Pharmaceutical and anticorrosive substance removal by woodchip column reactor: removal process and effects of operational parameters†
Received
16th October 2019
, Accepted 6th December 2019
First published on 9th December 2019
Abstract
Urban stormwater has recently been considered a potential water resource to augment urban water supplies; however, the existence of emerging contaminants limits urban stormwater utilization. This study aims to use woodchip bioreactors, which are natural and inexpensive, to remove emerging contaminants from artificial stormwater, with a focus on the contaminant removal processes in the woodchip bioreactor and on the effects of operational parameters on the system performance. Seven commonly detected emerging contaminants – acetaminophen (ACE), caffeine (CAFF), carbamazepine (CBZ), ibuprofen (IBU), sulfathiazole (SFZ), benzotriazole (BT) and 5-methyl-1H-benzotriazole (5-MeBT) – were studied. The results showed that the removal efficiency and removal processes are heavily dependent on the compound. ACE and CAFF have the highest removal efficiencies (≥80%), and sorption and biodegradation are both crucial for their removal. However, IBU exhibits very limited sorption and biodegradation and hence has the worst removal (≤15%). The removal efficiencies of the other compounds (SFZ, CBZ, BT and 5-MeBT) range from ∼30 to 60%, and sorption is likely the main removal process. The effects of several operational parameters, including woodchip type, operation time, season and flow rate, on the removal rate of emerging contaminants were also explored. The results of this study showed that the woodchip column system, which is capable of sorption and biodegradation, represents a promising treatment process for removing emerging contaminants from urban stormwater.
Environmental significance
Woodchip bioreactors have been considered to naturally and inexpensively remove emerging contaminants. This study further explored the removal processes and operational factors of the woodchip system for emerging contaminants (acetaminophen, caffeine, carbamazepine, ibuprofen, sulfathiazole, benzotriazole and 5-methyl-1H-benzotriazole) removal. Sorption and biodegradation are two main processes for compounds removal; however, their contribution are heavily dependent on the compound. The results implied that the woodchip system with the sorption and biodegradation capability is a promising method for emerging contaminants removal from urban stormwater.
|
1. Introduction
Water scarcity is becoming one of the most critical issues around the world, and it is driving water-limited countries to reuse treated wastewater to supplement limited water resources. For instance, in California, reclaimed wastewater has been used for irrigation of crops and landscapes, replenishment of wetlands and groundwater recharge. A method of capturing and treating urban stormwater to augment the urban water supply has also been recently proposed.1 In Taiwan, the extremely uneven temporal and spatial distribution of rainfall lead to serious water shortages, prompting the Taiwanese government to explore other potential water sources, such as urban stormwater runoff or wastewater treatment plant (WWTP) effluent.
Unfortunately, these potential water resources (i.e., urban stormwater and WWTP effluent) usually contain pollutants, such as excess nutrients (e.g., nitrogen and phosphorous) and emerging contaminants.2–4 In particular, the issue of emerging contaminants has received extensive concern due to their potential threat to ecosystems and human bodies.5 Many emerging contaminants, such as pharmaceuticals, personal care products, endocrine disrupting chemicals and benzotriazole compounds, are considered to be prevalent and persistent in aquatic environments and pose the risk of inducing toxic effects in aquatic organisms and even humans.6,7 Therefore, to ensure the quality and safety of these recycled waters, the emerging contaminants problem should be well managed.
Common treatments for emerging contaminant abatement, such as advanced oxidation processes (e.g., ozonation and the UV/H2O2 process), often require high capital and operation costs and energy consumption. With this in mind, woodchip bioreactors, which are inexpensive, easily obtainable and have long lifetimes, have potential.8,9 Woodchip systems have been used to successfully remove nitrates from groundwater and surface water.10,11 Woodchips serve as a carbon source and electron donor to maintain the growth of microbes under anaerobic conditions.8,11,12 Additionally, woodchips can be used as promising adsorbent materials. Woodchips contain hydrophobic (lignin) and hydrophilic (cellulose and hemicellulose) materials,13 which can adsorb various pollutants, such as dyes, polynuclear aromatic hydrocarbons, persistent organic pollutants and pesticides.14–17 Currently, there is limited information on the removal of emerging contaminants using the woodchip bioreactor system. Ilhan et al.18 demonstrated that woodchip denitrification walls have the additional benefit of retaining herbicides and antibiotics. Woodchip bioreactors also remove veterinary antibiotics and their transformation byproducts well (>80%).9 However, very few available studies focus on elucidating the removal processes of emerging contaminants in woodchip bioreactors. Information on the influence of the operational parameters on the removal of emerging contaminants by woodchip reactors is also limited.
The previous work done by Halaburka et al.,19 which used the same Californian woodchip column system as in this current work, revealed that the woodchip bioreactor exhibited high nitrate removal efficiency. The objective of this continuation study is (1) to further utilize this woodchip column to remove emerging contaminants from artificial urban stormwater and to understand their removal processes during passage through the column and (2) to adapt the woodchip system to local conditions by utilizing Taiwanese woodchips to investigate the impact of variations in the operational parameters (woodchip type, operation time, season and flow rate) on the emerging contaminant removal. Considering that pharmaceuticals and anticorrosive substances are two types of emerging contaminants frequently detected in aquatic environments,20–22 in this work, we selected five commonly occurring pharmaceuticals (acetaminophen (ACE), caffeine (CAFF), carbamazepine (CBZ), ibuprofen (IBU), sulfathiazole (SFZ)) and two anticorrosive substances (benzotriazole (BT) and 5-methyl-1H-benzotriazole (5-MeBT)) as target emerging contaminants; their physical and chemical properties are shown in Table 1.
