DOI:
10.1039/C9FO01590F
(Review Article)
Food Funct., 2020,
11, 66-82
Emerging strategies for the activity assay and inhibitor screening of alpha-glucosidase
Received
20th July 2019
, Accepted 17th November 2019
First published on 19th November 2019
Abstract
The high incidence of diabetes mellitus has caused widespread concern around the world, and has quickly become one of the most prevalent and costly chronic diseases. Alpha-glucosidase has been considered as the main target enzyme in the prevention and treatment of type 2 diabetes, and the inhibition of alpha-glucosidase activity can control postprandial blood glucose levels of diabetics and keep blood glucose levels normal. However, most of the current antidiabetic drugs in the clinic have many side effects. Recently, the screening of alpha-glucosidase inhibitors, from natural products, with excellent activity and less side effects has been successfully achieved, which also proposes more requirements and challenges for the approaches of alpha-glucosidase inhibitor (AGI) screening because of the trace level of active compounds in natural products, large-scale sample screening, and complex matrix of natural products. In this review, we comprehensively summarized the available strategies for the assay of alpha-glucosidase activity and AGI screening for the first time, including classic strategies such as high throughput screening methods (e.g. capillary electrophoresis strategies, HPLC/LC-MS strategies), emerging sensing strategies (e.g. colorimetric sensing, fluorescence sensing, electrochemical sensing, and surface plasmon resonance sensing) and other strategies. Finally, future opportunities and challenges in future research are tentatively proposed.
1. Introduction
Diabetes mellitus as a chronic, multifactorial metabolic disease has caused widespread concern around the world due to its severity and high incidence.1–3 The hyperglycemia of diabetics over a prolonged period can cause a number of complications such as foot ulcers, diabetic retinopathy, nephropathy, stroke, cardiovascular diseases, neuropathy, and so on.4,5 In the past few decades, the prevalence of diabetes has risen rapidly and there are more than 415 million people estimated with diabetes mellitus around the world. Surprisingly, the number of people with diabetes mellitus is expected to reach 642 million in 2040.6 In addition, there is a growing incidence of diabetes mellitus at a younger age including some obese children even before puberty.7,8 Type 2 diabetes (T2D) as the main type of diabetes (which accounts for more than 90% of all diabetes mellitus incidences) is caused via insulin resistance/insulin deficiency.9–12
Alpha (α)-glucosidase (GAA) as an important carbohydrate hydrolysis enzyme plays a key role in transforming the oligosaccharides and disaccharides to glucose, and the produced monosaccharides can be absorbed by the small intestine, leading to an increase in blood glucose levels.13–17 Therefore, GAA has been recognized as the main target enzyme in the prevention and treatment of T2D,18,19 and the alpha-glucosidase inhibitors (AGIs) can play important roles in controlling the postprandial blood glucose levels of diabetics and keeping the blood glucose levels normal by delaying the digestion of carbohydrates and diminishing the absorption of monosaccharides.20–23 Thus, it is of great importance to screen AGIs for the prevention and treatment of T2D. Furthermore, AGIs are also considered as potential antiviral drugs against hepatitis B virus (HBV) and human immunodeficiency virus (HIV) infection.24,25 Up to now, unremitting efforts have been devoted to screening AGIs from organic compounds and natural products,26,27 and there are many well-known AGIs produced by organic compounds for the treatment of diabetes, such as acarbose, miglitol, nojirimycin, voglibose, and so on.14,19,20,26,28 However, there are some obvious side effects (e.g. abdomen pain, abdominal bloating, increased flatulence, diarrhea, and cramping).14,29 Therefore, screening the natural active compounds with low side effects from natural products as AGIs has attracted more and more attention. In addition, the American Association of Clinical Endocrinologists (AACE) and International Diabetes Federation (IDF) have considered natural AGIs as a first line therapy.30 It is of great importance to develop simple, rapid, easy-to-use strategies to screen AGIs from natural products.
So far, there are plenty of strategies developed for the GAA activity assays and screening of AGIs (Fig. 1), such as classic strategy derived high throughput screening (HTS) methods (e.g. capillary electrophoresis (CE), high performance liquid chromatography-mass spectrometry(LC-MS)), new colorimetric sensing, fluorescence sensing, electrochemical sensing and so on. Herein, according to the detection principles, we categorized the strategies for the assay of GAA activity and AGI screening into three classes in general, including classic strategies, emerging sensing strategies, and other strategies. Moreover, the representative strategies for the assay of GAA activity and AGI screening are compared.
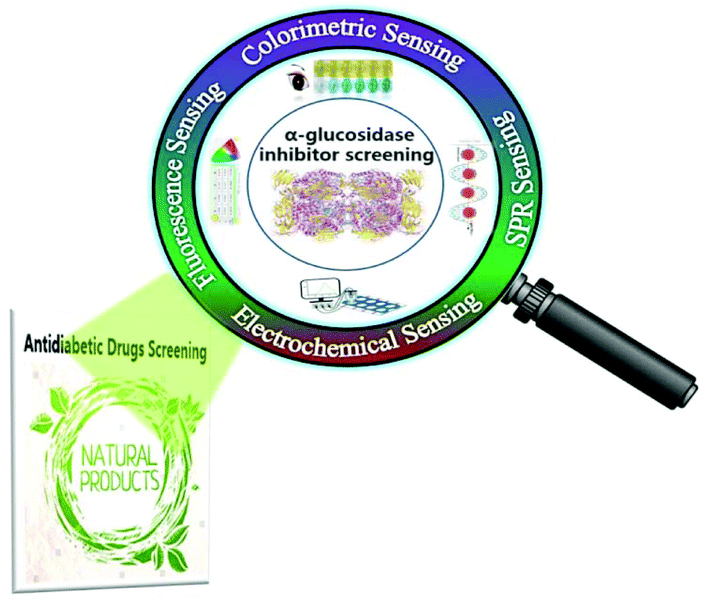 |
| Fig. 1 Schematic illustration of emerging strategies for the activity assay and inhibitor screening of alpha-glucosidase. | |
2. The strategies for the activity assay and inhibitor screening of α-glucosidase
2.1 Classic strategies
The colorimetric assay as a classic strategy is widely used for GAA activity detection and AGI screening because of its practicability and simplicity. Through this colorimetric assay strategy (Fig. 2a), the GAA activity and the effect of AGIs can be determined by using the GAA hydrolysis of p-nitrophenyl-α-D-glucopyranoside (pNPG) to generate p-nitrophenol (pNP) and then measuring ultraviolet absorption at 400 nm of pNP.6,31 On this basis, many great HTS methods (Table 1) as important strategies for AGI screening from complex natural product extracts have been established in the past few decades, such as capillary electrophoresis (CE), high performance liquid chromatography (HPLC) with different detectors (e.g. diode array detectors (DADs) and MS) and so on.32 Among these methods, the CE-based enzyme detection methods have been extensively studied and widely applied to the investigation of the inhibition kinetics, enzyme kinetics, and enzyme inhibitor screening due to their merits of minimal sample consumption, high efficiency separation, short analysis time, and flexible connection with different detectors.33 In this assay, the used enzymes generally exist with two forms (free and immobilized, respectively). Compared to the enzymes with a free form, the immobilized enzymes show higher operational and storage stability and better reusability. Moreover, the immobilized enzymes have also been confirmed to be endowed with tolerance to high temperatures and extreme pH.
