DOI:
10.1039/C9FO01414D
(Paper)
Food Funct., 2020,
11, 524-533
Bioactive peptides derived from crimson snapper and in vivo anti-aging effects on fat diet-induced high fat Drosophila melanogaster
Received
1st July 2019
, Accepted 17th November 2019
First published on 18th November 2019
Abstract
Crimson snapper is an important marine economy fish, and its scales are rich in collagen. Crimson snapper scale peptides (CSSPs) obtained from crimson snapper scales by enzymatic hydrolysis exhibited significantly antioxidant activities to DPPH, ABTS and hydroxyl radicals in vitro. In vivo antioxidant and anti-aging effects of CSSPs on the Drosophila melanogaster aging model constructed by lard were investigated in this study. The results showed that CSSPs could prolong the mean lifespan, 50% survival days and the maximum lifespan of Drosophila, and especially when the concentration of CSSPs was increased to 6 mg mL−1, the mean lifespan of male and female Drosophila increased by 21.2% and 26.2%, respectively, indicating the effective life extension effect of CSSPs. Meanwhile, the addition of CSSPs effectively reduced the contents of malondialdehyde (MDA) and protein carbonylation (PCO) and increased the levels of total superoxide dismutase (T-SOD) and catalase (CAT) in Drosophila, which showed a significant dose dependence. Besides, the antioxidant-related genes SOD1, SOD2 and CAT were up-regulated in Drosophila when fed with diets containing CSSPs. In conclusion, CSSPs played an antioxidant and anti-aging role by reducing the accumulation of peroxide products and improving the activity of antioxidant enzymes, as well as by up-regulating the expression of antioxidant-related genes. The results suggest that CSSPs have potential to be used in the food and healthcare industries as potential food-borne antioxidant and anti-aging agents.
1. Introduction
During life activities, the aerobic metabolism of organisms causes the mitochondria to produce reactive oxygen species (ROS), such as superoxide anion radicals (O2˙−), hydroxyl radicals (˙OH), and hydroperoxide groups (HO2˙). These free radicals, as a metabolite of organisms, are indispensable for the normal life activities of organisms.1 Under normal physiological conditions, organisms have internal antioxidant enzyme defense systems such as SOD, CAT and glutathione peroxidase (GSH-Px), and the generation and scavenging of free radicals are in a relatively balanced state.2 However, this balance is broken when the organism is stimulated by the external environment, and the free radicals accumulate in large quantities, causing damage to the mitochondria, resulting in mitochondrial dysfunction, which further leads to cell death and metabolism disorders, causing damage to cells and tissues. Based on this, Harman proposed the widely accepted theory of free radical aging.3 This theory shows that there is an inevitable connection between human aging and excessive accumulation of free radicals. Thus maintaining the dynamic balance of free radicals in living organisms is of great significance for the extension of lifespan and the postponement of aging.
Crimson snapper (Lutjanus erythropterus) is an important commercial fish, which is mainly distributed in the Red Sea, the Indian Ocean, the South China Sea and Taiwan. The scales are scraps produced during the production of canned and minced fish products. The waste utilization of scales not only reduces environmental pollution but also increases the economic value of aquatic products. Crimson snapper scales are rich in collagen and are ideal sources for the preparation of bioactive peptides such as antioxidant peptides. In fact, numerous studies have been reported on the preparation of antioxidant peptides using collagen.4–7 The mechanism by which antioxidant peptides exert antioxidant effects mainly includes providing hydrogen atoms or electrons to participate in scavenging free radical reactions, forming an inert complex with metal ions by chelation to inhibit the formation of ROS and free radicals and up-regulating the levels of endogenous antioxidant enzymes such as SOD, CAT and GSH-Px.8,9 Antioxidant peptides have the ability to effectively scavenge free radicals in vitro, suggesting that they may also have the ability to scavenge excess free radicals and improve antioxidant enzyme activity in organisms, thus achieving anti-aging effects.
Drosophila melanogaster (fruit fly) is an animal model commonly used in genetics and epigenetics studies.10 Many metabolic pathways, physiological conditions and growth and development of Drosophila are basically similar to those of mammals. Drosophila has similar growth, reproduction, and senescence stages as humans and also shows a decline in exercise and cognitive behavior during aging.11 At the genetic level, Drosophila genes have very high homology with other species. For example, Drosophila has homology with more than 70% of the known pathogenic genes in humans,12 making it an ideal material for gene research. In addition, Drosophila is similar to mammals in protein molecular basis, the signal transduction pathway, the synaptic transmission mechanism and the neural coding mode, as well as the occurrence and pathogenesis of neurological diseases.13 These advantages make Drosophila become an excellent model for the study of the aging process and related diseases.
Recent evidence suggests that high-fat diets increased the accumulation of lipid hydroperoxides, accelerated the aging process, and induced higher mortality in Drosophila.14,15 Besides, the high-fat diets reduced insulin sensitivity and shortened the lifespan of Drosophila by activating the body's JAK-STAT signaling pathway.15 Therefore, in this study, antioxidant peptides were prepared from crimson snapper scales. While measuring their antioxidant capacity in vitro, the aging model of Drosophila melanogaster fed on a high-fat diet was used to explore their antioxidant and anti-aging effects in vivo. The results suggest that crimson snapper scale peptides have potential to be used as antioxidant and anti-aging agents in the food and healthcare industries.