Table 1 The physicochemical properties of the seven target compounds
Compound |
Molecular structure |
Molecular weight |
Application |
pKa |
log Kow |
Acetaminophen (ACE) |
|
151.16 |
NSAIDs |
9.4 (ref. 56) |
0.46 (ref. 56) |
Caffeine (CAFF) |
|
194.19 |
Psycho-stimulants |
10.4 (ref. 57) |
−0.07 (ref. 57) |
Carbamazepine (CBZ) |
|
236.26 |
Psychiatric drugs |
14.0 (ref. 58) |
2.25 (ref. 58) |
Ibuprofen (IBU) |
|
206.29 |
NSAIDs |
4.4 (ref. 59) |
3.8 (ref. 59) |
Sulfathiazole (SFZ) |
|
255.32 |
Antibiotics |
2.2; 7.2 (ref. 60) |
0.72 (ref. 61) |
Benzotriazole (BT) |
|
119.12 |
Anticorrosives |
8.2 (ref. 62) |
1.44 (ref. 63) |
5-Methyl-1H-benzotriazole (5-MeBT) |
|
133.12 |
Anticorrosives |
8.5 (ref. 62) |
1.71 (ref. 63) |
2. Methods
2.1 Chemicals and materials
The seven target compounds (ACE (99%), CAFF (99%), CBZ (99%), IBU (99.8%), SFZ (≥98%), BT (99%) and 5-MeBT (98%)), formic acid (≥98%), ammonium acetate (7.5 M), magnesium chloride (MgCl2, >99%), sodium bicarbonate (NaHCO3, >99.5%), and ammonium chloride (NH4Cl, >99.9%) were purchased from Sigma-Aldrich (St. Louis, MO, USA). HPLC-grade methanol was purchased from Merck (Darmstadt, Germany). Calcium chloride (CaCl2, 96%), sodium sulfate (Na2SO4, 99%) and disodium hydrogen phosphate (Na2HPO4, ≥99%) were purchased from J.T. Baker (Deventer, Netherlands). Sodium nitrate (NaNO3, >99.9%) was purchased from Fluka (Seelze, Germany). Sodium hydroxide (NaOH) was purchased from Nacalai Tesque (Kyoto, Japan). The expanded clay was obtained from the Jianguo Flower Market (Taipei, Taiwan). Milli-Q (deionized, DI) water with a conductance of 18.2 MΩ was prepared using a Millipore system and was used in all experiments. Stock solutions of ACE, CAFF, BT and 5-MeBT (1000 mg L−1) were prepared in Milli-Q water; stock solutions of CBZ (1000 mg L−1) was prepared in 1
:
1 (v/v) HPLC-grade methanol and Milli-Q water; stock solutions of SFZ and IBU (1000 mg L−1) were prepared with 200 μL of NaOH (1 M) in a total volume of 10 mL. All stock solutions were stored in amber glass bottles at 4 °C under dark conditions for a maximum of 30 days.
2.2 Woodchip column design and experiment
This study is designed to be a continuation of the work of Halaburka et al.,19 who studied the same woodchip column system as in this work and investigated nitrate removal. This work utilizes the same column with the same Californian woodchips (Column_CA) to explore emerging contaminant removal and elucidate the detailed removal processes in the column. Emerging contaminant removal experiments were previously performed in Column_CA in California, and Column_CA was then shipped to Taiwan to conduct additional experiments. Column_CA was prepared by adding 700 g of Californian woodchips. The Californian woodchips were obtained from an arborist woodchip waste pile in Portola Valley, CA, and were composed of wood from a mix of species, including California redwood (Sequoia sempervirens), oak (Quercus sp.), and Douglas fir (Pseudotsuga menziesii), as described previously in Halaburka et al.19 Acrylic bioreactor columns (10 cm i.d. × 50 cm) were constructed with a sample port at 50 cm to allow sampling from the center of the column. The woodchips were dried at 50 °C for 48 h in a drying oven and then sieved to obtain diameters between 2 and 10 mm. Column_CA was aged for 13 months by a flow of artificial stormwater prior to the column experiments. Upon completion of the experiments, the hydraulic properties of the column, including the drainable porosity, specific retention and total porosity, were determined (Table S1†); detailed information regarding the measurement process has been published in the previous study.19 The total porosity of Column_CA was 0.84, which is similar to that in the previous study (0.87 ± 0.03).19
Fig. S1† shows the schematic diagram of the woodchip column experimental setup. The artificial stormwater matrix was prepared in the laboratory by adding 0.75 mM CaCl2, 0.075 mM MgCl2, 0.33 mM Na2SO4, 1 mM NaHCO3, 0.0715 mM NH4Cl, and 0.016 mM Na2HPO4 to DI water; this composition represents the average concentrations of major ions in urban stormwater.23–25 NaNO3 was spiked into the stormwater to reach the initial nitrate concentration of 10 mg N L−1. Then, the artificial stormwater solution was sterilized via autoclaving (121 °C, 100 kPa) for 45 min. The seven target compounds (with initial concentrations of 100 μg L−1) were spiked into the 50 L polyethylene bucket filled with artificial stormwater and then pumped through PVC tubes to the woodchip columns. The columns were operated in continuous upflow mode by variable-speed digital peristaltic pumps (Hondwen BT100-02-YZ15 model, Taiwan) to maintain saturated hydraulic conditions in order to achieve a constant water flow and stable woodchip performance in the column and to avoid the interference caused by the presence of air within the column during the experiment. The flow rates were controlled at 1 mL min−1 and the initial solution pH value was 6.5. The column and the PVC tubes were covered by aluminum foil to prevent photolysis.
2.3 Removal process identification
The potential removal processes of the target compounds in the woodchip column include hydrolysis, sorption and biodegradation.21,26 Although it is difficult to completely separate the effects of each different process in the column, we attempted to simplify the system and design the following experiments to better understand the removal of the emerging contaminants through the three processes of hydrolysis, sorption and biodegradation in the woodchip column. The details of the experimental design are described as follows.
2.3.1 Hydrolysis.
The hydrolysis tests were performed in batch serum bottle experiments for 14 days. The serum bottles containing artificial stormwater (100 mL) were autoclaved (121 °C, 100 kPa) for 45 min to inhibit microbial growth. Subsequently, the seven target compounds were added to the bottle to achieve the desired initial concentration (100 μg L−1). The experimental conditions were controlled at temperature of 20 ± 1 °C and an initial solution pH of 6.5. All serum bottles were covered with aluminum foil to prevent photolysis. Then, 1 mL samples were taken from the liquid phase at various time intervals and were passed through 0.22 μm PTFE filters. The samples were stored at 4 °C under dark conditions until analysis.
2.3.2 Biodegradation.
Another column filled with expanded clay (Column_EC) was used to help clarify the biodegradation potential of each compound during passage through the woodchip bioreactor by mimicking the microorganism communities in Column_CA. To culture the microorganisms similar to those in Column_CA in Column_EC, the effluent of Column_CA was fed to Column_EC at 1 mL min−1 for one month. Column_EC was subsequently fed artificial stormwater at the same flow rate (1 mL min−1) for 2 weeks. Then, the seven target compounds (with initial concentrations of 100 μg L−1) were spiked in the artificial stormwater and flowed through Column_EC; the initial solution pH was controlled at 6.5. The effluent of Column_EC was sampled at designated time intervals, filtered through 0.22 μm PTFE filters and stored at 4 °C under dark conditions until analysis.
Before conducting the Column_EC experiments, the expanded clay sorption test was performed in a batch serum bottle experiment for 48 h by adding the sorbent (0.5 g of expanded clay), the artificial stormwater (100 mL) and the seven target compounds (each of them was 100 μg L−1); the detailed experimental procedure and conditions are as the woodchip sorption tests, which are described in Section 2.4. Our sorption tests showed that the expanded clay exhibits minimal adsorption capacity for the seven target compounds (Fig. S4†); therefore, biodegradation can be considered the major removal process in this column.
