DOI:
10.1039/C9SC03175H
(Edge Article)
Chem. Sci., 2019,
10, 9099-9103
Cu-catalyzed oxygenation of alkene-tethered amides with O2via unactivated C
C bond cleavage: a direct approach to cyclic imides†
Received
27th June 2019
, Accepted 4th August 2019
First published on 6th August 2019
5- and 6-membered cyclic imide moieties are frequently present as the key subunit in many pharmaceuticals and bioactive compounds1 (Fig. 1). For instance, 5-membered cyclic imides (succinimides) i, ii and iii are commonly used to treat petit mal epilepsy,2 while 6-membered cyclic imides (glutarimides) iv, v, and vi could be used as sedative-hypnotics3 and antineoplastic and immunomodulatory drugs.4 Interestingly, the penitential thalidomide vi returned to the market for the treatment of cancer under the brand name Immunoprin,5 since the crisis of thalidomide in the 1960s.6 The evolution of synthetic methods for cyclic imides is continuously driven forward by their importance in medicinal chemistry. Typical methods for the synthesis of simple imides include the ammonolysis of anhydrides at high temperature,7 the oxidation of lactams with strong or special oxidants,8 the reduction of unsaturated imides such as maleimide,9 and metal-catalyzed carbonylation of various precursors.10 However, the approach to polysubstituted cyclic imides remains challenging due to tedious transformations that are required for the preparation of anhydride precursors.
 |
| Fig. 1 Some pharmaceuticals with succinimide and glutarimide moieties. | |
It is instructive that the difunctionalization of alkenes is such a versatile transformation for the assembly of two individual functional groups across C
C double bonds.11 Alkene-tethered amides, which serve as the substrates for intramolecular amidation of unactivated alkenes initiated by the photo-induced amidyl radical formation or metal-mediated amidocyclization, have emerged as the ideal precursor of γ-lactams in recent years with well-established transformations12 (Scheme 1(a1)).
 |
| Scheme 1 The strategies for the oxygenation of unactivated alkenyl amides. | |
Recently, O2 or air has been regarded as an ideal oxidant because of its inexpensive, environmentally benign and high atom economy characteristics, which attract academic and industrial attention.13 The oxygenations of olefins enable efficient protocols for the construction of epoxides,14 diols,15 and ketones16 with/without C
C double bond cleavage.17 Given what we discovered in our previous studies on the oxygen mediated C
C bond cleavage,18 we proposed that the geminal olefin amino-oxygenation of pent-4-enamides via chemoselective C
C double bond cleavage would be highly promising to produce succinimides in the presence of oxygen (Scheme 1b). To date, the aerobic oxidation of enamides has only been reported in the Pd(II)-catalyzed intramolecular aza-Wacker-type cyclization.19 Recently, significant aminooxygenation of 4-pentenylsulfonamides was reported by Chemler and coworkers (Scheme 1(a2)),20 in which the C
C bond cleavage was successfully achieved but in two steps. DABCO was required as a base with the formation of γ-lactam products. To the best of our knowledge, the chemoselective cleavage of C
C double bonds in alkene-tethered amides for cyclic imide synthesis has not been accomplished yet.
Our investigation commenced with N-methoxy alkenyl amide 1a. After a lot of experiments, we were surprised to find that the unactivated C
C double bond could be cleaved with the incorporation of one oxygen atom using O2. Encouraged by the copper catalyzed olefin amino-oxygenation which delivered 2a in 47% yield (Table 1, entry 1), a variety of conditions were screened (see the ESI†). The control experiments demonstrated that the reaction could not work in the absence of the copper catalyst, or oxygen atmosphere (Table 1, entries 2 and 3). Besides, different copper catalysts, solvents, additives and ligands were also screened (Table 1, entries 5–10). The yields sharply decreased when bases or acids were used as additives. Finally, we found that with copper acetate as the catalyst and bathocuproine (Ligand II) as the ligand the unactivated C
C double bond geminal amino-oxygenation reaction in toluene proceeded well and produced the desired succinimide product 2a with excellent efficiency (83% isolated yield, Table 1, entry 10).
Table 1 Screening of reaction conditionsa
Reaction conditions: 1a (0.2 mmol), catalyst (0.02 mmol), ligand (0.024 mmol) and additive (1.0 equiv.) were stirred in solvent (2.0 mL) at 80 °C under O2.
