DOI:
10.1039/C9FO00530G
(Paper)
Food Funct., 2020,
11, 965-976
α-Bisabolol protects against β-adrenergic agonist-induced myocardial infarction in rats by attenuating inflammation, lysosomal dysfunction, NLRP3 inflammasome activation and modulating autophagic flux
Received
13th March 2019
, Accepted 19th November 2019
First published on 25th November 2019
Abstract
Emerging evidence demonstrates that NLRP3 inflammasome activation, lysosomal dysfunction, and impaired autophagic flux play a crucial role in the pathophysiology of myocardial infarction (MI). Therapeutic strategies targeting NLRP3 activation, lysosomal enzyme release and correcting autophagy have shown beneficial effects in suppressing early inflammatory responses in cardiovascular diseases. Thus, the agents, which inhibit NLRP3 activation and correct lysosome dysfunction and impaired autophagic flux, can be potential drugs for MI. The present study evaluated the effects and elucidated the NLRP3 inflammasome mediated mechanism of α-bisabolol, a dietary sesquiterpene alcohol in a rat model of isoproterenol (ISO)-induced MI. In the present study, male albino Wistar rats were pre- and co-treated with intraperitoneal injection of α-bisabolol (25 mg kg−1) daily for 10 days along with the subcutaneous injection of ISO (85 mg kg−1) at an interval of 24 h for two days (9th and 10th day). ISO injections induced MI as evidenced by the elevated cardiac marker enzyme in serum and altered oxidative stress markers in the total heart and lysosomal fractions. ISO also caused activation of NLRP3 inflammasomes mediating TLR4-NFκB/MAPK signaling pathways and lysosomal dysfunction along with induction and release of proinflammatory cytokines. Interestingly, treatment with α-bisabolol favorably corrected the morphological, histopathological, ultrastructural, biochemical and molecular abnormalities induced by ISO-induced MI in rats. Furthermore, the ultrastructural studies also confirmed the improvement in the autophagic mechanism. The findings of the present study clearly demonstrate that α-bisabolol attenuates oxidative stress and inflammation by inhibiting NLRP3 inflammasome activation and TLR4-NFκB/MAPK signaling pathways along with correcting lysosomal dysfunction and impaired autophagic flux. The underlying pharmacological and molecular mechanism of cardioprotection was attributed to its antioxidant, free radical scavenging and anti-inflammatory properties.
Introduction
Myocardial infarction (MI) is one of the lethal manifestations of cardiovascular diseases. It occurs as a result of imbalance between coronary blood supply and myocardial demand precipitated by the rupture of lipid-laden plaques and the formation of blood clots. Both these events cause irreversible damage to the certain areas of the heart muscle. Among numerous pathogenic triggers prompting the rupture of plaques, inflammation is one of the major risk factors that plays a crucial role in the pathogenesis of MI.1
To mimic human MI in experimental models, isoproterenol (ISO), a synthetic catecholamine and a β-adrenergic agonist, has attracted attention due to its potential of causing MI in a physiological manner. The necrotic lesions developed via free radical mediated oxidative stress after supramaximal doses of ISO resemble MI in humans.2 ISO induced MI is a common and well standardized model to study the impact of many drugs and cardiac function.3–8 ISO upon auto-oxidation generates highly cytotoxic free radicals that stimulate lipid peroxidation and cause irreversible damage to the myocardium.4 In addition to oxidative stress, inflammation is another inevitable mechanism involved in the pathogenesis of ISO induced MI.9
In numerous pathways of inflammation, lysosomal enzymes, which upon intracellular release after myocardial ischemia, may directly or by the activation of complement pathways lead to cell injury and death.10 The release of lysosomal enzymes plays a crucial role in the activation of the nucleotide-binding domain, leucine-rich-containing family, and pyrin domain-containing-3 (NLRP3) inflammasomes.11 Furthermore, alterations in the activities of lysosomal enzymes have been noticed in patients with MI.12 Cytokines are inflammatory mediators which trigger plaque rupture and intraluminal thrombosis via formation of atheroma and rapid evolution of the atheromatous injury.13 Pro-inflammatory cytokines are elevated in acute myocardial injury and infarction and they are highly responsible for the initiation and maintenance of systemic and vascular inflammation correlated with the coronary artery disease.14
Emerging evidence revealed that toll-like receptors (TLRs) may play a role in the expression of NLRP3 inflammasome proteins as well as the precursors of interleukin-1β (IL1β) and interleukin-18 (IL-18) via activation of nuclear factor kappa B (NF-κB) and mitogen-activated protein kinase (MAPK) signaling pathways.15 Activation of TLRs may result in the release of several inflammatory cytokines via various signaling cascades including NF-κB activation.16 NF-κB is the traditional priming signal for the transcription of the NLRP3 gene.17 There is compelling evidence that both the NF-κB and MAPK signaling pathways play a pivotal role in regulating the expression and activation of NLRP3 inflammasomes during ischemic conditions.15 The NLRP3 inflammasome has emerged as an important regulator of inflammation and its activation forms a complex with its adaptor apoptosis-associated speck-like protein containing a CARD (ASC) which leads to the activation of pro-caspase-1 and the formation of an active caspase-1 p10/20 tetramer. The activated caspase-1 converts pro-IL-1β into its mature form.18 During oxidative stress, thioredoxin interacting/inhibiting protein (TXNIP) can directly bind NLRP3 and lead to NLRP3 inflammasome assembly.19 Autophagy plays a vital role in maintaining cellular homeostasis and survival by recycling damaged organelles and proteins. Impairment in autophagic flux alters myocardial function and triggers heart failure during stress conditions.20 Furthermore, its activation inversely regulates the activation of NLRP3 inflammasomes responsible for inflammation in coronary arteries.21
Increasing attention has been paid to sesquiterpenes for their multipharmacological properties. α-Bisabolol is a sesquiterpene alcohol found in essential oils derived from a variety of plants, including chamomile (Chamomilla recutita L.),22 salvia (Salvia runcinata),23Plinia Cerrocampanensi24 and wood of Candeia (Eremanthus erythropappus).25 α-Bisabolol has generated considerable economic interest since it possesses a delicate floral odor and has been shown to possess antioxidant,26 anti-inflammatory,27 gastroprotective,28 antimutagenic, antibiotic29 and anti-spasmodic30 properties. The United States Food and Drug Administration has classified α-bisabolol as ‘generally regarded as safe’ due to its low toxicity that encourages its use as an active ingredient in commercial products. α-Bisabolol is non-toxic when administered orally in mice and rats (LD50 13–14 g per kg body weight).31 To the best of our knowledge, this is the first study carried out on the protective effects of α-bisabolol especially on inflammation associated with the activation of NLRP3 inflammasomes in ISO induced myocardial infarcted rats.
Methods
Drugs and chemicals
α-Bisabolol, isoproterenol hydrochloride, thiobarbituric acid, trichloroacetic acid, para-nitrophenyl β-D-glucuronide, nitrophenyl β-N-galactopyranoside, para-nitrophenol, α-N-benzoyl-DL-arginine-p-nitroanilide hydrochloride, ethylene diamine tetra acetic acid, and L-cysteine were purchased from Sigma Chemical Company, St Louis, MO. All the other chemicals used were of analytical grade.