2.4 Woodchip sorption tests and sorption isotherm
The sorption tests and sorption isotherm experiments were conducted in batch serum bottle experiments for 48 h. The sorbent (Californian woodchips) and the artificial stormwater were sequentially added to the serum bottles, and the bottles were then autoclaved (121 °C, 100 kPa) for 45 min to inhibit microbial growth. Then, the seven target compounds were added to the bottle. All serum bottles were covered with aluminum foil to prevent photolysis and were placed on a platform shaker at 75 rpm. The experimental conditions were controlled at a temperature of 20 ± 1 °C and an initial solution pH of 6.5.
For sorption tests, serum bottles containing artificial stormwater (100 mL), 0.5 g of Californian woodchips and the seven target compounds (each at a concentration of 100 μg L−1) were used. During the experiment, 1 mL samples were taken from the liquid phase at various times (0, 2, 6, 12, 24 and 48 h) and were passed through 0.22 μm PTFE filters. The samples were stored at 4 °C under dark conditions until analysis.
For each sorption isotherm experiment, serum bottles containing artificial stormwater (25 mL) and 0.5 g of Californian woodchips were used. The initial concentrations of the seven compounds in the serum bottles were 10, 50, 100, 200, 300, 400 and 500 μg L−1. For each concentration, bottles were prepared in triplicate. After equilibration for 48 h, 1 mL of the supernatant was sampled and filtered with 0.22 μm PTFE filters. The samples were stored at 4 °C under dark conditions until analysis. Based on mass balance, the concentrations of the target compounds adsorbed on the woodchip (CS, mg kg−1) were calculated from their measured concentration in the aqueous phase (CW, mg L−1). Sorption data were fitted to the Freundlich sorption isotherm, CS = Kf × CWn, where CS (mg kg−1) is the amount of chemicals sorbed onto the woodchips, CW (mg L−1) is the equilibrium concentration of chemicals in solution and Kf ((mg kg−1)/(mg L−1)n) and n are the Freundlich constant and linearity factor, respectively.
2.5 Chemical analysis
Liquid chromatography-tandem mass spectrometry (LC–MS/MS) (Applied Biosystems, Foster City, CA, USA) equipped with an electrospray ionization (ESI) interface was used to analyze the seven target pharmaceuticals. The liquid chromatography system contained a pump (Agilent 1200 Series Binary Pump), a degasser (Agilent 1200 Series Micro Vacuum Degasser), and an autosampler (Agilent 1200 Series Autosampler). Analyses underwent separation through a ZORBAX Eclipse XDB-C18 column (Agilent, Palo Alto, CA, USA, 150 × 4.6, 5 μm) at a flow rate of 1 mL min−1. Analyses were performed in positive mode for ACE, CAFF, CBZ, SFZ, BT and 5-MeBT; IBU was performed in negative mode. The detailed information regarding the LC condition and the mass spectrometry information are in Text S1.†
2.6 Effect of operational parameters on compounds removal
The above woodchip experiments were performed by using California woodchips. To adapt the woodchip bioreactor to local conditions, local Taiwanese woodchips were utilized to fill another column (Column_TW) to conduct woodchip column experiments. The Taiwanese woodchips were cedar (Taiwania cryptomerioides) and were obtained from a furniture factory in Taiwan. Column_TW was aged for 3 months prior to the column experiments. The total porosity value of Column_TW was 0.83 (Table S1†). The experimental procedure for Column_TW is the same as that for Column_CA, which is described in Section 2.2. The emerging contaminant removal in Column_TW was conducted and compared with that in Column_CA; then, several operational parameters, including the operation time (15 days and 31 days), season (February in 2018 and August in 2017) and flow rate (1 mL min−1 and 5 mL min−1), were varied to understand their effect on the removal of the emerging contaminants in Column_TW. Regarding the seasonal effect, the two months, February and August, represent the coldest and hottest months in Taiwan; the average temperatures of these two months were 16.5 and 29.2 °C, respectively (Central Weather Bureau, R.O.C. (https://www.cwb.gov.tw/eng/)). All the woodchip column experiments were performed indoors.
One-way analysis of variance (ANOVA) was performed to assess the statistically significant differences in compound removal rates under each operational condition (woodchip type, operation time, season and flow rate). A p-value less than 0.05 was considered statistically significant. All statistical analyses were performed with SPSS statistical software for Windows (version 12.0, SPSS Inc., Chicago, IL, USA).
3. Results and discussion
3.1. Emerging contaminant removal in the woodchip column
The initial experiments were done to explore whether differences in the removal of the emerging contaminants (with initial concentrations of 1 mg L−1) occurred between the two different regions, i.e., California and Taiwan. The removal in California and that in Taiwan are shown in Fig. S2;† overall, the results showed that the compounds have similar removal trends in the two locations when using the same Californian woodchip column (Column_CA). This highlights the good reproducibility of the woodchip column experiment. In the following experiments, to provide a more comprehensive and realistic evaluation of the performance of the woodchip bioreactor with respect to emerging contaminants removal, we decided to lower the initial compound concentration to 100 μg L−1. Furthermore, 2 other commonly detected emerging contaminants (CAFF and SFZ) were also included in the target compound list.
Fig. 1 shows the removal efficiencies of the seven emerging contaminants (initial concentration of 100 μg L−1) in Column_CA. We found that the degree of removal of the compounds through woodchip bioreactors is very dependent on the compound. Complete removal was observed for ACE, while IBU was persistent, and the removal efficiencies (%) were ranked as follows: ACE (almost completely removed) > CAFF (∼80%) > CBZ (∼60%) > SFZ ≈ 5-MeBT (∼50%) > BT (∼30%) > IBU (∼10%). In the woodchip column, hydrolysis, sorption, and biodegradation are the processes that may result in compound removal;21,26 photolysis can be ignored since the whole woodchip column system was covered by opaque aluminum foil. The removal processes for the seven compounds during passage through Column_CA were explored, as discussed in the following section.