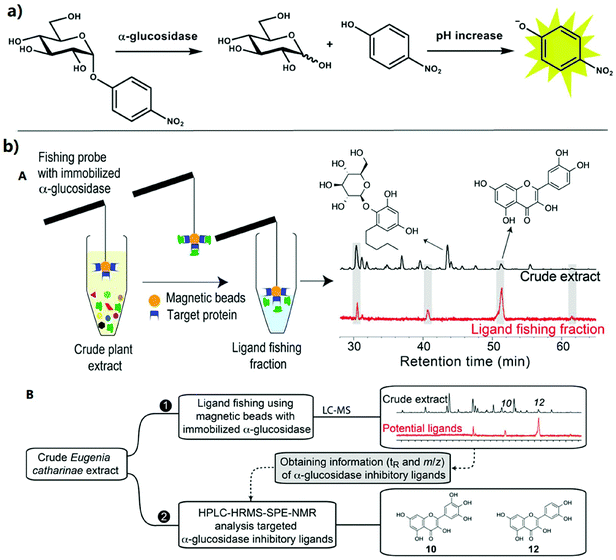 |
| Fig. 2 Classic strategies for the activity assay and inhibitor screening of alpha-glucosidase. (a) The reaction was performed to measure GAA activity within this method. pNPG is hydrolyzed to release glucose and pNP. Then, a p-nitrophenolate anion having a yellow color was generated.31 (b) (A) Schematic diagram of ligand fishing with the immobilized enzyme for GAA inhibition profiling. (B) Schematic workflow for ligand fishing with N-terminus-coupled GAA magnetic beads combined with HPLC-HRMS-SPE-NMR analysis of E. catharinae extract.44 | |
Table 1 Reported classic strategy derived HTS methods for AGI screening
Classic strategy derived HTS methods |
Detection methods |
Equipment |
Characteristics |
Ref. |
CE strategies |
|
Short analysis time, |
27, 90 and 91
|
high separation efficiency, |
minimal sample consumption, and |
flexible connection with different detectors |
LC-MS strategies |
|
High speed, |
38–41 and 92–98
|
excellent sensitivity, |
excellent separation capability, and |
low tested compounds consumption |
HPLC-HRMS-SPE-NMR strategies |
|
Excellent sensitivity, |
42–45 and 99–101
|
excellent separation capability, |
detects all organic chemical classes, and |
reliable compound identification |
In recent years, the AGIs from natural products have gained continued importance for the prevention and treatment of T2D. The HPLC/LC-MS strategies have been widely used for screening the AGIs from natural products due to their merits of high efficiency separation, rapid and sensitive determination, and low tested compound consumption.32 By combining HPLC with diverse detectors, plenty of HPLC strategies have been developed for AGI screening, such as high-performance liquid chromatography-solid phase extraction-nuclear magnetic resonance spectroscopy (HPLC-SPE-NMR), high-performance liquid chromatography-diode array detectors (HPLC-DADs), high-performance liquid chromatography-solid phase extraction-tube-transfer nuclear magnetic resonance spectroscopy (HPLC-SPE-ttNMR), and so on.34 For example, based on the hyphenation of SPE, HPLC, and ttNMR, Schmidt et al. developed an analytical platform for a high-resolution AGI screening. The developed platform could rapidly screen the GAA inhibition analytes from complex matrices, and then achieve the structural identification of AGIs. The study showed that quercetin as the most potent inhibitor possessed a half-maximal inhibitory concentration (IC50) of 8.1 ± 0.4 μM.35 Although these strategies could be used to effectively screen active compounds, their applications to active compound screening in complex matrices were limited due to the compatibility problems of the baseline drift caused by the inactivation of biological reagents in the organic solution and the gradient elution during HPLC separation.36 To solve this problem, Guo et al. successfully developed a novel on-line HPLC-Fourier-transform mass spectrometry (HPLC-FTMS) strategy for rapidly screening AGIs from green tea extracts.36 With this method, the compounds could be separated through HPLC and could generate the on-line reaction with GAA, and the substrate solutions could be delivered through the flow injection. UV detection could be used for the collection of enzyme inhibition signals. Four components from green tea with GAA inhibition action were further identified by HPLC-FTMS. The proposed on-line strategy could simultaneously detect the enzyme inhibition profiles and chromatographic fingerprints of the green tea with different brands.36
Meanwhile, HPLC-MS strategies as reliable and powerful analytical approaches have been extensively studied for rapid identification and screening of AGIs, such as HPLC-HRMS, HPLC-DAD-MS, HPLC-Fourier transform-ion cyclotron resonance (FT-ICR) MS, UHPLC-quadruple time-of-flight-(QTOF)MS, HPLC-electrospray ionization (ESI)-TOF/MS, HPLC-HRMS-SPE-NMR, HPLC-PDA-HRMS-SPE-NMR, and so on.37,38 For instance, Zaharudin et al. used the solution of acetone extracts from seaweed, water, and methanol to achieve the GAA inhibition assay hyphenated with HPLC-HRMS. The results demonstrated that the acetone extracts from Undaria pinnatifida possessed the strongest inhibition effect for GAA activity, with an IC50 of 0.08 ± 0.002 mg mL−1.39 In another study, based on HPLC with the FT-ICR MS technique, Xiao et al. investigated the polyphenolics in four berries and successfully identified the anthocyanins. The results showed the inhibition activity of the four berries for GAA with an order cranberry < blueberry < bilberry < mulberry.38 To quickly identify and screen the specific AGI components from guava leaf tea (GLT), Wang et al. developed a novel strategy by combining bioaffinity ultrafiltration with HPLC-ESI-TOF/MS (BAUF-HPLC-ESI-TOF/MS). The proposed approach could be applied to quickly identify and recognize potential AGIs from GLT extracts.40 Recently, by combining ultra-filtration with UPLC-QTOF-MS/MS, Zhang et al. developed a new strategy for screening AGIs and measuring the content of polyphenols and AGIs for the first time. On this basis, the AGIs screened in vitro with IC50 values were approximately 167–433 fold that of acarbose.41 In addition, the combination of the high-resolution bioassay and HPLC-HRMS-SPE-NMR has been proven to be a good choice for efficiently and rapidly screening AGIs from natural products.42,43 For example, Staerk et al. combined the bioanalytical platform with magnetic ligand fishing and HPLC-HRMS-SPE-NMR for the inhibition profiling of GAA and the structural identification of AGIs from crude plant extracts, respectively (Fig. 2b). In this platform, magnetic beads with N-terminus-coupled GAA were successfully prepared for catalysis. In addition, the proposed platform could be used as a powerful strategy for AGI screening from crude plant extracts.44 In a very recent study, their group combined the high-resolution GAA inhibition profiling with the HPLC-photodiode array (PDA)-HRMS-SPE-NMR to identify AGIs from Machilus litseifolia. This platform realized the high-throughput screening and identification of 13 dicoumaroylated flavonol rhamnosides.45
Although these HTS strategies show the advantages of highly efficient separation, and rapid and sensitive determination, there are some disadvantages limiting their extensive applications, such as the requirement of expensive equipment and well-trained operators. Moreover, these HTS strategies were still based on the conventional colorimetric strategy, which limits the further promotion of HTS performance to a certain degree. For example, the accuracy of detection may be affected due to the overlap of absorption between pNP and AGIs. Therefore, there is a great need to develop a fast-response, sensitive, and practical strategy as an alternative to classic methods.18
2.2 Emerging sensing strategies
To improve the sensitivity, facility, reproducibility, and selectivity of AGI screening methods, a series of novel sensing strategies have been developed to sensitively detect GAA activity and rapidly screen AGIs, such as new colorimetric sensing strategies, fluorescence sensing strategies, electrochemical sensing strategies, surface plasmon resonance (SPR) sensing strategies, etc. (Table 2).
