC reaction under only oxidants such as peroxides,5 quinones6 and hypervalent iodine reagents,7 O2,8 or KOtBu/DMF.9 Though every above-mentioned metal-free CDC protocol has its own advantages, further exploitation of more simple, efficient and metal-free CDC approaches to forge C–X (X = C, N, O, S, etc) bonds using various novel mediators under mild conditions is still the goal pursuit by many scientific workers.
Carbon tetrabromide (CBr4), as a commercially available and cheap reagent, which has been utilized as a organocatalyst or a stoichiometric reagent for a variety of organic transformations, has attracted considerable attention from chemists.10 Some literature surveys showed that CBr4 was used to catalyze the deprotection of trialkylsilyl esters and b-(trimethylsilyl)ethoxymethyl ethers,10b,11 esterifications,10h expoxide ring opening,12 the acetalization of aldehydes,13 the Friedel–Crafts alkylation indoles with carbonyl compounds,14 the carboxylation of indoles with CBr4/MeOH,15 and esterification of methyl aromatic,16 etc. Furthermore, most importantly, it has been found that CBr4 also played an extremely important role in the formation of C–X (X = C, N, O, S, etc) bonds in the field of cross-dehydrogenation coupling (CDC) reactions to construct the physiological and biological active compounds via C–H functionalization under metal-free conditions.17 For example, Huang's group developed an efficient and facile CBr4-mediated CDC reaction to form the C–O bond and C–S bond under metal-free conditions.17a,b Equally, the Huo and other groups have also demonstrated a series of the CBr4-promoted CDC and DOD reactions via C–C bond and C–N bond formation to construct successfully complex heterocyclic compounds such as imidazo[1, 2-a]pyridines, imidazo[1, 2-a]pyrimidines and imidazoles.17c–k The advancements of these reactions clearly showed that CBr4 had great potential in organic synthesis. Therefore, it is an urgent mission for organic chemists to further develop its greater potential in organic chemistry at present.
As an important class of organic synthons, amidines have been frequently applied in the synthesis of various heterocyclic ring systems such as quinazolines,18 quinazolinones,19 pyrimidines,20 triazoles,21 and benzimidazoles.22 Especially importantly, N-(2-pyridyl) amidines, as one of the most important N-aryl amidines, have also been employed for the formation of different biological active compounds bearing nitrogen-containing heterocyclic skeletons, including 1, 2, 4-triazoles and imidazo[1, 2-a]-pyridines, by the direct C–H functionalization for N–N and C–N bond formation in the presence of a catalyst and oxidant. For example, when a large variety of oxidants were used, including air,23a,g PIFA (phenyliodinebis(trifluoroacetate)),23b NaClO,23c Pb(OAc)4,23e,g and I2,23f 1,2,4-triazoles with various substituents can be afforded by intramolecular oxidative N–N bond formation from N-(2-pyridyl)amidines substrates (Scheme 1a). Significantly, in 2016, Chang's group24 reported one example for the synthesis of 2-aminoimidazo[1, 2-a]-pyridines from N-(2-pyridyl)amidines via intramolecular oxidative C–N bond formation using I2/KI as reagent (Scheme 1b), in which the bonding mode of reaction was completely different from a previously reported one by Chang and co-workers23g even under the same reaction conditions. These reports, as a consequence, indicated clearly that the results of the reaction could be greatly affected by the structure of the substrates and the reaction conditions. Although great progress has already been made in this area, there is still an urgent requirement to develop highly efficient and environmentally benign synthetic methods to construct the diverse core framework in structure via oxidative CDC strategies on account of the increasing demands of structural novelty and diversity in both biomedical research and drug discovery. Accordingly, in view of our continuous interest in amidines and ketenimines,25 we herein report a transition-metal-free oxidative CDC cyclization reaction of N-(2-pyridyl)amidines via the sequential dual C–H functionalization of the C(sp3)–H bond on the same carbon atom involving C–N and C–C bond formation, in which the new heterocyclic skeletons constructed from amidines via radical reactions or nucleophilic substitution reactions are promoted only by CBr4 under mild conditions.
 |
| Scheme 1 Previous works on the oxidative cyclization of N-(2-pyridyl)amidines and this work. | |
Results and discussion
The desire to create novel and diverse compounds in structure continues to activate us to investigate the reaction process of amidines by using CBr4. Firstly, the oxidative cyclic conditions for the CDC strategy were optimized. To our delight, the reaction of N-(2-pyridyl)amidine (1a) and CBr4 (2) was carried out at room temperature for 10 h in the presence of K2CO3 under N2, giving the corresponding imidazo[1, 2-a]-pyridine core-like product in 25% yield (Table 1, entry 1). 3a had been confirmed by 1H NMR, 13C NMR, and HRMS. Morever, we also observed that when the reaction temperature was elevated to 60 °C, the yield of 3a was also increased to 37% accordingly (Table 1, entry 2). To further increase the yield of 3a, the reactions were performed under different bases. As shown in Table 1 (Table 1, entries 2–7), it was found that K2CO3 turned out to be the best base in improving the yield of CDC reaction (entry 2, 37%). However, when TEA and DBU as bases were employed, two reactions furnished 3a only in trace amounts (entries 4 and 7). We, subsequently, attempted to perform the reaction in various commonly used solvents. It was shown that, in contrast to DCM, the use of other solvents was found to be less effective (Table 1, entry 2 and entries 8–12). To render further improve the yield of the transformation, a change in the amount of reaction substrates was also investigated. The results indicated that the molar ratio of 1a
:
2 was enhanced to 1
:
1.2, the yield of reaction products was the best (Table 1, entries 13–19). However, it could be found that if the amount of CBr4 was further increased, the yield of the product 3a would decrease (Table 1, entry 13, 31% yield). In addition, it was noteworthy that the longer reaction time and the more suitable higher temperature were required for the formation of 3a (Table 1, entries 16–19). Finally, the optimized reaction conditions were obtained as follows: the CDC reaction system with the ratio of 1
:
1.2 (1a
:
2) was carried out at 100 °C in DCM for 12 h in the presence of K2CO3 under N2.
Table 1 Optimization of the reaction conditions a
Under the optimized conditions given above, the scope and generality of the reaction in regard to different N-(2-pyridyl)amidines (1), which were furnished from the reaction of 2-amino pyridines, terminal alkynes and sulfonyl azides under Cu(I) and base,25,26 were investigated, and the results are summarized in Table 2. All tested amidines reacted smoothly with CBr4 were efficiently transformed into their corresponding products (Table 2, 3a–3ad) with moderate to good yields, reflecting wide scope of this CDC reaction system. The structure of product 3a was also further confirmed by single-crystal X-ray diffraction, as also shown in Table 2. Moreover, it was found that the yields remained relatively stable and only N-(2-pyridyl)amidines derived from terminal alkynes with electron-withdrawing group on phenyl rings afforded slightly lower yields of products than those from other terminal alkynes (Table 2, 3a–3i). Meanwhile, the effect of different N-(2-pyridyl)amidines from sulfonyl azides was surveyed too. The results indicated that the yields of products derived from sulfonyl azides bearing electron-donating group on phenyl rings were obviously higher than those of products without substituents on phenyl rings of sulfonyl azides, for example, 3a and 3x or 3b and 3y.