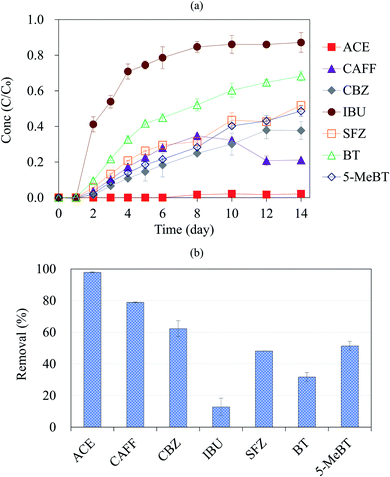 |
| Fig. 1 Target emerging contaminant removal in Californian woodchip column: (a) amount of target compounds remaining in the column effluent and (b) % removal (condition: [compound]0 = 100 μg L; C/C0 represents the effluent concentration of the target compound divided by the influent concentration (C0)). | |
3.1.1. Removal process identification.
Several experiments were designed and conducted to understand the removal process of the seven target compounds during passage through the woodchip column system (Column_CA); the experimental design has been described in detail in Section 2.3. Hydrolysis tests were conducted first. As shown in Fig. S3,† the seven compounds did not degrade via hydrolysis, indicating the unimportance of hydrolysis for the compounds in the woodchip column. To further test the biodegradation potential of the emerging contaminants, we used expanded clay to set up Column_EC, which had previously been fed with the effluent from Column_CA for one month to cultivate a microorganism community similar to that in Column_CA. The same recipe of artificial stormwater was used under the same operational conditions as Column_CA to study the removal of the seven compounds in Column_EC. In Fig. 2, the results showed that only ACE and CAFF were removed in Column_EC; ACE was almost entirely removed after 19 days, and CAFF had a removal efficiency of approximately 40%. Based on the expanded clay sorption test (Fig. S4†), we observed that ACE, CAFF and the other five compounds were not adsorbed by the expanded clay; therefore, the removal of ACE and CAFF presumably occurred through the biodegradation process. To summarize, the results of the Californian woodchip column experiment (Fig. 1) and Column_EC test (Fig. 2) indicate that, among the seven target compounds, biodegradation processes were important in the removal of ACE and CAFF during passage through the woodchip column reactor. This finding is in agreement with previous studies that have described ACE and CAFF as easily biodegraded in both wastewater treatments and the natural environment.27–29
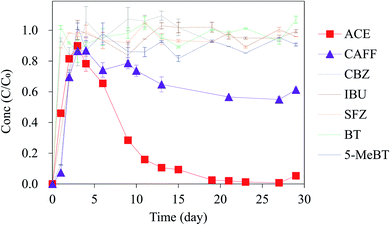 |
| Fig. 2 Target emerging contaminant removal in expanded clay column (condition: [compound]0 = 100 μg L; C/C0 represents the effluent concentration of the target compound divided by the influent concentration (C0)). | |
Based on the results in Fig. 2, the biodegradation was likely insignificant for the target compounds other than ACE and CAFF, including CBZ, IBU, SFZ, BT and 5-MeBT. Chemical structure would affect the organic compound biodegradability.30 For example, CBZ contains two aromatic rings, which results in its recalcitrant characteristic toward biodegradation.31,32 Compound SFZ bears a sulfur atom; previous studies indicated that the presence of sulfur in a compound decreases biodegradability.33,34 For IBU, even though biodegradation is not restricted by structure, it differs greatly under aerobic and anaerobic conditions. Zwiener et al.35 found that the removal efficiency of IBU was very high under aerobic conditions (90%); however, under anaerobic conditions, the removal efficiency of IBU was only 15%. Suarez et al.36 also noted that IBU is easily biodegraded only under aerobic conditions. In this work, the woodchip column experiment tends to exhibit microaerobic or anoxic conditions,37 which explains the poor removal of IBU in our woodchip column reactor (Fig. 1). Moreover, the results in this work corresponded with previous studies investigating the biodegradation of CBZ, SFZ, BT and 5-MeBT; these four compounds showed no biodegradation or very slow biodegradation in other biological treatment systems.21,33,38–40
3.2. Sorption studies
Based on the discussion in Section 3.1, several compounds exhibited no removal in Column_EC but were still partially removed in Column_CA (CBZ, SFZ, BT and 5-MeBT; ∼30–60% removal), which implies that sorption likely dominates their removal in the Californian woodchip column reactor. To understand the difference in the sorbabilities of the compounds, sorption experiments were conducted. Fig. 3 shows the sorption experiment for the seven target compounds with Californian woodchips. As shown in our result, sorbability is best for SFZ and worst for IBU, and the sorbabilities of the target compounds rank in the following order: SFZ > ACE ≈ CAFF ≈ CBZ ≈ BT ≈ 5-MeBT > IBU.
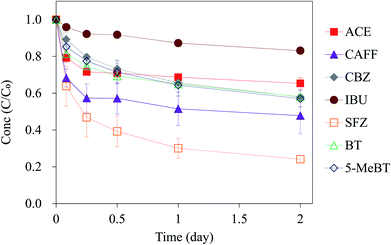 |
| Fig. 3 Sorption test of target compounds by Californian woodchips (condition: [compound]0 = 100 μg L−1, woodchip amount = 2 g, batch reactor volume = 100 mL; y axis shows the aqueous phase concentration of the target compounds). | |
The physicochemical properties of the target compounds significantly affect their sorbabilities.41 Due to different pKa values (Table 1), the compounds are present in different dissociated forms in the solution, thus resulting in different sorption affinities with the woodchip adsorbent. Under the experimental pH condition in this work (pH = 6.5), IBU (pKa = 4.4) existed mostly in anion form; the poor sorption between IBU and the woodchip (Fig. 3) is very likely because electrostatic repulsion occurred between anionic IBU and the negatively charged woodchip. Dechene et al.42 observed a similar result and noted that the low sorption between anionic herbicides with the negatively charged sorbent (biochar-amended soil) is presumably due to a repulsive force. Other six compounds, ACE, CAFF, CBZ, BT, 5-MeBT and SFZ, are present in neutral forms under a pH of 6.5; hence, they would have higher sorbabilities than IBU. SFZ showed the highest sorbability; Thiele-Bruhn43 indicated that the hydrogen bond and van der Waals interactions lead to the sorption between the sulfonamide and the organic adsorbent.
