DOI:
10.1039/C9SC04122B
(Edge Article)
Chem. Sci., 2019,
10, 10601-10606
Cu-catalyzed C–C bond formation of vinylidene cyclopropanes with carbon nucleophiles†
Received
19th August 2019
, Accepted 30th September 2019
First published on 9th October 2019
Abstract
The development of Cu-catalyzed addition of carbon nucleophiles to vinylidene cyclopropanes was reported. The reactions with 1,1-bisborylmethane provided homopropargylic boronate products by forming a C–C bond at the terminal carbon atom of the allene moiety of vinylidene cyclopropanes. Alkynyl boronates are also suitable nucleophile precursors in reactions with vinylidene cyclopropanes, and skipped diynes were obtained in high yields. In addition, the Cu-enolate generated from the initial addition of nucleophilic copper species to vinylidene cyclopropanes can be intercepted by an external electrophile. As such, vinylidene cyclopropane serves as a linchpin to connect a nucleophile and an electrophile by forming two carbon–carbon bonds sequentially.
Introduction
Vinylidene cyclopropanes are a class of molecules that have an allene moiety connected to a cyclopropane.1 Although highly strained (strain energy estimated to be about 50 kcal mol−1),1b vinylidene cyclopropanes are stable at ambient temperature, and can be readily prepared from commercially available materials.1,2 However, under appropriate reaction conditions (Lewis acidic conditions, for example), vinylidene cyclopropanes have been shown to participate in reactions to generate carbocycles and heterocycles that are useful building blocks in organic synthesis.3 Several transition metal-catalyzed transformations of vinylidene cyclopropanes have also been investigated.2,3a–c
Recently, Cu-based nucleophiles, including boron, silicon and hydrogen, have been shown to react with allenes to form allylcopper species.4–7 However, few methods are currently available allowing for catalytic reactions of Cu-based carbon nucleophiles with allenes.8 We developed and report herein our studies on Cu-catalyzed reactions of vinylidene cyclopropanes with carbon nucleophiles generated from 1,1-bisborylmethane and alkynyl boronates to deliver synthetically useful homopropargylic boronate and skipped diyne products.
When considering the reaction pathways of Cu-based carbon nucleophiles with vinylidene cyclopropanes A, two potential scenarios may arise in this process as illustrated in Scheme 1. Based on a plethora of prior studies on copper catalyzed reactions with allenes,4–7 it is anticipated that the addition of nucleophilic copper species [Cu]–R to vinylidene cyclopropane A should occur in a pathway where the R group adds to the central carbon atom of the allene moiety to generate allylcopper intermediate B (pathway 1, shown in blue in Scheme 1). Interception of intermediate B with a source of proton should provide vinyl cyclopropane product C (or an alkylidene cyclopropane product). As such, the cyclopropane ring of A remains intact. Similar reaction pathways have also been observed in Pt-catalyzed hydroboration of vinylidene cyclopropanes.2 On the other hand, it is also conceivable that the migratory insertion step may occur with R group adding to the terminal carbon atom of the allene moiety to form vinylcopper intermediate D (pathway 2, shown in red in Scheme 1).9 Vinylcopper D could undergo a subsequent β-carbo elimination to open the cyclopropane ring to form homopropargylic copper intermediate E, which can react with a proton source to give alkyne product F. At the outset of our studies, it is not clear which pathway will be favoured in reactions with vinylidene cyclopropane A. However, in the case where E1 and E2 substituents in A are electron-withdrawing groups (ester group for instance), we anticipate that pathway 2 (shown in red in Scheme 1) should be more favourable because β-carbo elimination of intermediate (D) will generate a stabilized Cu-enolate (e.g., E). Moreover, releasing the ring strain of cyclopropane in compounds A and D may also serve as a thermodynamic driving force for this reaction pathway.