2. Materials and methods
2.1. Materials
The crimson snapper scales used in this research were kindly provided by Sea-100 Food Co., Ltd (Fujian, China) and stored in a dry environment. The commercial protease, papain (EC 3.4.22.2), was purchased from Sigma-Aldrich Chemical Co. (St Louis, USA). 1,1-Diphenyl-2-picrylhydrazyl (DPPH) was obtained from Tokyo Chemical Industry Co., Ltd and 2,2′-azinobis-3-ethylbenzthiazoline-6-sulphonate (ABTS) was purchased from Shanghai Macklin Biochemical Co., Ltd. All the commercial kits used in the experiments were purchased from Nanjing Jiancheng Bioengineering Co. (Nanjing, China). The RNAprep pure Tissue Kit and RealUniversal Color PreMix (SYBR Green) were purchased from TIANGEN BIOTECH (Beijing, China) Co., Ltd. TransScript® One-Step gDNA Removal and cDNA Synthesis SuperMix was purchased from TransGen Biotech Co., Ltd (Beijing, China). Primers used for the gene analysis were synthesized by Sangon Biotech (Shanghai, China). All other reagents were of analytical purity.
2.2. Preparation of CSSPs
In brief, the crimson snapper scales were washed, dried, purified and ground into powder. Subsequently, the scales were dispersed in deionized water (6%, w/w) and subjected to ultrasonic processing at 50 °C for 1.5 h, and then they were treated at 121 °C and 0.1 MPa for 1 h to make the scales dissolve in collagen. Based on the screening of hydrolysis conditions to produce peptides with antioxidant activities (data not shown), the scales were then hydrolyzed with 9.6% papain (w/w) for 7.8 h at 60 °C after adjusting the pH of the mixture to 5.8. After hydrolysis, the hydrolysates were heated to 100 °C for 10 min to inactivate the protease and then centrifuged at 10
000 rpm for 10 min and the supernatant was lyophilized which was named CSSPs.
2.3. Analysis of the amino acid composition and molecular mass distribution of CSSPs
The amino acid composition and content of CSSPs were determined as described in our previous work using a High Speed Amino Acid Analyzer Model L-8900 (Hitachi Co., Japan). The peptides had been hydrolyzed in 6 M HCl at 110 °C for 24 h before being determined.16
The molecular mass distribution of CSSPs was determined by using a HPLC system (Waters™ 650E Advanced Protein Purification System, Waters Corporation, Milford, MA, USA) at the Instrumental Analysis Center of Jiangnan University (Jiangsu, China). The operating conditions were the same as those used in the method of Yang et al.17
2.4.
In vitro antioxidant activity assays
2.4.1. DPPH radical scavenging assay.
An appropriate amount of CSSPs was dissolved in distilled water to make different concentrations of CSSP sample solutions. The method of DPPH radical scavenging activity was based on that reported in the study by Wu et al.18 Briefly, 0.5 mL of sample was fully mixed with 0.5 mL of DPPH (0.1 mM, 95% ethanol) and incubated in the dark at room temperature for 30 min, and then the absorbance at 517 nm was measured. Meanwhile, DPPH was replaced by 95% ethanol as the sample reference, and the blank group was 0.5 mL of DPPH mixed with 0.5 mL of 95% ethanol. The DPPH radical scavenging activity of the sample was calculated according to the following formula: | DPPH scavenging activity (%) = (1 − (Ai − Aj)/A0) × 100, | (1) |
where A0 is the absorbance of the blank group. Ai and Aj are the absorbance of the sample and reference groups, respectively.
2.4.2. ABTS radical scavenging assay.
The determination of ABTS radical scavenging activity was carried out in accordance with the method described by Wang et al.19 7 mM of ABTS solution and 2.45 mM of K2S2O8 solution were mixed in the same volume and incubated in the dark for 16 h. Then the absorbance of the mixture was adjusted to 0.70 ± 0.02 at 734 nm using phosphate buffer (5 mM, pH 7.4) before used. The mixture was added to the sample in equal volume and incubated in the dark for 10 min, and then the absorbance was measured at 734 nm. The blank group replaced the sample with distilled water. The ABTS radical scavenging activity of the sample was calculated as follows: | ABTS scavenging activity (%) = (A0 − Ai)/A0 × 100, | (2) |
where A0 and Ai are the absorbance of the blank and sample groups, respectively.
2.4.3. Hydroxyl radical scavenging assay.
The hydroxyl radical scavenging activity of CSSP samples was measured according to the method described previously.20 0.5 mL of sample was mixed with 150 μL of FeSO4 (8 mM), 0.5 mL of salicylic acid (3 mM) and 125 μL of H2O2 (20 mM) and incubated at 37 °C for 30 min. After cooling with water, 225 μL of distilled water was added to make the total volume reach 1.5 mL and then centrifuged at 3000 rpm for 10 min. The absorbance of the supernatant was measured at 510 nm, and distilled water was used instead of the sample as the blank control. The hydroxyl radical scavenging activity was calculated according to the following formula: |  | (3) |
where Acontrol and Asample are the absorbance of the blank and sample groups, respectively.
2.5. Fly husbandry and diet preparation
The fly strain used in this study was wild-type Drosophila melanogaster Canton-S, which was obtained from the Shanghai Institute of Biochemistry and Cell Biology. The basal diet contains 7.3% corn flour (w/v), 1.3% soybean meal (w/v), 0.8% agar (w/v), 2.0% glucose (w/v), 2.0% saccharose (w/v) and 0.3% yeast (w/v). Besides, 0.2% methylparaben (w/v) and 0.8% propanoic acid (v/v) were added to prevent mold growth. To make a high fat diet, we added 10% lard (w/v) and emulsifier 1% Tween-20 (v/v) to the basal diet and well mixed. For rearing the flies, 5 mL of the diet was prepared per vial. All the flies were raised in a temperature humidity chamber at 25 °C and a relative humidity of 60% with a 12 h dark/light cycle.
2.6. Lifespan analysis
Newly enclosed flies were separated by sex and then randomly divided into five groups (200 flies per group, 25 flies per vial). The non-lard control group (NCT) was fed with basal diet. The lard control group (LCT) was fed with high fat diet. The other three groups (CSSP1, CSSP3 and CSSP6) were raised on high fat diet containing 1 mg mL−1, 3 mg mL−1, and 6 mg mL−1 CSSPs, respectively. All groups were transferred to the corresponding vials containing fresh diet every 3 or 4 days. Everyday the dead flies were counted to analyze the prolonging lifespan effect of CSSPs on Drosophila melanogaster.