Table 2 Substrate scopes of CDC reaction system for the formation of 3 a
Optimal reaction conditions: 1 (0.2 mmol), 2 (0.24 mmol), K2CO3 (0.4 mmol) in dry DCM (2 mL), sealed and then stirred at 100 °C for 12 h under N2. Isolated yield of 3 are given, 1 as reference. |
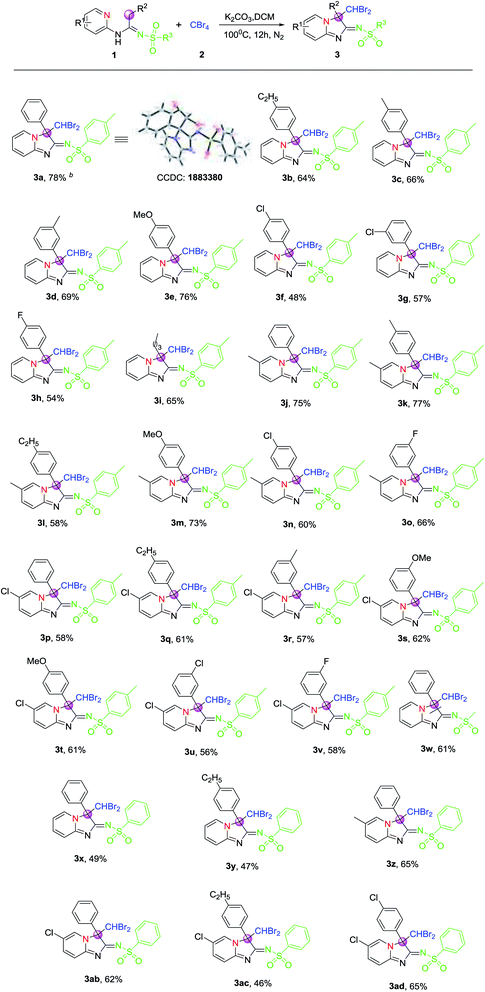 |
From the results of the investigation in Table 2, we found that an appropriate temperature rise for the reaction would contribute to the formation of the products 3. To further gain mechanistic insights into this transformation, a series of control experiments were performed under the envisaged conditions. However, when the reaction was carried out in the dark according to eqn (1) in Scheme 2 under the reaction conditions of entry 1 (Table 1), the yield is equal to that of entry 1. Similarly, the reaction was performed in the dark according to eqn (2) in Scheme 2 under standard reaction conditions, the desired product 3a was also isolated in 76% yield, which was also close to that of the reaction in Table 1 (entry 18, 78%). These results indicated that the visible light was not essential for the successful completion of the reaction. In addition, the reaction of 1a and 2 was conducted in the presence of 1 equiv. of TEMPO as a radical scavenger under the optimized conditions, only the trace amount of 3a was observed. This result suggested that radical processes might be involved in the CDC reaction system.
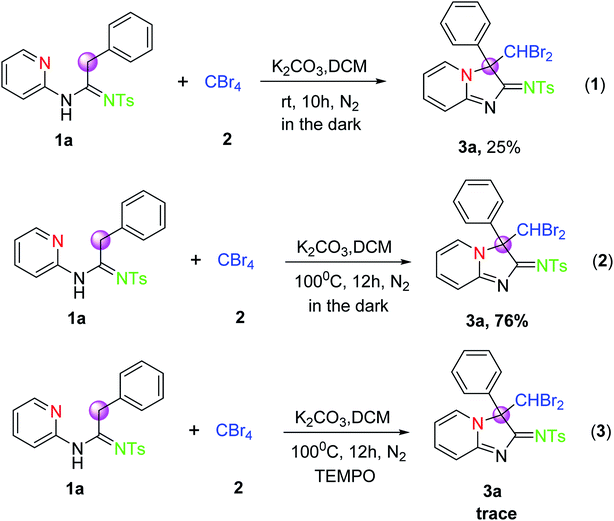 |
| Scheme 2 Control experiments. | |
Based on these facts mentioned above and previous literatures,17 a tentative mechanism for the transformation of 1a and CBr4 into 3a is proposed, as depicted in Scheme 3. Initially, substrate 1a can easily tautomerize into intermediate 1a′ in the presence of the α-hydrogen of the amidine group under such reaction conditions. And then, a hydrogen atom of intermediate 1a′ is abstracted by CBr3 radical, which is derived from the homolytic cleavage of the C–Br bond of CBr4 upon heating accompanied by the formation of Br radical, to deliver the radical intermediate A. Intermediate A reacts rapidly with Br radical to afford intermediate B. Subsequently, a base-promoted intramolecular nucleophilic substitution of intermediate C, which is achieved from intermediate B in the presence of base, will occur to provide intermediate D. Intermediate D will tautomerize into intermediate D′ again. Finally, the desired product 3a via intermediate E will be provided by the nucleophilic substitution of intermediate D′ with CHBr3 under base. Meanwhile, we can also not rule out another possible mechanism route that experienced a base promoted nucleophilic substitution. Thus, another possible mechanism process is also described by us (see ESI†).
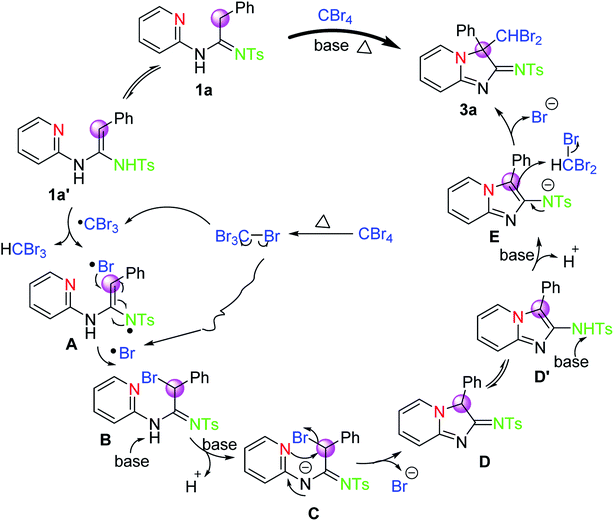 |
| Scheme 3 The tentative mechanistic pathway for the formation of 3a. | |
In conclusion, we have demonstrated a facile metal-free strategy to form 2-iminoimidazo[1, 2-a]-pyridines by CBr4-mediated intramolecular CDC reaction under mild conditions, in which the new heterocyclic skeletons were constructed from amidines by the sequential C(sp3)–H bifunctionalization mode on the same carbon atom involving C–N and C–C bond formation. The surveys could better reveal that the results of the reaction depended greatly on the structure of the substrates and the reaction conditions. Moreover, this approach further indicates that amidines have abundant reactivity under various reaction conditions again. This work also provides an excellent example for CBr4 as an efficient reagent to initiate radical reactions under initiator-free conditions or to give rise to nucleophilic substitution reactions only by base.
Experimental section
General remarks
All reagents were purchased from commercial suppliers, and were used without further purification. All solvents were treated according to standard procedures. The progress of reactions was monitored by TLC. For chromatographic purifications, 200–300 mesh silica gel was used. 1H (500 MHz) and 13C (126 MHz) NMR spectra were recorded with tetramethylsilane as an internal standard. HRMS measurements were carried out using the ESI ionization technique with an FT-ICR analyzer. Data are reported as follows: chemical shift, multiplicity (s = singlet, d = doublet, t = triplet, q = quartet, m = multiplet), coupling constants (Hz) and integration. CCDC (for 3a) contains the supplementary crystallographic data for this paper.
Procedure for generation of N-(2-pyridyl)amidines 1
N-(2-Pyridyl)amidines (1) were prepared as reported in the literature1f,26a according to the reaction equation (see ESI†) or are commercially available.
General procedure for the synthesis of 3
Under N2, a mixture of N-(2-pyridyl)amidines (1) (0.2 mmol), CBr4 (2) (0.24 mmol), K2CO3 (0.3 mmol), in dry DCM (2 mL) was added to a sealed tube. And then, the mixture was stirred at 100 °C for 12 h. The reaction mixture was then cooled to room temperature. The solvent was removed under reduced pressure to give a residue. The crude product was purified on silica gel column chromatography using ethyl acetate and petroleum ether as the eluent to afford the desired products 3.
Characterization data for 3
N-(3-(Dibromomethyl)-3-phenylimidazo[1,2-a]pyridin-2(3H)-ylidene)-4-methylbenzenesulfonamide (3a). A yellow solid (78%). Mp: 216–218 °C. 1H NMR (500 MHz, chloroform-d) δ 8.42 (d, J = 6.4 Hz, 1H), 8.02 (t, J = 7.6 Hz, 1H), 7.85 (d, J = 8.1 Hz, 2H), 7.49 (d, J = 8.8 Hz, 1H), 7.37 (d, J = 7.7 Hz, 3H), 7.17 (d, J = 7.9 Hz, 2H), 7.09 (d, J = 7.7 Hz, 3H), 6.59 (s, 1H), 2.35 (s, 3H). 13C NMR (126 MHz, chloroform-d) δ 176.06, 165.89, 145.29, 142.25, 138.75, 135.34, 133.92, 129.73, 129.33, 128.62, 127.61, 125.53, 117.68, 114.59, 81.42, 45.46, 21.53. HRMS: calcd for C21H17Br2N3O2S [M + H]+ 533.9481; found 533.9486.