Experimental animals
The experiment was performed with male albino Wistar rats weighing 160–180 g acquired from the local experimental animal breeding facility of the College of Medicine & Health Sciences (CMHS), United Arab Emirates University. Rats were housed in polypropylene cages (47 × 34 × 20 cm) lined with husk (replaced every 24 h) in a 12 h light/dark cycle at around 22 °C. Rats were fed on a standard rodent chow diet (National Feed and Flour Production and Marketing Company LLC., Abu Dhabi, UAE) and water ad libitum. The experimental protocol (A31/14) for animal experimentation was approved by the Animal Ethics Committee of United Arab Emirates University.
Induction of MI in Wistar rats
ISO (85 mg per kg body weight) dissolved in saline was subcutaneously injected into rats at an interval of 24 h for 2 days. ISO-induced MI was confirmed by elevated activity of serum lactate dehydrogenase (LDH) in rats.
Experimental design
A total of 80 animals were separated into four groups containing twenty rats each. Group I: Normal control rats; Group II: Rats were intraperitoneally treated with α-bisabolol (25 mg per kg body weight) daily for a period of 10 days; Group III: Rats were subcutaneously injected with ISO (85 mg per kg body weight) at an interval of 24 h for 2 days (on 9th and 10th day); Group IV: Rats were pre- and co-treated intraperitoneally with α-bisabolol (25 mg per kg body weight) daily for a period of 10 days and were subcutaneously injected with ISO at an interval of 24 h for two days (9th and 10th day). The dose and the mode of administration of α-bisabolol were selected based on our previous pilot study wherein we have screened many doses of α-bisabolol with different treatment protocols using different routes of administration.5 Twelve hours after the second dose of ISO injection i.e. 24 hours after the first dose of ISO injection (i.e. on 11th day), the rats were anesthetized by pentobarbital sodium (60 mg per kg body weight) and the blood samples were collected in dry tubes without anticoagulants to obtain serum. The rats under anesthesia will be sacrificed by cervical decapitation and the hearts were excised immediately and rinsed in ice cold saline. The heart tissue with determined weight was homogenized in Tris-HCl buffer (0.1 M; pH 7.4) and used for biochemical estimations. For western blotting, the heart tissues were homogenized in RIPA buffer. The heart tissues were also used for ultrastructural, histological and myocardial infarct size studies.
Biochemical estimations
Assay of cardiac marker enzymes.
The activities of serum LDH were assayed by using a Vet Test 8008 Chemistry Analyzer, UK and by using commercial kits.
Measurement of the infarct size.
The myocardial infarct size was analyzed by staining hearts with 2,3,5-triphenyltetrazolium chloride (TTC).32
Estimation of lipid peroxidation products and antioxidants in the myocardium.
The concentration/activities of heart TBARS, superoxide dismutase (SOD) and catalase were estimated by the method of Fraga et al.,33 Kakkar et al.34 and Sinha.35
Separation of subcellular fractions and estimation/assay of heart lysosomal lipid peroxidation and lysosomal enzymes in the serum, heart and subcellular fractions.
The lysosomal and cytosolic fractions were isolated according to the method of Sathish et al.36 The levels of heart lysosomal thiobarbituric acid reactive substances (TBARS) were estimated by the method of Fraga et al.33 The activities of β-glucuronidase, β-galactosidase, cathepsin-B and cathepsin-D in the serum/heart tissue homogenate/subcellular fractions were assayed according to the methods of Kawai and Anno,37 Conchie et al.,38 Barrett39 and Sapolsky et al.,40 respectively.
Estimation of pro-inflammatory and anti-inflammatory cytokines.
The levels of pro-inflammatory cytokines such as tumor necrosis factor-α (TNF-α), interleukin-6 (IL-6), interleukin-1β (IL-1β) and interleukin-10 (IL-10) were estimated using the commercially available ELISA kits purchased from R&D system (Minneapolis, MN, USA).
Western blot analysis.
The heart tissue samples were homogenized in an ice-cold RIPA buffer and the homogenate was centrifuged at 12
000 rpm for 30 min at 4 °C to remove the debris. The protein content in the samples was estimated using the Pierce™ BCA protein assay kit (Thermo Fisher Scientific, Rockford, IL, USA). The protein samples were loaded and separated by SDS-polyacrylamide (12%). The membranes were incubated with the blocking buffer containing 5% bovine serum albumin for 1 h to reduce non-specific binding sites. The proteins were transferred onto a PVDF membrane and incubated with specific primary antibodies against inducible nitric oxide synthase (iNOS) (1
:
1000) (Sigma, St Louis, MO, USA) cyclooxygenase-2 (COX-2) (1
:
1000), IκB (1
:
1000), NLRP3 (1
:
1000), ASC (1
:
1000), IL-1β (1
:
500), TXNIP (1
:
1000), P38-MAPK (1
:
1000) and P38-MAPK (1
:
1000), TLR4 (1
:
1000), t-ERK (1
:
1000), p-ERK (1
:
1000), (Abcam, Cambridge, MA USA), caspase-1-p20 (1
:
1000) (Adipogen Life sciences, USA), NF-κB-p65 (1
:
1000), p-IκB (1
:
500), p-NF-κB-p65 (1
:
500), t-JNK (1
:
1000), p-JNK (1
:
1000) (Santa Cruz Biotechnology, Dallas, TX, USA) and β-actin (1
:
2000) (MERCK Millipore, USA) with gentle shaking overnight at 4 °C. Then after, the membranes were incubated with their corresponding secondary antibodies (anti-rabbit or anti-mouse IgG conjugated to horseradish peroxidase) for 1 h at room temperature and the protein bands were visualized using an enhanced chemiluminescence pico kit (Thermo Fisher Scientific, Rockford, IL, USA). The intensity of the developed bands was quantified using Image J software (NIH, USA).
Histopathology of the myocardium.
The heart tissues obtained from rats of all the experimental groups were washed immediately with ice cold saline and then fixed in 10% buffered neutral formalin solution. After fixation, the heart tissues were processed by embedding in paraffin. Then, the tissues were sectioned and stained with hematoxylin and eosin and examined under a light microscope (10×) and photomicrographs were taken.
Transmission electron microscopy (TEM) study.
The ultrastructure of the heart mitochondrial specimen was examined by Transmission Electron Microscopy (TEM) (Fei, Teenai BioTwin G2, Holland) (Magnification ×5000). Small pieces of the heart tissues were taken and rinsed in 0.1 M phosphate buffer (pH 7.2). Approximately, 1 mm heart pieces were trimmed and immediately fixed into 3% ice-cold glutaraldehyde in 0.1 M phosphate buffer (pH 7.2) and kept at 4 °C for 12 h. The tissue processing for the TEM study was carried out and the grids containing sections were stained with 2% uranyl acetate and 0.2% lead acetate. The sections were examined under a transmission electron microscope.
Estimation of the protein in the total heart and subcellular fractions.
The protein content in the total heart and subcellular fractions was estimated by using a Pierce™ BCA protein assay kit (Thermo Fisher Scientific, Rockford, IL, USA).
Results
α-Bisabolol restores the activities of serum LDH and myocardial infarct size
ISO induced rats showed significantly (P < 0.05) elevated LDH activity in the serum with increased myocardial infarct size whereas α-bisabolol (25 mg per kg body weight) pre- and co-treated rats showed significantly (P < 0.05) decreased activities of serum LDH and myocardial infarct size (Fig. 1A–C).