2.7. Feeding assay
The method of measuring the food intake of flies was based on that reported by Xiao-xuan Xin with some modifications.21 Newly enclosed flies (either 20 female or 20 male flies) were collected and raised on different experimental diets for 3 days. Subsequently, the flies were starved for 1 hour and then transferred to the corresponding diets containing 0.5% (w/w) brilliant blue for 5 hours. After this, the flies were frozen and homogenized in 1.2 mL of normal saline and then centrifuged at 10
000 rpm for 2 min. 0.9 mL of supernatant was taken and normal saline was added to it to a final volume of 1.2 mL. The solution was filtered through a 0.22 μm membrane and the absorbance was measured at 625 nm.22 Besides, dyed diets were treated in the same way and finally quantified the food intake of flies through the standard curve of the absorbance-diet weight.
2.8. Fly weight measurement
At the age of 1 day, files were collected and raised on experimental diets. After 14 days, the flies were anesthetized with CO2 and transferred to empty vials to starve for 30 min, and then the body weight of files (30 flies per vial) was measured.
2.9. Climbing ability test
Drosophila has a living habit of climbing upwards in an enclosed space, and so it is possible to measure the body's motor function through its climbing ability. The climbing ability can be expressed by the number of flies that climb up a fixed distance within a specified time. In brief, 30 newly enclosed flies were raised on each diet for 14 days and then transferred to an empty vial. Subsequently, the vial was gently shaken so that the flies could fall to the bottom, and then the flies began climbing up. The number of flies climbing upwards more than 7 cm within 30 s was recorded. The climbing ability can be expressed as the percentage of flies that climbed more than 7 cm. Each experimental group was repeated three times.
2.10.
In vivo antioxidant activity
Flies were raised on various diets for 7 and 21 days and were sacrificed by CO2 anesthesia and then frozen at −20 °C. Then, the mixture of 100 mg of flies and 0.9 mL of normal saline was homogenized in an ice bath and centrifuged at 10
000 rpm for 10 min to prepare 10% tissue homogenate. The total protein content in flies was determined according to the kit instructions, and the tissue homogenate concentration was adjusted according to the instructions to measure the T-SOD and CAT activities or MDA and PCO contents in flies.
2.11. Analysis of antioxidant-related gene expression
Flies were fed with the corresponding diets for 21 days. The total RNA of flies was extracted using the RNAprep Pure Tissue Kit. The cDNA was constructed by using TransScript® One-Step gDNA Removal and cDNA Synthesis SuperMix. A q-PCR (quantitative polymerase chain reaction) was carried out on a Bio-Rad CFX Connect™ Real-Time System (Bio-Rad Laboratories, Inc., US). The antioxidant genes included SOD1 (5′-GCGGCGTTATTGGCATTG-3′, 3′-ACTAACAGACCACAGGCTATG-5′), SOD2 (5′-CACATCAACCACACCATCTTC-3′, 3′-GCTCTTCCACTGCGACTC-5′) and CAT (5′-TGAACTTCCTGGATGAGATGTC-3′, 3′-TCTTGGCGGCACAATACTG-5′), which were normalized with RP49 (5′-AGGGTATCGACAACAGAGTG-3′, 3′-CACCAGGAACTTCTTGAATC-5′), a housekeeping gene used for normalization. The expression of the target genes was calculated using the 2−ΔΔCt method.
2.12. Statistics analysis
All results were expressed as mean ± SD. The data of antioxidant activity in vitro had been analyzed by one-way ANOVA using SPSS 19.0. Kaplan–Meier analysis was performed for analyzing the lifespan. The software GraphPad Prism 7.04 was used to analyze the significant difference in the food intake, body weight, climbing ability, antioxidant activity in vivo and gene expression by using the t-test and p < 0.05 was considered as statistically significant.
3. Results and discussion
3.1. Amino acid composition and molecular mass distribution of CSSPs
The amino acid composition plays a key role in the antioxidant activity of peptides, and some amino acids are even the active sites of antioxidant peptides.23 For example, the N-terminal of antioxidant peptides usually contains a hydrophobic amino acid residue, which acts as a hydrogen donor and interacts with other amino acids to enhance the hydrophobic properties of peptides, thereby enhancing the antioxidant capacity.24 In addition, hydrophobic amino acid residues are associated with lipid or fatty acid affinity and can prevent a series of lipid peroxidation reactions, thus inhibiting oxidation. Polar amino acids also contribute greatly to the antioxidant activity of peptides. It has been reported that the higher the proportion of polar amino acids in peptides, the stronger antioxidant activity they tend to have, which is due to the chelation of the side chains, thus inhibiting the oxidation of free radicals.25,26 The amino acid composition of CSSPs is shown in Table 1, in which the hydrophobic amino acid content was as high as 29.91%, and the polar amino acid content was 52.54%, indicating that CSSPs may have strong antioxidant activity.
Table 1 Amino acid composition of CSSPs
Amino acid |
Content (g per 100 g) |
Amino acid |
Content (g per 100 g) |
Asp |
4.51 |
Cys |
2.11 |
Glu |
8.74 |
Val |
2.53 |
Ser |
2.45 |
Met |
1.90 |
His |
3.62 |
Phe |
2.01 |
Gly |
19.20 |
Ile |
1.27 |
Thr |
1.88 |
Leu |
2.24 |
Arg |
5.94 |
Lys |
2.97 |
Ala |
8.18 |
Pro |
11.78 |
Tyr |
1.12 |
|
|
The molecular weight reflects the degree of hydrolysis of fish scales. Peptides with different molecular weights have different antioxidant activities. Studies have shown that peptides with a lower molecular weight tend to have higher antioxidant capacity.27 The molecular weight distribution of CSSPs was determined by HPLC. As shown in Fig. 1, the molecular weight of CSSPs was mainly concentrated at below 3000 Da, accounting for 99.07% of proteolytic products, indicating that all CSSPs were almost small molecular peptides. Again, these results may also contribute to the antioxidant activity of CSSPs.