Determined by 1H NMR analysis using 1,1,2,2-tetrachloroethane as the internal standard. The numbers in parentheses are isolated yields.
Under an argon atmosphere.
Not detected.
This reaction was carried out under air.
|
|
Subsequently, a good number of pent-4-enamides were smoothly converted to succinimides in moderate to good yields (Table 2). Several N-alkoxy protecting groups were well tolerated (up to 84%, 2a–2c) while substrates bearing bulky groups showed poor conversion (2d and 2e). When a hydrogen atom (2f) or benzyl group (2g) was attached to the amide nitrogen, the reaction did not work. The reason is that the alkyl-metal intermediate formation might be favored with the assistance of alkoxy protecting groups.12d,e α-Geminal substituted substrates worked well in this transformation (Table 2, 2h–2o), producing polysubstituted and spiro-succinimides in moderate to good yields. It is noteworthy that the reaction could contain one of the identical allyl groups specifically to give the allylic imide in 44% yield (2o). The mono-methyl or benzyl substituted enamides were also tolerated, and the desired products could be obtained in fair yields (2p and 2q). To our delight, the vinylcyclohexane derived enamide underwent the process smoothly to afford the corresponding imide 2s. 2-Vinylbenzamide was also compatible to give the synthetically important phthalimide 2t albeit the efficiency is a little bit low because the conjugated alkenes would undergo unwanted oxidation. Unfortunately, the alkene-tethered amide without alkylation of the backbone did not work. Notably, the glutarimide derivatives 4a–4d were also obtained in moderate yields with hex-5-enamides (Table 3).
Table 2 Substrate scope for the synthesis of succinimidesa
Reaction conditions: see entry 10, Table 1. Isolated yields.
Reaction for 48 hours.
|
|
Table 3 Substrate scope for the synthesis of glutarimidesa
Reaction conditions: see entry 10, Table 1. Isolated yields.
|
|
To demonstrate the synthetic value of our strategy, several late-stage modifications of biologically active compounds were carried out under standard conditions (Scheme 2).
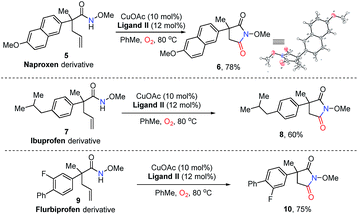 |
| Scheme 2 Application in the synthesis of drug analogues. | |
Naproxen, approved by the USA Food and Drug Administration (FDA) as an anti-inflammatory, antipyretic and analgesic agent, could deliver succinimide 6 in 78% yield via analogue 5 under standard conditions (Scheme 2). The structure of 6 was confirmed by X-ray single crystal structural analysis. Additionally, the derivatives of best-selling drugs ibuprofen and flurbiprofen could also undergo the present transformation to afford the desired product 8 in 60% yield and 10 in 75% yield respectively. These results provide efficient approaches to drug analogues for future medicinal chemistry studies.
Furthermore, our strategy can be applied to the synthesis of two pharmaceutical compounds ethosuximide i and methsuximide ii (Fig. 1). As shown in Scheme 3, the amidocyclization of 11 gave N-benzyloxy succinimide 12 in 69% yield under standard conditions, followed by the removal of the N-benzyloxy group by hydrogenation and treatment with 2-bromoacetophenone and triethylamine to furnish21 the ethosuximide in high yield (13, 83%), which possesses antiepileptic effects. This method avoids the use of highly toxic hydrocyanic acid in industrial production. Similarly, the methsuximide 17 could also be obtained from succinimide 15 in good overall yield.
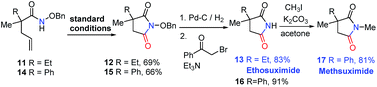 |
| Scheme 3 Synthesis of succinimide-containing medicines. | |
In order to probe the mechanism, some control experiments were designed and investigated (Scheme 4). Firstly, the reaction was conducted in the presence of TEMPO as a radical scavenger, and the difunctionalization product 18 could be obtained in 87% yield, with the formation of 2a in only 10% yield (Scheme 4a). This result indicates that an alkyl radical intermediate was produced after the intramolecular amido-cyclization process. Then, to investigate the intermediacy of an aldehyde in the C
C bond cleavage, 2-pyrrolidinone 19 was employed under standard conditions. The formation of 2r with some unconsumed raw materials compared with the results in Table 2 indicates that an aldehyde might be involved in this transformation (Scheme 4b). In addition, the isotopic labeling studies under 18O2 delivered the labeled succinimide [18O]-2a in 80% yield (67% 18O) due to the exchange with H2O (see the ESI†), which supports our expectation. We also studied the reaction kinetic profile, which showed the initial increase and later consumption of the aldehyde intermediate along with the formation of succinimide (Fig. 2). This result was in accordance with our aforementioned observation. Tentative studies on trapping intermediates were also carried out by EPR (see the ESI†).