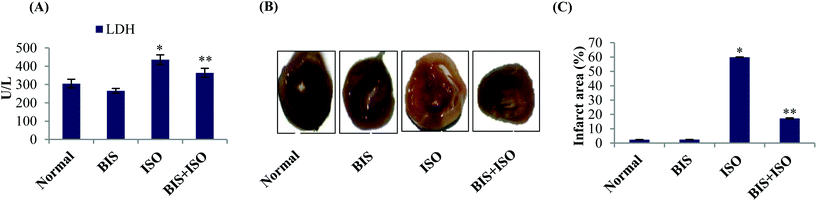 |
| Fig. 1 Effect of α-bisabolol on the cardiac marker enzyme and myocardial infarct size in ISO induced rats. (A) Levels of serum LDH. Each column is mean ± standard error of the mean (SEM) for six rats in each group; columns not sharing a common symbol (* and **) differ significantly with each other (*P < 0.05 vs. normal control, **P < 0.05 vs. ISO control). (B) TTC staining of the myocardium. (C) Planimetry analysis. Representative photomicrographs of the infarct area (white patches) and non-infarcted area (red patches) for all groups were shown and their respective infarct area in percentage was determined using Image J software. Values are represented as mean ± SEM (n = 3). Columns not sharing a common symbol (* and **) differ significantly with each other (*P < 0.05 vs. normal control, **P < 0.05 vs. ISO control). | |
α-Bisabolol attenuates oxidative stress in the myocardium
The levels of myocardial TBARS were significantly (P < 0.05) increased and the activities of enzymatic antioxidants such as SOD and catalase were significantly (P < 0.05) decreased in ISO induced rats compared to normal control rats. α-Bisabolol (25 mg per kg body weight) pre- and co-treatment significantly (P < 0.05) decreased the levels of myocardial TBARS and significantly (P < 0.05) increased the activities of SOD and catalase compared to ISO alone induced rats (Fig. 2A–C).
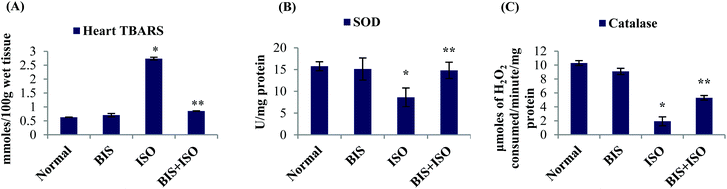 |
| Fig. 2 Effect of α-bisabolol on myocardial oxidative stress. (A) Concentration of TBARS. (B) Activity of SOD. (C) Activity of catalase. Each column is mean ± SEM for eight rats in each group; columns not sharing a common symbol (* and **) differ significantly with each other (*P < 0.05 vs. normal control, **P < 0.05 vs. ISO control). *Units: mmoles per 100 g wet tissue for heart TBARS; U mg−1 protein for SOD; μmoles of H2O2 consumed per minute per mg protein for catalase. | |
α-Bisabolol attenuates lysosomal dysfunction in ISO-induced rats
ISO induced myocardial infarcted rats showed a significant (P < 0.05) increase in the levels/activities of lysosomal TBARS and lysosomal enzymes such as β-glucuronidase, β-galactosidase, cathepsin-B and cathepsin-D in the serum and heart compared to normal control rats. α-Bisabolol pre and co-treatment significantly (P < 0.05) decreased the levels/activities of heart lysosomal TBARS and lysosomal enzymes in the serum and heart of ISO induced rats compared to ISO alone induced rats (Table 1).
Table 1 Effect of α-bisabolol on levels/activities of lysosomal enzymes in the serum, heart and subcellular fractions in ISO induced rats
Groups |
Normal |
BIS |
ISO |
BIS + ISO |
Each value is mean ± standard error of the mean (SEM) for eight rats in each group; columns not sharing a common symbol (* and **) differ significantly with each other (*P < 0.05 vs. normal control, **P < 0.05 vs. ISO control). |
Lysosomal TBARS (mmoles per 100 g protein) |
0.50 ± 0.02 |
0.49 ± 0.03 |
1.43 ± 0.03* |
0.57 ± 0.01** |
Serum lysosomal enzymes
|
β-Glucuronidase (μmoles of p-nitrophenol liberated per h per ml) |
23.26 ± 1.1 |
22.21 ± 1.54 |
34.44 ± 2.67* |
27.83 ± 0.96** |
β-Galactosidase (μmoles of p-nitrophenol liberated per h per ml) |
17.56 ± 0.75 |
18.0 ± 1.34 |
32.44 ± 1.71* |
24.11 ± 1.06** |
Cathepsin-B (μmoles of p-nitroaniline liberated per h per ml) |
32.24 ± 1.27 |
31.6 ± 1.37 |
44.98 ± 2.44* |
36.31 ± 1.12 ** |
Cathepsin-D (μmoles of tyrosine liberated per h per ml) |
25.48 ± 0.81 |
25.89 ± 0.26 |
41.89 ± 2.5* |
33.48 ± 0.77** |
Heart lysosomal enzymes
|
β-Glucuronidase (μmoles of p-nitrophenol liberated per h per 100 mg protein) |
18.02 ± 1.24 |
16.25 ± 0.87 |
25.5 ± 0.88* |
16.66 ± 0.57** |
β-Galactosidase (μmoles of p-nitrophenol liberated per h per mg protein) |
16.6 ± 1.04 |
17.76 ± 0.4 |
29.13 ± 1.0* |
16.68 ± 0.79** |
Cathepsin-B (μmoles of p-nitroaniline liberated per h per 100 mg protein) |
22.19 ± 1.9 |
19.93 ± 0.98 |
31.22 ± 1.17* |
19.46 ± 0.85** |
Cathepsin-D (μmoles of tyrosine liberated per h per 100 mg protein) |
35.71 ± 6.27 |
29.95 ± 1.58 |
52.38 ± 1.60* |
31.38 ± 1.09** |
Lysosomal enzymes (subcellular fractions)
|
Lysosomal fraction
|
β-Glucuronidase (μmoles of p-nitrophenol liberated per h per mg protein) |
27.43 ± 1.19 |
24.73 ± 1.28 |
13.64 ± 0.70* |
24.11 ± 0.74** |
Cathepsin-D (μM of tyrosine liberated per h per 100 mg protein) |
26.67 ± 1.09 |
24.03 ± 1.17 |
14.53 ± 0.74* |
20.93 ± 0.47** |
Cytosolic fraction
|
β-Glucuronidase (μmoles of p-nitrophenol liberated per h per mg protein) |
17.36 ± 0.96 |
13.19 ± 0.22 |
42.36 ± 0.60* |
33.84 ± 0.72** |
Cathepsin-D (μM of tyrosine liberated per h per 100 mg protein) |
11.41 ± 0.55 |
8.98 ± 0.11 |
17.61 ± 0.31* |
11.73 ± 0.24** |
α-Bisabolol prevents the leakage of β-glucuronidase and cathepsin-D into the cytosol
The levels of β-glucuronidase and cathepsin-D were significantly (P < 0.05) increased in the cytosolic fraction than the lysosomal fraction of ISO induced rats compared to normal control rats. α-Bisabolol pre and co-treatment prevents the leakage of these lysosomal enzymes into the cytosolic fraction of ISO induced rats compared to ISO induced rats (Table 1).