Table 2 Reported emerging sensing strategies for the GAA activity assay and AGI screening
Emerging sensing strategies |
Detection methods |
Signal output units |
Linear range (U mL−1) of GAA activity |
LOD (U mL−1) of GAA activity |
LOD (μM) of AGIs |
Ref. |
—, not reported. |
Newly emerged colorimetric sensing |
pAPG-functionalized AuNPs |
0.05–1.1 |
0.004 |
— |
48
|
Unmodified AuNPs |
0.0025–0.05 |
0.001 |
— |
49
|
AuNRs |
0.0025–0.045 |
0.0005 |
— |
50
|
Ag3PO4 nanozyme |
— |
— |
0.01 |
52
|
GAA@GOx@Cu-MOF |
— |
— |
0.007 |
53
|
Fluorescence sensing |
N-doped CDs |
— |
— |
0.01 |
60
|
N-doped CDs |
— |
0.01 |
0.01 |
55
|
N,B-CDs |
— |
0.003 |
0.01 |
64
|
Cationic conjugated polymers |
— |
— |
— |
68
|
β-CD-coated QDs |
— |
— |
— |
69
|
β-CD-CQDs |
— |
0.0006 |
— |
54
|
MnO2 nanosheet, Ag NCs |
0.2–8 |
0.03 |
0.01 |
66
|
PBA-CQD nanoprobe |
— |
0.00033 |
— |
67
|
Electrochemical sensing |
MNPs/pAPG |
— |
— |
— |
73
|
Apt 1/pAPG/GSH/AuNPs |
— |
0.005 |
— |
71
|
CNH modified electrode |
0.001–0.016 |
0.00056 |
— |
72
|
SPR sensing |
Ag nanoprism |
— |
— |
0.005 |
6
|
2.2.1 Newly emerged colorimetric sensing strategies.
Colorimetric sensing has gained extensive attention owing to its facile operation, easy read-out and fast visual detection via the naked eye or low-cost portable devices, which can be used to detect some analytes based on the color variation during a chemical reaction.46,47 To improve the sensitivity and selectivity of the GAA activity assay and AGI screening, some novel colorimetric sensing strategies (e.g. gold nanoparticle (AuNP)-based colorimetric sensing assays) have been successfully developed. Owing to the unique optical properties and the ability for specific surface chemistry design of AuNPs, the AuNP-based colorimetric sensing assays have been extensively applied to the detection of various targets.48,49 On this basis, Chen et al. successfully developed a new colorimetric sensor for sensitively detecting GAA activity and screening AGIs via unmodified AuNPs (Fig. 3a). The proposed sensing method utilized the triple-catalytic reaction caused by GAA. In the presence of GAA, the cysteine in the system was oxidized to cystine and the aggregation of AuNPs was hindered. Nevertheless, the cysteine could induce the aggregation of AuNPs with the addition of AGIs. The recovered aggregation of AuNPs could lead to an increasing absorbance at 600 nm and the color of the solution changed from wine red to violet. The newly developed strategy could be used to sensitively detect GAA activity with a good linear relationship ranging from 0.0025 to 0.05 U mL−1, and with a limit of detection (LOD) of 0.001 U mL−1.48 Although AuNP-based colorimetric sensing assays showed the advantages of technical simplicity, high selectivity and sensitivity, the self-aggregation of AuNPs may lead to a high background and false positive results in complex specimen solutions, which hindered their wide applications.50 To overcome these defects, gold nanorod (AuNR)-based etching approaches have been successfully developed, and exhibited the merits of high stability, larger extinction coefficient, no need for modification/marker, possessing tunable plasma bands, and eliminating the possibility of false positive results caused by self-aggregation.50 For example, Cheng et al. developed an ultrasensitive colorimetric sensing system for the detection of GAA activity and the evaluation of AGIs through the iodine-mediated etching of AuNRs. In this strategy, 2-O-α-D-glucopyranosyl-L-ascorbic acid (AA-2G) as the substrate of GAA was hydrolyzed to produce ascorbic acid (AA). Then, KIO3 was in situ reduced to generate I2via the hydrolysate AA, and subsequently the as-obtained I2 etched the AuNRs. The AuNRs showed a blue-shift of the localized surface plasmon resonance (LSPR) absorption, which could be clearly observed through UV-vis spectroscopy.51 The developed method could sensitively detect GAA with a good linear relationship ranging from 2.5 to 45 U L−1 with a low GAA LOD of 0.5 U L−1. Moreover, this method was used to selectively and efficiently screen AGIs from natural products. The result demonstrated that the IC50 value was estimated to be 1.031 and 1.258 mg mL−1 for Ningxia wolfberry and black goji berries, respectively. In addition, the AuNR sensor system exhibited the virtues of simplicity, low cost, high selectivity, and excellent sensitivity.50 In recent years, the nanozymes developed for signal amplification have attracted extensive interest from multiple fields. In view of the merits of nanozymes (including robustness under extreme conditions, design flexibility, ease of storage and treatment, facile construction and purification, low-cost, and easy miniaturization), our group for the first time developed a novel colorimetric sensing approach for ultrasensitive detection of GAA activity by using the AA to trigger the Ag3PO4 nanozyme (Fig. 3b). In this method, the Ag3PO4 NPs exhibited an excellent peroxidase-like activity and could catalyze the oxidation of the substrate 3,3,5,5-tetramethylbenzidine (TMB) to produce a blue color in the presence of visible light. The proposed strategy could sensitively detect AGIs with an ultra-low LOD of 10 nM, and a LOD of 1 μM for a naked-eye assay. Moreover, the developed sensing assay could open a novel avenue for efficient and stable cascade signal amplification through nanozymes.52 In a very recent study, Zhong et al. successfully developed a novel bifunctional hybrid enzyme-catalytic metal organic framework (MOF) reactor (GAA@GOx@Cu-MOF) mediated colorimetric sensing strategy for the screening of AGIs. In this reactor, the GAA@GOx@Cu-MOFs with a flower-shaped globular structure were successfully fabricated based on the self-assembling of GAA, GOx, 4,4′-bipyridine, and Cu2+. The as-obtained GAA@GOx@Cu-MOFs showed outstanding advantages of high sensitivity, great selectivity, and high catalytic stability, and possessed approximately 4.58 times catalytic activity compared to the free enzymes. Herein, maltose served as the initial substrate of the reactor, and the absorbance of oxTMB was employed as the signal output. Maltose could be hydrolyzed by GAA@GOx@Cu-MOFs to produce H2O2. Then Cu-MOFs with enzyme-like catalytic activity could oxidize TMB to oxTMB in the presence of the produced H2O2. When the AGIs were introduced, the generation of H2O2 was hindered, leading to the inhibition of TMB oxidation. On this basis, the proposed method could achieve a highly sensitive GAA inhibitor screening with a low LOD of 7.05 nM for acarbose.53 Although the emerged colorimetric sensing strategies show some improved advantages, their accuracy and sensitivity may be easily influenced by the interference generated from the sample background color.
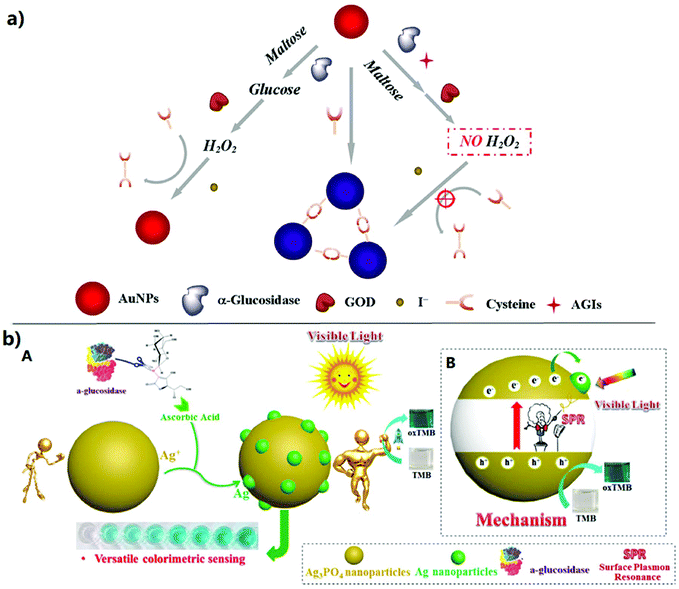 |
| Fig. 3 Colorimetric sensing strategies for the activity assay and inhibitor screening of alpha-glucosidase. (a) Schematic illustration of the proposed method for the GAA activity assay and AGI screening.49 (b) Schematic illustration of the colorimetric sensing strategy based on the signal amplification by an ascorbic acid adjusted Ag3PO4 nano-peroxidase and application to versatile bio-sensing (i.e. ascorbic acid, α-glucosidase activity and inhibitor screening).52 (A) The colorimetric sensing strategy based on SPR for the activity assay of alpha-glucosidase. (B) The photocatalytic mechanism of Ag3PO4. | |
2.2.2 Fluorescence sensing strategies.
Compared to the traditional colorimetric assays, AuNP-based colorimetric sensing assays have made much improvement in terms of sensitivity and selectivity. However, the colorimetric sensing strategies are still inferior to the fluorescence sensing methods in terms of anti-interference and sensitivity.54 Thus, in recent years, plenty of fluorescence sensing strategies have also been developed for the GAA activity assay and AGI screening, such as fluorescence resonance energy transfer (FRET)-based fluorescence sensing, inner filter effect (IFE)-based fluorescence sensing, electron transfer (ET)-based fluorescence sensing and so on.55