N-(3-(Dibromomethyl)-3-(4-ethylphenyl)imidazo[1,2-a]pyridin-2(3H)-ylidene)-4-methylbenzenesulfonamide (3b). A light yellow solid (64%). Mp: 227–229 °C 1H NMR (500 MHz, chloroform-d) δ 8.42 (d, J = 6.5 Hz, 1H), 8.00 (t, J = 7.7 Hz, 1H), 7.87 (d, J = 8.2 Hz, 2H), 7.50 (d, J = 8.9 Hz, 1H), 7.18 (t, J = 9.4 Hz, 4H), 7.06 (t, J = 6.8 Hz, 1H), 7.00 (d, J = 8.2 Hz, 2H), 6.58 (s, 1H), 2.65 (q, J = 7.5 Hz, 2H), 2.36 (s, 3H), 1.23 (t, J = 7.6 Hz, 3H). 13C NMR (126 MHz, chloroform-d) δ 176.28, 166.01, 146.12, 144.77, 142.12, 138.89, 135.34, 131.23, 128.78, 128.56, 127.68, 127.54, 125.47, 117.70, 114.00, 81.36, 45.66, 28.40, 21.52, 15.15. HRMS: calcd for C23H21Br2N3O2S [M + H]+ 563.9774; found 563.9783.
N-(3-(Dibromomethyl)-3-(p-tolyl)imidazo[1,2-a]pyridin-2(3H)-ylidene)-4-methylbenzenesulfonamide (3c). A light yellow solid (66%). Mp: 182–184 °C 1H NMR (500 MHz, chloroform-d) δ 8.40 (d, J = 6.5 Hz, 1H), 8.01 (t, J = 7.6 Hz, 1H), 7.86 (d, J = 8.2 Hz, 2H), 7.48 (d, J = 8.9 Hz, 1H), 7.16 (d, J = 7.9 Hz, 4H), 7.07 (t, J = 6.8 Hz, 1H), 6.96 (d, J = 8.2 Hz, 2H), 6.56 (s, 1H), 2.34 (d, J = 8.2 Hz, 6H). 13C NMR (126 MHz, chloroform-d) δ 176.27, 165.95, 144.96, 142.15, 139.95, 138.89, 135.35, 131.07, 129.99, 128.58, 127.67, 125.39, 117.68, 114.19, 81.33, 45.70, 21.54, 21.10. HRMS: calcd for C22H19Br2N3O2S [M + H]+ 549.9617; found 549.9625.
N-(3-(Dibromomethyl)-3-(m-tolyl)imidazo[1,2-a]pyridin-2(3H)-ylidene)-4-methylbenzenesulfonamide (3d). A yellow solid (69%). Mp: 178–180 °C 1H NMR (500 MHz, chloroform-d) δ 8.40 (d, J = 6.5 Hz, 1H), 8.01 (t, J = 7.7 Hz, 1H), 7.84 (d, J = 8.2 Hz, 2H), 7.49 (d, J = 8.8 Hz, 1H), 7.24 (t, J = 7.7 Hz, 1H), 7.19–7.14 (m, 3H), 7.08 (t, J = 6.8 Hz, 1H), 6.85 (d, J = 6.8 Hz, 2H), 6.57 (s, 1H), 2.32 (d, J = 19.1 Hz, 6H). 13C NMR (126 MHz, chloroform-d) δ 176.09, 166.01, 144.82, 142.15, 139.24, 135.33, 133.91, 130.53, 129.16, 128.57, 127.64, 127.51, 126.00, 122.65, 117.68, 114.02, 81.37, 45.55, 21.61, 21.52. HRMS: calcd for C22H19Br2N3O2S [M + H]+ 549.9617; found 549.9627.
N-(3-(Dibromomethyl)-3-(4-methoxyphenyl)imidazo[1,2-a]pyridin-2(3H)-ylidene)-4-methylbenzenesulfonamide (3e). A light yellow solid (76%). Mp: 155–157 °C 1H NMR (500 MHz, chloroform-d) δ 8.42 (d, J = 6.5 Hz, 1H), 8.00 (t, J = 7.7 Hz, 1H), 7.88 (d, J = 8.1 Hz, 2H), 7.49 (d, J = 8.8 Hz, 1H), 7.18 (d, J = 7.9 Hz, 2H), 7.04 (dd, J = 16.4, 7.8 Hz, 3H), 6.88 (d, J = 8.8 Hz, 2H), 6.55 (s, 1H), 3.82 (s, 3H), 2.37 (s, 3H). 13C NMR (126 MHz, chloroform-d) δ 176.59, 166.11, 160.59, 144.79, 142.27, 139.03, 135.41, 128.70, 127.81, 127.05, 126.06, 117.86, 114.78, 114.04, 81.17, 55.54, 46.00, 21.64. HRMS: calcd for C22H19Br2N3O3S [M + H]+ 565.9567; found 565.9573.
N-(3-(4-Chlorophenyl)-3-(dibromomethyl)imidazo[1,2-a]pyridin-2(3H)-ylidene)-4-methylbenzenesulfonamide (3f). A light yellow solid (48%). Mp: 172–174 °C 1H NMR (500 MHz, chloroform-d) δ 8.41 (s, 1H), 7.84 (d, J = 7.4 Hz, 2H), 7.58 (s, 1H), 7.42–7.37 (m, 2H), 7.33–7.30 (m, 2H), 7.18 (d, J = 7.4 Hz, 2H), 7.05–7.02 (m, 2H), 6.57 (s, 1H), 2.35 (s, 3H). 13C NMR (126 MHz, chloroform-d) δ 175.69, 165.56, 145.58, 142.56, 138.54, 136.09, 135.24, 132.50, 129.61, 129.10, 128.80, 127.60, 127.31, 127.07, 118.01, 114.93, 81.07, 45.19, 21.59. HRMS: calcd for C21H16Br2ClN3O2S [M + H]+ 569.9070; found 569.9074.
N-(3-(3-Chlorophenyl)-3-(dibromomethyl)imidazo[1,2-a]pyridin-2(3H)-ylidene)-4-methylbenzenesulfonamide (3g). A yellow solid (57%). Mp: 161–163 °C 1H NMR (500 MHz, chloroform-d) δ 8.42 (d, J = 6.5 Hz, 1H), 8.04 (t, J = 7.7 Hz, 1H), 7.86 (d, J = 8.1 Hz, 2H), 7.53 (d, J = 8.8 Hz, 1H), 7.39 (d, J = 8.0 Hz, 1H), 7.34 (t, J = 7.9 Hz, 1H), 7.19 (d, J = 8.0 Hz, 2H), 7.10 (t, J = 7.6 Hz, 2H), 7.02 (s, 1H), 6.53 (s, 1H), 2.37 (s, 3H). 13C NMR (101 MHz, chloroform-d) δ 175.21, 165.38, 145.62, 142.12, 138.24, 135.63, 135.05, 130.42, 129.64, 129.07, 128.39, 127.15, 125.77, 125.51, 123.82, 117.32, 80.73, 44.85, 21.14. HRMS: calcd for C21H16Br2ClN3O2S [M + H]+ 569.9070; found 569.9070.
N-(3-(Dibromomethyl)-3-(4-fluorophenyl)imidazo[1,2-a]pyridin-2(3H)-ylidene)-4-methylbenzenesulfonamide (3h). A yellow solid (54%). Mp: 203–205 °C 1H NMR (500 MHz, chloroform-d) δ 8.42 (d, J = 6.5 Hz, 1H), 8.02 (t, J = 8.1 Hz, 1H), 7.87 (d, J = 8.1 Hz, 2H), 7.51 (d, J = 8.9 Hz, 1H), 7.19 (d, J = 7.9 Hz, 2H), 7.14–7.10 (m, 2H), 7.08 (d, J = 7.7 Hz, 3H), 6.55 (s, 1H), 2.37 (s, 3H). 13C NMR (126 MHz, chloroform-d) δ 175.79, 165.67, 145.39, 142.17, 138.62, 135.19, 129.86, 128.47, 127.70, 127.64, 127.37, 117.42, 116.29, 116.11, 114.74, 80.83, 45.37, 21.33. HRMS: calcd for C21H16Br2FN3O2S [M + H]+ 553.9367; found 553.9376.