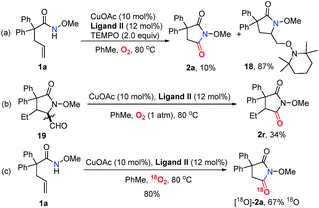 |
| Scheme 4 Mechanistic studies. | |
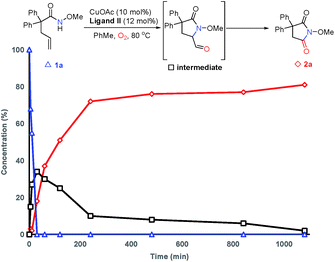 |
| Fig. 2 Kinetic profile of the C C bond oxygenation reaction. | |
Based on previously reported12e,20 and our own mechanistic studies, a plausible mechanism is shown in Scheme 5. We proposed that copper(I) is oxidized to copper(II) by O2 in the initial step. Then, copper(II)-catalyzed alkene cis-amidocupration affords an unstable organocopper(II) intermediate B. Primary radical C, which could be trapped by TEMPO (Scheme 4a), is subsequently generated by the C–Cu homolysis of the intermediate B.22 The mechanism is not completely clear yet. Alternatively, the lack of detection of the amidyl radical by EPR analysis (see the ESI†) could not fully disprove its presence under the reaction conditions. The primary radical C may also be generated by the addition of the amidyl radical to the double bond. Then, the radical species C is trapped by molecular oxygen and produces the superoxide radical D. Then, the intramolecular 1,3-hydrogen migration occurs to form the intermediate E, followed by the O–O homolysis to give the aldehyde F and hydroxyl radical which is unstable and easily reduced in situ to give the hydroxide anion. The intermediate aldehyde F could be directly transformed into copper(II) enolate G which undergoes formal [2 + 2] cycloaddition with another molecule of oxygen to give the 1,2-dioxetane Jvia radical species H and the cyclic peroxo intermediate I.23 Then the ring opening process occurs to form the succinimide 2a and release carbon monoxide.
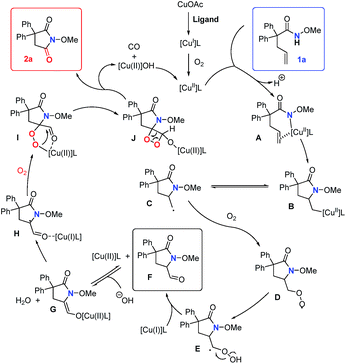 |
| Scheme 5 Proposed mechanism of the reaction. | |
In summary, we developed a novel molecular oxygen mediated geminal amino-oxygenation of unactivated olefins in alkene-tethered amides via a chemoselective cyclization/C
C bond cleavage processes that revealed an efficient approach to polysubstituted succinimides and glutarimides. Our reaction exhibited good functional group tolerance under simple conditions. The success of this protocol in the late-stage modification of biologically active compounds and the synthesis of pharmaceuticals would motivate further exploration of the transformations of unactivated alkenes.
Conflicts of interest
The authors declare no competing financial interest.
Acknowledgements
Financial support from the National Basic Research Program of China (973 Program) (No. 2015CB856600), the National Natural Science Foundation of China (21632001, 21772002, and 81821004), the Drug Innovation Major Project (2018ZX09711-001), and the Open Research Fund of Shanghai Key Laboratory of Green Chemistry and Chemical Processes is greatly appreciated. We thank Xinyi Zhao of our group for reproducing the results of 2n and 4b.
Notes and references
-
(a) A. M. Crider, T. M. Kolczynski and K. M. Yates, J. Med. Chem., 1980, 23, 324 CrossRef CAS PubMed;
(b) E. D. Deeks, Drugs, 2015, 75, 1393 CrossRef CAS PubMed.