α-Bisabolol attenuates induction and release of pro-inflammatory cytokines and inflammatory mediators
The levels/expressions of pro inflammatory cytokines such as TNF-α, IL-6, and IL-1β and the inflammatory mediators such as iNOS and COX-2 were significantly (P < 0.05) increased and the level of anti-inflammatory cytokine, IL-10 was significantly (P < 0.05) decreased in ISO induced rats. α-Bisabolol treatment showed significantly (P < 0.05) decreased levels/expressions of pro-inflammatory cytokines (TNF-α, IL-1β, IL-6) and inflammatory mediators (iNOS and COX-2) with significantly (P < 0.05) increased levels of IL-10 in ISO induced myocardial infarcted rats compared to ISO induced rats (Fig. 3A–F).
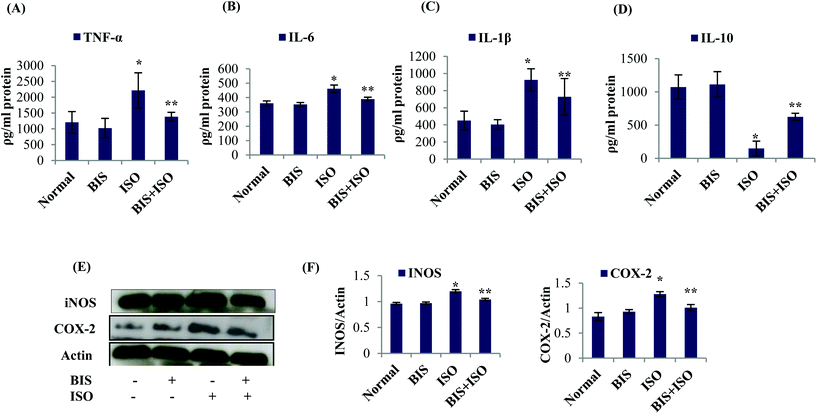 |
| Fig. 3 (A–D) Effect of α-bisabolol on cytokines, histology and the expressions of inflammatory mediators of the heart. (A–D) Levels of pro-inflammatory cytokines (TNF-α, IL-6, IL-1β) and anti-inflammatory cytokine (IL-10). Each column is mean ± SEM for eight rats in each group; columns not sharing a common symbol (* and **) differ significantly with each other (*P < 0.05 vs. normal control, **P < 0.05 vs. ISO control). (E and F) Effect of α-bisabolol on the myocardial protein expressions of inflammatory mediators, (E). Expressions of iNOS and COX-2 in the myocardium. (F and G) Quantification of Western blots assayed for iNOS and COX-2; Columns not sharing a common symbol (* and **) differ significantly with each other (*P < 0.05 vs. normal control, **P < 0.05 vs. ISO control). Lane 1: Normal control, Lane 2: α-bisabolol (25 mg kg−1), Lane 3: ISO (85 mg kg−1), Lane 4: α-bisabolol (25 mg kg−1) + ISO (85 mg kg−1). | |
α-Bisabolol salvages the histoarchitecture of the myocardium
The normal control rats showed normal architecture of heart without any pathological changes (Fig. 4A). Rats treated with α-bisabolol showed normal myocardial architecture (Fig. 4B). Rats induced with ISO showed separation of cardiac muscle fibers and inflammatory cells (Fig. 4C). Pre and co-treatment with α-bisabolol revealed mild separation of cardiac muscle fibers with less inflammatory cells in the heart of ISO induced myocardial infarcted rats (Fig. 4D).
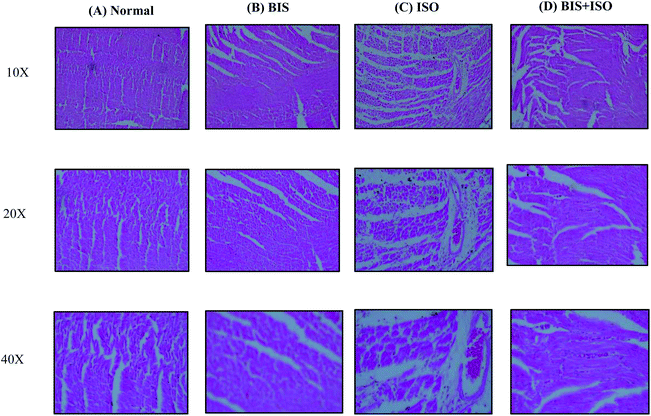 |
| Fig. 4 Histopathological observations of the heart. (A) Normal control rat's heart showing normal architecture of the myocardium, (B) α-bisabolol alone (per se) treated rat's heart showing normal cardiac muscle bundles with no pathological changes, (C) ISO (85 mg per kg body weight)-induced rat's heart showing infarcted zone with inflammatory cells, (D) α-bisabolol pre- and co-treated rat's heart showing mild separation of cardiac muscle fibers with less inflammatory cells. | |
α-Bisabolol attenuates NLRP3 inflammasome activation by inhibiting TLR4 mediated NF-κB/MAPK signaling
ISO induced rats showed a significant (P < 0.05) increase in the expressions of TLR4/NF-κB (TLR4, p-NFκB-p65, p-IκBα), MAPK (p-ERK1/2, p-P38, p-JNK) and inflammasome complexes (NLRP3, ASC, pro-IL-1β, caspase-1-p20 and TXNIP) proteins compared to normal control rats. α-Bisabolol treatment significantly (P < 0.05) decreased the expressions of all these proteins in ISO induced myocardial infarcted rats compared to ISO alone induced rats (Fig. 5A and B).