N-(3-Butyl-3-(dibromomethyl)imidazo[1,2-a]pyridin-2(3H)-ylidene)-4-methylbenzenesulfonamide (3i). A light yellow solid (65%). Mp: 185–187 °C 1H NMR (500 MHz, chloroform-d) δ 8.27 (d, J = 6.4 Hz, 1H), 7.94 (dd, J = 21.5, 7.9 Hz, 3H), 7.41 (d, J = 8.8 Hz, 1H), 7.19 (d, J = 8.0 Hz, 2H), 7.04 (t, J = 6.8 Hz, 1H), 5.99 (s, 1H), 2.34 (s, 3H), 2.20 (s, 2H), 2.06 (s, 2H), 1.15–1.11 (m, 2H), 0.69 (t, J = 7.3 Hz, 3H). 13C NMR (126 MHz, chloroform-d) δ 176.65, 165.67, 144.34, 142.32, 138.73, 133.60, 128.59, 127.96, 117.47, 114.64, 79.27, 46.09, 38.38, 25.17, 22.15, 21.52, 13.52. HRMS: calcd for C19H21Br2N3O2S [M + H]+ 515.9774; found 515.9787.
N-(3-(Dibromomethyl)-6-methyl-3-phenylimidazo[1,2-a]pyridin-2(3H)-ylidene)-4-methylbenzenesulfonamide (3j). A yellow solid (75%). Mp: 146–148 °C 1H NMR (500 MHz, chloroform-d) δ 8.21 (s, 1H), 7.86 (t, J = 8.4 Hz, 3H), 7.45 (d, J = 9.0 Hz, 1H), 7.39 (d, J = 7.6 Hz, 3H), 7.17 (d, J = 8.0 Hz, 2H), 7.09 (d, J = 6.9 Hz, 2H), 6.61 (s, 1H), 2.40 (s, 3H), 2.36 (s, 3H). 13C NMR (126 MHz, chloroform-d) δ 176.01, 164.56, 147.34, 142.03, 134.11, 133.07, 129.78, 129.64, 129.27, 128.86, 128.73, 128.54, 127.87, 127.63, 125.60, 124.73, 121.09, 117.20, 81.68, 45.60, 21.51, 18.04. HRMS: calcd for C22H19Br2N3O2S [M + Na]+ 571.9437; found 571.9442.
N-(3-(Dibromomethyl)-6-methyl-3-(p-tolyl)imidazo[1,2-a]pyridin-2(3H)-ylidene)-4-methylbenzenesulfonamide (3k). A yellow solid (77%). Mp: 211–213 °C 1H NMR (500 MHz, chloroform-d) δ 8.18 (s, 1H), 7.86 (t, J = 8.3 Hz, 3H), 7.44 (d, J = 9.0 Hz, 1H), 7.16 (d, J = 5.2 Hz, 4H), 6.95 (d, J = 7.9 Hz, 2H), 6.57 (s, 1H), 2.38 (s, 3H), 2.34 (d, J = 4.2 Hz, 6H). 13C NMR (126 MHz, chloroform-d) δ 176.26, 164.44, 147.42, 141.98, 139.80, 133.09, 131.19, 129.92, 128.52, 127.62, 125.49, 124.80, 117.13, 81.62, 45.74, 21.51, 21.08, 18.03. HRMS: calcd for C23H21Br2N3O2S [M + H]+ 563.9774; found 563.9780.
N-(3-(Dibromomethyl)-3-(4-ethylphenyl)-6-methylimidazo[1,2-a]pyridin-2(3H)-ylidene)-4-methylbenzenesulfonamide (3l). A light yellow solid (58%). Mp: 190–192 °C 1H NMR (500 MHz, chloroform-d) δ 8.20 (s, 1H), 7.86 (d, J = 8.2 Hz, 3H), 7.45 (d, J = 9.0 Hz, 1H), 7.18 (dd, J = 16.2, 8.1 Hz, 4H), 6.98 (d, J = 8.3 Hz, 2H), 6.60 (s, 1H), 2.65 (q, J = 7.5 Hz, 2H), 2.37 (d, J = 17.3 Hz, 6H), 1.24 (s, 3H). 13C NMR (126 MHz, chloroform-d) δ 176.26, 164.55, 147.14, 146.00, 141.95, 133.13, 128.73, 128.50, 127.65, 125.57, 124.50, 117.16, 81.66, 45.73, 28.39, 21.50, 18.02, 15.14. HRMS: calcd for C24H23Br2N3O2S [M + Na]+ 599.9750; found 599.9789.
N-(3-(Dibromomethyl)-3-(4-methoxyphenyl)-6-methylimidazo[1,2-a]pyridin-2(3H)-ylidene)-4-methylbenzenesulfonamide (3m). A yellow solid (73%). Mp: 148–150 °C 1H NMR (500 MHz, chloroform-d) δ 8.18 (s, 1H), 7.85 (d, J = 8.0 Hz, 3H), 7.43 (d, J = 9.0 Hz, 1H), 7.16 (d, J = 7.9 Hz, 2H), 7.00 (d, J = 8.7 Hz, 2H), 6.87 (d, J = 8.8 Hz, 2H), 6.55 (s, 1H), 3.79 (s, 3H), 2.36 (d, J = 15.9 Hz, 6H). 13C NMR (126 MHz, chloroform-d) δ 176.49, 164.33, 160.37, 147.39, 142.05, 133.10, 129.50, 128.56, 127.57, 127.06, 126.33, 125.98, 117.17, 114.61, 81.47, 55.42, 45.85, 21.49, 18.03. HRMS: calcd for C23H21Br2N3O3S [M + H]+ 579.9723; found 579.9725.
N-(3-(4-Chlorophenyl)-3-(dibromomethyl)-6-methylimidazo[1,2-a]pyridin-2(3H)-ylidene)-4-methylbenzenesulfonamide (3n). A yellow solid (60%). Mp: 151–153 °C 1H NMR (500 MHz, chloroform-d) δ 8.17 (s, 1H), 7.88 (d, J = 8.7 Hz, 1H), 7.84 (d, J = 8.2 Hz, 2H), 7.46 (d, J = 9.0 Hz, 1H), 7.34 (d, J = 8.7 Hz, 2H), 7.17 (d, J = 8.0 Hz, 2H), 7.04 (d, J = 8.6 Hz, 2H), 6.53 (s, 1H), 2.37 (d, J = 15.7 Hz, 6H). 13C NMR (126 MHz, chloroform-d) δ 175.57, 164.54, 147.66, 142.21, 132.80, 132.67, 131.04, 129.45, 129.01, 128.96, 128.60, 128.08, 127.61, 127.08, 120.99, 117.32, 117.24, 81.19, 45.25, 21.51, 18.05. HRMS: calcd for C22H18Br2ClN3O2S [M + H]+ 583.9226; found 583.9229.
N-(3-(Dibromomethyl)-3-(3-fluorophenyl)-6-methylimidazo[1,2-a]pyridin-2(3H)-ylidene)-4-methylbenzenesulfonamide (3o). A yellow solid (66%). Mp: 127–129 °C 1H NMR (500 MHz, chloroform-d) δ 8.16 (s, 1H), 7.90 (d, J = 8.8 Hz, 1H), 7.80 (d, J = 8.1 Hz, 2H), 7.42 (d, J = 9.0 Hz, 1H), 7.34–7.29 (m, 1H), 7.12 (d, J = 8.0 Hz, 2H), 7.04 (t, J = 8.0 Hz, 1H), 6.82 (t, J = 7.0 Hz, 2H), 6.48 (s, 1H), 2.32 (d, J = 16.8 Hz, 6H). 13C NMR (126 MHz, chloroform-d) δ 174.68, 163.35, 147.50, 141.36, 138.09, 135.55, 132.30, 130.27, 130.20, 128.51, 127.78, 126.64, 125.28, 120.76, 116.12, 112.81, 112.61, 80.55, 44.50, 20.65, 20.59, 17.13. HRMS: calcd for C22H18Br2FN3O2S [M + H]+ 567.9523; found 567.9528.
N-(6-Chloro-3-(dibromomethyl)-3-phenylimidazo[1,2-a]pyridin-2(3H)-ylidene)-4-methylbenzenesulfonamide (3p). A yellow solid (58%). Mp: 144–146 °C 1H NMR (500 MHz, chloroform-d) δ 8.40 (s, 1H), 7.99 (d, J = 9.2 Hz, 1H), 7.84 (d, J = 8.1 Hz, 2H), 7.59 (d, J = 9.5 Hz, 1H), 7.40 (d, J = 7.4 Hz, 3H), 7.18 (d, J = 7.9 Hz, 2H), 7.09 (d, J = 6.9 Hz, 2H), 6.55 (s, 1H), 2.36 (s, 3H). 13C NMR (126 MHz, chloroform-d) δ 175.93, 164.80, 145.90, 142.44, 138.67, 133.58, 132.83, 129.96, 129.63, 129.50, 129.04, 128.71, 127.91, 127.57, 125.38, 118.51, 81.91, 45.09, 21.54. HRMS: calcd for C21H16Br2ClN3O2S [M + H]+ 569.9070; found 569.9075.