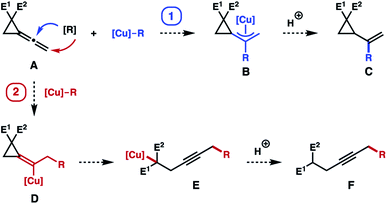 |
| Scheme 1 Potential competing pathways for Cu-catalyzed reactions with vinylidene cyclopropane. | |
Results and discussion
To test our hypothesis, vinylidene cyclopropane 1a bearing two methyl ester groups was synthesized, and reactions with 1,1-bisborylmethane 210 were conducted. It has been shown that 1,1-bisborylmethane 2 readily undergoes transmetalation with a copper catalyst under basic conditions to give a nucleophilic Cu–CH2Bpin species that can react with carbonyl, imine or allylic electrophiles.11,12 However, the nature of base plays a significant role in the transmetalation process. To identify an appropriate base for this transformation, reactions conducted with several tert-butoxide bases were examined first. As shown in Table 1, the experiments were performed with 10 mol% CuCl as the catalyst and 1.1 equiv. base in THF at ambient temperature. The reaction with LiOtBu as the base gave homopropargylic boronate 3a in 37% yield (entry 1, Table 1). When NaOtBu was employed as the base, the yield of boronate product improved significantly, and 3a was isolated in 80% yield (entry 2, Table 1). The reaction performed with KOtBu as the base, however, gave product 3a in only 10% yield (entry 3, Table 1). Reactions with several methoxide bases were examined next. The yield of 3a (48%) in the reaction with LiOMe as the base (entry 4, Table 1) is similar to the one conducted with LiOtBu. The best results were achieved when NaOMe was utilized as the base, and homopropargylic boronate 3a was isolated in 87% yield (entry 5, Table 1). In contrast to the case of KOtBu, boronate 3a was generated in a substantial yield (63% vs. 10%) with KOMe as the base (entry 6, Table 1). The presence of a bidentate phosphine ligand appears to slightly affect the efficiency of the transformation, as the reaction with 10 mol% of xantphos gave product 3a in 65% yield (entry 7, Table 1). The reaction conducted in toluene without the ligand also gave a lower yield of 3a (entry 8, Table 1). In all cases, the reactions proceeded through the ring-opening pathway to give homopropargylic boronate 3a as the product (pathway 2 in Scheme 1). Formation of vinyl cyclopropane or alkylidene cyclopropane product (derived from pathway 1 in Scheme 1) was not detected. The presence of a base and the copper catalyst is required for this transformation. A detectable amount product 3a was not formed without the added base (entry 9, Table 1). Similarly, when the reaction of 1a, 2 and NaOMe was conducted in the absence of the copper catalyst, formation of product 3a was again not detected, thereby eliminating a base-promoted deborylative alkylation pathway.13
Table 1 Development of conditions for the reaction of 1,1-bisborylmethane 2 with vinylidene cyclopropane 1aa

|
Entry |
Base |
Solvent |
Yieldb (%) |
Vinylidene cyclopropane 1a (0.1 mmol, 1 equiv.), CuCl (10 mol%), pinBCH2Bpin 2 (0.11 mmol, 1.1 equiv.), base (0.11 mmol, 1.1 equiv.), THF (0.5 mL).
Yields of isolated products are listed.
10 mol% of xantphos was added to the reaction.
The reaction was conducted in the absence of CuCl.
|
1 |
LiOtBu |
THF |
37 |
2 |
NaOtBu |
THF |
80 |
3 |
KOtBu |
THF |
10 |
4 |
LiOMe |
THF |
48 |
5 |
NaOMe |
THF |
87 |
6 |
KOMe |
THF |
63 |
7c |
NaOMe |
THF |
65 |
8 |
NaOMe |
Toluene |
62 |
9 |
No base |
THF |
NR |
10d |
NaOMe |
THF |
NR |
Table 2 summarizes the scope of vinylidene cyclopropane 1 that participated in the reactions with 1,1-bisboryl methane 2 under the developed conditions. In general, these reactions worked well to give homopropargylic boronic ester products 3a–h in 55–87% yields. In the case of 3d, a by-product derived from ester exchange was also isolated (∼10%). The developed conditions are also suitable for reactions of vinylidene cyclopropanes with different substitution patterns. For instance, the reaction of 1,1-bisborylmethane 2 with vinylidene cyclopropane bearing an ethyl group at the terminal olefin unit of the allene (R1 = H, R2 = Et) gave product 3i in 85% yield. A variety of substitution groups on the cyclopropane ring are tolerated under the reaction conditions. Reactions of 2 with vinylidene cyclopropanes substituted with iPr-, npentyl-, or Ph-group proceeded smoothly to give products 3j–l in 72–86% yields. In all cases, formation of vinyl cyclopropane product (e.g., C in Scheme 1) was not observed.