The sorption isotherms for the target compounds are shown in Fig. S5.† The sorption results of the compounds were fitted well to the Freundlich model (R2 ≥ 0.902), and the Freundlich constant, Kf ((mg kg−1)/(mg L−1)n), and linearity factor, n, were estimated and are reported in Table 2. The sorption of ACE, SFZ, 5-MeBT, BT and CAFF to the woodchip exhibited linearity or near-linearity (n ranged between 0.77 and 1.04); CBZ was less linear (n was 0.62). IBU showed very limited adsorption and hence the Kf and n values were hard to calculate. Among all the compounds, except for ACE (n = 1.04), the five other compounds have n values less than 1, which implied that an increasing aqueous-phase compound concentration lead to a decrease in sorption. This is possibly because sorption at higher energy sites of the woodchip occurs first and is followed sorption at lower energy sites.44,45 Moreover, to understand and compare the sorbabilities of the target compounds, the linear partition coefficient (Kd) was calculated (Table 2). SFZ has the highest Kd value (Kd = 75.4 L kg−1), which is consistent with the sorption experiment (Fig. 3). The Kd values obtained in this work are also compared with those in other studies investigating the soil sorption of the same emerging contaminants. The higher Kd values by woodchip sorption indicate better sorption in the current case. For example, the Kd values of ACE (46.1 L kg−1) and CBZ (11.9 L kg−1) are approximately 6 to 10 times larger than the Kd values of the two compounds (7.7 L kg−1 and 1.2 L kg−1, respectively) for the model soil, i.e., Elliot silt loam soil.46 The Kd value of BT in this work (16.6 L kg−1) was similar to that of the other woodchip column study (Kd = 14.9 L kg−1).47
Table 2 Freundlich sorption parameters (Kf, n) and linear partition coefficients (Kd) of the target compound sorbed to the Californian woodchips
|
Freundlich isotherm |
K
d
|
K
f
|
n
|
R
2
|
ACE |
46.7 ± 4.9 |
1.04 |
1.000 |
46.1 ± 6.6 |
CAFF |
26.9 ± 1.6 |
0.77 |
0.902 |
48.4 ± 1.0 |
CBZ |
7.5 ± 1.8 |
0.62 |
0.930 |
11.9 ± 0.4 |
IBU |
— |
— |
— |
— |
SFZ |
67.1 ± 3.6 |
0.91 |
0.999 |
75.4 ± 17.1 |
BT |
14.8 ± 1.1 |
0.84 |
0.989 |
16.6 ± 3.6 |
5-MeBT |
24.4 ± 2.8 |
0.87 |
0.988 |
23.6 ± 7.1 |
To summarize the results of the sorption experiments in this section and the removal process identification discussed in Section 3.1, the compound IBU, with limited sorption and biodegradation, exhibits the lowest removal efficiency in the woodchip column (only 13% removal; Fig. 1). SFZ has strong sorption, suggesting that the ∼50% SFZ removal in the column reactor (Fig. 1) was dominated by sorption. Ilhan et al.26 studied the removal of another sulfonamide, sulfamethazine, in a woodchip bioreactor and noted the importance of sorption. Additionally, ACE and CAFF exhibited both strong sorption and biodegradation; hence, they have excellent removal efficiencies (≥80%) in the column system. In particular, the approximately 100% removal of ACE in the woodchip column was achieved due to its very high biodegradability. Our results agree with the findings of Wade,48 who investigated the remediation of ACE with woodchips and indicated that both abiotic sorption and biotic biodegradation contributed for its removal, with biodegradation being more important. The rest of the compounds, CBZ, BT and 5-MeBT, have similar sorbabilities, and their removal efficiencies range from ∼30 to 60%, most likely due to sorption.
3.3 Effects of operational parameters
In this section, to suit the woodchip type to the local conditions in order to better explore the applicability of the woodchip column bioreactor system for emerging contaminant removal, local Taiwanese woodchips were utilized. First, the emerging contaminant removal in Column_TW (using Taiwanese woodchips) was compared with that in Column_CA (using Californian woodchips), as shown in Fig. 4(a). We found that Column_TW also exhibited promising results for the removal of the studied compounds. However, the compound removal trends in the two columns differed. For instance, SFZ, BT and 5-MeBT exhibited higher removal rates, while ACE and CAFF showed lower removal rates in Column_TW than in Column_CA (p < 0.05). A possible explanation of these differences in compound removal between the Taiwanese and Californian woodchips is as follows. The two types of the woodchips may be composed of different proportions of hydrophobic (lignin) and hydrophilic (cellulose and hemicellulose) components; this would lead to different sorption affinities of the woodchips with respect to the seven compounds, which have distinct hydrophobicity/hydrophilicity properties (Table 1).14 The aging time of the woodchips was also possibly another factor (the aging times of the Taiwanese woodchips and Californian woodchips were 3 and 13 months, respectively). Halaburka et al.19 and Addy et al.49 noted that the aging time of woodchips has a significant impact on nitrate removal; however, information on the influence of woodchip aging time on emerging contaminant removal remains limited. Further investigation is required to thoroughly understand the effects of woodchip composition and aging time on the removal rate of emerging contaminants.
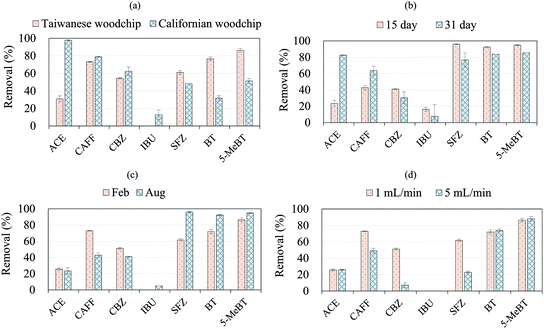 |
| Fig. 4 Effects of operational parameters on target emerging contaminant removal (%): (a): effect of woodchip type; (b), (c) and (d): effects of operation time, season and flow rate in the Taiwanese woodchip column, respectively (condition: [compound]0 = 100 μg L; operation time = 14 days for (a) and 15 days for (c) and (d)). | |
Fig. 4(b) demonstrates the effect of operation time for compound removal in Column_TW (the corresponding compound removal curve is provided in Fig. S6†). Higher removal efficiencies of ACE and CAFF were observed after 31 days than after 15 days (p < 0.05). Based on Fig. S6,† it is observed that the effluent concentrations (C/C0) of ACE and CAFF increase for the first 15 days, after which they continuously decrease. This phenomenon is speculated to result from an initial removal by sorption alone, followed by simultaneous sorption and biodegradation, since microorganisms have gradually adapted to the woodchip column environment and possess the ability to biodegrade ACE and CAFF. Similarly, Abdi et al.50 investigated pesticide remediation using a woodchip bioreactor and conclude that the presence of pesticides can cause the microbial communities in the woodchip bioreactor to adapt to pesticide pollutants. LeFevre et al.51 also observed that exposure to naphthalene enriched the population of naphthalene-degrading bacteria in the bioretention media. Additionally, our results on ACE and CAFF removal (Fig. 4(b) and S6†) further support the importance of the biodegradation process of these two compounds in the woodchip column, as discussed in Section 3.2. In contrast, the compounds SFZ, BT and 5-MeBT exhibited slightly higher removal efficiencies after 15 days of operation time than after 31 days (p < 0.05); this is likely due to the remobilization of compounds that were previously adsorbed on the woodchips,47 resulting in lower removal rates over longer operation times (31 day).