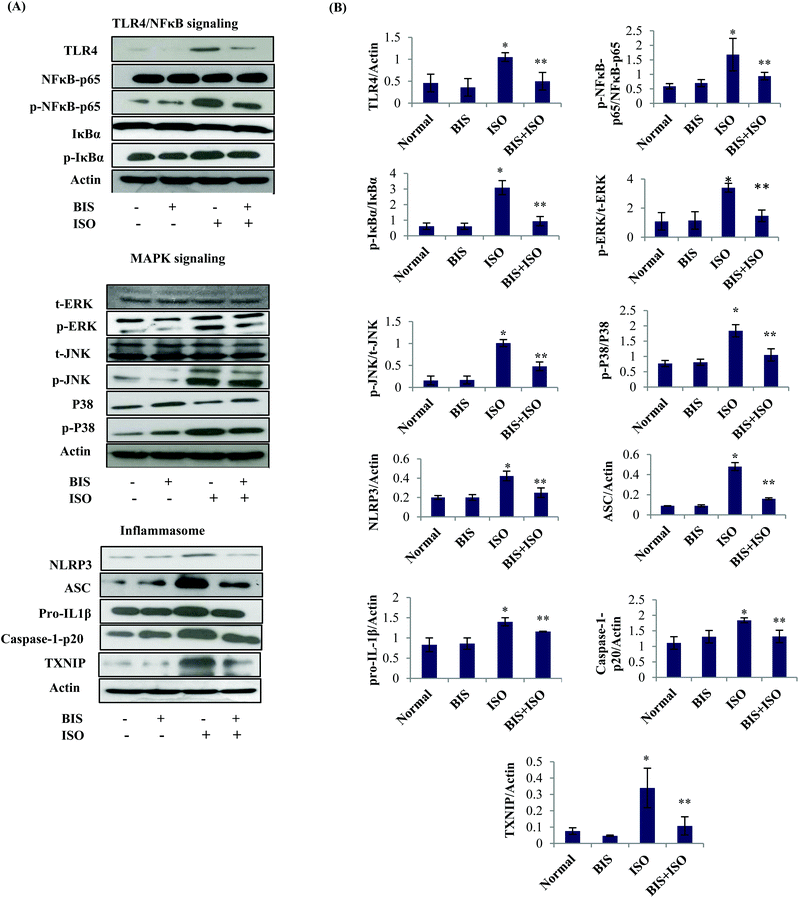 |
| Fig. 5 Effect of α-bisabolol on TLR4 mediated NFκB/MAPK signaling and NLRP3 inflammasome activation. (A) Myocardial protein expressions of TLR4, NFκB-p65, p-NFκB-p65, IκBα, p-IκBα, t-ERK, p-ERK, t-JNK, p-JNK, P38 and p-P38, NLRP3, ASC, IL1β, caspase-1-p20 and TXNIP. (B) Quantification of Western blots assayed for the myocardial protein expressions of NF-κB/MAPK signaling and NLRP3 inflammasome proteins. Lane 1: Normal control, Lane 2: α-bisabolol (25 mg kg−1), Lane 3: ISO (85 mg kg−1) Lane 4: α-bisabolol (25 mg kg−1) + ISO (85 mg kg−1). Columns not sharing a common symbol (* and **) differ significantly with each other (*P < 0.05 vs. normal control, **P < 0.05 vs. ISO control). | |
α-Bisabolol augments autophagic flux in the myocardium
The expressions of autophagic proteins were slightly increased but not significantly along with a significant (P < 0.05) decrease in the phosphorylation of mTOR in ISO induced rats compared to normal control rats. Interestingly, we have found a significant (P < 0.05) increase in the protein expressions of autophagic proteins with a significant (P < 0.05) decrease in the phosphorylation of mTOR in the heart of α-bisabolol pre and co-treated myocardial infarcted rats (Fig. 6A and B).
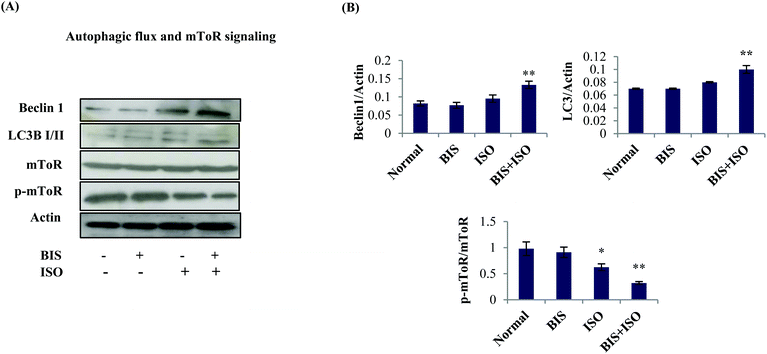 |
| Fig. 6 Effect of α-bisabolol on autophagic flux and mTOR signaling. (A) Myocardial protein expressions of Beclin-1, LC3BI/II, mTOR and p-mTOR. (B) Quantification of Western blots assayed for autophagic machineries and mTOR signaling. Lane 1: Normal control, Lane 2: α-bisabolol (25 mg kg−1), Lane 3: ISO (85 mg kg−1) Lane 4: α-bisabolol (25 mg kg−1) + ISO (85 mg kg−1). Columns not sharing a common symbol (* and **) differ significantly with each other (*P < 0.05 vs. normal control, **P < 0.05 vs. ISO control). | |
α-Bisabolol protects the ultrastructure of the myocardium
The TEM image of α-bisabolol alone treated rats showed normal and active autophagic vacuoles (Fig. 7, i–iii). ISO induced rats showed completely inactive and abnormal autophagic vacuoles (Fig. 7, iv–vi). α-Bisabolol pre and co-treated ISO induced rats showed more active autophagic vacuoles (Fig. 7, vii–ix).
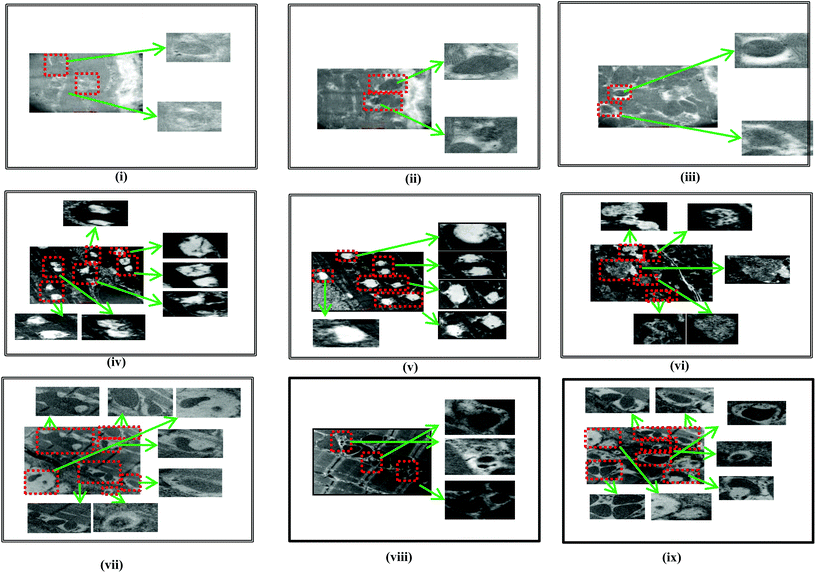 |
| Fig. 7 Ultrastructural findings of the myocardium. (i)–(iii): The TEM image of α-bisabolol alone treated rats showed normal and active autophagic vacuoles. (iv)–(vi): ISO induced rats showed impaired autophagic vacuoles. (vii)–(ix): α-Bisabolol pre and co-treated ISO-induced rats showed autophagic vacuoles which contains acid phosphatase activity (black deposits) indicating that fusion with lysosomes has occurred. | |
For all the biochemical parameters studied, α-bisabolol (25 mg per kg body weight) alone treated rats (Group-II) did not show any significant effect compared to normal control rats (Group-I).
Discussion
In response to β-adrenergic stimulation, the release of cardiac marker enzymes such as LDH followed by the alterations in the integrity of the plasma membrane has been observed in ISO induced rats. This is due to the sarcolemmal damage triggered by the β-adrenergic receptor agonist, which has rendered it leaky.9 The extent of myocardial damage is detected by the direct staining of TTC dye with LDH to form a red formazan precipitate. The infarcted area lacks the LDH enzyme and therefore fails to stain with the dye. The area of infarction may be related to LDH leakage and loss of membrane integrity.41 α-Bisabolol defends the myocardium by inhibiting the leakage of LDH into the circulation in ISO induced myocardial infarcted rats by virtue of its potent free radical scavenging and membrane stabilizing property.