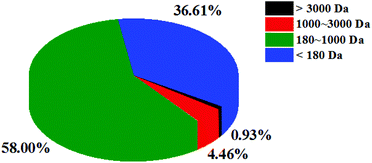 |
| Fig. 1 The molecular mass distribution of CSSPs. | |
3.2.
In vitro antioxidant activities of CSSPs
Free radicals are atoms or groups of unpaired electrons formed by the covalent bonds of a compound molecule under external conditions such as light and heat. Excessive accumulation of free radicals can pose a threat to health through oxidative damage to membrane lipids, proteins and DNA. DPPH and ABTS free radical scavenging assays are common methods to evaluate the antioxidant activity.28,29 In addition, hydroxyl radicals are the most reactive oxygen radicals in biological cells, and the hydroxyl radical scavenging ability can even reveal the antioxidant capacity of the test substance in vivo.30 In this study, DPPH, ABTS and hydroxyl radical scavenging activities were measured to explore the antioxidant activity of CSSPs in vitro, and the results are shown in Fig. 2. The scavenging activities of CSSPs on free radicals exhibited an obvious concentration dependency. The half maximal elimination concentrations (EC50) of CSSPs to DPPH, ABTS and hydroxyl radicals were 1.85 mg mL−1, 56.0 μg mL−1 and 3.73 mg mL−1, respectively. Compared with other antioxidant peptides prepared from marine by-products, CSSPs showed excellent antioxidant activity in vitro and potential use as antioxidants.31
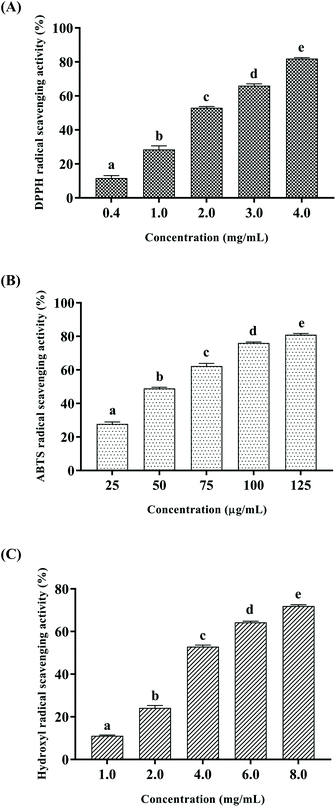 |
| Fig. 2 Antioxidant activities of CSSPs in vitro. (A) DPPH radical scavenging activity, (B) ABTS radical scavenging assay, and (C) hydroxyl radical scavenging assay. Data are represented as mean ± SD. Statistical significances were analyzed by one-way ANOVA. | |
3.3. Effect of CSSPs on the lifespan in flies fed with fat diet
Free radical scavenging experiments in vitro showed that CSSPs had good scavenging activity against radicals. Excessive accumulation of free radicals will damage cells, tissues and organs by attacking biological macromolecules such as proteins, lipids and nucleic acids, cause oxidative damage, accelerate the aging of the body, and induce various chronic diseases.3 Therefore, in view of the antioxidant activity of CSSPs, we speculated that it also had anti-aging activity, which was verified by the survival experiment of Drosophila. The experimental results are shown in Table 2 and Fig. 3. Compared with the NCT group, the LCT group in which 10% lard was added to the basal diet significantly reduced the mean lifespan of both male and female flies by 49.0% and 70.9%, respectively, indicating that excessive dietary fat can reduce the lifespan of Drosophila, which was consistent with the results of K. J. Woodcock and L. Wang.15,32 Surprisingly, the addition of CSSPs to the diets while adding lard increased the mean lifespan, 50% survival days and the maximum lifespan of flies in a concentration-dependent manner. Among them, male flies showed the most significant improvement in the CSSP6 group, with the mean lifespan increased from 21.2 days to 25.7 days and the maximum lifespan increased from 43.7 days to 52.1 days, both of which were significant (p < 0.05). The mean lifespan of female flies in the CSSP6 group increased from 13.0 days to 16.4 days, and 50% survival days increased from 13.1 days to 16.6 days. In addition, the addition of CSSPs in the diets increased the maximum lifespan of female flies in each group. CSSPs showed excellent longevity effects on Drosophila.