The effect of season (February and August) on compound removal is shown in Fig. 4(c). Since the woodchip column experiments were conducted under indoor conditions, the woodchip system was less susceptible to changes in several environmental factors (e.g., rain, wind and humidity); thus, the temperature effect is the main factor leading to seasonal variation in the removal of the emerging contaminants by the woodchip bioreactor. The average temperatures in February and August were 16.5 and 29.2 °C, respectively (Central Weather Bureau, R.O.C.; https://www.cwb.gov.tw/eng/). In our results, we found that no significant difference in removal efficiency was observed for the compound ACE between these two months (p = 0.33); however, the compounds SFZ, BT and 5-MeBT exhibited higher removal rates in August (p < 0.05), while CAFF and CBZ exhibited higher removal rates in February (p < 0.05). Previous studies have shown that temperature variation can influence the microbial community growth, resulting in changes in contaminant removal in the biological treatment system.52,53
Fig. 4(d) shows the effect of different flow rates (1 mL min−1 and 5 mL min−1) on compound removal. Result shows that the high flow rate (5 mL min−1) did not result in a significant difference for the removal of the compounds ACE, BT and 5-MeBT (p = 0.51, 0.45 and 0.67 for ACE, BT and 5-MeBT, respectively). However, for CAFF, CBZ and SFZ, the removal rates under the flow rate of 1 mL min−1 were obviously higher than those under the flow rate of 5 mL min−1 (p < 0.05). At the lower flow rate, pollutants have more time to be in contact with the adsorbent; this possibly leads to greater removal in the column.54,55 Moreover, nitrate removal was also obviously affected by increasing flow rate; in this work, nitrate removal dropped from 90% to 50% when the flow rate increased from 1 mL min−1 to 5 mL min−1, which is consistent with the work of Halaburka et al.19 Therefore, to achieve good removal for both emerging contaminants and nitrate, the flow rate should be carefully considered when the operational parameters of the woodchip bioreactor are designed.
4. Conclusions
This study enhances our understanding of the fate of five pharmaceuticals and two anticorrosive substances in woodchip bioreactors. Results from this work indicated that the removal process is heavily dependent on the compound. ACE and CAFF show good removal efficiencies (≥80%) in the Californian woodchip reactor; these two compounds experience both sorption and biodegradation processes. Conversely, for IBU, the very limited sorption and biodegradation tendencies result in poor removal (only ∼10% removal in Column_CA). The other four compounds, SFZ, CBZ, BT and 5-MeBT, exhibited ∼30 to 60% removal. The sorption study shows that the adsorption of all target compounds (except IBU) fit Freundlich isotherms well, and SFZ has the highest Kf and Kd values. These sorption results can be extrapolated to other emerging contaminants in woodchip systems. The effects of several operational parameters, including woodchip type, operation time, season and flow rate, on the removal rate of emerging contaminants were also studied. Further investigation should explore the effects of woodchip composition and aging time on the sorption and biodegradation behaviors of emerging contaminants, and the woodchip system should be applied for real stormwater treatment to evaluate the system practicability. In summary, by providing adsorption sites for the contaminants and carbon sources for microorganism growth, the woodchip bioreactor represents a promising method of handling emerging contaminants and further reducing the risks posed by these contaminants in urban stormwater.
Conflicts of interest
There are no conflicts to declare.
Acknowledgements
This work was financially supported by National Taiwan University from Excellence Research Program-Core Consortiums (NTU-CC-108L891305) within the framework of the Higher Education Sprout Project of the Ministry of Education (MOE) in Taiwan. The authors thank Dr Richard G. Luthy's research group in Department of Civil and Environmental Engineering at Stanford University for providing the woodchip column reactor.
References
- R. G. Luthy, S. Sharvelle and P. Dillon, Urban Stormwater to Enhance Water Supply, Environ. Sci. Technol., 2019, 53, 5534–5542 CrossRef CAS PubMed.
- G. H. LeFevre, K. H. Paus, P. Natarajan, J. S. Gulliver, P. J. Novak and R. M. J. J. o. E. E. Hozalski, Review of dissolved pollutants in urban storm water and their removal and fate in bioretention cells, J. Environ. Eng., 2014, 141, 04014050 CrossRef.
- N. H. Tran, M. Reinhard and K. Y. H. Gin, Occurrence and fate of emerging contaminants in municipal wastewater treatment plants from different geographical regions-a review, Water Res., 2018, 133, 182–207 CrossRef CAS PubMed.
- J. Gan, S. Bondarenko, L. Oki, D. Haver and J. X. Li, Occurrence of Fipronil and Its Biologically Active Derivatives in Urban Residential Runoff, Environ. Sci. Technol., 2012, 46, 1489–1495 CrossRef CAS PubMed.
- K. Noguera-Oviedo and D. S. Aga, Lessons learned from more than two decades of research on emerging contaminants in the environment, J. Hazard. Mater., 2016, 316, 242–251 CrossRef CAS PubMed.
- S. W. Li and A. Y. C. Lin, Increased acute toxicity to fish caused by pharmaceuticals in hospital effluents in a pharmaceutical mixture and after solar irradiation, Chemosphere, 2015, 139, 190–196 CrossRef CAS PubMed.
- A. R. Katritzky, K. Kasemets, S. Slavov, M. Radzvilovits, K. Tamm and M. Karelson, Estimating the toxicities of organic chemicals in activated sludge process, Water Res., 2010, 44, 2451–2460 CrossRef CAS PubMed.
- L. A. Schipper, W. D. Robertson, A. J. Gold, D. B. Jaynes and S. C. Cameron, Denitrifying bioreactors-An approach for reducing nitrate loads to receiving waters, Ecol. Eng., 2010, 36, 1532–1543 CrossRef.
- N. Gottschall, M. Edwards, E. Craiovan, S. K. Frey, M. Sunohara, B. Ball, E. Zoski, E. Topp, I. Khan, I. D. Clark and D. R. Lapen, Amending woodchip bioreactors with water treatment plant residuals to treat nitrogen, phosphorus, and veterinary antibiotic compounds in tile drainage, Ecol. Eng., 2016, 95, 852–864 CrossRef.
- W. D. Robertson, Nitrate removal rates in woodchip media of varying age, Ecol. Eng., 2010, 36, 1581–1587 CrossRef.
- D. W. Blowes, W. D. Robertson, C. J. Ptacek and C. Merkley, Removal of Agricultural Nitrate from Tile-Drainage Effluent Water Using in-Line Bioreactors, J. Contam. Hydrol., 1994, 15, 207–221 CrossRef CAS.
- W. D. Robertson, J. L. Vogan and P. S. Lombardo, Nitrate removal rates in a 15-year-old permeable reactive barrier treating septic system nitrate, Ground Water Monit. Rev., 2008, 28, 65–72 CrossRef CAS.
-
E. Sjostrom, Wood chemistry: fundamentals and applications, Gulf professional publishing, 1993 Search PubMed.
- J. M. Marin-Benito, E. Herrero-Hernandez, M. S. Rodriguez-Cruz, M. Arienzo and M. J. Sanchez-Martin, Study of processes influencing bioavailability of pesticides in wood-soil systems: Effect of different factors, Ecotoxicol. Environ. Saf., 2017, 139, 454–462 CrossRef CAS PubMed.
- A. Shukla, Y. H. Zhang, P. Dubey, J. L. Margrave and S. S. Shukla, The role of sawdust in the removal of unwanted materials from water, J. Hazard. Mater., 2002, 95, 137–152 CrossRef CAS PubMed.