N-(6-Chloro-3-(Dibromomethyl)-3-(4-ethylphenyl)imidazo[1,2-a]pyridin-2(3H)-ylidene)-4-methylbenzenesulfonamide (3q). A yellow solid (61%). Mp: 149–151 °C 1H NMR (500 MHz, chloroform-d) δ 8.41 (s, 1H), 7.97–7.93 (m, 1H), 7.86 (d, J = 8.1 Hz, 2H), 7.53 (d, J = 9.5 Hz, 1H), 7.21 (dd, J = 16.9, 8.1 Hz, 4H), 7.00 (d, J = 8.2 Hz, 2H), 6.55 (s, 1H), 2.67 (q, J = 7.6 Hz, 2H), 2.38 (s, 3H), 1.24 (d, J = 7.6 Hz, 3H). 13C NMR (126 MHz, chloroform-d) δ 176.21, 164.75, 146.36, 145.80, 142.36, 132.90, 130.83, 129.55, 128.95, 128.67, 128.50, 127.95, 127.60, 125.36, 121.33, 118.44, 81.91, 45.22, 28.39, 21.53, 15.12. HRMS: calcd for C23H20Br2ClN3O2S [M + H]+ 597.93834; found 597.93830.
N-(6-Chloro-3-(dibromomethyl)-3-(m-tolyl)imidazo[1,2-a]pyridin-2(3H)-ylidene)-4-methylbenzenesulfonamide (3r). A yellow solid (57%). Mp: 183–185 °C 1H NMR (500 MHz, chloroform-d) δ 8.40 (s, 1H), 7.99 (d, J = 9.3 Hz, 1H), 7.84 (d, J = 8.0 Hz, 2H), 7.61 (d, J = 9.5 Hz, 1H), 7.28–7.26 (m, 1H), 7.20 (dd, J = 16.1, 7.7 Hz, 3H), 6.88 (s, 1H), 6.84 (d, J = 7.7 Hz, 1H), 6.54 (s, 1H), 2.36 (d, J = 13.9 Hz, 6H). 13C NMR (126 MHz, chloroform-d) δ 176.01, 164.77, 146.12, 142.40, 139.46, 138.80, 133.51, 132.79, 130.75, 129.31, 128.69, 127.50, 125.86, 122.51, 121.41, 118.60, 81.91, 45.09, 21.63, 21.54. HRMS: calcd for C22H18Br2ClN3O2S [M + H]+ 583.9226; found 583.9231.
N-(6-Chloro-3-(dibromomethyl)-3-(3-methoxyphenyl)imidazo[1,2-a]pyridin-2(3H)-ylidene)-4-methylbenzenesulfonamide (3s). A yellow solid (62%). Mp: 145–147 °C 1H NMR (500 MHz, chloroform-d) δ 8.42 (s, 1H), 7.97 (d, J = 8.9 Hz, 1H), 7.86 (d, J = 8.1 Hz, 2H), 7.57 (d, J = 9.5 Hz, 1H), 7.32 (d, J = 8.0 Hz, 1H), 7.19 (d, J = 7.9 Hz, 2H), 6.94 (d, J = 8.6 Hz, 1H), 6.64 (d, J = 8.0 Hz, 2H), 6.51 (s, 1H), 3.77 (s, 3H), 2.37 (s, 3H). 13C NMR (126 MHz, chloroform-d) δ 175.79, 164.72, 160.20, 145.92, 142.47, 138.73, 134.88, 132.86, 130.54, 128.73, 127.53, 121.32, 118.47, 117.45, 114.84, 112.03, 81.74, 55.51, 45.03, 21.52. HRMS: calcd for C22H18Br2ClN3O3S [M + H]+ 597.9196; found 597.9191.
N-(6-Chloro-3-(dibromomethyl)-3-(4-methoxyphenyl)imidazo[1,2-a]pyridin-2(3H)-ylidene)-4-methylbenzenesulfonamide (3t). A yellow solid (61%). Mp: 134–136 °C 1H NMR (500 MHz, chloroform-d) δ 8.40 (s, 1H), 7.96 (d, J = 8.9 Hz, 1H), 7.85 (d, J = 7.9 Hz, 2H), 7.55 (d, J = 9.0 Hz, 1H), 7.19 (d, J = 7.8 Hz, 2H), 7.02 (d, J = 8.6 Hz, 2H), 6.90 (d, J = 8.4 Hz, 2H), 6.52 (s, 1H), 3.81 (s, 3H), 2.37 (s, 3H). 13C NMR (126 MHz, chloroform-d) δ 176.48, 164.59, 160.57, 145.97, 142.50, 132.87, 131.08, 128.75, 127.53, 126.87, 125.38, 121.49, 118.48, 114.83, 81.72, 55.49, 45.35, 21.54. HRMS: calcd for C22H18Br2ClN3O3S [M + H]+ 597.9196; found 597.9192.
N-(6-Chloro-3-(3-chlorophenyl)-3-(dibromomethyl)imidazo[1,2-a]pyridin-2(3H)-ylidene)-4-methylbenzenesulfonamide (3u). A light yellow solid (56%). Mp: 231–233 °C 1H NMR (500 MHz, chloroform-d) δ 8.41 (s, 1H), 7.98 (d, J = 9.4 Hz, 1H), 7.85 (d, J = 8.1 Hz, 2H), 7.57 (d, J = 9.5 Hz, 1H), 7.42 (d, J = 8.1 Hz, 1H), 7.37 (t, J = 8.2 Hz, 1H), 7.22 (d, J = 7.9 Hz, 2H), 7.06 (s, 2H), 6.49 (s, 1H), 2.39 (s, 3H). 13C NMR (126 MHz, chloroform-d) δ 175.16, 164.92, 145.94, 142.60, 135.59, 135.47, 132.50, 130.78, 130.26, 128.77, 127.56, 125.52, 123.75, 121.48, 118.62, 81.23, 44.59, 21.54. HRMS: calcd for C21H15Br2Cl2N3O2S [M + H]+ 603.8679; found 603.8678.
N-(6-Chloro-3-(dibromomethyl)-3-(3-fluorophenyl)imidazo[1,2-a]pyridin-2(3H)-ylidene)-4-methylbenzenesulfonamide (3v). A yellow solid (58%). Mp: 145–147 °C 1H NMR (500 MHz, chloroform-d) δ 8.39 (s, 1H), 8.02 (d, J = 9.3 Hz, 1H), 7.83 (d, J = 8.1 Hz, 2H), 7.62 (d, J = 9.4 Hz, 1H), 7.37 (q, J = 7.8 Hz, 1H), 7.18 (d, J = 7.9 Hz, 2H), 7.11 (t, J = 6.8 Hz, 1H), 6.87 (t, J = 9.8 Hz, 2H), 6.48 (s, 1H), 2.35 (s, 3H). 13C NMR (126 MHz, chloroform-d) δ 175.31, 164.81, 163.89, 161.91, 146.28, 142.63, 138.49, 135.72, 132.54, 131.30, 128.77, 127.55, 121.72, 121.18, 118.70, 117.08, 113.18, 112.98, 81.31, 44.61, 21.54. HRMS: calcd for C21H15Br2ClFN3O2S [M + H]+ 585.8997; found 585.8999.
N-(3-(Dibromomethyl)-3-phenylimidazo[1,2-a]pyridin-2(3H)-ylidene)methanesulfonamide (3w). A yellow solid (61%). Mp: 256–258 °C 1H NMR (500 MHz, chloroform-d) δ 8.50 (d, J = 6.4 Hz, 1H), 8.09 (t, J = 7.5 Hz, 1H), 7.59 (d, J = 8.8 Hz, 1H), 7.41 (d, J = 5.7 Hz, 3H), 7.15 (d, J = 5.6 Hz, 3H), 6.66 (s, 1H), 3.15 (s, 3H). 13C NMR (126 MHz, chloroform-d) δ 176.80, 165.98, 145.12, 135.43, 129.89, 129.46, 125.50, 117.58, 114.24, 81.37, 45.89, 39.93. HRMS: calcd for C15H13Br2N3O2S [M + H]+ 457.9167; found 457.9168.