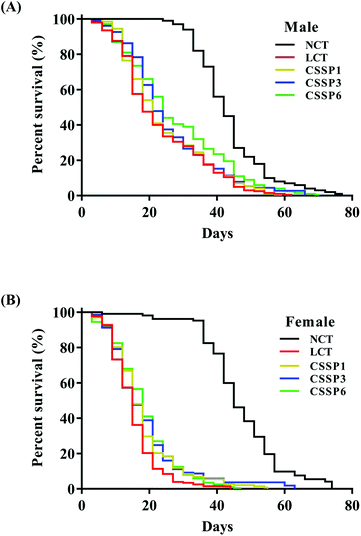 |
| Fig. 3 Survival curves of Drosophila reared on different diets. Survival days of male (A) and female (B) Drosophila (200 flies per group, 25 flies per vial). NCT, non-lard control group; LCT, lard control group. CSSP1, CSSP3 and CSSP6 represent 10% lard diets containing 1 mg mL−1, 3 mg mL−1 and 6 mg mL−1 of CSSPs, respectively. | |
Table 2 Effect of CSSPs at different doses on the lifespan of Drosophila
|
Mean lifespan (days) |
50% survival (days) |
Maximum lifespan (days) |
*p < 0.05 compared with the LCT group of the corresponding gender. |
Male |
NCT |
41.6 ± 2.4 |
41.5 ± 2.5 |
60.6 ± 8.6 |
LCT |
21.2 ± 3.8 |
19.1 ± 6.2 |
43.7 ± 8.0 |
CSSP1 |
22.2 ± 2.1 |
19.9 ± 2.7 |
47.2 ± 7.0 |
CSSP3 |
23.2 ± 1.7 |
21.8 ± 3.1 |
47.3 ± 6.6 |
CSSP6 |
25.7 ± 4.0* |
23.6 ± 5.7 |
52.1 ± 7.1* |
|
Female |
NCT |
44.6 ± 3.3 |
44.5 ± 2.5 |
62.4 ± 7.1 |
LCT |
13.0 ± 2.6 |
13.1 ± 3.0 |
24.8 ± 4.4 |
CSSP1 |
15.7 ± 3.9 |
14.4 ± 3.0 |
34.0 ± 8.9* |
CSSP3 |
15.2 ± 2.2 |
14.5 ± 3.5 |
33.1 ± 7.6* |
CSSP6 |
16.4 ± 2.1* |
16.6 ± 2.5* |
32.6 ± 6.5* |
3.4. Effect of CSSPs on feeding and the average body weight of flies
Dietary restriction (DR) has been shown to be one of the most effective life-prolonging measures in Drosophila.33 Common dietary restrictions include short-term hunger, intermittent fasting, regular fasting, and reduced calorie intake.34 Since the flies were reared on the experimental diets when they just emerged, we were unsure whether adding lard or CSSPs to the diets affected the taste of the food, thereby reducing the feed intake of the flies and thus prolonging the lifespan through DR simulation, and so it was necessary to study the changes in the feed intake of flies. In the experiments, we tracked the changes in the feed intake of flies by adding bright blue to the diets. After eating a diet containing bright blue, the flies’ bellies turned visible blue (Fig. 4A). As shown in Fig. 4A, there was no significant change in the feed intake of male flies in each group, regardless of whether lard or CSSPs were added to the diets. The female flies had different results. After the addition of lard, the feed intake of female flies in each group did not decrease but increased, and there was no significant change in the feed intake of female flies after the addition of CSSPs compared with the LCT group.
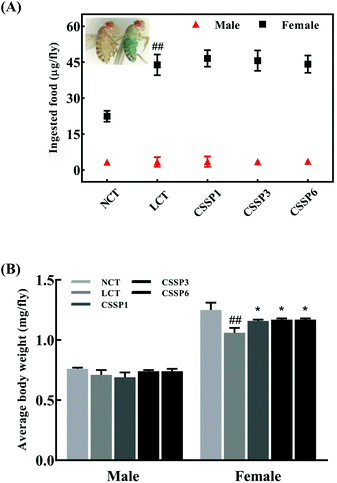 |
| Fig. 4 Effects of CSSPs on feeding (A) and average body weight (B) of Drosophila. Data are represented as mean ± SD. #p < 0.05 and ##p < 0.01 compared with the NCT group of the corresponding gender. *p < 0.05 and **p < 0.01 compared with the LCT group of the corresponding gender. | |
Body weight changes in Drosophila can also be used as an indicator of feed intake. Compared with the NCT group, the body weight of the male experimental groups did not change significantly, which indicated that the weight of male flies was not reduced by DR (Fig. 4B). However, in female flies, the LCT group had a significant weight loss and recovered after the addition of CSSPs. With these results, we eliminated the effect of DR on longevity in Drosophila.
3.5. Effect of CSSPs on the climbing ability of flies
Aging is a process accompanied by a decline in physical activity. Functional, metabolic, and structural degradation of Drosophila skeletal muscle is an age-related process.11Drosophila has a natural negative tendency to crawl. When falling to the bottom of the vial, young and energetic flies quickly climb up, while aging flies cannot climb up or fall back to the bottom of the vial after a period of climbing. Using this method, we investigated the effect of CSSPs on the motor ability of aging flies. As shown in Fig. 5, CSSPs had the most obvious improvement in the climbing ability of male flies. After the addition of 10% lard, the climbing ability of male flies was significantly declined (p < 0.05). While supplementing 3 mg mL−1 or 6 mg mL−1 of CSSPs in the diets, the climbing ability of flies increased significantly (p < 0.01). However, the improvement effect of CSSPs on female flies was not obvious, which was consistent with the phenomenon of abdominal enlargement and reduced movement in female flies that we found in the experiment.
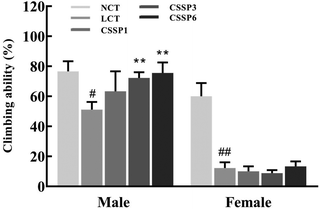 |
| Fig. 5 Effect of CSSPs on the climbing ability of Drosophila. Data are represented as mean ± SD. #p < 0.05 and ##p < 0.01 compared with the NCT group of the corresponding gender. **p < 0.01 compared with the LCT group of the corresponding gender. | |
3.6. Effect of CSSPs on MDA and PCO levels in flies
The levels of lipid peroxidation and protein carbonylation increased with the aging of the body.14,35 In the living body, free radicals act on lipids to induce a peroxidation reaction, and the oxidation end-product is malondialdehyde, which causes cross-linking polymerization of macromolecules such as proteins and nucleic acids, and is cytotoxic. Protein carbonylation is a process of oxidative damage of proteins. The side chain of the amino acid residue is attacked by oxygen free radicals and finally converted into carbonyl products.36 In the experiment, the production of MDA and PCO in fruit flies was measured by using kits to explore the protective effect of CSSPs on oxidative damage in aging flies. As shown in Fig. 6, the MDA level in the LCT group of female flies increased significantly on the 21th day (p < 0.01) and decreased obviously after the addition of 6 mg mL−1 of CSSPs (p < 0.05). The effect of CSSPs on reducing the MDA level was more pronounced in the male groups. On the 7th day, CSSPs had a tendency to reduce the MDA level in male flies, and on the 21th day, CSSPs showed a significant concentration-dependent reduction in MDA. When the CSSP addition concentrations were 1 mg mL−1, 3 mg mL−1 and 6 mg mL−1, the levels of MDA were reduced by 13.4%, 33.3% (p < 0.05) and 36.9% (p < 0.01), respectively.