The generation of highly cytotoxic free radicals destabilizes the myocardium by elevating lipid peroxidation in ISO induced myocardial infarcted rats. Impairment in the efficiency of antioxidants might be due to the myocardial cell damage via oxidative stress triggered β1-adrenoceptor activation in the myocardium. The deleterious effects could be due to the autoxidized ISO metabolites which interfere with the antioxidants such as SOD and catalase.3 α-Bisabolol by virtue of its potent free radical scavenging effect attenuates oxidative stress in the myocardium by decreasing the lipid peroxidation and by augmenting antioxidant status in ISO induced rats.
The free radicals produced by ISO destabilize lysosomal membranes by reacting with its lipid bilayer resulting in the rupture of lysosomes and the release of acid hydrolases and cathepsins into the cytosol and to the circulation. The release of acid hydrolases from the lysosome to the cytosol leads to myocardial cellular injury and death in the ischemic heart and it stimulates the formation of inflammatory mediators such as oxygen radicals and prostaglandins in the myocardium.9 The release of lysosomal enzymes into the cytosol is also associated with the activation of NLRP3 inflammasomes.11 α-Bisabolol pre and co-treatment prevents lysosomal dysfunction mediated inflammasome activation and the associated incidence of inflammation in rats.
A report from Kiernan et al.42 has revealed that enhanced TLR4 activation during hypoxic conditions induces inflammatory signaling. TLR4 is a crucial factor involved in the activation of inflammatory NF-κB and MAPK signaling cascades downstream to TLR4 including JNK, P38 and ERK1/2.43 It is well known that MAPK and NF-κB activations walk hand in hand in response to oxidative stress.44 The proteosomal degradation of the NF-κB inhibitors (IκBs) during the activation of TLR4 enables the translocation of NF-κB into the nucleus45 and it plays an important role in the secretion of pro-inflammatory cytokines.46 NF-κB-p65 is an active subunit of NF-κB heterodimers and is one of the key transcription factors in the regulation of inflammatory response.20 Activated NF-κB triggers the transcription of pro-inflammatory genes (TNF-α, IL-1β and IL-6) and regulates the inflammatory mediators (iNOS and COX-2) which are known to play pivotal roles in the pathogenesis of acute and chronic inflammation.46,47 Evidence from histological findings has revealed inflammatory cells in the myocardium with the separation of cardiac muscle fibers in ISO induced rats. The activation of NLRP3 is inevitable during ischemic conditions due to the increased production of ROS via up-regulation of TXNIP which aggravates myocardial tissue injury with the aid of elevated myocardial IL-1β and IL-18 levels.48 The recruitment of ASC and the procaspase-1 promoted by NLRP3 results in the formation of mature IL-1β. The activation of NLRP3 inflammasomes is closely associated with the activation of NF-κB and MAPK signaling cascades, which acts downstream of TLRs under ischemic conditions.49 To reveal the potential of α-bisabolol against inflammation, the activation of NLRP3 inflammasomes in ISO induced rats was studied. As we assumed, α-bisabolol significantly suppressed NLRP3 inflammasome activation. Therefore, the inhibition of TLR4 mediated NF-κB and NLRP3 signaling pathways is one of the mechanisms of α-bisabolol in protecting the myocardium against inflammation which is evidenced by the histological and molecular findings of our study in ISO induced myocardial infarcted rats.
Enhanced autophagic flux possesses the ability to inhibit NF-κB activation by suppressing the release of IκBα, an inhibitory subunit from the latent cytoplasmic form of NF-κB.50 The autophagy mechanism eliminates the damaged organelles by promoting their turnover and prevents the accumulation of highly fragile and aging organelles.51 Mitochondrial damage and accumulation are found in line with the awakening of NLRP3 inflammasomes via the release of mitochondrial DNA.52 Moreover, the autophagosome engulfs IL-1β and delivers into the lysosomal machinery for degradation.53 The inhibition of autophagic flux enhances NLRP3-inflammasome mediated inflammation which leads to various inflammatory disorders. On the other hand, activation of autophagy abrogates the production of NLRP3-inflammasome mediated production of IL-1β and IL-18 thereby preventing tissue damage. It also inhibits the TLR4 mediated non canonical inflammasome activation by eliminating damaged phagosomes and prevents the production of IL-1β and pyroptosis (Fig. 8).51 Hence, autophagy prevents excessive inflammation by disrupting multiple pathways of inflammasomes. Interestingly, our study showed that α-bisabolol has the potential to augment autophagic flux as evidenced by molecular and ultrastructural findings which show that autophagic vacuoles contain acid phosphatase activity (black deposits) indicating that fusion with lysosomes has occurred. Thus, α-bisabolol protects the myocardium from inflammation and inhibits NLRP3 inflammasome activation by enhancing autophagic flux in ISO induced myocardial infarcted rats.
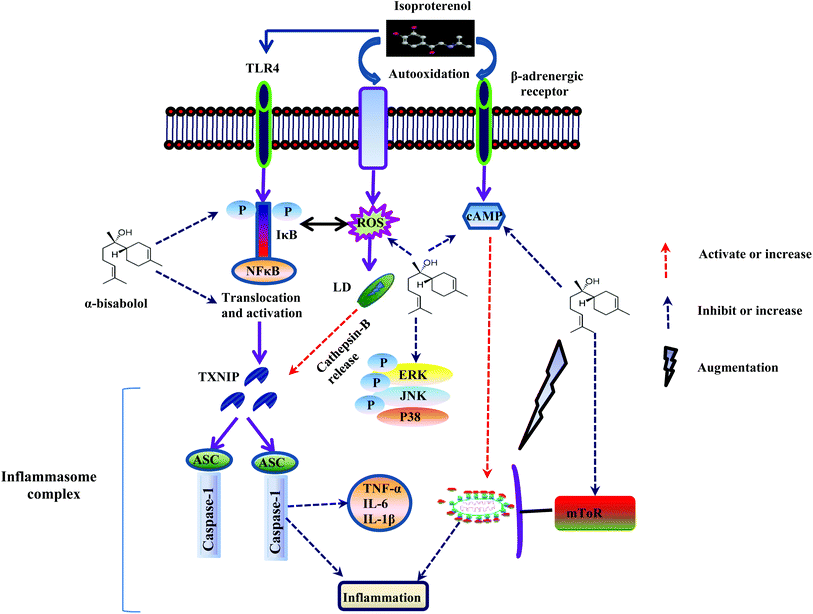 |
| Fig. 8 Schematic representation of the effect of α-bisabolol against ISO-induced inflammation, lysosomal dysfunction, NLRP3 inflammasome activation and the associated complications. | |
Conclusions
The present study findings revealed the anti-inflammatory effects of α-bisabolol in ISO-induced MI in rats. The underlying mechanism of the observed cardioprotective effects of α-bisabolol involves amelioration of inflammation by preventing activation of NLRP3 inflammasomes via inhibiting oxidative stress mediated activation of TLR4-NF-κB/MAPK signaling and lysosomal dysfunction in ISO-induced MI in rats. Additionally, the potential of α-bisabolol in enhancing autophagic flux was also showed to play a crucial factor in the inhibition of NLRP3 inflammasome activation in ISO-induced MI in rats.
Author contributions
MFNM designed the study and performed the biochemical analysis and western blotting. He also analyzed and compiled the data and wrote the first draft manuscript. SA carried out the animal work (dosing), ELISA and histology. FL performed the TEM study and chemical preparations. ST did the supervision of the TEM study. SG and EA provided critical inputs in revising the manuscript. SG involved in manuscript correction. SO secured the funding for the research, designed and supervised the study.