N-(3-(Dibromomethyl)-3-phenylimidazo[1,2-a]pyridin-2(3H)-ylidene)benzenesulfonamide (3x). A yellow solid (49%). Mp: 139–141 °C 1H NMR (500 MHz, chloroform-d) δ 8.43 (d, J = 4.3 Hz, 1H), 7.98 (d, J = 7.6 Hz, 2H), 7.60 (d, J = 5.9 Hz, 5H), 7.54 (s, 1H), 7.46 (d, J = 7.6 Hz, 2H), 7.39 (d, J = 7.1 Hz, 3H), 7.25 (s, 1H), 7.09 (d, J = 6.5 Hz, 3H), 6.60 (s, 1H). 13C NMR (126 MHz, chloroform-d) δ 176.20, 165.73, 145.51, 143.23, 135.47, 131.98, 129.54, 129.28, 128.79, 127.93, 127.42, 126.08, 125.51, 117.46, 114.89, 81.53, 45.33. HRMS: calcd for C20H15Br2N3O2S [M + Na]+ 543.9124; found 543.9128.
N-(3-(Dibromomethyl)-3-(4-ethylphenyl)imidazo[1,2-a]pyridin-2(3H)-ylidene)benzenesulfonamide (3y). A yellow solid (47%). Mp: 147–149 °C 1H NMR (500 MHz, chloroform-d) δ 8.42 (d, J = 6.1 Hz, 1H), 7.97 (d, J = 7.9 Hz, 2H), 7.49 (d, J = 8.4 Hz, 1H), 7.43 (d, J = 7.6 Hz, 1H), 7.37 (d, J = 7.0 Hz, 2H), 7.18 (d, J = 7.5 Hz, 2H), 7.07 (dd, J = 14.5, 7.5 Hz, 2H), 6.98 (d, J = 6.8 Hz, 2H), 6.58 (s, 1H), 2.65–2.62 (m, 2H), 1.21 (d, J = 6.6 Hz, 3H). 13C NMR (126 MHz, chloroform-d) δ 176.48, 165.92, 146.16, 145.06, 135.39, 131.69, 128.81, 128.22, 127.93, 127.63, 125.47, 117.63, 114.34, 81.47, 45.52, 28.39, 15.17. HRMS: calcd for C22H19Br2N3O2S [M + H]+ 549.9617; found 549.9619.
N-(3-(Dibromomethyl)-6-methyl-3-phenylimidazo[1,2-a]pyridin-2(3H)-ylidene)benzenesulfonamide (3z). A light yellow solid (65%). Mp: 121–123 °C 1H NMR (500 MHz, chloroform-d) δ 8.19 (s, 1H), 7.90 (d, J = 7.2 Hz, 3H), 7.42 (dd, J = 17.0, 7.9 Hz, 3H), 7.37–7.33 (m, 4H), 7.08–7.04 (m, 2H), 6.62 (s, 1H), 2.37 (s, 3H). 13C NMR (101 MHz, chloroform-d) δ 175.93, 163.62, 147.96, 143.26, 141.20, 133.60, 133.05, 131.62, 129.38, 129.01, 128.53, 127.71, 127.00, 125.68, 125.43, 116.49, 81.72, 45.21, 17.63. HRMS: calcd for C21H17Br2N3O2S [M + H]+ 535.9461; found 535.9470.
N-(6-Chloro-3-(dibromomethyl)-3-phenylimidazo[1,2-a]pyridin-2(3H)-ylidene)benzenesulfonamide (3ab). A yellow solid (62%). Mp: 141–143 °C. 1H NMR (500 MHz, chloroform-d) δ 8.41 (s, 1H), 8.00 (d, J = 8.4 Hz, 1H), 7.96 (d, J = 7.6 Hz, 2H), 7.59 (d, J = 9.1 Hz, 1H), 7.49–7.43 (m, 2H), 7.42–7.38 (m, 4H), 7.09 (d, J = 6.9 Hz, 2H), 6.56 (s, 1H). 13C NMR (101 MHz, chloroform-d) δ 176.05, 164.62, 145.97, 141.20, 133.32, 132.80, 131.89, 129.93, 129.45, 129.00, 128.02, 127.80, 127.41, 125.27, 118.40, 81.93, 44.82. HRMS: calcd for C20H14Br2ClN3O2S [M + Na]+ 577.8733; found 577.8738.
N-(6-Chloro-3-(dibromomethyl)-3-(4-ethylphenyl)imidazo[1,2-a]pyridin-2(3H)-ylidene)benzenesulfonamide (3ac). A yellow solid (46%). Mp: 135–137 °C 1H NMR (500 MHz, chloroform-d) δ 8.40 (s, 1H), 7.94 (d, J = 6.6 Hz, 2H), 7.61–7.51 (m, 2H), 7.48–7.43 (m, 2H), 7.38 (s, 2H), 7.23–7.19 (m, 2H), 7.00–6.97 (m, 1H), 6.57 (s, 1H), 2.68–2.61 (m, 2H), 1.22 (d, J = 6.6 Hz, 3H). 13C NMR (126 MHz, chloroform-d) δ 176.45, 164.67, 146.47, 146.03, 141.35, 133.03, 132.38, 131.99, 130.68, 129.54, 129.04, 129.00, 128.14, 127.53, 126.35, 125.42, 118.49, 82.12, 45.06, 28.42, 15.16. HRMS: calcd for C22H18Br2ClN3O2S [M + H]+ 583.9226; found 583.9229.
N-(6-Chloro-3-(4-chlorophenyl)-3-(dibromomethyl)imidazo[1,2-a]pyridin-2(3H)-ylidene)benzenesulfonamide (3ad). A yellow solid (65%). Mp: 160–162 °C 1H NMR (500 MHz, chloroform-d) δ 8.39 (s, 1H), 7.96 (d, J = 7.7 Hz, 2H), 7.59 (d, J = 9.4 Hz, 1H), 7.48 (d, J = 7.3 Hz, 1H), 7.41 (t, J = 8.2 Hz, 3H), 7.37 (d, J = 8.4 Hz, 2H), 7.05 (d, J = 8.4 Hz, 2H), 6.54 (s, 1H). 13C NMR (101 MHz, chloroform-d) δ 180.53, 169.20, 151.07, 145.74, 144.01, 140.77, 137.44, 137.31, 136.78, 136.64, 134.30, 132.81, 132.07, 131.67, 126.73, 123.01, 116.37, 86.31, 82.41, 49.37. HRMS: calcd for C20H13Br2Cl2N3O2S [M + H]+ 589.8522; found 589.8528.
Conflicts of interest
There are no conflicts of interest to declare.
Acknowledgements
This research was supported by the Horizontal Projects of Shanghai Institute of Technology (J2017-150, J2017-202).
Notes and references
- Selected literatures for C–H functionalization:
(a) V. Ritleng, C. Sirlin and M. Pfeffer, Chem. Rev., 2002, 102, 1731–1770 CrossRef CAS PubMed;
(b) D. Alberico, M. E. Scott and M. Lautens, Chem. Rev., 2007, 107, 174–238 CrossRef CAS PubMed;
(c) I. A. I. Mkhalid, J. H. Barnard, T. B. Marder, J. M. Murphy and J. F. Hartwig, Chem. Rev., 2010, 110, 890–931 CrossRef CAS PubMed;
(d) J. Wencel-Delord, T. Dröge, F. Liu and F. Glorius, Chem. Soc. Rev., 2011, 40, 4740–4761 RSC;
(e) N. Kuhl, M. N. Hopkinson, J. Wencel-Delord and F. Glorius, Angew. Chem., Int. Ed., 2012, 51, 10236–10254 CrossRef CAS PubMed;
(f) F. P. Yi, S. X. Zhang, L. R. Zhang, W. Y. Yi and R. Yu, Asian J. Org. Chem., 2017, 6, 1808–1817 CrossRef CAS;
(g) J. Xie, H. L. Jiang, Y. X. Cheng and C. J. Zhu, Chem. Commun., 2012, 48, 979–981 RSC.
- R. Jana, T. P. Pathak and M. S. Sigman, Chem. Rev., 2011, 111, 1417–1492 CrossRef CAS PubMed.
- Selected literatures for CDC reactions, see:
(a) C. J. Li, Acc. Chem. Res., 2009, 42, 335–344 CrossRef CAS PubMed;
(b) S. A. Girard, T. Knauber and C. J. Li, Angew. Chem., Int. Ed., 2014, 53, 74–100 CrossRef CAS PubMed;
(c) M. Klussmann and D. Sureshkumar, Synthesis, 2011, 353–369 CrossRef CAS.
- R. G. Bergman, Nature, 2007, 446, 391–393 CrossRef CAS PubMed.