2.2.2.1 FRET-based fluorescent sensing.
Fluorescence sensing is performed mainly through target analyte-mediated fluorescence enhancement (“turn-on”) or fluorescence quenching (“turn-off”). Among these fluorescence sensing strategies, FRET-based fluorescence sensing is usually performed using a quencher and a fluorophore probe to generate an acceptor–donor FRET pair.56 Due to the overlap between the fluorescence emission band of the donor and the absorption band of the quencher, the FRET will lead to fluorescence quenching.57 Based on this principle, FRET-based fluorescence sensing has been developed in multiple fields, such as biological analysis,58 environmental monitoring, food safety,59 and so on.60 Therefore, plenty of FRET-based fluorescent sensors have been developed for the study and determination of biomolecules. Among these sensors, fluorescent proteins and organic dyes generally serve as acceptors or donors, respectively. Nevertheless, because of their defects of low stability, fixed emission/absorption spectra, photobleaching, and short observation time, these fluorescent sensors need to overcome some huge obstacles. To solve these difficulties, quantum dot (QD) nanoparticles were developed to serve as excellent resonant energy donors owing to their advantages of excellent photostability, broad excitation, high quantum yields, narrow emission, and large Stokes shifts.61 For example, our group successfully developed a FRET-based “turn-on” nanofluorescent sensor (Fig. 4a) for the GAA activity assay and AGI screening utilizing nitrogen-doped carbon dot-hexagonal cobalt oxyhydroxide nanoflakes (N-doped CD-CoOOH) architecture.60 In this fluorescent sensor, carbon dots (CDs) as the signal output of fluorescence could be prepared through a one-pot synthesis strategy, and the CDs could be efficiently turned off by CoOOH nanosheets through the FRET. CoOOH nanosheets in combination with CDs were used for constructing a FRET-based fluorescent sensor platform. L-Ascorbic acid-2-O-α-D-glucopyranosyl (AAG) as the substrate of GAA was innovatively introduced. When AAG was hydrolyzed via GAA to produce AA, the produced AA could quickly reduce the CoOOH nanosheets to generate Co2+. The destruction of CoOOH nanosheets lead to the stop of FRET, and thus the fluorescence of CDs was recovered. The developed fluorescent sensor platform could be used to sensitively detect the AA with a LOD of 5 nM, which ensured a sensitive detection of GAA activity. By using acarbose as the AGI model, its inhibition rate was studied and was proportional to the logarithm of the concentration ranging from 10−9 to 10−3 M, and with a correlation coefficient of R2 = 0.996. Moreover, acarbose could be sensitively detected with an ultralow LOD of approximately 10−9 M. In addition, the sensor platform exhibited excellent sensitivity and applicability and was used to evaluate the inhibiting ability of seven active compounds from natural products.60 Although the FRET-based fluorescence sensing assay exhibits satisfactory selectivity and sensitivity, some drawbacks still exist, such as the complexity of constructing a FRET-based sensor platform and poor solubility during the enzymatic reaction.62
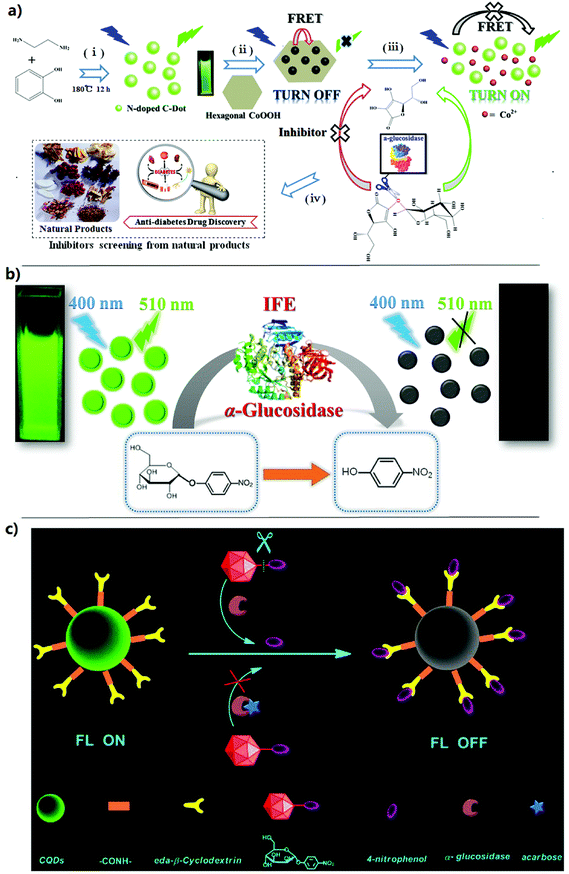 |
| Fig. 4 Fluorescence sensing strategies for the activity assay and inhibitor screening of alpha-glucosidase. (a) Schematic illustration of the preparation of N-doped carbon dots (i) and FRET-based sensing platform (ii), hydrolysis of AAG by α-glucosidase to stop FRET and yields of fluorescent signal readouts (iii) and application of the proposed sensing platform to screen the α-glucosidase inhibitor from natural products (iv).60 (b) Schematic illustration of N, B-CDs as an ultrasensitive fluorescent probe for α-glucosidase activity and its inhibitor in water and living cells based on the IFE.64 (c) Schematic illustration of the strategy for the detection of GAA activity and screening of inhibitors based on supramolecular self-assembly between the β-CD-CQD nanoprobe and 4-nitrophenol.54 | |
2.2.2.2 IFE-based fluorescent sensing.
Due to its disadvantages such as time consuming, poor solubility during the enzymatic reaction and the complexity of construction, the practical applications of FRET-based fluorescence sensing may be limited. Fortunately, the IFE as an alternative principle for the fluorescence sensing assay has been extensively studied because of its enhancement of selectivity and sensitivity.55 When the absorption spectrum of interference absorbers in the sensing system overlaps with the fluorescence emission or excitation spectra of fluorophores, the IFE occurs. Therefore, the absorption response can be converted exponentially into the variation of fluorescence intensity, which will make fluorescence sensing assays more sensitive and facile.63 Recently, IFE-based fluorescence sensing strategies have been successfully developed for the enzyme activity assay and their inhibitor screening.55,62,64,65 For example, Kong et al. proposed a novel, sensitive and facile fluorescence sensing approach based on the GAA activated IFE on nitrogen-doped CDs for the detection of GAA activity and AGI screening for the first time. The N-doped CDs were synthesized through a one-step hydrothermal synthesis strategy, which could be excellently used as a fluorescence signal output due to their good green emission and the fluorescence quantum yield of 30%. With the hydrolysis of the substrate 4-nitrophenyl-α-D-glucopyranoside (NGP) by GAA, pNP as a powerful absorber was released to quench the fluorescence of N-doped CDs owing to its great molar absorptivity complementarily overlapping with the excitation spectra of the N-doped CDs. By transforming the absorption signals into fluorescence signals, a fluorescence sensing approach could be achieved for the detection of GAA activity. And the proposed method could effectively avoid the complex surface modification of the N-doped CDs or the building of fluorescence nanoprobes. Moreover, the developed IFE-based fluorescence sensing strategy showed a low LOD of 0.01 U mL−1 and could be used for inhibitor screening with excellent sensitivity and applicability. Furthermore, acarbose as a typical AGI had been studied with a low LOD of 10−8 M. In addition, the proposed strategy exhibited plenty of advantages such as excellent selectivity, simplicity, high sensitivity, lost cost, and good reproducibility, which also provided novel insight into the applications of N-doped CDs to construct sensitive and facile biosensors.53 Similarly, Huang et al. recently developed a simple, excellently selective, and highly sensitive IFE-based fluorescence strategy (Fig. 4b) for the detection of GAA activity and AGI screening in living cells and water. In this method, CDs doped with boron and nitrogen (N, B-CDs) were synthesized with a fluorescence quantum yield of 47% as a good fluorescence signal output. With the addition of 4-nitrophenylglucoside, the fluorescence of N, B-CDs was turned off by the yellow pNP produced from the hydrolysis of 4-nitrophenylglucoside because of an IFE. The developed approach was employed for the detection of GAA activity with a low LOD of 3 mU mL−1. Moreover, acarbose as a typical AGI could be detected at a low concentration of 10 nM. In addition, the IFE-based fluorescence sensing method may be used for the activity assay of other enzymes.63 In a recent study, Shi et al. successfully developed a novel MnO2 nanosheet (NS)-based ratiometric fluorescent sensor based on the IFE for the GAA activity assay and its inhibitor screening. MnO2 NS with an excellent oxidase-mimicking activity could oxidize OPD to produce 2,3-diaminophenazine (DAP) which showed strong fluorescence emission at 575 nm. However, the fluorescence of Ag nanoclusters (NCs) at 450 nm could be quenched by the produced DAP because of the IFE. AAG served as a GAA substrate, which could be hydrolyzed to generate ascorbic acid (AA). MnO2 NS was reduced by AA to Mn2+, losing its oxidase-like activity. Therefore, DAP would not be produced, and the IFE was hindered by the fluorescence weakening of DAP and the fluorescence enhancement of AgNCs. Based on the fluorescence intensity ratio of DAP to AgNCs, a new fluorescent sensor was constructed for the GAA activity assay and its inhibitor screening. The developed sensor could be used to detect GAA activity ranging from 0.2 to 8 U mL−1 with a LOD of 0.03 U mL−1. Moreover, acarbose as a typical AGI could be detected with a low LOD of 10−8 M.66 The IFE-based fluorescence sensing methods for the GAA activity assay and AGI screening show enhanced selectivity and sensitivity, but the components in the screened samples may interfere with this sensing method, such as the absorption spectrum of potential compounds in the samples overlap with the fluorescence emission or excitation spectra of fluorophores, affecting the accuracy of the analytical results.55,62