Funding
This work was supported by a UPAR grant (Grant No. 31M195) from UAE University, Al Ain, Abu Dhabi.
Conflicts of interest
The authors declare no conflict of interest.
Acknowledgements
The authors wish to thank United Arab Emirates University for the funding and also Dr Saeed Tariq, Department of Anatomy, United Arab Emirates University for carrying out the Transmission Electron Microscopy study.
References
- T. Tanaka and K. Ozaki, Inflammation as a risk factor for myocardial infarction, J. Hum. Genet., 2006, 51, 595–604 CrossRef CAS PubMed.
- A. P. Ithayarasi and C. S. Devi, Effect of alpha tocopherol on lipid peroxidation in isoproterenol induced myocardial infarction in rats, Indian J. Physiol. Pharmacol., 1997, 41, 369–376 CAS.
- M. F. Nagoor Meeran, G. S. Jagadeesh and P. Selvaraj, Thymol, a dietary monoterpene phenol abrogates mitochondrial dysfunction in β-adrenergic agonist induced myocardial infarcted rats by inhibiting oxidative stress, Chem.-Biol. Interact., 2016, 244, 159–168 CrossRef CAS PubMed.
- M. F. Nagoor Meeran and P. Stanely Mainzen Prince, Protective effects of thymol on altered plasma lipid peroxidation and nonenzymic antioxidants in isoproterenol-induced myocardial infarcted rats, J. Biochem. Mol. Toxicol., 2012, 26, 368–373 CrossRef CAS PubMed.
- M. F. Nagoor Meeran, F. Laham, H. Al-Taee, S. Azimullah and S. Ojha, Protective effects of α-bisabolol on altered hemodynamics, lipid peroxidation, and nonenzymatic antioxidants in isoproterenol-induced myocardial infarction: In vivo and in vitro evidences, J. Biochem. Mol. Toxicol., 2018, 32(10) DOI:10.1002/jbt.22200.
- P. Mukthambaa and K. Srinivasan, Hypolipidemic influence of dietary fenugreek (Trigonella foenum-graecum) seeds and garlic (Allium sativum) in experimental myocardial infarction, Food Funct., 2015, 6, 3117–3125 RSC.
- J. Wang, W. Shen, J.-Y. Zhang, C.-H. Jiaa and M.-L. Xie, Stevioside attenuates isoproterenol-induced mouse myocardial fibrosis through inhibition of the myocardial NF-κB/TGF-β1/Smad signaling pathway, Food Funct., 2019, 10, 1179–1190 RSC.
- S. N. Goyal, C. Sharma, U. B. Mahajan, C. R. Patil, Y. O. Agrawal, S. Kumari, D. S. Arya and S. Ojha, Protective effects of cardamom in isoproterenol-induced myocardial infarction in rats, Int. J. Mol. Sci., 2015, 16(11), 27457–27469 CrossRef CAS PubMed.
- M. F. Nagoor Meeran, G. S. Jagadeesh and P. Selvaraj, Thymol attenuates inflammation in isoproterenol induced myocardial infarcted rats by inhibiting the release of lysosomal enzymes and downregulating the expressions of proinflammatory cytokines, Eur. J. Pharmacol., 2015, 754, 153–161 CrossRef CAS PubMed.
- M. Mari Kannan and S. Darlin Quine, Pharmacodynamics of ellagic acid on cardiac troponin-T, lyosomal enzymes and membrane bound ATPases: mechanistic clues from biochemical, cytokine and in vitro studies, Chem.-Biol. Interact., 2011, 193, 154–161 CrossRef CAS PubMed.
- Y. Chen,
et al., Endothelial Nlrp3 inflammasome activation associated with lysosomal destabilization during coronary arteritis, Biochim. Biophys. Acta, 2015, 1853, 396–408 CrossRef CAS PubMed.
- E. Welman, A. P. Selwyn, T. J. Peters, J. F. Colbeck and K. M. Fox, Plasma lysosomal enzyme activity in acute myocardial infarction, Cardiovasc. Res., 1978, 12, 99–105 CrossRef CAS PubMed.
- R. Ross, Atherosclerosis-an inflammatory disease, N. Engl. J. Med., 1999, 340, 115–126 CrossRef CAS PubMed.
- M. R. Cusack, M. S. Marber, P. D. Lambiase, C. A. Bucknall and S. R. Redwood, Systemic inflammation in unstable angina is the result of myocardial necrosis, J. Am. Coll. Cardiol., 2002, 39, 1917–1923 CrossRef CAS PubMed.
- D. Y. Fann, Y. A. Lim, Y. L. Cheng, K. Z. Lok, P. Chunduri and S. H. Baik, Evidence that NF-κB and MAPK signaling promotes NLRP inflammasome activation in neurons following ischemic stroke, Mol. Neurobiol., 2018, 55, 1082–1096 CrossRef CAS PubMed.
- J. Yang,
et al., Astragaloside IV attenuates inflammatory cytokines by inhibiting TLR4/NF-кB signaling pathway in isoproterenol-induced myocardial hypertrophy, J. Ethnopharmacol., 2013, 150, 1062–1070 CrossRef CAS PubMed.
- F. G. Bauernfeind,
et al., Cutting edge: NF-kappaB activating pattern recognition and cytokine receptors license NLRP3 inflammasome activation by regulating NLRP3 expression, J. Immunol., 2009, 183, 787–791 CrossRef CAS PubMed.
- B. Luo,
et al., Rosuvastatin alleviates diabetic cardiomyopathy by inhibiting NLRP3 inflammasome and MAPK pathways in a type 2 diabetes rat model, Cardiovasc. Drugs Ther., 2014, 28, 33–43 CrossRef CAS PubMed.
- R. Zhou, A. Tardivel, B. Thorens, I. Choi and J. Tschopp, Thioredoxin interacting protein links oxidative stress to inflammasome activation, Nat. Immunol., 2010, 11, 136–140 CrossRef CAS PubMed.
- H. P. Mao, X. Y. Wang, Y. H. Gao, Y. X. Chang, L. Chen and Z. C. Niu, Danhong injection attenuates isoproterenol-induced cardiac hypertrophy by regulating p38 and NF-κB pathway, J. Ethnopharmacol., 2016, 186, 20–29 CrossRef CAS PubMed.
- O. Bhat, N. Meng, X. Yuan, H. P. Lohner and L. Li, Protective role of autophagy in NLRP3 inflammasome activation and medial thickening of mouse coronary arteries, FASEB J., 2017, 31, 2948–2959 CrossRef PubMed.
- A. Orav, A. Raal and E. Arak, Content and composition of the essential oil of Chamomilla recutita (L.) Rauschert from some European countries, Nat. Prod. Res., 2010, 24, 48–55 CrossRef CAS PubMed.
- G. P. Kamato, N. P. Makunga, W. P. Ramogola and A. M. Viljoen, South African Salvia species: a review of biological activities and phytochemistry, J. Ethnopharmacol., 2008, 119, 664–672 CrossRef PubMed.
- R. Vila,
et al., Composition and biological activity of the essential oil from leaves of Plinia cerrocampanensis, a new source of alpha-bisabolol, Bioresour. Technol., 2010, 101, 2510–2514 CrossRef CAS PubMed.