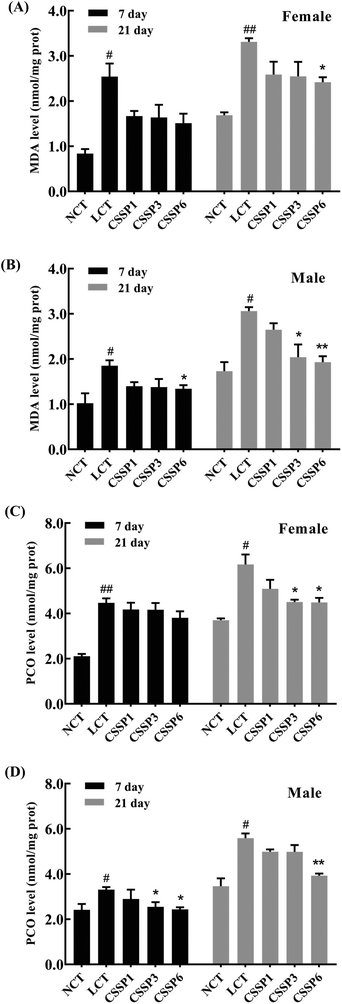 |
| Fig. 6 Effects of CSSPs on MDA (A/B) and PCO (C/D) levels in Drosophila. Data are represented as mean ± SD. #p < 0.05 and ##p < 0.01 compared with the NCT group of the corresponding gender. *p < 0.05 and **p < 0.01 compared with the LCT group of the corresponding gender. | |
CSSPs also significantly reduced the PCO level in aging flies (Fig. 6C and D). On the 21th day, the PCO level of the female flies in CSSP3 and CSSP6 groups was significantly decreased (p < 0.05). The PCO level in CSSP3 and CSSP6 groups of male flies was significantly reduced on day 7 (p < 0.05), and on the 21th day, the PCO level of the CSSP6 group decreased by 29.7% (p < 0.01). These results demonstrate the effective protection of CSSPs against oxidative damage in Drosophila.
3.7. Effect of CSSPs on antioxidant enzyme activities
Organisms produce a large amount of reactive oxygen species (ROS) during metabolism, and the excessive accumulation of these free radicals accelerates aging and death by damaging proteins, cell membrane lipids and DNA. Antioxidant enzymes, by scavenging excess free radicals in the body to achieve the dynamic balance of free radicals in the body, are an important defense system of organisms against environmental stimuli such as external oxidative stress.37 This experiment determined the effect of supplementing different doses of CSSPs on the activities of T-SOD and CAT in Drosophila.
As shown in Fig. 7, the activity of T-SOD and CAT in both female and male flies decreased with the aging of flies, and it was more obvious in the LCT groups. On the 7th day, the addition of CSSPs had no significant effect on the activity of T-SOD and CAT in both female and male flies but began to affect significantly after 21 days, especially in male flies. On the 21th day, the higher the amount of CSSPs added to the diets, the higher the T-SOD activity of male flies. However for female flies, the T-SOD activity could be significantly increased (p < 0.05) when the amount of CSSPs added reached 6 mg mL−1. At the same time, the addition of CSSPs to the diets could significantly improve the CAT activity in both male and female flies, especially in males. When CSSPs were added at 6 mg mL−1, the CAT activity in male flies even increased by 56.6% (p < 0.01); it increased from 16.6 U per mg prot to 26.0 U per mg prot. Although the experimental results showed that the addition of CSSPs could not completely prevent the oxidative damage caused by lard in Drosophila, CSSPs could partially improve antioxidant enzyme activities, indicating the effective antioxidant effect of CSSPs in Drosophila.
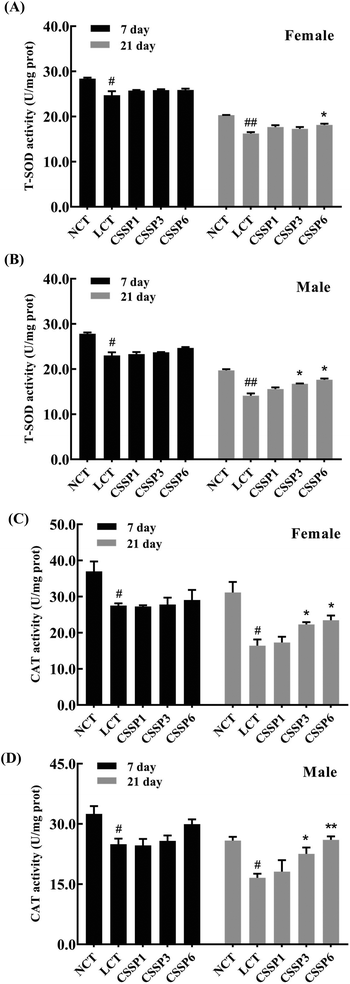 |
| Fig. 7 Effects of CSSPs on T-SOD (A/B) and CAT (C/D) levels in Drosophila. Data are represented as mean ± SD. #p < 0.05 and ##p < 0.01 compared with the NCT group of the corresponding gender. *p < 0.05 and **p < 0.01 compared with the LCT group of the corresponding gender. | |
3.8. Effect of CSSPs on antioxidant-related gene expression
In order to determine the effect of CSSPs on the expression levels of antioxidant-related genes, the q-PCR was employed to measure the mRNA relative expression levels, such as SOD1, SOD2 and CAT. As the results show in Fig. 8, for both male and female flies, compared with the NCT group, the SOD1, SOD2 and CAT gene expressions have varying degrees of decline in the LCT group, and supplementation with CSSPs in the lard diets can up-regulate the expression of these genes, especially in male flies.