Table 2 Scope of vinylidene cyclopropane 1 for reactions with bis[(pinacolato)boryl]methane 2a,b
Vinylidene cyclopropane 1 (0.1 mmol, 1 equiv.), CuCl (10 mol%), pinBCH2Bpin 2 (0.11 mmol, 1.1 equiv.), NaOMe (0.11 mmol, 1.1 equiv.), THF (0.5 mL).
Yields of isolated products are listed.
NaOtBu was used as the base. A tert-butyl ester byproduct derived from ester exchange was also isolated (∼10%).
|
|
Skipped diynes (e.g., 5, Scheme 2) are useful intermediates in organic synthesis as the alkyne units can undergo a variety of functional group transformations. In particular, they can serve as important precursors to numerous fatty acid natural products.14 Therefore, a novel approach to access skipped diynes would be valuable. Based on the results obtained from Tables 1 and 2, we envisioned that skipped diynes 5 could be prepared from the reaction of vinylidene cyclopropanes 1 with an alkyne nucleophile (Scheme 2). Initial experiments using lithiated alkynes under the Cu-catalyzed reaction conditions failed to produce an appreciable amount of skipped diynes 5. However, we discovered that the targeted skipped diyne 5a was generated from the reaction of vinylidene cyclopropane 1a with alkynyl boronate 4a as the nucleophile precursor. After further optimization of the reaction conditions, NaOtBu was identified as the choice of base for Cu-catalyzed reactions of 1 with alkynyl boronate 4, and the reaction of 1a with boronate 4a produced diyne 5a in 76% yield (Scheme 2).
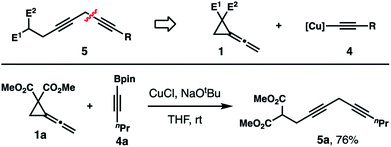 |
| Scheme 2 Proposed synthesis of skipped diynes from Cu-catalyzed alkyne addition to vinylidene cyclopropanes. | |
The developed conditions were adopted to explore the scope of vinylidene cyclopropane 1 and alkynyl boronic ester 4. As summarized in Table 3, a variety of alkynyl boronates, including alkyl, aryl or tBuMe2Si-group substituted boronates, participated in the reactions with vinylidene cyclopropanes 1 to give skipped diynes 5a–l in 53–87% yield. It appears that the aryl substituted alkynyl boronic esters are less reactive than alkyl substituted ones as shown in cases of 5g and 5h.
Table 3 Scope of vinylidene cyclopropane 1 and alkynyl boronate 4 for the synthesis of skipped diynes 5a,b
Vinylidene cyclopropane 1 (0.1 mmol, 1 equiv.), CuCl (10 mol%), alkynyl boronate 4 (0.11 mmol, 1.1 equiv.), NaOtBu (0.11 mmol, 1.1 equiv.), THF (0.5 mL).
Yields of isolated products are listed.
|
|
Although it is known that nucleophilic copper species can react with alkyl halides to form alkylation products; the results from 5i–j demonstrated that the reaction conditions tolerate alkyl chlorides. Reactions with vinylidene cyclopropanes substituted either on the cyclopropane group or at the terminal olefin unit of the allene moiety of 1 proceeded smoothly to give products 5m–o in 68–83% yields.
As shown in Scheme 3, the initial addition of nucleophilic [Cu]–R species to vinylidene cyclopropane 1a should generate vinylcopper intermediate D, which underwent a subsequent β-carbo elimination to open the cyclopropane ring to produce Cu-[C]-enolate E or Cu-[O]-enolate G (or a mixture of two species). We surmised that, in the presence of an electrophile (other than proton), Cu-enolate E or G should react with the electrophile to give product H. However, to implement such a process, it is required that the reaction rate of vinylidene cyclopropane 1 with copper nucleophile [Cu]–R is much faster than the reaction rate of external electrophiles with the copper nucleophile to minimize the formation of side products generated from [Cu]–R and electrophiles (for example, R–El).