2.2.2.3 Other fluorescence sensing strategies.
Based on previous literature reports, there are some other fluorescence sensing strategies reported for the detection of GAA activity and AGI screening.54,67 For example, Cao et al. developed a fluorescent biosensor to sensitively screen AGIs using the fluorescence signal amplification of cationic conjugated polymers. In the presence of GAA and NGP, the fluorescence of cationic polyfluorenylene phenylene (PFP) would be quenched. Upon the addition of AGIs, the fluorescence would be recovered. On this basis, a fluorescence sensing approach was successfully developed for AGI screening. Moreover, this method exhibited an enhanced sensitivity and provided a novel strategy for the effective detection of enzyme activities and the screening of other enzyme inhibitors.68 Recently, Liu et al. developed a simple and effective fluorescence sensing strategy for the detection of GAA activity and AGI screening by utilizing β-cyclodextrin-coated quantum dots (β-CD-coated QDs). The fluorescence signal of β-CD-coated QDs could be quenched by pNP (which was a hydrolysis product of the GAA reaction) because of an ET process, which could cause the fluorescence turn off. With the addition of AGIs, the fluorescence signal of the sensing system turned on. Owing to the excellent performances of QDs, the proposed approach showed simplicity, high speed, and high sensitivity for AGI screening. The feasibility of the proposed method could be evaluated using two AGIs (acarbose and 2,4,6-tribromophenol), and the IC50 values of the two AGIs were 0.55 mM and 24 μM, respectively.69 Similarly, Tang et al. also established a fluorescent biosensor (Fig. 4c) for the sensitive detection of GAA activity and AGI screening based on a CQD nanoprobe via supramolecular self-assembly. In this biosensor, the β-CD-coated QD nanoprobe was prepared by β-CD covalently bonding to the surface of the CQDs. The self-assembly between the β-CD-coated QD nanoprobe and pNP via host–guest recognition was driven when pNP was present. The supramolecular assembly showed non-fluorescent properties, which lead to a strong fluorescence quenching of the β-CD-coated QDs. The NGP as the substrate of GAA could be hydrolyzed to pNP in the presence of GAA, and then the fluorescence of the β-CD-coated QD nanoprobe was quenched. The linear correlation between the quenched fluorescence of the β-CD-coated QDs and the GAA level could be applied to construct a quantitative assay of GAA activity. The as-established method exhibited excellent selectivity and sensitivity and possessed a low LOD of 0.6 U L−1. In addition, the proposed assay was assessed utilizing acarbose as an example, which showed that this method could be used for effective AGI screening.54 In another study, Ao et al. rationally designed a new reversible fluorescence nanoswitch based on dynamic covalent B–O bonds for the GAA activity assay, which could be switched with the variation of the pH value. The fluorescence nanoswitch depends on the fact that pNP could react with the phenyl boronic acid functionalized carbon quantum dot (PBA-CQD) probe to produce a non-fluorescent conjugate through B–O bonds, and the making and breaking of the dynamic B–O bonds could be controlled via the change of pH. The reaction between the boronic acid and pNP was first identified and could lead to the fluorescence turn off, which could be applied to develop a general approach for the determination of enzyme activity using the substrates that could produce pNP via enzymatic reactions. The developed strategy exhibited high sensitivity for GAA activity with a low LOD of 0.33 U L−1.67
2.2.3 Electrochemical sensing strategies.
Electrochemical sensing strategies are mainly based on the variation of output-electrical signals produced by the chemical reactions between the target analytes and the electrode-immobilized recognition elements. The generation of electrical signals is related to the concentrations of target analytes, which has been widely used for quantitative assays of target analytes.46,70 In recent years, electrochemical sensing has been widely applied in analysis owing to its low cost, simplicity, high sensitivity, good reproducibility, and fast response. Furthermore, electrochemical instrumentation can be easily used for the production of sensors.18 Compared with the traditional colorimetric methods, the electrochemical sensing strategies are proven to be simple and cost-effective. Therefore, the electrochemical sensing strategies have been developed for the detection of GAA activity and AGI screening.71,72 For example, Zhang et al. successfully established an electrochemical sensing strategy (Fig. 5a) for the GAA activity assay and AGI screening via a one-step displacement reaction. Compared with dopamine (DA)/AgNPs, a stronger binding of magnetic nanoparticles/4-aminophenyl-α-D-glucopyranoside to the pyrene boric acid immobilized on the surface of the graphite electrode (GE) could well achieve the one-step displacement reaction. The membrane bound and isolated enzyme activity could be well assessed through this developed strategy because GAA could specifically catalyze the magnetic nanoparticles/4-aminophenyl-α-D-glucopyranoside (MNPs/pAPG) into the MNPs/pAP which had no binding ability with the pyrene boric acid (PBA). At the same time, signal amplification could be completed through DA immobilized on the outer layer of AgNPs. Moreover, the accuracy of this approach could be enhanced via magnetic separation.73 Recently, Li et al. prepared a reusable electrochemical biosensor for the detection of GAA activity and AGI screening. In this sensor, the aptamer of ATP could be split as split aptamer 1 (Apt 1) and split aptamer 2 (Apt 2), and the AuNPs modified by Apt 1 and pAPG were linked to Apt 2. Thus, the functionalized AuNPs could be immobilized on the surface of the gold electrode and could achieve a salt-induced regeneration. The glycosyl of pAPG was cut off in the presence of GAA, and a strong current signal was produced through the electroactive phenolic hydroxyls. Moreover, the prepared biosensor could be easily recovered when it was incubated in water with a subsequent dissociation of Apt 1 and pAPG modified AuNPs. Based on the enzyme-activated electrochemical signal generation and recovery, the biosensor for the assay of GAA activity and AGI screening was successfully developed. This biosensor showed good sensitivity for the assay of GAA activity with a LOD of 0.005 U mL−1.71 In a very recent study, Liu et al. developed a new, facile, and sensitive ratiometric electrochemical sensor (Fig. 5b) using a new integrated electrochemical probe which could simultaneously produce a target response signal and a reference signal for the detection of GAA activity and AGI screening. The proposed ratiometric electrochemical sensor combined the advantages of expanding the electroactive surface area and facilitating the electron transfer of electrodes with the modification of carbon nanohorns. Moreover, the newly developed electrochemical sensor could conquer the defects of the traditional single-electric signal electrochemical sensors like the potential interferences from electrochemical systems and substrates, and could improve the accuracy and sensitivity of electrochemical sensors. The ratiometric signal of the electrochemical sensor could verify the assay of GAA activity ranging from 0.001 to 0.016 U mL−1, with a LOD of 0.00056 U mL−1. Meanwhile, mulberry bark and acarbose as AGIs could be applied to evaluate the feasibility of the electrochemical sensor for AGI screening, with the IC50 values of 3.38 mg mL−1 and 0.55 mM, respectively. In addition, the reliability and reproducibility of the proposed electrochemical sensor were greatly improved because the ratios of the target response signal and the reference signal as the output signal could minimize the deviations caused by the different systems and electrodes.72 Although electrochemical sensing has been widely used because of its advantages including low cost, simplicity, high sensitivity, and fast response, it still suffers from low stability, poor repeatability, and the requirement of analytes’ outstanding electroactivity.74