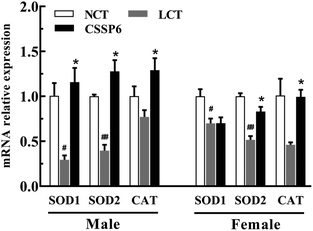 |
| Fig. 8 Effects of CSSPs on antioxidant-related gene (SOD1, SOD2 and CAT) expression. Data are represented as mean ± SD. #p < 0.05 and ##p < 0.01 compared with the NCT group of the corresponding gender. *p < 0.05 compared with the LCT group of the corresponding gender. | |
By measuring the relative expression levels of antioxidant-related genes SOD1, SOD2 and CAT, we found the reason why antioxidant enzyme activities in Drosophila were improved after adding CSSPs to the lard diets. In other words, CSSPs enhanced antioxidant enzyme activities by up-regulating the expression of antioxidant-related genes in the experimental group, so as to improve the antioxidant enzyme system in Drosophila and thus to extend its lifespan.
4. Conclusion
In this study, CSSPs were prepared from crimson snapper scales by enzymatic hydrolysis. According to the molecular weight distribution and amino acid composition analysis, the molecular weight of CSSPs was mainly concentrated at below 3000 Da, and they were rich in hydrophobic amino acids and polar amino acids. In vitro free radical scavenging experiments showed that CSSPs had strong antioxidant activity. The antioxidant and anti-aging experiments of CSSPs in vivo showed that CSSPs could prolong the mean lifespan, 50% survival days and the maximum lifespan of Drosophila. At the same time, the addition of CSSPs can significantly improve the climbing ability of male Drosophila, reduce the levels of MDA and PCO and improve the activities of T-SOD and CAT by up-regulating the expression of antioxidant-related genes SOD1, SOD2 and CAT in Drosophila. This indicated that CSSPs exerted anti-aging effects on Drosophila by eliminating or reducing the levels of peroxide products and improving the antioxidant enzyme system in vivo. As a result, CSSPs have potential to be used as antioxidants and anti-aging agents in the food and healthcare industries.
Conflicts of interest
There are no conflicts to declare.
Acknowledgements
This work was supported by the National Key R&D Program of China (No. 2018YFD0901106) and the Natural Science Foundation of China (No. 31771922).
References
- A. Sanz, R. Stefanatos and G. McIlroy, Production of reactive oxygen species by the mitochondrial electron transport chain in Drosophila melanogaster, J. Bioenerg. Biomembr., 2010, 42, 135–142 CrossRef CAS PubMed.
- A. T. Girgih, R. He, S. Malomo, M. Offengenden, J. Wu and R. E. Aluko, Structural and functional characterization of hemp seed (Cannabis sativa L.) protein-derived antioxidant and antihypertensive peptides, J. Funct. Foods, 2014, 6, 384–394 CrossRef CAS.
- D. Harman, Aging: A Theory Based on Free Radical and Radiation Chemistry, J. Gerontol., 1956, 11, 298–300 CrossRef CAS PubMed.
- L. Cai, X. Wu, Y. Zhang, X. Li, S. Ma and J. Li, Purification and characterization of three antioxidant peptides from protein hydrolysate of grass carp (Ctenopharyngodon idella) skin, J. Funct. Foods, 2015, 16, 234–242 CrossRef CAS.
- R. Wu, C. Wu, D. Liu, X. Yang, J. Huang, J. Zhang, B. Liao and H. He, Antioxidant and anti-freezing peptides from salmon collagen hydrolysate prepared by bacterial extracellular protease, Food Chem., 2017, 248, 346–352 CrossRef PubMed.
- N. M. Sarbon, F. Badii and N. K. Howell, Purification and characterization of antioxidative peptides derived from chicken skin gelatin hydrolysate, Food Hydrocolloids, 2018, 85, 311–320 CrossRef CAS.
- L. Zhang, Y. Zheng, X. Cheng, M. Meng, Y. Luo and B. Li, The anti-photoaging effect of antioxidant collagen peptides from silver carp (Hypophthalmichthys molitrix) skin is preferable to tea polyphenols and casein peptides, Food Funct., 2017, 8, 1698–1707 RSC.
- J. Dai and R. J. Mumper, Plant phenolics: extraction, analysis and their antioxidant and anticancer properties, Molecules, 2010, 15, 7313–7352 CrossRef CAS PubMed.
- M. N. Alam, N. J. Bristi and M. Rafiquzzaman, Review on in vivo, and in vitro methods evaluation of antioxidant activity, Saudi Pharm. J., 2013, 21, 143–152 CrossRef PubMed.
- A. Brandt and A. Vilcinskas, The Fruit Fly Drosophila melanogaster as a Model for Aging Research, Adv. Biochem. Eng./Biotechnol., 2013, 135, 63–77 CrossRef CAS PubMed.
- Y. He and H. Jasper, Studying aging in Drosophila, Methods, 2014, 68, 129–133 CrossRef CAS PubMed.
- N. Minois, How should we assess the impact of genetic changes on ageing in model species?, Ageing Res. Rev., 2006, 5, 52–59 CrossRef CAS PubMed.
- M. A. Jones and M. Grotewiel,
Drosophila as a model for age-related impairment in locomotor and other behaviors, Exp. Gerontol., 2011, 46, 320–325 CrossRef PubMed.
- M. C. Wang, D. Bohmann and H. Jasper, JNK Signaling Confers Tolerance to Oxidative Stress and Extends Lifespan in Drosophila, Dev. Cell, 2003, 5, 811–816 CrossRef CAS.
- K. J. Woodcock, K. Kierdorf, C. A. Pouchelon, V. Vivancos, M. S. Dionne and F. Geissmann, Macrophage-Derived upd3 Cytokine Causes Impaired Glucose Homeostasis and Reduced Lifespan in Drosophila Fed a Lipid-Rich Diet, Immunity, 2015, 42, 133–144 CrossRef CAS PubMed.