- L. Sorel, Acta Neurol. Psychiatr. Belg., 1960, 60, 551 CAS.
-
(a) H. Keberle, K. Hoffmann and K. Bernhard, Experientia, 1962, 18, 105 CrossRef CAS PubMed;
(b) R. Kato and P. Vassanelli, Biochem. Pharmacol., 1962, 11, 779 CrossRef CAS PubMed.
- R. J. Santen, S. Santner, B. Davis, J. Veldhuis, E. Samojlik and E. Ruby, J. Clin. Endocrinol. Metab., 1978, 47, 1257 CrossRef CAS PubMed.
-
(a) S. K. Kumar, S. V. Rajkumar, A. Dispenzieri, M. Q. Lacy, S. R. Hayman, F. K. Buadi, S. R. Zeldenrust, D. Dingli, S. J. Russell, J. A. Lust, P. R. Greipp, R. A. Kyle and M. A. Gertz, Blood, 2008, 111, 2516 CrossRef CAS PubMed;
(b) A. Palumbo and K. Anderson, N. Engl. J. Med., 2011, 364, 1046 CrossRef CAS PubMed.
-
(a) W. G. Mcbride, Lancet, 1961, 278, 1358 CrossRef;
(b) W. Lenz, R. A. Pfeiffer, W. Kosenow and D. J. Hayman, Lancet, 1962, 279, 45 CrossRef.
-
(a) T. Katoh, K. Nishide, M. Node and H. Ogura, Heterocycles, 1999, 50, 833 CrossRef CAS;
(b) C. G. Overberger, D. W. Wang, R. K. Hill, G. R. Krow and D. W. Ladner, J. Org. Chem., 1981, 46, 2757 CrossRef CAS;
(c) M. A. Ali, S. K. Moromi, A. S. Touchy and K. Shimizu, ChemCatChem, 2016, 8, 891 CrossRef CAS;
(d) Y. Liu, J. Fu, D. Ren, Z. Song, F. Jin and Z. Huo, ChemistrySelect, 2018, 3, 724 CrossRef CAS;
(e) J. Obniska, K. Kaminski, D. Skrzynska and J. Pichor, Eur. J. Med. Chem., 2009, 44, 2224 CrossRef CAS PubMed.
-
(a) Y. Zhang, D. Riemer, W. Schilling, J. Kollmann and S. Das, ACS Catal., 2018, 8, 6659 CrossRef CAS;
(b) R. Ito, N. Umezawa and T. Higuchi, J. Am. Chem. Soc., 2005, 127, 834 CrossRef CAS PubMed;
(c) C. Liu, Q. Lu, Z. Huang, J. Zhang, F. Liao, P. Peng and A. Lei, Org. Lett., 2015, 17, 6034 CrossRef CAS PubMed;
(d) C. Annese, L. D'Accolti, C. Fusco, G. Licini and C. Zonta, Chem.–Eur. J., 2017, 23, 259 CrossRef CAS PubMed;
(e) A. Maji, A. Hazra and D. Maiti, Org. Lett., 2014, 16, 4524 CrossRef CAS PubMed.
-
(a) M. Bayat and J. M. Fox, J. Heterocycl. Chem., 2016, 53, 1661 CrossRef CAS;
(b) Y.-J. Liu, X.-Q. Pei, H. Lin, P. Gai, Y.-C. Liu and Z.-L. Wu, Appl. Microbiol. Biotechnol., 2012, 95, 635 CrossRef CAS PubMed;
(c) C. Metallinos, J. Zaifman, L. V. Belle and L. Dodge, Organometallics, 2009, 28, 4534 CrossRef CAS.
-
(a) K. M. Driller, H. Klein, R. Jackstell and M. Beller, Angew. Chem., Int. Ed., 2009, 48, 6041 CrossRef CAS PubMed;
(b) P. Williamson, A. Galván and M. J. Gaunt, Chem. Sci., 2017, 8, 2588 RSC;
(c) L. Zeng, H. Li, S. Tang, X. Gao, Y. Deng, G. Zhang, C.-W. Pao, J.-L. Chen, J.-F. Lee and A. Lei, ACS Catal., 2018, 8, 5448 CrossRef CAS.