- T. B. Boving and W. Zhang, Removal of aqueous-phase polynuclear aromatic hydrocarbons using aspen wood fibers, Chemosphere, 2004, 54, 831–839 CrossRef CAS PubMed.
- M. A. Olivella, P. Jove, A. Bianchi, C. Bazzicalupi and L. Cano, An integrated approach to understanding the sorption mechanism of phenanthrene by cork, Chemosphere, 2013, 90, 1939–1944 CrossRef CAS PubMed.
- Z. E. Ilhan, S. K. Ong and T. B. Moorman, Herbicide and Antibiotic Removal by Woodchip Denitrification Filters: Sorption Processes, Water, Air, Soil Pollut., 2012, 223, 2651–2662 CrossRef CAS.
- B. J. Halaburka, G. H. LeFevre and R. G. Luthy, Evaluation of Mechanistic Models for Nitrate Removal in Woodchip Bioreactors, Environ. Sci. Technol., 2017, 51, 5156–5164 CrossRef CAS PubMed.
- K. H. Y. Chung, Y. C. Lin and A. Y. C. Lin, The persistence and photostabilizing characteristics of benzotriazole and 5-methyl-1H-benzotriazole reduce the photochemical behavior of common photosensitizers and organic compounds in aqueous environments, Environ. Sci. Pollut. Res., 2018, 25, 5911–5920 CrossRef CAS PubMed.
- J. L. Wang and S. Z. Wang, Removal of pharmaceuticals and personal care products (PPCPs) from wastewater: A review, J. Environ. Manage., 2016, 182, 620–640 CrossRef CAS PubMed.
- S. Mompelat, B. Le Bot and O. Thomas, Occurrence and fate of pharmaceutical products and by-products, from resource to drinking water, Environ. Int., 2009, 35, 803–814 CrossRef CAS PubMed.
- J. E. Grebel, S. K. Mohanty, A. A. Torkelson, A. B. Boehm, C. P. Higgins, R. M. Maxwell, K. L. Nelson and D. L. Sedlak, Engineered Infiltration Systems for Urban Stormwater Reclamation, Environ. Eng. Sci., 2013, 30, 437–454 CrossRef CAS.
- S. K. Mohanty, A. A. Torkelson, H. Dodd, K. L. Nelson and A. B. Boehm, Engineering Solutions to Improve the Removal of Fecal Indicator Bacteria by Bioinfiltration Systems during Intermittent Flow of Stormwater, Environ. Sci. Technol., 2013, 47, 10791–10798 CrossRef CAS PubMed.
- B. A. Ulrich, E. A. Im, D. Werner and C. P. Higgins, Biochar and Activated Carbon for Enhanced Trace Organic Contaminant Retention in Stormwater Infiltration Systems, Environ. Sci. Technol., 2015, 49, 6222–6230 CrossRef CAS PubMed.
- Z. E. Ilhan, S. K. Ong and T. B. Moorman, Dissipation of Atrazine, Enrofloxacin, and Sulfamethazine in Wood Chip Bioreactors and Impact on Denitrification, J. Environ. Qual., 2011, 40, 1816–1823 CrossRef CAS PubMed.
- K. M. Onesios, J. T. Yu and E. J. Bouwer, Biodegradation and removal of pharmaceuticals and personal care products in treatment systems: a review, Biodegradation, 2009, 20, 441–466 CrossRef CAS PubMed.
- S. K. Maeng, S. K. Sharma, C. D. T. Abel, A. Magic-Knezev and G. L. Amy, Role of biodegradation in the removal of pharmaceutically active compounds with different bulk organic matter characteristics through managed aquifer recharge: Batch and column studies, Water Res., 2011, 45, 4722–4736 CrossRef CAS PubMed.
- S. A. Snyder, J. Leising, P. Westerhoff, Y. Yoon, H. Mash and B. Vanderford, Biological and physical attenuation of endocrine pisruptors and pharmaceuticals: Implications for water reuse, Ground Water Monit. Rev., 2004, 24, 108–118 CrossRef CAS.
- H. Loonen, F. Lindgren, B. Hansen, W. Karcher, J. Niemela, K. Hiromatsu, M. Takatsuki, W. Peijnenburg, E. Rorije and J. Struijs, Prediction of biodegradability from chemical structure: Modeling of ready biodegradation test data, Environ. Toxicol. Chem., 1999, 18, 1763–1768 CrossRef CAS.
- V. Martinez-Hernandez, R. Meffe, S. H. Lopez and I. de Bustamante, The role of sorption and biodegradation in the removal of acetaminophen, carbamazepine, caffeine, naproxen and sulfamethoxazole during soil contact: A kinetics study, Sci. Total Environ., 2016, 559, 232–241 CrossRef CAS PubMed.
-
A. Boxall, Transformation products of synthetic chemicals in the environment, Springer, 2009 Search PubMed.
- C. Bertelkamp, J. Reungoat, E. R. Cornelissen, N. Singhal, J. Reynisson, A. J. Cabo, J. P. van der Hoek and A. R. D. Verliefde, Sorption and biodegradation of organic micropollutants during river bank filtration: A laboratory column study, Water Res., 2014, 52, 231–241 CrossRef CAS PubMed.
- R. W. Okey and H. D. Stensel, A QSAR-based biodegradability model - A QSBR, Water Res., 1996, 30, 2206–2214 CrossRef CAS.
- C. Zwiener, T. Glauner and F. H. Frimmel, Biodegradation of pharmaceutical residues investigated by SPE-GC/ITD-MS and on-line derivatization, HRC J. High Resolut. Chromatogr., 2000, 23, 474–478 CrossRef CAS.
- S. Suarez, J. M. Lema and F. Omil, Removal of Pharmaceutical and Personal Care Products (PPCPs) under nitrifying and denitrifying
conditions, Water Res., 2010, 44, 3214–3224 CrossRef CAS PubMed.
- E. V. Lopez-Ponnada, T. J. Lynn, M. Peterson, S. J. Ergas and J. R. Mihelcic, Application of denitrifying wood chip bioreactors for management of residential non-point sources of nitrogen, J. Biol. Eng., 2017, 11, 16 CrossRef CAS PubMed.
- B. Blair, A. Nikolaus, C. Hedman, R. Klaper and T. Grundl, Evaluating the degradation, sorption, and negative mass balances of pharmaceuticals and personal care products during wastewater treatment, Chemosphere, 2015, 134, 395–401 CrossRef CAS PubMed.
- Y. S. Liu, G. G. Ying, A. Shareef and R. S. Kookana, Biodegradation of three selected benzotriazoles under aerobic and anaerobic conditions, Water Res., 2011, 45, 5005–5014 CrossRef CAS PubMed.
- H. Y. Dong, X. J. Yuan, W. D. Wang and Z. M. Qiang, Occurrence and removal of antibiotics in ecological and conventional wastewater treatment processes: A field study, J. Environ. Manage., 2016, 178, 11–19 CrossRef CAS PubMed.