- Selected literatures for the formation of C–X (X = C, N) bonds via CDC reactions using peroxides as oxidant, see:
(a) L. K. Jin, J. Feng, G. P. Lu and C. Cai, Adv. Synth. Catal., 2015, 357, 2105–2110 CrossRef CAS;
(b) Z. H. Jia, T. Nagano, X. S. Li and A. S. C. Chan, Eur. J. Org. Chem., 2013, 858–861 CrossRef CAS;
(c) R. Xia, H. Y. Niu, G. R. Qu and H. M. Guo, Org. Lett., 2012, 14, 5546–5549 CrossRef CAS PubMed;
(d) W. P. Mai, H. H. Wang, Z. C. Li, J. W. Yuan, Y. M. Xiao, L. R. Yang, P. Mao and L. B. Qu, Chem. Commun., 2012, 48, 10117–10119 RSC;
(e) T. He, L. Yu, L. Zhang, L. Wang and M. Wang, Org. Lett., 2011, 13, 5016–5019 CrossRef CAS PubMed;
(f) W. Liu, S. Liu, H. Xie, Z. Qing, J. Zenga and P. Cheng, RSC Adv., 2015, 5, 17383–17388 RSC;
(g) M. Wan, H. X. Lou and L. Liu, Chem. Commun., 2015, 51, 13953–13956 RSC.
- Selected literatures for the formation of C–X (X = C, N) bonds via CDC reactions using quinones as oxidant, see:
(a) S. S. Kong, L. Q. Zhang, X. L. Dai, L. Z. Tao, C. S. Xie, L. Shi and M. Wang, Adv. Synth. Catal., 2015, 357, 2453–2456 CrossRef CAS;
(b) K. Kawasumi, Q. Y. Zhang, Y. Segawa, L. T. Scott and K. Itami, Nat. Chem., 2013, 5, 739–744 CrossRef CAS PubMed;
(c) K. Alagiri, P. Devadig and K. R. Prabhu, Chem. – Eur. J., 2012, 18, 5160–5164 CrossRef CAS PubMed;
(d) S. H. Gwon and S. G. Kim, Tetrahedron : Asymmetry, 2012, 23, 1251–1255 CrossRef CAS;
(e) A. S. K. Tsang, P. Jensen, J. M. Hook, A. S. K. Hashmi and M. H. Todd, Pure Appl. Chem., 2011, 83, 655–665 CAS;
(f) D. Ramesh, U. Ramulu, S. Rajaram, P. Prabhakar and Y. Venkateswarlu, Tetrahedron Lett., 2010, 51, 4898–4903 CrossRef CAS;
(g) L. Y. Zhai, R. Shukla, S. H. Wadumethrige and R. Rathore, J. Org. Chem., 2010, 75, 4748–4760 CrossRef CAS PubMed;
(h) X. G. Liu, Z. L. Meng, C. K. Li, H. X. Lou and L. Liu, Angew. Chem., Int. Ed., 2015, 54, 6012–6015 CrossRef CAS PubMed;
(i) X. G. Liu, S. T. Sun, Z. L. Meng, H. X. Lou and L. Liu, Org. Lett., 2015, 17, 2396–2399 CrossRef CAS PubMed;
(j) Z. L. Meng, S. T. Sun, H. Q. Yuan, H. X. Lou and L. Liu, Angew. Chem., Int. Ed., 2014, 53, 543–547 CrossRef CAS PubMed.
- Selected literatures for the formation of C–X (X = C, N) bonds via CDC reactions using hypervalent iodine reagents as oxidant, see:
(a) A. P. Antonchick and L. Burgmann, Angew. Chem., Int. Ed., 2013, 52, 3267–3271 CrossRef CAS PubMed;
(b) K. Morimoto, T. Dohi and Y. Kita, Eur. J. Org. Chem., 2013, 1659–1662 CrossRef CAS;
(c) K. Matcha and A. P. Antonchick, Angew. Chem., Int. Ed., 2013, 52, 2082–2086 CrossRef CAS PubMed;
(d) J. W. Wang, Y. C. Yuan, R. Xiong, D. Zhang-Negrerie, Y. F. Du and K. Zhao, Org. Lett., 2012, 14, 2210–2213 CrossRef CAS PubMed;
(e) T. Dohi, M. Ito, I. Itani, N. Yamaoka, K. Morimoto, H. Fujioka and Y. Kita, Org. Lett., 2011, 13, 6208–6211 CrossRef CAS PubMed;
(f) K. Morimoto, N. Yamaoka, C. Ogawa, T. Nakae, H. Fujioka, T. Dohi and Y. Kita, Org. Lett., 2010, 12, 3804–3807 CrossRef CAS PubMed.
- Selected literatures for the formation of C–X (X = C, N) bonds via CDC reactions using O2 as oxidant, see:
(a) H. Ueda, K. Yoshida and H. Tokuyama, Org. Lett., 2014, 16, 4194–4197 CrossRef CAS PubMed;
(b) Á. Pintér, A. Sud, D. Sureshkumar and M. Klussmann, Angew. Chem., Int. Ed., 2010, 49, 5004–5007 CrossRef PubMed;
(c) B. Schweitzer-Chaput, A. Sud, Á. Pintér, S. Dehn, P. Schulze and M. Klussmann, Angew. Chem., Int. Ed., 2013, 52, 13228–13232 CrossRef CAS PubMed;
(d) C. D. Huo, Y. Yuan, M. X. Wu, X. D. Jia, X. C. Wang, F. A. Chen and J. Tang, Angew. Chem., Int. Ed., 2014, 53, 13544–13547 CrossRef CAS PubMed;
(e) J. Liu, F. Liu, Y. Z. Zhu, X. G. Ma and X. D. Jia, Org. Lett., 2015, 17, 1409–1412 CrossRef CAS PubMed;
(f) X. D. Jia, Y. Z. Zhu, Y. Yuan, X. W. Zhang, S. W. Lv, L. Zhang and L. L. Luo, ACS Catal., 2016, 6, 6033–6036 CrossRef CAS;
(g) X. D. Jia, F. F. Peng, C. Qing, C. D. Huo and X. C. Wang, Org. Lett., 2012, 14, 4030–4033 CrossRef CAS PubMed.
- Selected literatures for the formation of C–X (X = C, N) bonds via CDC reactions using KOtBu–DMF as reagents see:
(a) W. T. Wei, X. J. Dong, S. Z. Nie, Y. Y. Chen, X. J. Zhang and M. Yan, Org. Lett., 2013, 15, 6018–6021 CrossRef CAS PubMed;
(b) Y. Y. Chen, X. J. Zhang, H. M. Yuan, W. T. Wei and M. Yan, Chem. Commun., 2013, 49, 10974–10976 RSC.
- Selected literatures for CBr4 as organocatalyst, see:
(a) P. R. Schreiner, O. Lauenstein, I. V. Kolomitsyn, S. Nadi and A. A. Fokin, Angew. Chem., Int. Ed., 1998, 37, 1895–1897 CrossRef CAS;
(b) M. Y. Chen and A. S. Y. Lee, J. Org. Chem., 2002, 67, 1384–1387 CrossRef CAS PubMed;
(c) Y. L. Zhong, J. Lee, R. A. Reamer and D. Askin, Org. Lett., 2004, 6, 929–931 CrossRef CAS PubMed;
(d) M. T. Crimmins and K. A. Emmitte, Org. Lett., 1999, 1, 2029–2032 CrossRef CAS PubMed;
(e) J. Wu, W. Sun, X. Sun and H. G. Xia, Green Chem., 2006, 8, 365–367 RSC;
(f) M. Y. Chen, K. C. Lu, A. S. Y. Lee and C. C. Lin, Tetrahedron Lett., 2002, 43, 2777–2780 CrossRef CAS;
(g) A. S. Y. Lee, Y. J. Hu and S. F. Chu, Tetrahedron, 2001, 57, 2121–2126 CrossRef;
(h) L. Zhang, Y. Luo, R. Fan and J. Wu, Green Chem., 2007, 9, 1022–1025 RSC;
(i) T. Sugai and A. Itoh, Tetrahedron Lett., 2007, 48, 9096–9099 CrossRef CAS;
(j) S. Hirashima, T. Nobuta, N. Tada, T. Miura and A. Itoh, Org. Lett., 2010, 12, 3645–3647 CrossRef CAS PubMed;
(k) T. Keshari, V. P. Srivastavaa and L. D. S. Yadav, RSC Adv., 2014, 4, 5815–5818 RSC.