-
(a) G. Yin, X. Mu and G. Liu, Acc. Chem. Res., 2016, 49, 2413 CrossRef CAS PubMed;
(b) Z.-L. Li, X.-H. Li, N. Wang, N.-Y. Yang and X.-Y. Liu, Angew. Chem., Int. Ed., 2016, 55, 15100 CrossRef CAS PubMed;
(c) X. Qi, F. Yu, P. Chen and G. Liu, Angew. Chem., Int. Ed., 2017, 56, 12692 CrossRef CAS PubMed;
(d) A. G. Dalling, T. Yamauchi, N. G. McCreanor, L. Cox and J. F. Bower, Angew. Chem., Int. Ed., 2019, 58, 221 CrossRef CAS PubMed;
(e) D. Zheng and A. Studer, Org. Lett., 2019, 21, 325 CrossRef CAS PubMed;
(f) B. Yang, X. Ren, X. Shen, T. Li and Z. Lu, Chin. J. Chem., 2018, 36, 1017 CrossRef CAS;
(g) H. Mei, Z. Yin, J. Liu, H. Sun and J. Han, Chin. J. Chem., 2019, 37, 292 CAS;
(h) X. Li, S. Song and N. Jiao, Acta Chim. Sin., 2017, 75, 1202 CrossRef.
-
(a) S. Nicolai, C. Piemontesi and J. Waser, Angew. Chem., Int. Ed., 2011, 50, 4680 CrossRef CAS PubMed;
(b) G. J. Choi and R. R. Knowles, J. Am. Chem. Soc., 2015, 137, 9226 CrossRef CAS PubMed;
(c) K. Shen and Q. Wang, Org. Chem. Front., 2016, 3, 222 RSC;
(d) K. Shen and Q. Wang, Chem. Sci., 2015, 6, 4279 RSC;
(e) K. Shen and Q. Wang, J. Am. Chem. Soc., 2017, 139, 13110 CrossRef CAS PubMed;
(f) Z. Li, L. Song and C. Li, J. Am. Chem. Soc., 2013, 135, 4640 CrossRef CAS PubMed;
(g) R. Abrams, Q. Lefebvre and J. Clayden, Angew. Chem., Int. Ed., 2018, 57, 13587 CrossRef CAS PubMed;
(h) J. Conway Jr and H. T. Rovis, J. Am. Chem. Soc., 2018, 140, 135 CrossRef PubMed;
(i) J. Derosa, R. Kleinmans, V. T. Tran, M. K. Karunananda, S. R. Wisniewski, M. D. Eastgate and K. M. Engle, J. Am. Chem. Soc., 2018, 140, 17878 CrossRef CAS PubMed;
(j) S. Zheng, Á. Gutiérrez-Bonet and G. A. Molander, Chem, 2019, 5, 339 CrossRef CAS PubMed;
(k) S. A. Shuler, G. Yin, S. B. Krause, C. M. Vesper and D. A. Watson, J. Am. Chem. Soc., 2016, 138, 13830 CrossRef CAS PubMed;
(l) F. Xu, S. A. Shuler and D. A. Watson, Angew. Chem., Int. Ed., 2018, 57, 12081 CrossRef CAS PubMed;
(m) P. Xiong, H.-H. Xu and H.-C. Xu, J. Am. Chem. Soc., 2017, 139, 2956 CrossRef CAS PubMed.
- For reviews, see:
(a) M. S. Sigman and D. R. Jensen, Acc. Chem. Res., 2006, 39, 221 CrossRef CAS PubMed;
(b) T. Punniyamurthy, S. Velusamy and J. Iqbal, Chem. Rev., 2005, 105, 2329 CrossRef CAS PubMed;
(c) A. E. Wendlandt, A. M. Suess and S. S. Stahl, Angew. Chem., Int. Ed., 2011, 50, 11062 CrossRef CAS PubMed;
(d) Z. Shi, C. Zhang, C. Tang and N. Jiao, Chem. Soc. Rev., 2012, 41, 3381 RSC;
(e) W. Wu and H. Jiang, Acc. Chem. Res., 2012, 45, 1736 CrossRef CAS PubMed;
(f) S. E. Allen, R. R. Walvoord, R. Padilla-Salinas and M. C. Kozlowski, Chem. Rev., 2013, 113, 6234 CrossRef CAS PubMed;
(g) J. Serrano-Plana, I. Garcia-Bosch, A. Company and M. Costas, Acc. Chem. Res., 2015, 48, 2397 CrossRef CAS PubMed;
(h) L. Boisvert and K. I. Goldberg, Acc. Chem. Res., 2012, 45, 899 CrossRef CAS PubMed;
(i) Y.-F. Liang and J. Ning, Acc. Chem. Res., 2017, 50, 1640 CrossRef CAS PubMed;
(j) D. Wang, A. B. Weinstein, P. B. White and S. S. Stahl, Chem. Rev., 2018, 118, 2636 CrossRef CAS PubMed;
(k) K. P. Bryliakov, Chem. Rev., 2017, 117, 11406 CrossRef CAS PubMed;
(l) X. Li and N. Jiao, Chin. J. Chem., 2017, 35, 1349 CrossRef CAS.