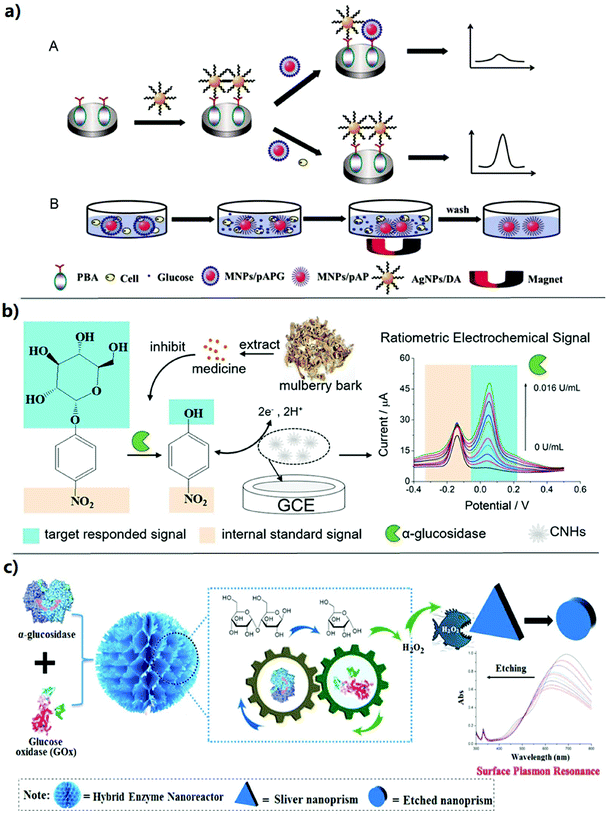 |
| Fig. 5 Electrochemical sensing and surface plasmon resonance sensing strategies for the activity assay and inhibitor screening of alpha-glucosidase. (a) Schematic representation for (A) the detection of GAA activity and (B) magnetic separation for the MNPs/pAP in a cell medium.71 (b) Schematic illustration of the proposed ratiometric sensing approach for the detection of GAA activity and its application in screening of AGIs.72 (c) Schematic illustration of the sensing platform based on the hybrid enzyme nanoreactor with the silver nanoplates as the signal response.6 | |
2.2.4 Surface plasmon resonance sensing strategies.
Surface plasmon resonance (SPR) as a real-time and label-free detection technology has been extensively studied and widely applied in multiple fields. When a metal surface (e.g. Au surface) is hit by a photon of incident light, SPR occurs. When the reflective index of the sensing medium changes (e.g. through biomolecule attachment), SPR can't be generated. Determination of analytes can be achieved through the measurement of the variation in the reflected light using a detector. Furthermore, the surface concentration can be quantified by tracking the resonance angle shifts or monitoring the reflected light intensity.75–77 On this basis, SPR sensing strategies have also recently been developed for the detection of GAA activity and AGI screening.6,52 For example, based on SPR, Kong et al. innovatively developed a novel, facile, and effective robust hybrid enzyme nanoreactor-mediated plasmonic sensing approach (Fig. 5c) for ultrasensitive AGI screening. In this method, to improve the stability of enzymes and the sensitivity of the AGI screening method, GAA and glucose oxidase (GOx) were produced in a multi-enzyme-inorganic nanoreactor with a flower shape via self-assembly for the first time. The prepared hybrid enzyme nanoreactor showed the advantages of robust stability, enhanced catalytic activity, and the character of assembly line. Moreover, the big specific surface area of the enzyme nanoreactor was beneficial to the detection of trace AGIs. Maltose as the substrate of GAA could be hydrolyzed to produce glucose, and then the produced glucose was catalyzed via GOx to produce H2O2. Subsequently, the Ag nanoprism was etched by the released H2O2 to form round nanodiscs, which could lead to a blue shift of the SPR absorption band. The developed strategy had been proved to be highly efficient, ultrasensitive, and robust for AGIs. And the proposed strategy exhibited high stability and excellent applicability when it was used to screen AGIs from natural products. Furthermore, ultrasensitive AGI screening could be achieved with a low LOD of 5 nM for acarbose by using hybrid enzyme nanoreactor-guided SPR. In addition, the proposed sensing approach could also provide novel insight into the inhibitor screening of other enzymes.6 The SPR sensing shows outstanding analytical performances such as real-time and label-free detection, fast response, high sensitivity and reproducibility, but the inherent drawback (the interference of non-specific bindings to output signals) of SPR still needs to be solved.75
2.3 Other strategies
In addition to the above strategies, there are some other methods reported for the assay of GAA activity and AGI screening.78,79 For example, Chen et al. developed a new strategy (namely after flowing through an immobilized receptor system (AFTIR)) for AGI screening from herbal medicines. In this system, the membrane-bound enzyme, GAA, was immobilized onto a chip as a receptor. The SPR and micro-fluid technology were applied to the detection and AGI screening in green tea and Chinese herbal medicines. The developed AFTIR system (GAA-CM5 chip) had been proven to be a highly efficient platform for purification and identification of active components. Owing to the usage of SPR optics principles, the proposed system could detect the active components with minimal sample mass. Moreover, the system could precisely control the flow speed and real-time reaction to exhibit the dissociation and binding of reactants.80 In a study, Shang et al. developed an effective strategy for screening and identifying AGIs from a mulberry leaf extract by using NMR techniques. The result demonstrated that the proposed approach could be used as a powerful tool to screen and identify the AGIs in complex samples.79 In another study, by combining with the affinity surface chemistry and carbohydrate microarray, Yang et al. developed a strategy for the assay of GAA activity by combining matrix-assisted laser desorption/ionization (MALDI)-TOF MS with the affinity surface chemistry and carbohydrate microarray. In this approach, carbohydrate probes were prepared for capturing the enzymatic reaction products, and adducts were loaded on the surface of fluorinated gold to produce an array. The activity of several enzymes could be detected from the ratio of intensities. Compared with the traditional colorimetric techniques, this approach could overcome matrix interference problems from the samples.78
3 Conclusion and prospective
Currently, diabetes mellitus as one of the most serious chronic diseases has caused widespread concern around the world owing to its severity and high incidence. GAA as an important target enzyme in the prevention and treatment of T2D has been extensively studied. Therefore, screening the AGIs for the prevention and treatment of T2D has attracted great attention. Owing to the side effects of organic compounds, screening the AGIs from natural products has become more important. At present, there are plenty of strategies developed for the GAA activity assays and AGI screening, such as the classic strategy derived high throughput screening (HTS) methods (e.g. CE and LC-MS), new colorimetric sensing strategies, fluorescence sensing strategies, electrochemical sensing strategies, SPR sensing strategies, and so on. In this review, we summarized the strategies for the assay of GAA activity and AGI screening for the first time, including classic strategies, emerging sensing strategies, and other strategies. Among these strategies, the HTS strategies have been widely applied in AGI screening from natural products by virtue of the advantages of HPLC/LC-MS technology including highly efficient separation, and rapid and sensitive determination. However, these HTS strategies were still based on the conventional colorimetric strategy, which limits the further promotion of HTS performance to a certain degree. Meanwhile, the detection accuracy may be disturbed due to the overlap of absorption between pNP and AGIs. Most importantly, in the process of screening inhibitors, the free GAA shows the disadvantage of instability under conventional and long-term storage conditions, affecting the sensitivity and accuracy of AGI screening. Recently, GAA and GOx have been prepared to be GAA@GOx@Cu-MOF reactors for AGI screening, and showed the merits of high stability, good selectivity, and excellent sensitivity.53 Similarly, a covalent organic framework (COF) as an emerging porous nanomaterial has also been applied for enzyme immobilization to improve their stability, which may be applied in GAA immobilization for AGI screening.81–83 The GAA immobilized onto the nanomaterials/prepared to be integrated nanozymes (INAzymes) can exhibit stronger enzymatic activity, higher stability, and better reusability.84 Thus, the development of GAA immobilization and nanozyme preparation strategies will achieve a stable and sensitive AGI screening.