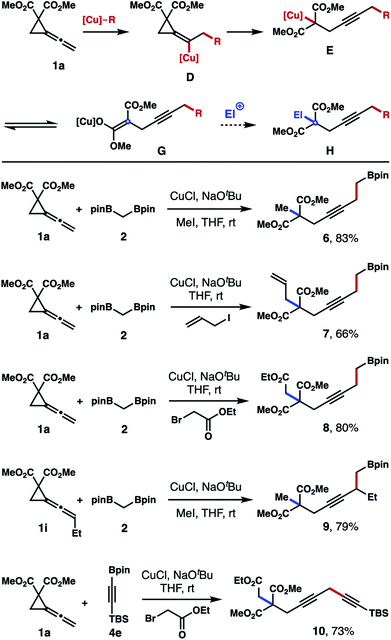 |
| Scheme 3 Three-component reaction of vinylidene cyclopropanes, Cu-nucleophiles and electrophiles. Reaction conditions: vinylidene cyclopropane 1 (0.1 mmol, 1 equiv.), CuCl (10 mol%), 1,1-bisborylmethane 2 or alkynyl boronate 4e (0.11 mmol, 1.1 equiv.), NaOtBu (0.11 mmol, 1.1 equiv.), electrophile (2 equiv.), THF (0.5 mL). Yields of isolated products are listed. | |
To validate our hypothesis, the reaction of vinylidene cyclopropane 1a with 1,1-bisboryl methane 2 was conducted in the presence of MeI. Gratifyingly, methylated boronate 6 was isolated in 83% yield from the reaction (Scheme 3). Product generated from deborylative methylation of 2 (e.g., Et–Bpin) was not detected. In addition to MeI, other electrophiles can also be used to intercept the Cu-enolate. For instance, when allylic iodide was used, allylated product 7 was isolated in 66% yield. Trapping the copper enolate with ethyl bromoacetate gave product 8 in 80% yield. Vinylidene cyclopropane with an ethyl group at the allene unit (1i) also participated in the three-component reaction to give product 9 in 79% yield. Intercepting the Cu-enolate generated from addition of alkynyl copper species to 1a with ethyl bromoacetate afforded product 10 in 73% yield. As such, vinylidene cyclopropane 1 serves as a linchpin to join a nucleophile ([Cu]–R) and an electrophile (El) by forming two carbon–carbon bonds sequentially (highlighted in red and blue in compounds 6–10, Scheme 3).
Derivatization of products obtained from these reactions is illustrated in Scheme 4. Decarboxylation of homopropargylic boronic ester 3a gave product 11 in 81% yield. Oxidation of 3a with NaBO3 produced alcohol 12 in 84% yield. Cu-catalyzed reduction of the alkyne group in 3a generated Z-alkene 13 in 77% yield with excellent selectivity.15 Boronate 3a participated in Pt-catalyzed alkyne diboration to furnish tetra-substituted alkene 14 in 93% yield.16
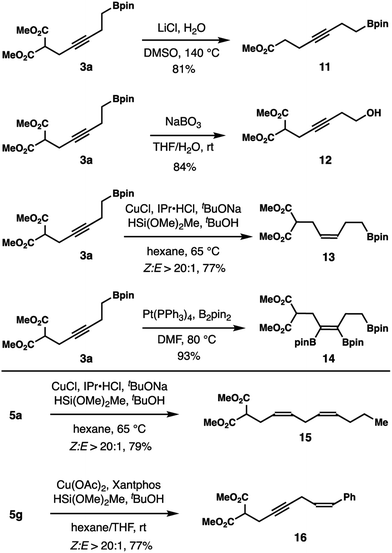 |
| Scheme 4 Transformation of the reaction products. | |
Transformations of skipped diynes were also conducted. Z-Selective reduction of diyne 5a with excess silanes gave skipped Z,Z-diene 15 in 79% yield.15 Such a skipped Z,Z-diene is a common structure motif in many fatty acid natural products. Partial reduction of diyne 5g with silane reducing reagent turned out to be highly regioselective.15 Skipped enyne 16 was obtained in 77% yield with only the phenyl substituted alkyne group being reduced. Products derived from reduction of the other alkyne unit (alkyl substituted) or both alkynes were not observed.