- A. T. De Souza,
et al., Supercritical extraction process and phase equilibrium of Candeia (Eremanthus erythropappus) oil using supercritical carbon dioxide, J. Supercrit. Fluids, 2008, 47, 182–187 CrossRef CAS.
- P. C. Braga, M. Dal Sasso, E. Fonti and M. Culici, Antioxidant activity of bisabolol: inhibitory effects on chemiluminescence of human neutrophil bursts and cell free systems, Pharmacology, 2009, 83, 110–115 CrossRef CAS PubMed.
- V. Jakovlev, O. Isaac, K. Thiemer and R. Kunde, Pharmacological investigations with compounds of chamomile: II. New investigations on the antiphlogistic effects of (-)-alpha-bisabolol and bisabolol oxides, Planta Med., 1979, 35, 125–140 CrossRef CAS PubMed.
- N. F. Moura Rocha,
et al., Gastroprotection of (-)- alpha-bisabolol on acute gastric mucosal lesions in mice: the possible involved pharmacological mechanisms, Fundam. Clin. Pharmacol., 2010, 24, 63–71 CrossRef PubMed.
- B. F. Brehm-Stecher and E. A. Johnson, Sensitization of Staphylococcus aureus and Escherichia coli to antibiotics by the sesquiterpenoids nerolidol, farnesol, bisabolol, and apritone, Antimicrob. Agents Chemother., 2003, 47, 3357–3360 CrossRef CAS PubMed.
- T. Seki,
et al., Antitumor effects of α-bisabolol against pancreatic cancer, Cancer Sci., 2011, 102, 2199–2205 CrossRef CAS PubMed.
- S. Ojha, S. Goyal, C. Sharma, S. Arora, S. Kumari and D. S. Arya, Cardioprotective effect of lycopene against isoproterenol-induced myocardial infarction in rats, Hum. Exp. Toxicol., 2013, 32, 492–503 CrossRef CAS PubMed.
- P. N. Khalil,
et al., Histochemical assessment of early myocardial infarction using 2,3,5-triphenyltetrazolium chloride in bloodperfused porcine hearts, J. Pharmacol. Toxicol. Methods, 2006, 54, 307–312 CrossRef CAS PubMed.
- C. G. Fraga, B. E. Leibovitz and A. L. Tappel, Lipid peroxidation measured as thiobarbituric acid-reactive substances in tissue slices: characterization and comparison with homogenates and microsomes, Free Radical Biol. Med., 1988, 4, 155–161 CrossRef CAS PubMed.
- P. Kakkar, B. Das and P. N. Viswanathan, A modified spectrophotometric assay of superoxide dismutase, Indian J. Biochem. Biophys., 1984, 21, 130–132 CAS.
- A. K. Sinha, Colorimetric assay of catalase, Anal. Biochem., 1972, 47, 389–394 CrossRef CAS PubMed.
- V. Sathish, K. K. Ebenezar and T. Devaki, Synergistic effect of nicorandil and amlodipine on lysosomal hydrolases during experimental myocardial infarction in rats, Biomed. Pharmacother., 2003, 57, 309–313 CrossRef CAS.
- Y. Kawai and K. Anno, Mucopolysaccharide-degrading enzymes from the liver of the squid. Ommastrephes sloanipacificus I. Hyaluronidase, Biochim. Biophys. Acta, 1971, 242, 428–436 CrossRef CAS.
- J. Conchie, A. L. Gelman and G. A. Levvy, Inhibition of glycosidases by aldonolactones of corresponding configuration. The C-4 and C-6- specificity of beta-glucosidase and beta-galactosidase, Biochem. J., 1967, 103, 609–615 CrossRef CAS PubMed.
- A. J. Barrett, A new assay for cathepsin B1 and other thiol proteinases, Anal. Biochem., 1972, 47, 280–293 CrossRef CAS PubMed.
- A. I. Sapolsky, R. D. Altman and D. S. Howell, Cathepsin D activity in normal and osteoarthritic human cartilage, Fed. Proc., 1973, 32, 1489–1493 CAS.
- M. F. Nagoor Meeran, G. S. Jagadeesh and P. Selvaraj, Catecholamine toxicity triggers myocardial membrane destabilization in rats: thymol and its counter action, RSC Adv., 2015, 5, 43338–43344 RSC.
- E. A. Kiernan, S. M. C. Smith, G. S. Mitchell and J. J. Watters, Mechanisms of microglial activation in models of inflammation and hypoxia: Implications for chronic intermittent hypoxia, J. Physiol., 2016, 594, 1563–1577 CrossRef CAS PubMed.
- Y. Li,
et al., MAPK signaling downstream to TLR4 contributes to paclitaxel- induced peripheral neuropathy, Brain, Behav., Immun., 2015, 49, 255–266 CrossRef CAS PubMed.
- G. Abdelhamid and A. O. El-Kadi, Buthionine sulfoximine, an inhibitor of glutathione biosynthesis, induces expression of soluble epoxide hydrolase and markers of cellular hypertrophy in a rat cardiomyoblast cell line: Roles of the NF-κB and MAPK signaling pathways, Free Radical Biol. Med., 2015, 82, 1–12 CrossRef CAS PubMed.
- T. Kawai and S. Akira, The role of pattern-recognition receptors in innate immunity: Update on toll- like receptors, Nat. Immunol., 2010, 11, 373–384 CrossRef CAS PubMed.
- J. Yang, Z. Wang and D. L. Dong-Lin Chen, Shikonin ameliorates isoproterenol (ISO)-induced myocardial damage through suppressing fibrosis, inflammation, apoptosis and ER stress, Biomed. Pharmacother., 2017, 93, 1343–1357 CrossRef CAS PubMed.
- D. H. Kim,
et al., Ginsenoside Rd inhibits the expressions of iNOS and COX-2 by suppressing NF-κB in LPS-stimulated RAW264.7 cells and mouse liver, J. Ginseng Res., 2013, 37, 54–63 CrossRef CAS PubMed.
- S. D. Anker and S. von Haehling, Inflammatory mediators in chronic heart failure: an overview, Heart, 2004, 90, 464–470 CrossRef CAS PubMed.
- K. Z. Lok, M. Basta, S. Manzanero and T. V. Arumugam, Intravenous immunoglobulin (IVIg) dampens neuronal toll-like receptor-mediated responses in ischemia, J. Neuroinflammation, 2015, 12, 73 CrossRef PubMed.
- X. Wu,
et al., Impaired autophagy contributes to adverse cardiac remodeling in acute myocardial infarction, PLoS One, 2014, 9, e112891 CrossRef PubMed.
- T. Saitoh and S. Akira, Regulation of inflammasomes by autophagy, J. Allergy Clin. Immunol., 2016, 138, 28–36 CrossRef CAS.
- K. Nakahira,
et al., Autophagy proteins regulate innate immune responses by inhibiting the release of mitochondrial DNA mediated by the NALP3 inflammasome, Nat. Immunol., 2011, 12, 222–230 CrossRef CAS PubMed.
- J. Harris,
et al., Autophagy controls IL-1beta secretion by targeting pro-IL-1beta for degradation, J. Biol. Chem., 2011, 286, 9587–9597 CrossRef CAS PubMed.
|
This journal is © The Royal Society of Chemistry 2020 |
Click here to see how this site uses Cookies. View our privacy policy here.