To overcome the problems of poor repeatability, selectivity, and limited sensitivity of the conventional colorimetric assay, some emerging sensing strategies have been developed to sensitively detect GAA activity and rapidly screen AGIs. Nevertheless, the emerging sensing strategies are still relatively rare. The relatively single sensing mode (mainly based on colorimetry, fluorescence, and electrochemistry) may limit the development of approaches for the detection of GAA activity and AGI screening in a certain degree. Therefore, new sensing strategies for the detection of GAA activity and AGI screening remain to be further investigated. Surface-enhanced Raman scattering (SERS) as a surface-sensitive technique has been extensively applied in biomedical analysis due to its specificity, sensitivity, and multiplexing capabilities.85,86 Recently, SERS sensing strategies have been used for enzyme activity assays with high sensitivity.87 SERS sensing may also be used to broaden a promising strategy for the detection of GAA activity. Furthermore, electrochemiluminescence (ECL) sensing has been extensively studied and applied in analytical sensing, and shows the advantages of high sensitivity, low background noise, wide detection range, and simplified optical setup.88 Similarly, photoelectrochemical (PEC) sensing has gained increasing attention due to its excellent advantages such as fast response, simple equipment, low background noise, and good sensitivity compared with conventional optical and electrochemical sensing.27,89 Therefore, these emerging techniques such as ECL and PEC sensing also can be potentially applied to develop new sensing strategies for the GAA activity assay and AGI screening. In addition, compared to the classic strategies, most of the emerging sensing strategies don't need expensive instruments, and significantly reduced sample consumption, which shows excellent applicability. However, the stability of these sensing strategies still needs to be further improved. Based on the newly established methods, the development of inexpensive, fast-speed, and portable devices with high specificity and sensitivity is critical for the assay of GAA activity and AGI screening.
The available HTS strategies such as HPLC/LC-MS methods are mostly based on the traditional colorimetric principle, which may limit the sensitivity and selectivity of HTS strategies for the assay of GAA activity and AGI screening. Furthermore, the highly active compounds from natural products are usually found in trace amounts, which are often difficult to effectively screen with these methods. Fortunately, new sensing strategies have been developed with high sensitivity and selectivity. By integration of the advantages of the emerging sensing strategies and the HTS strategies, promising high-throughput strategies with higher sensitivity and stability can be constructed for ultra-sensitive AGI screening from complex natural products. This review hopefully can stimulate interdisciplinary research at the intersection of bio-analytical chemistry and drug screening.
Abbreviations
AGIs | Alpha-glucosidase inhibitors |
AuNPs | Gold nanoparticles |
AuNRs | Gold nanorods |
AA | Ascorbic acid |
Ag NCs | Ag nanoclusters |
AAG |
L-Ascorbic acid-2-O-α-D-glucopyranosyl |
AA-2G | 2-O-α-D-Glucopyranosyl-L-ascorbic acid |
Apt1 | Aptamer1 |
Apt2 | Aptamer2 |
AFTIR | After flowing through an immobilized receptor system |
CE | Capillary electrophoresis |
COF | Covalent organic framework |
CD-CoOOH | Carbon dot-hexagonal cobalt oxyhydroxide nanoflakes |
DA | Dopamine |
ESI | Electrospray ionization |
ECL | Electrochemiluminescence |
ET | Electron transfer |
FT-ICR | Fourier transform-ion cyclotron resonance |
FRET | Fluorescence resonance energy transfer |
GAA | Alpha-glucosidase |
GLT | Guava leaf tea |
GE | Graphite electrode |
GOx | Glucose oxidase |
HBV | Hepatitis B virus |
HIV | Human immunodeficiency virus |
HPLC | High performance liquid chromatography |
HTS | High-throughput screening |
HPLC-BCD | HPLC-biochemical detection |
IDF | International Diabetes Federation |
INAzymes | Integrated nanozymes |
IFE | Inner filter effect |
K
i
| Inhibition constant |
LC-MS | High performance liquid chromatography-mass spectrometry |
LOD | Limit of detection |
LSPR | Localized surface plasmon resonance |
MNPs/pAPG | Magnetic nanoparticles/4-aminophenyl-α-D-glucopyranoside |
MALDI | Matrix-assisted laser desorption/ionization |
MOF | Metal organic framework |
NS | Nanosheet |
NMR | Nuclear magnetic resonance spectroscopy |
N-doped CDs | Nitrogen-doped CDs |
NGP | 4-Nitrophenyl-α-D-glucopyranoside |
N,B-CDs | CDs doped with boron and nitrogen |
pNP |
p-Nitrophenol |
pNPG |
p-Nitrophenyl-α-D-glucopyranoside |
PBA-CQD | Phenyl boronic acid functionalized carbon quantum dot |
PFP | Polyfluorenylene phenylene |
PEC | Photoelectrochemical |
PDA | Photodiode array |
QTOF | Quadruple time-of-flight |
SERS | Surface-enhanced Raman scattering |
SPR | Surface plasmon resonance |
TCMs | Traditional Chinese medicines |
TMB | 3,3,5,5-Tetramethylbenzidine |
ttNMR | Tube-transfer nuclear magnetic resonance spectroscopy |
β-CD-coated QDs | β-Cyclodextrin-coated quantum dots |
Conflicts of interest
There are no conflicts to declare.
Acknowledgements
This work was supported by the Natural Science Foundation of Shandong Province (ZR2017JL012), the National Natural Science Foundation of China (21677085, 31801454 and 21537001), the Project funded by China Postdoctoral Science Foundation (2018M640574), and the Youth Innovation Team of Shaanxi Universities.
References
- American Diabetes Association, Standards of medical care in diabetes—2013, Diabetes Care, 2013, 36, S11 CrossRef.
- K. Ogurtsova, J. da Rocha Fernandes, Y. Huang, U. Linnenkamp, L. Guariguata, N. H. Cho, D. Cavan, J. Shaw and L. Makaroff, IDF Diabetes Atlas: Global estimates for the prevalence of diabetes for 2015 and 2040, Diabetes Res. Clin. Pract., 2017, 128, 40–50 CrossRef CAS PubMed.
- D. G. Hardie, Targeting an energy sensor to treat diabetes, Science, 2017, 357, 455 CrossRef CAS PubMed.
- Diagnosis and Classification of Diabetes Mellitus, Diabetes Care, 2010, 33, S62–S69.
- M. L. Marcovecchio, M. Lucantoni and F. Chiarelli, Role of chronic and acute hyperglycemia in the development of diabetes complications, Diabetes Technol. Ther., 2011, 13, 389–394 CrossRef CAS PubMed.
- W. Kong, D. Wu, N. Hu, N. Li, C. Dai, X. Chen, Y. Suo, G. Li and Y. Wu, Robust hybrid enzyme nanoreactor mediated plasmonic sensing strategy for ultrasensitive screening of anti-diabetic drug, Biosens. Bioelectron., 2018, 99, 653–659 CrossRef CAS.
- NCD Risk Factor Collaboration, Worldwide trends in diabetes since 1980: a pooled analysis of 751 population-based studies with 4.4 million participants, Lancet, 2016, 387, 1513–1530 CrossRef.
- D. A. Fox, N. Islam, J. Sutherland, K. Reimer and S. Amed, Type 1 diabetes incidence and prevalence trends in a cohort of Canadian children and youth, Pediatr. Diabetes, 2018, 19, 501–505 CrossRef.