Conclusions
In summary, we developed Cu-catalyzed reactions of vinylidene cyclopropanes with carbon nucleophiles. These reactions proceeded through a ring-opening pathway to give products containing an alkyne group, which is distinct from the pathway typically involved in Cu-catalyzed addition of B, Si, or H nucleophiles to allenes. These reactions generated homopropargylic boronate and skipped diyne products in good yields from vinylidene cyclopropanes and carbon nucleophiles derived from 1,1-bisboryl methane and alkynyl boronates. In addition, the Cu-enolate generated from the initial addition of nucleophilic copper species to vinylidene cyclopropanes can be intercepted by an external electrophile. As such, vinylidene cyclopropane serves as a linchpin to join a nucleophile and an electrophile by forming two carbon–carbon bonds sequentially. The reaction products underwent a variety of subsequent transformations to provide synthetically useful intermediates. Synthetic application of this method will be reported in due course.
Conflicts of interest
There are no conflicts to declare.
Acknowledgements
Financial support provided by Auburn University and Auburn Intramural Grants Program is gratefully acknowledged.
Notes and references
- For selected reviews on vinylidene cyclopropanes, see:
(a) H. Maeda and K. Mizuno, J. Synth. Org. Chem., Jpn., 2004, 62, 1014 CrossRef CAS;
(b) M. Shi, L.-X. Shao, J.-M. Lu, Y. Wei, K. Mizuno and H. Maeda, Chem. Rev., 2010, 110, 5883 CrossRef CAS PubMed;
(c) S. Yang and M. Shi, Acc. Chem. Res., 2018, 51, 1667 CrossRef CAS PubMed.
- M. J. Campbell, P. D. Pohlhaus, G. Min, K. Ohmatsu and J. S. Johnson, J. Am. Chem. Soc., 2008, 130, 9180 CrossRef CAS PubMed.
- For selected recent references with vinylidene cyclopropanes, see:
(a) S. Yang, K.-H. Rui, X.-Y. Tang, Q. Xu and M. Shi, J. Am. Chem. Soc., 2017, 139, 5957 CrossRef CAS PubMed;
(b) C. Ji, Q. Xu and M. Shi, Adv. Synth. Catal., 2017, 359, 974 CrossRef CAS;
(c) W. Yuan, X. Dong, M. Shi, P. McDowell and G. Li, Org. Lett., 2012, 14, 5582 CrossRef CAS PubMed;
(d) L. Wu and M. Shi, Chem.–Eur. J., 2011, 17, 13160 CrossRef CAS PubMed;
(e) L. Wu and M. Shi, Eur. J. Org. Chem., 2011, 2011, 1099 CrossRef;
(f) L. Wu and M. Shi, Chem.–Eur. J., 2010, 16, 1149 CrossRef CAS PubMed;
(g) L. Wu and M. Shi, J. Org. Chem., 2010, 75, 2296 CrossRef CAS PubMed.
- For selected recent reviews:
(a) K. Semba, T. Fujihara, J. Terao and Y. Tsuji, Tetrahedron, 2015, 71, 2183 CrossRef CAS;
(b) A. P. Pulis, K. Yeung and D. J. Procter, Chem. Sci., 2017, 8, 5240 RSC.