- X. Chen, J. Wu, L. Li and S. Wang, Cryoprotective Activity and Action Mechanism of Antifreeze Peptides Obtained from Tilapia Scales on Streptococcus thermophilus during Cold Stress, J. Agric. Food Chem., 2019, 67, 1918–1926 CrossRef CAS PubMed.
- Q. Yang, X. Cai, M. Huang, L. Jia and S. Wang, Immunomodulatory effects of Pseudostellaria heterophylla peptide on spleen lymphocytes via a Ca2+/CaN/NFATc1/IFN-γ pathway, Food Funct., 2019, 10, 3466–3476 RSC.
- H. Wu, H. Chen and C. Shiau, Free amino acids and peptides as related to antioxidant properties in protein hydrolysates of mackerel (Scomber austriasicus), Food Res. Int., 2003, 36, 949–957 CrossRef CAS.
- B. Wang, Z. Li, C. Chi, Q. Zhang and H. Luo, Preparation and evaluation of antioxidant peptides from ethanol-soluble proteins hydrolysate of Sphyrna lewini muscle, Peptides, 2012, 36, 240–250 CrossRef CAS PubMed.
- Y. Zhang, X. Duan and Y. Zhuang, Purification and characterization of novel antioxidant peptides from enzymatic hydrolysates of tilapia (Oreochromis niloticus) skin gelatin, Peptides, 2012, 38, 13–21 CrossRef PubMed.
- X. Xin, Y. Chen, D. Chen, F. Xiao, L. D. Parnell, J. Zhao, L. Liu, J. M. Ordovas, C. Lai and L. Shen, Supplementation with Major Royal-Jelly Proteins Increases Lifespan, Feeding, and Fecundity in Drosophila, J. Agric. Food Chem., 2016, 64, 5803–5812 CrossRef CAS PubMed.
- W. Qiu, X. Chen, Y. Tian, D. Wu, M. Du and S. Wang, Protection against oxidative stress and anti-aging effect in Drosophila of royal jelly-collagen peptide, Food Chem. Toxicol., 2019 DOI:10.1016/j.fct.2019.110881.
- Z. Qian, W. Jung and S. Kim, Free radical scavenging activity of a novel antioxidative peptide purified from hydrolysate of bullfrog skin, Rana catesbeiana Shaw, Bioresour. Technol., 2008, 6, 1690–1698 CrossRef PubMed.
- H. Chen, K. Muramoto, F. Yamauchi and K. Nokihara, Antioxidant Activity of Designed Peptides Based on the Antioxidative Peptide Isolated from Digests of a Soybean Protein, J. Agric. Food Chem., 1996, 44, 2619–2623 CrossRef.
- S. Xiao, J. Wang, K. Jin, X. Jian and Q. Peng, Purification and characterization of antioxidative peptides from protein hydrolysate of lecithin-free egg yolk, J. Am. Oil Chem. Soc., 2001, 78, 651–656 CrossRef.
- A. Saiga, S. Tanabe and T. Nishimura, Antioxidant activity of peptides obtained from porcine myofibrillar proteins by protease treatment, J. Agric. Food Chem., 2003, 51, 3661–3667 CrossRef CAS PubMed.
- N. Chen, H. Yang, Y. Sun, J. Niu and S. Liu, Purification and identification of antioxidant peptides from walnut (Juglans regia L.) protein hydrolysates, Peptides, 2012, 38, 344–349 CrossRef CAS PubMed.
- R. Re, N. Pellegrini, A. Proteggente, A. Pannala, M. Yang and C. RiceEvans, Antioxidant activity applying an improved ABTS radical cation decolorization assay, Free Radical Biol. Med., 1999, 26, 1231–1237 CrossRef CAS.
- O. P. Sharma and T. K. Bhat, DPPH antioxidant assay revisited, Food Chem., 2009, 113, 1202–1205 CrossRef CAS.
- L. G. d. Valle, Oxidative stress in aging: Theoretical outcomes and clinical evidences in humans, Biomed. Pharmacother., 2011, 1, 1–7 Search PubMed.
- A. Sila and A. Bougatef, Antioxidant peptides from marine by-products: Isolation, identification and application in food systems. A review, J. Funct. Foods, 2016, 21, 10–26 CrossRef CAS.
- L. Wang, Y. M. Li, L. Lei, Y. Liu, X. Wang, K. Y. Ma, C. Zhang, H. Zhu, Y. Zhao and Z. Chen, Purple sweet potato anthocyanin attenuates fat-induced mortality in Drosophila melanogaster, Exp. Gerontol., 2016, 82, 95–103 CrossRef CAS PubMed.
- L. Fontana and L. Partridge, Promoting Health and Longevity through Diet: From Model Organisms to Humans, Cell, 2015, 161, 106–118 CrossRef CAS PubMed.
- C. Lee and V. Longo, Dietary restriction with and without caloric restriction for healthy aging, F1000Research, 2016, 5, 117–124 Search PubMed.
- J. Zheng, R. M. II and S. L. Helfand, Calorie restriction delays lipid oxidative damage in Drosophila melanogaster, Aging Cell, 2005, 4, 209–216 CrossRef CAS PubMed.
- J. Zheng and O. A. Bizzozero, Traditional reactive carbonyl scavengers do not prevent the carbonylation of brain proteins induced by acute glutathione depletion, Free Radical Res., 2010, 44, 258–266 CrossRef CAS.
- X. Cai, Q. Huang and S. Wang, Isolation of a novel lutein–protein complex from Chlorella vulgaris and its functional properties, Food Funct., 2015, 6, 1893–1899 RSC.
|
This journal is © The Royal Society of Chemistry 2020 |
Click here to see how this site uses Cookies. View our privacy policy here.