-
M. Grassi, G. Kaykioglu, V. Belgiorno and G. Lofrano, in Emerging compounds removal from wastewater, Springer, 2012, pp. 15–37 Search PubMed.
- A. Dechene, I. Rosendahl, V. Laabs and W. Amelung, Sorption of polar herbicides and herbicide metabolites by biochar-amended soil, Chemosphere, 2014, 109, 180–186 CrossRef CAS PubMed.
- S. Thiele-Bruhn, T. Seibicke, H. R. Schulten and P. Leinweber, Sorption of sulfonamide pharmaceutical antibiotics on whole soils and particle-size fractions, J. Environ. Qual., 2004, 33, 1331–1342 CrossRef CAS PubMed.
- A. Bialk-Bielinska, J. Maszkowska, W. Mrozik, A. Bielawska, M. Kolodziejska, R. Palavinskas, P. Stepnowski and J. Kumirska, Sulfadimethoxine and sulfaguanidine: Their sorption potential on natural soils, Chemosphere, 2012, 86, 1059–1065 CrossRef CAS PubMed.
- L. M. Peruchi, A. H. Fostier and S. Rath, Sorption of norfloxacin in soils: Analytical method, kinetics and Freundlich isotherms, Chemosphere, 2015, 119, 310–317 CrossRef CAS PubMed.
- H. Yamamoto, Y. Nakamura, S. Moriguchi, Y. Nakamura, Y. Honda, I. Tamura, Y. Hirata, A. Hayashi and J. Sekizawa, Persistence and partitioning of eight selected pharmaceuticals in the aquatic environment: Laboratory photolysis, biodegradation, and sorption experiments, Water Res., 2009, 43, 351–362 CrossRef CAS PubMed.
- N. Ashoori, M. Teixido, S. Spahr, G. H. LeFevre, D. L. Sedlak and R. G. Luthy, Evaluation of pilot-scale biochar-amended woodchip bioreactors to remove nitrate, metals, and trace organic contaminants from urban stormwater runoff, Water Res., 2019, 154, 1–11 CrossRef CAS PubMed.
-
J. P. Wade, Biotic and Abiotic Remediation of Acetaminophen with Woodchip and Biochar-amended Woodchip Adsorbents, Virginia Tech, 2015 Search PubMed.
- K. Addy, A. J. Gold, L. E. Christianson, M. B. David, L. A. Schipper and N. A. Ratigan, Denitrifying Bioreactors for Nitrate Removal: A Meta-Analysis, J. Environ. Qual., 2016, 45, 873–881 CrossRef CAS PubMed.
- D. E. Abdi, J. S. Owen Jr, J. C. Brindley, A. C. Birnbaum, P. C. Wilson, F. O. Hinz, G. Reguera, J.-Y. Lee, B. M. Cregg and D. R. J. W. R. Kort, Nutrient and pesticide remediation using a two-stage bioreactor-adsorptive system under two hydraulic retention times, Water Res., 2020, 170, 115311 CrossRef PubMed.
- G. H. LeFevre, P. J. Novak and R. M. Hozalski, Fate of Naphthalene in Laboratory-Scale Bioretention Cells: Implications for Sustainable Stormwater Management, Environ. Sci. Technol., 2012, 46, 995–1002 CrossRef CAS PubMed.
- T. Panswad, A. Doungchai and J. Anotai, Temperature effect on microbial community of enhanced biological phosphorus removal system, Water Res., 2003, 37, 409–415 CrossRef CAS PubMed.
-
B. E. Rittmann and P. L. McCarty, Environmental biotechnology: principles and applications, Tata McGraw-Hill Education, 2012 Search PubMed.
- R. P. Han, D. D. Ding, Y. F. Xu, W. H. Zou, Y. F. Wang, Y. F. Li and L. Zou, Use of rice husk for the adsorption of congo red from aqueous solution in column mode, Bioresour. Technol., 2008, 99, 2938–2946 CrossRef CAS PubMed.
- M. T. Uddin, M. Rukanuzzaman, M. M. R. Khan and M. A. Islam, Adsorption of methylene blue from aqueous solution by jackfruit (Artocarpus heteropyllus) leaf powder: A fixed-bed column study, J. Environ. Manage., 2009, 90, 3443–3450 CrossRef PubMed.
- S. W. Nam, D. J. Choi, S. K. Kim, N. Her and K. D. Zoh, Adsorption characteristics of selected hydrophilic and hydrophobic micropollutants in water using activated carbon, J. Hazard. Mater., 2014, 270, 144–152 CrossRef CAS PubMed.
- A. Karnjanapiboonwong, A. N. Morse, J. D. Maul and T. A. Anderson, Sorption of estrogens, triclosan, and caffeine in a sandy loam and a silt loam soil, J. Soils Sediments, 2010, 10, 1300–1307 CrossRef CAS.
- T. Scheytt, P. Mersmann, R. Lindstadt and T. Heberer, 1-octanol/water partition coefficients of 5 pharmaceuticals from human medical care: Carbamazepine, clofibric acid, diclofenac, ibuprofen, and propyphenazone, Water, Air, Soil Pollut., 2005, 165, 3–11 CrossRef CAS.
- J. N. Brown, N. Paxeus, L. Forlin and D. G. J. Larsson, Variations in bioconcentration of human pharmaceuticals from sewage effluents into fish blood plasma, Environ. Toxicol. Pharmacol., 2007, 24, 267–274 CrossRef CAS PubMed.
- A. L. Boreen, W. A. Arnold and K. McNeill, Photochemical fate of sulfa drugs in the aquatic environment: Sulfa drugs containing five-membered heterocyclic groups, Environ. Sci. Technol., 2004, 38, 3933–3940 CrossRef CAS PubMed.
- M. S. Diaz-Cruz, M. J. L. de Alda and D. Barcelo, Determination of antimicrobials in sludge from infiltration basins at two artificial recharge plants by pressurized liquid extraction-liquid chromatography-tandem mass spectrometry, J. Chromatogr. A, 2006, 1130, 72–82 CrossRef CAS PubMed.
- D. S. Hart, L. C. Davis, L. E. Erickson and T. M. Callender, Sorption and partitioning parameters of benzotriazole compounds, Microchem. J., 2004, 77, 9–17 CrossRef CAS.
- J. Casado, R. Nescatelli, I. Rodriguez, M. Ramil, F. Marini and R. Cela, Determination of benzotriazoles in water samples by concurrent derivatization-dispersive liquid-liquid microextraction followed by gas chromatography-mass spectrometry, J. Chromatogr. A, 2014, 1336, 1–9 CrossRef CAS PubMed.
Footnote |
† Electronic supplementary information (ESI) available. See DOI: 10.1039/c9em00470j |
|
This journal is © The Royal Society of Chemistry 2020 |
Click here to see how this site uses Cookies. View our privacy policy here.