- For selected recent references on Cu-catalyzed reactions of allene with boron nucleophiles, see:
(a) K. Semba, T. Fujihara, J. Terao and Y. Tsuji, Angew. Chem., Int. Ed., 2013, 52, 12400 CrossRef CAS PubMed;
(b) K. Semba, M. Shinomiya, T. Fujihara, J. Terao and Y. Tsuji, Chem.–Eur. J., 2013, 19, 7125 CrossRef CAS PubMed;
(c) W. Yuan, X. Zhang, Y. Yu and S. Ma, Chem.–Eur. J., 2013, 19, 7193 CrossRef CAS PubMed;
(d) F. Meng, K. P. McGrath and A. H. Hoveyda, Nature, 2014, 513, 367 CrossRef CAS PubMed;
(e) K. Semba, N. Bessho, T. Fujihara, J. Terao and Y. Tsuji, Angew. Chem., Int. Ed., 2014, 53, 9007 CrossRef CAS PubMed;
(f) F. Meng, X. Li, S. Torker, Y. Shi, X. Shen and A. H. Hoveyda, Nature, 2016, 537, 387 CrossRef CAS PubMed;
(g) W. Zhao and J. Montgomery, J. Am. Chem. Soc., 2016, 138, 9763 CrossRef CAS PubMed;
(h) J. Zhao and K. J. Szabó, Angew. Chem., Int. Ed., 2016, 55, 1502 CrossRef CAS PubMed;
(i) K. Yeung, R. E. Ruscoe, J. Rae, A. P. Pulis and D. J. Procter, Angew. Chem., Int. Ed., 2016, 55, 11912 CrossRef CAS PubMed;
(j) Y. Huang, S. Torker, X. Li, J. del Pozo and A. H. Hoveyda, Angew. Chem., Int. Ed., 2019, 58, 2685 CrossRef CAS PubMed.
- For selected recent references on Cu-catalyzed reactions of allene with silicon nucleophile, see:
(a) Y. Tani, T. Fujihara, J. Terao and Y. Tsuji, J. Am. Chem. Soc., 2014, 136, 17706 CrossRef CAS PubMed;
(b) J. Rae, Y. C. Hu and D. J. Procter, Chem.–Eur. J., 2014, 20, 13143 CrossRef CAS PubMed;
(c) Z.-T. He, X.-Q. Tang, L.-B. Xie, M. Cheng, P. Tian and G.-Q. Lin, Angew. Chem., Int. Ed., 2015, 54, 14815 CrossRef CAS PubMed;
(d) T. Fujihara, A. Sawada, T. Yamaguchi, Y. Tani, J. Terao and Y. Tsuji, Angew. Chem., Int. Ed., 2017, 56, 1539 CrossRef CAS PubMed.
- For selected recent references on Cu-catalyzed reactions of allene with hydrogen nucleophiles, see:
(a) D. Zhao, K. Oisaki, M. Kanai and M. Shibasaki, J. Am. Chem. Soc., 2006, 128, 14440 CrossRef CAS PubMed;
(b) Y. Tani, K. Kuga, T. Fujihara, J. Terao and Y. Tsuji, Chem. Commun., 2015, 51, 13020 RSC;
(c) R. Y. Liu, Y. Yang and S. L. Buchwald, Angew. Chem., Int. Ed., 2016, 55, 14077 CrossRef CAS PubMed;
(d) M. Lee, M. Nguyen, C. Brandt, W. Kaminsky and G. Lalic, Angew. Chem., Int. Ed., 2017, 56, 15703 CrossRef CAS PubMed;
(e) E. Y. Tsai, R. R. Liu, Y. Yang and S. L. Buchwald, J. Am. Chem. Soc., 2018, 140, 2007 CrossRef CAS PubMed;
(f) R. R. Liu, Y. Zhou, Y. Yang and S. L. Buchwald, J. Am. Chem. Soc., 2019, 141, 2251 CrossRef CAS PubMed;
(g) G. Xu, B. Fu, H. Zhao, Y. Li, G. Zhang, Y. Wang, T. Xiong and Q. Zhang, Chem. Sci., 2019, 10, 1802 RSC.
- T. Itoh, Y. Shimizu and M. Kanai, Org. Lett., 2014, 16, 2736 CrossRef CAS PubMed.
- For an example of reaction with allene to form a vinyl copper intermediate due to steric factors, see: W. Yuan, L. Song and S. Ma, Angew. Chem., Int. Ed., 2016, 55, 3140 CrossRef CAS PubMed.