-
(a) J. S. Hess, S. Leelasubcharoen, A. L. Rheingold, D. J. Doren and K. H. Theopold, J. Am. Chem. Soc., 2002, 124, 2454 CrossRef CAS PubMed;
(b) H. Tanaka, H. Nishikawa, T. Uchida and T. Katsuki, J. Am. Chem. Soc., 2010, 132, 12034 CrossRef CAS PubMed;
(c) M. Tada, S. Muratsugu, M. Kinoshita, T. Sasaki and Y. Iwasawa, J. Am. Chem. Soc., 2010, 132, 713 CrossRef CAS PubMed;
(d) K. Schröder, B. Join, A. J. Amali, K. Junge, X. Ribas, M. Costas and M. Beller, Angew. Chem., Int. Ed., 2011, 50, 1425 CrossRef PubMed;
(e) S. Koya, Y. Nishioka, H. Mizoguchi, T. Uchida and T. Katsuki, Angew. Chem., Int. Ed., 2012, 51, 8243 CrossRef CAS PubMed.
-
(a) R. Raja, G. Sankar and J. M. Thomas, Chem. Commun., 1999, 829 RSC;
(b) B. Yang and Z. Lu, Chem. Commun., 2017, 53, 12634 RSC;
(c) A. Wang and H. Jiang, J. Org. Chem., 2010, 75, 2321 CrossRef CAS PubMed;
(d) C. Döbler, G. M. Mehltretter, U. Sundermeier and M. Beller, J. Am. Chem. Soc., 2000, 122, 10289 CrossRef;
(e) D. R. Boyd, N. D. Sharma, N. I. Bowers, I. N. Brannigan, M. R. Groocock, J. F. Malone, G. McConville and C. C. R. Allen, Adv. Synth. Catal., 2005, 347, 1081 CrossRef CAS;
(f) C. Dobler, G. Mehltretter and M. Beller, Angew. Chem., Int. Ed., 1999, 38, 3026 CrossRef CAS.
-
(a) R. S. Drago, B. B. Corden and C. W. Barnes, J. Am. Chem. Soc., 1986, 108, 2453 CrossRef CAS PubMed;
(b) Y. Deng, X. J. Wei, H. Wang, Y. Sun, T. Noel and X. Wang, Angew. Chem., Int. Ed., 2017, 56, 832 CrossRef CAS PubMed;
(c) A. Fujiya, A. Kariya, T. Nobuta, N. Tada, T. Miura and A. Itoh, Synlett, 2014, 25, 884 CrossRef CAS;
(d) X. Baucherel, J. Uziel and S. Jugé, J. Org. Chem., 2001, 66, 4504 CrossRef CAS PubMed;
(e) S. Agasti, A. Dey and D. Maiti, Chem. Commun., 2016, 52, 12191 RSC;
(f) B. Xiong, X. Zeng, S. Geng, S. Chen, Y. He and Z. Feng, Green Chem., 2018, 20, 4521 RSC;
(g) X. Jiang, Y. Zhai, J. Chen, Y. Han, Z. Yang and S. Ma, Chin. J. Chem., 2018, 36, 15 CrossRef CAS;
(h) W. Liang, Z. Zhang, D. Yi, Q. Fu, S. Chen, L. Yang, F. Du, J. Ji and W. Wei, Chin. J. Chem., 2017, 35, 1378 CrossRef CAS.