- A. S. Y. Lee and F. Y. Su, Tetrahedron Lett., 2005, 46, 6305–6309 CrossRef CAS.
- J. S. Yadav, B. V. S. Reddy, K. Harikishan, C. Madan and A. V. Narsaiah, Synthesis, 2005, 2897–2900 CrossRef CAS.
- C. D. Huo and T. H. Chan, Adv. Synth. Catal., 2009, 351, 1933–1938 CrossRef CAS.
- C. D. Huo, C. G. Sun, C. Wang, X. D. Jia and W. J. Chang, ACS Sustainable Chem. Eng., 2013, 1, 549–553 CrossRef CAS.
- Q. Q. Yang, M. Marchini, W. J. Xiao, P. Ceroni and M. Bandini, Chem. – Eur. J., 2015, 21, 18052–18056 CrossRef CAS PubMed.
- S. I. Hirashima, T. Nobuta, N. Tada, T. Miura and A. Itoh, Org. Lett., 2010, 12, 3645–3647 CrossRef CAS PubMed.
- Selected literatures for CBr4 as cross-dehydrogenation coupling reagent, see:
(a) J. Tan, F. S. Liang, Y. M. Wang, X. Cheng, Q. Liu and H. J. Yuan, Org. Lett., 2008, 10, 2485–2488 CrossRef CAS PubMed;
(b) X. X. Liu, J. H. Pu, X. L. Luo, X. F. Cui, Z. Y. Wu and G. S. Huang, Org. Chem. Front., 2018, 5, 361–365 RSC;
(c) Z. C. Shen, P. Yang and Y. Tang, J. Org. Chem., 2016, 81, 309–317 CrossRef CAS PubMed;
(d) C. D. Huo, H. S. Xie, M. X. Wu, X. D. Jia, X. C. Wang, F. J. Chen and J. Tang, Chem. – Eur. J., 2015, 21, 5723–5726 CrossRef CAS PubMed;
(e) F. J. Chen, Y. J. Wang, S. J. Zhao, W. Jiang and C. D. Huo, Org. Biomol. Chem., 2017, 15, 7710–7714 RSC;
(f) J. Tang, S. J. Zhao, Y. Y. Wei, Z. J. Quan and C. D. Huo, Org. Biomol. Chem., 2017, 15, 1589–1592 RSC;
(g) C. D. Huo, H. S. Xie, F. J. Chen, J. Tang and Y. J. Wang, Adv. Synth. Catal., 2016, 358, 724–730 CrossRef CAS;
(h) C. D. Huo, M. X. Wu, F. J. Chen, X. D. Jia, Y. Yuan and H. S. Xie, Chem. Commun., 2015, 51, 4708–4711 RSC;
(i) C. D. Huo, J. Tang, H. S. Xie, Y. J. Wang and J. Dong, Org. Lett., 2016, 18, 1016–1019 CrossRef CAS PubMed;
(j) X. Q. Zhou, H. J. Ma, C. Shi, Y. X. Zhang, X. X. Liu and G. S. Huang, Eur. J. Org. Chem., 2017, 237–240 CrossRef CAS;
(k) X. G. Zhang, P. Wu, Y. J. Fu, F. D. Zhang and B. H. Chen, Tetrahedron Lett., 2017, 58, 870–873 CrossRef CAS.
- Y. Ohta, Y. Tokimizu, S. Oishi, N. Fujii and H. Ohno, Org. Lett., 2010, 12, 3963–3965 CrossRef CAS PubMed.
- B. Ma, Y. Wang, J. L. Peng and Q. Zhu, J. Org. Chem., 2011, 76, 6362–6366 CrossRef CAS PubMed.
-
(a) E. D. Anderson and D. L. Boger, J. Am. Chem. Soc., 2011, 133, 12285–12292 CrossRef CAS PubMed;
(b) E. D. Anderson and D. L. Boger, Org. Lett., 2011, 13, 2492–2494 CrossRef CAS PubMed.
- G. M. Castanedo, P. S. Seng, N. Blaquiere, S. Trapp and S. T. Staben, J. Org. Chem., 2011, 76, 1177–1179 CrossRef CAS PubMed.
- J. S. Peng, M. Ye, C. J. Zong, F. Y. Hu, L. T. Feng, X. Y. Wang, Y. F. Wang and C. X. Chen, J. Org. Chem., 2011, 76, 716–719 CrossRef CAS PubMed.
- Selected literatures for the synthesis of 1,2,4-triazoles from N-(2-pyridyl)amidines substrates, see:
(a) S. Ueda and H. Nagasawa, J. Am. Chem. Soc., 2009, 131, 15080–15081 CrossRef CAS PubMed;
(b) Z. S. Zheng, S. Y. Ma, L. L. Tang, D. Zhang-Negrerie, Y. F. Du and K. Zhao, J. Org. Chem., 2014, 79, 4687–4693 CrossRef CAS PubMed;
(c) V. J. Grenda, R. E. Jones, G. Gal and M. Sletzinger, J. Org. Chem., 1965, 30, 259–261 CrossRef CAS;
(d) K. T. Potts, H. R. Burton and J. Bhattacharyya, J. Org. Chem., 1966, 31, 260–265 CrossRef CAS;
(e) J. D. Bower and G. R. Ramage, J. Chem. Soc., 1957, 4506–4510 RSC;
(f) L. N. Song, X. H. Tian, Z. G. Lv, E. T. Li, J. Wu, Y. X. Liu, W. Q. Yu and J. B. Chang, J. Org. Chem., 2015, 80, 7219–7225 CrossRef CAS PubMed;
(g) X. Meng, C. Yu and P. Zhao, RSC Adv., 2014, 4, 8612–8616 RSC.
- X. H. Tian, L. Song, M. M. Wang, Z. G. Lv, J. Wu, W. Q. Yu and J. B. Chang, Chem. – Eur. J., 2016, 22, 7617–7622 CrossRef CAS PubMed.
-
(a) F. P. Yi, Q. H. Sun, J. Sun, C. Fu and W. Y. Yi, J. Org. Chem., 2019, 81, 6780–6787 CrossRef PubMed;
(b) Y. Huang, W. Y. Yi, Q. H. Sun and F. P. Yi, Adv. Synth. Catal., 2018, 360, 3074–3082 CrossRef CAS;
(c) Y. Huang, W. Y. Yi, Q. H. Sun, L. R. Zhang and F. P. Yi, RSC Adv., 2018, 8, 74–79 RSC;
(d) F. P. Yi, S. X. Zhang, Y. Huang, L. R. Zhang and W. Y. Yi, Eur. J. Org. Chem., 2017, 102–110 CrossRef CAS.
- Selective literatures for the Synthesis of amidines by Cu-catalyzed MCRs.
(a) I. Bae, H. Han and S. Chang, J. Am. Chem. Soc., 2005, 127, 2038–2039 CrossRef CAS PubMed;
(b) S. L. Cui, J. Wang and Y. G. Wang, Org. Lett., 2008, 10, 1267–1269 CrossRef CAS PubMed;
(c) J. J. Wang, P. Lu and Y. G. Wang, Org. Chem. Front., 2015, 2, 1346–1351 RSC;
(d) D. P. Chauhan, S. J. Varma, A. Vijeta, P. Banerjee and P. Talukdar, Chem. Commun., 2014, 50, 323–325 RSC;
(e) D. Zhang, I. Nakamura and M. Terada, Org. Lett., 2014, 16, 5184–5187 CrossRef CAS PubMed;
(f) W. Choi, J. Kim, T. Ryu, K. B. Kim and P. H. Lee, Org. Lett., 2015, 17, 3330–3333 CrossRef CAS PubMed;
(g) J. J. Wang, J. Y. Liu, H. L. Ding, J. Wang, P. Lu and Y. G. Wang, J. Org. Chem., 2015, 80, 5842–5850 CrossRef CAS PubMed;
(h) J. Kim, Y. Lee, J. Lee, Y. Do and S. Chang, J. Org. Chem., 2008, 73, 9454–9457 CrossRef CAS PubMed and the cited references.
Footnote |
† Electronic supplementary information (ESI) available: Detailed experimental procedures, characterization data, and 1H NMR, 13C NMR and X-ray spectra of final products (PDF). CCDC 1883380. For ESI and crystallographic data in CIF or other electronic format see DOI: 10.1039/c9ra09265j |
|
This journal is © The Royal Society of Chemistry 2019 |
Click here to see how this site uses Cookies. View our privacy policy here.