- For selected reviews:
(a) N. Miralles, R. J. Maza and E. Fernandez, Adv. Synth. Catal., 2018, 360, 1306 CrossRef CAS;
(b) R. Nallagonda, K. Padala and A. Masarwa, Org. Biomol. Chem., 2018, 16, 1050 RSC;
(c) C. Wu and J. Wang, Tetrahedron Lett., 2018, 59, 2128 CrossRef CAS.
- For selected recent references on Cu-catalyzed reactions with 1,1-bisboryl alkanes, see:
(a) M. V. Joannou, B. S. Moyer and S. J. Meek, J. Am. Chem. Soc., 2015, 137, 6176 CrossRef CAS PubMed;
(b) M. V. Joannou, B. S. Moyer, M. J. Goldfogel and S. J. Meek, Angew. Chem., Int. Ed., 2015, 54, 14141 CrossRef CAS PubMed;
(c) J. Kim, S. Park, J. Park and S. H. Cho, Angew. Chem., Int. Ed., 2016, 55, 1498 CrossRef CAS PubMed;
(d) Y. Shi and A. H. Hoveyda, Angew. Chem., Int. Ed., 2016, 55, 3455 CrossRef CAS PubMed;
(e) S. A. Murray, J. C. Green, S. B. Tailor and S. J. Meek, Angew. Chem., Int. Ed., 2016, 55, 9065 CrossRef CAS PubMed;
(f) Z.-Q. Zhang, B. Zhang, X. Lu, J.-H. Liu, X.-Y. Lu, B. Xiao and Y. Fu, Org. Lett., 2016, 18, 952 CrossRef CAS PubMed;
(g) J. Kim, K. Ko and S. H. Cho, Angew. Chem., Int. Ed., 2017, 56, 11584 CrossRef CAS PubMed.
-
(a) T. Miura, J. Nakahashi and M. Murakami, Angew. Chem., Int. Ed., 2017, 56, 6989 CrossRef CAS PubMed;
(b) J. Park, S. Choi, Y. Lee and S. H. Cho, Org. Lett., 2017, 19, 4054 CrossRef CAS PubMed;
(c) S. Gao, J. Chen and M. Chen, Chem. Sci., 2019, 10, 3637 RSC.
-
(a) J. R. Coombs, L. Zhang and J. P. Morken, J. Am. Chem. Soc., 2014, 136, 16140 CrossRef CAS PubMed;
(b) K. Hong, X. Liu and J. P. Morken, J. Am. Chem. Soc., 2014, 136, 10581 CrossRef CAS PubMed.
-
(a) S. Gueugnot, M. Alami, G. Linstrumelle, L. Mambu, Y. Petit and M. Larchevêque, Tetrahedron, 1996, 52, 6635 CrossRef CAS;
(b) Y. Suhara, S. Oka, A. Kittaka, H. Takayama, K. Waku and T. Sugiura, Bioorg. Med. Chem., 2007, 15, 854 CrossRef CAS PubMed;
(c) M. A. Lapitskaya, L. L. Vasiljeva and K. K. A. Pivnitsky, Synthesis, 1993, 65 CrossRef CAS;
(d) V. R. Krishnamurthy, A. Dougherty, C. A. Haller and E. L. Chaikof, J. Org. Chem., 2011, 76, 5433 CrossRef CAS PubMed;
(e) L. Qi, M. M. Meijler, S.-H. Lee, C. Sun and K. D. Janda, Org. Lett., 2004, 6, 1673 CrossRef CAS PubMed.
- K. Semba, T. Fujihara, T.-H. Xu, J. Terao and Y. Tsuji, Adv. Synth. Catal., 2012, 354, 1542 CrossRef CAS.
- T. Ishiyama, N. Matsuda, N. Miyaura and A. Suzuki, J. Am. Chem. Soc., 1993, 115, 11018 CrossRef CAS.
Footnote |
† Electronic supplementary information (ESI) available. See DOI: 10.1039/c9sc04122b |
|
This journal is © The Royal Society of Chemistry 2019 |
Click here to see how this site uses Cookies. View our privacy policy here.