- For reviews on the unstrained C–C bond functionalization, see:
(a) C.-H. Jun, Chem. Soc. Rev., 2004, 33, 610 RSC;
(b) F. Chen, T. Wang and N. Jiao, Chem. Rev., 2014, 114, 8613 CrossRef CAS PubMed;
(c) L. Souillart and N. Cramer, Chem. Rev., 2015, 115, 9410 CrossRef CAS PubMed;
(d) X. Wu and C. Zhu, Chin. J. Chem., 2019, 37, 171 CAS;
(e) A. Dermenci, J. W. Coe and G. Dong, Org. Chem. Front., 2014, 1, 567 RSC;
(f) G. Urgoitia, R. SanMartin, M. T. Herrero and E. Domínguez, ACS Catal., 2017, 7, 3050 CrossRef CAS;
(g) P. Sivaguru, Z. Wang, G. Zanoni and X. Bi, Chem. Soc. Rev., 2019, 48, 2615 RSC.
-
(a) T. Wang and N. Jiao, J. Am. Chem. Soc., 2013, 135, 11692 CrossRef CAS PubMed;
(b) R. Lin, F. Chen and N. Jiao, Org. Lett., 2012, 14, 4158 CrossRef CAS.
-
(a) R. M. Trend, Y. K. Ramtohul, E. M. Ferreira and B. M. Stoltz, Angew. Chem., Int. Ed., 2003, 42, 2892 CrossRef CAS PubMed;
(b) G. Yang, C. Shen and W. Zhang, Angew. Chem., Int. Ed., 2012, 51, 9141 CrossRef CAS;
(c) X. Kou, Y. Li, L. Wu, X. Zhang, G. Yang and W. Zhang, Org. Lett., 2015, 17, 5566 CrossRef CAS PubMed;
(d) G. Yang and W. Zhang, Org. Lett., 2012, 14, 268 CrossRef CAS;
(e) K.-T. Yip and D. Yang, Org. Lett., 2011, 13, 2134 CrossRef CAS PubMed;
(f) K.-T. Yip, N.-Y. Zhu and D. Yang, Org. Lett., 2009, 11, 1911 CrossRef CAS;
(g) P. G. Andersson and J.-E. Bäckvall, J. Am. Chem. Soc., 1992, 114, 8696 CrossRef CAS.
- T. Wdowik and S. R. Chemler, J. Am. Chem. Soc., 2017, 139, 9515 CrossRef CAS.
-
(a) M. A. Silvers, G. T. Robertson, C. M. Taylor and G. L. Waldrop, J. Med. Chem., 2014, 57, 8947 CrossRef CAS PubMed;
(b) J. Pohlmann, T. Lampe, M. Shimada, P. G. Nell, J. Pernerstorfer, N. Svenstrup, N. A. Brunner, G. Schiffer and C. Freiberg, Bioorg. Med. Chem. Lett., 2015, 15, 1189 CrossRef PubMed;
(c) D. J. Dixon and S. G. Davies, Chem. Commun., 1996, 1797 RSC;
(d) K. V. Nikitin and N. P. Andryukhova, Mendeleev Commun., 2000, 10, 32 CrossRef.
-
(a) J.-W. Jiao, H.-Y. Bi, P.-S. Zou, Z.-X. Wang, C. Liang and D.-L. Mo, Adv. Synth. Catal., 2018, 360, 3254 CrossRef CAS;
(b) Y. Miller, L. Miao, A. S. Hosseini and S. R. Chemler, J. Am. Chem. Soc., 2012, 134, 12149 CrossRef CAS PubMed;
(c) F. C. Sequeira, B. W. Turnpenny and S. R. Chemler, Angew. Chem., Int. Ed., 2010, 49, 6365 CrossRef CAS PubMed;
(d) A. Bunescu, T. M. Ha, Q. Wang and J. Zhu, Angew. Chem., Int. Ed., 2017, 56, 10555 CrossRef CAS PubMed.
-
(a) J. Cossy, D. Belotti, V. Bellosta and D. Brocca, Tetrahedron Lett., 1994, 35, 6089 CrossRef CAS;
(b) Y. Seki, K. Tanabe, D. Sasaki, Y. Sohma, K. Oisaki and M. Kanai, Angew. Chem., Int. Ed., 2014, 53, 6501 CrossRef CAS.
Footnote |
† Electronic supplementary information (ESI) available. CCDC 1912652. For ESI and crystallographic data in CIF or other electronic format see DOI: 10.1039/c9sc03175h |
|
This journal is © The Royal Society of Chemistry 2019 |
Click here to see how this site uses Cookies. View our privacy policy here.