- M. Zhang, N. Du, L. Wang, X. Wang, Y. Xiao, K. Zhang, Q. Liu and P. Wang, Conjugated fatty acid-rich oil from Gynostrmma pentaphyllum seed can ameliorate lipid and glucose metabolism in type 2 diabetes mellitus mice, Food Funct., 2017, 8, 3696–3706 RSC.
- F. Dang, Y. Jiang, R. Pan, Y. Zhou, S. Wu, R. Wang, K. Zhuang, W. Zhang, T. Li and C. Man, Administration of Lactobacillus paracasei ameliorates type 2 diabetes in mice, Food Funct., 2018, 9, 3630–3639 RSC.
- H. E. Lebovitz, Type 2 diabetes mellitus—current therapies and the emergence of surgical options, Nat. Rev. Endocrinol., 2011, 7, 408 CrossRef CAS.
- H. Rasouli, S. M.-B. Hosseini-Ghazvini, H. Adibi and R. Khodarahmi, Differential α-amylase/α-glucosidase inhibitory activities of plant-derived phenolic compounds: a virtual screening perspective for the treatment of obesity and diabetes, Food Funct., 2017, 8, 1942–1954 RSC.
- J. H. Kim, C. W. Cho, H. Y. Kim, K. T. Kim, G.-S. Choi, H.-H. Kim, I. S. Cho, S. J. Kwon, S.-K. Choi, J.-Y. Yoon, S. Y. Yang, J. S. Kang and Y. H. Kim, α-Glucosidase inhibition by prenylated and lavandulyl compounds from Sophora flavescens roots and in silico analysis, Int. J. Biol. Macromol., 2017, 102, 960–969 CrossRef CAS.
- L. Han, C. Fang, R. Zhu, Q. Peng, D. Li and M. Wang, Inhibitory effect of phloretin on α-glucosidase: Kinetics, interaction mechanism and molecular docking, Int. J. Biol. Macromol., 2017, 95, 520–527 CrossRef CAS.
- R. Tundis, M. R. Loizzo and F. Menichini, Natural Products as α-Amylase and α-Glucosidase Inhibitors and their Hypoglycaemic Potential in the Treatment of Diabetes: An Update, Mini-Rev. Med. Chem., 2010, 10, 315–331 CrossRef CAS.
- B.-H. Lee, D. R. Rose, A. H.-M. Lin, R. Quezada-Calvillo, B. L. Nichols and B. R. Hamaker, Contribution of the Individual Small Intestinal α-Glucosidases to Digestion of Unusual α-Linked Glycemic Disaccharides, J. Agric. Food Chem., 2016, 64, 6487–6494 CrossRef CAS.
- H. Wen, B. Tang, A. J. Stewart, Y. Tao, Y. Shao, Y. Cui, H. Yue, J. Pei, Z. Liu, L. Mei, R. Yu and L. Jiang, Erythritol Attenuates Postprandial Blood Glucose by Inhibiting α-Glucosidase, J. Agric. Food Chem., 2018, 66, 1401–1407 CrossRef CAS.
- M. Mohiuddin, D. Arbain, A. K. M. S. Islam, M. S. Ahmad and M. N. Ahmad, Alpha-Glucosidase Enzyme Biosensor for the Electrochemical Measurement of Antidiabetic Potential of Medicinal Plants, Nanoscale Res. Lett., 2016, 11, 95 CrossRef CAS.
- Y. Yao, X. Cheng and G. Ren, α-Glucosidase inhibitory activity of protein-rich extracts from extruded adzuki bean in diabetic KK-Ay mice, Food Funct., 2014, 5, 966–971 RSC.
- U. Salar, M. Taha, K. M. Khan, N. H. Ismail, S. Imran, S. Perveen, S. Gul and A. Wadood, Syntheses of new 3-thiazolyl coumarin derivatives, in vitro α-glucosidase inhibitory activity, and molecular modeling studies, Eur. J. Med. Chem., 2016, 122, 196–204 CrossRef CAS.
- L. Zhang, Z.-c. Tu, T. Yuan, H. Wang, X. Xie and Z.-f. Fu, Antioxidants and α-glucosidase inhibitors from Ipomoea batatas leaves identified by bioassay-guided approach and structure-activity relationships, Food Chem., 2016, 208, 61–67 CrossRef CAS.
- Y. Jiao, D. Hua, D. Huang, Q. Zhang and C. Yan, Characterization of a new heteropolysaccharide from green guava and its application as an α-glucosidase inhibitor for the treatment of type II diabetes, Food Funct., 2018, 9, 3997–4007 RSC.
- F. Puzzo, P. Colella, M. G. Biferi, D. Bali, N. K. Paulk, P. Vidal, F. Collaud, M. Simon-Sola, S. Charles, R. Hardet, C. Leborgne, A. Meliani, M. Cohen-Tannoudji, S. Astord, B. Gjata, P. Sellier, L. van Wittenberghe, A. Vignaud, F. Boisgerault, M. Barkats, P. Laforet, M. A. Kay, D. D. Koeberl, G. Ronzitti and F. Mingozzi, Rescue of Pompe disease in mice by AAV-mediated liver delivery of secretable acid α-glucosidase, Sci. Transl. Med., 2017, 9, eaam6375 CrossRef.
- Q. Shen, J. Shao, Q. Peng, W. Zhang, L. Ma, A. S. C. Chan and L. Gu, Hydroxycoumarin Derivatives: Novel and Potent α-Glucosidase Inhibitors, J. Med. Chem., 2010, 53, 8252–8259 CrossRef CAS.
- Y. Nakao, T. Maki, S. Matsunaga, R. W. M. van Soest and N. Fusetani, Penasulfate A, a new alpha-glucosidase inhibitor from a marine sponge Penares sp, J. Nat. Prod., 2004, 67, 1346–1350 CrossRef CAS.
- G. Chi, Y. Qi, J. Li, L. Wang and J. Hu, Polyoxomolybdates as α-glucosidase inhibitors: Kinetic and molecular modeling studies, J. Inorg. Biochem., 2019, 193, 173–179 CrossRef CAS.
- A. Zhang, F. Ye, J. Lu and S. Zhao, Screening α-glucosidase inhibitor from natural products by capillary electrophoresis with immobilised enzyme onto polymer monolith modified by gold nanoparticles, Food Chem., 2013, 141, 1854–1859 CrossRef CAS.
- M.-Z. Lin, W.-M. Chai, Y.-L. Zheng, Q. Huang and C. Ou-Yang, Inhibitory kinetics and mechanism of rifampicin on α-glucosidase: Insights from spectroscopic and molecular docking analyses, Int. J. Biol. Macromol., 2019, 122, 1244–1252 CrossRef CAS PubMed.
-
H. M. J. Krans, in Side Eff Drugs Annu, ed. J. K. Aronson, Elsevier, 2003, vol. 26, pp. 461–476 Search PubMed.
- G. Chen and M. Guo, Rapid Screening for alpha-Glucosidase Inhibitors from Gymnema sylvestre by Affinity Ultrafiltration-HPLC-MS, Front. Pharmacol., 2017, 8, 228 CrossRef.
-
M. D. Rugen, M. Rejzek, H. Naested, B. Svensson and R. A. Field, in Plant Chemical Genomics: Methods
and Protocols, ed. F. Fauser and M. Jonikas, Springer New York, New York, NY, 2018, pp. 101–115, DOI:10.1007/978-1-4939-7874-8-9.
- G. Cheng, J. Xing, Z. Pi, S. Liu, Z. Liu and F. Song, α-Glucosidase immobilization on functionalized Fe3O4 magnetic nanoparticles for screening of enzyme inhibitors, Chin. Chem. Lett., 2019, 30, 656–659 CrossRef CAS.
- J. Iqbal, S. Iqbal and C. E. Müller, Advances in immobilized enzyme microbioreactors in capillary electrophoresis, Analyst, 2013, 138, 3104–3116 RSC.
- P.-C. Guo, H.-D. Shen, J.-J. Fang, T.-M. Ding, X.-P. Ding and J.-F. Liu, On-line high-performance liquid chromatography coupled with biochemical detection method for screening of α-glucosidase inhibitors in green tea, Biomed. Chromatogr., 2018, 32, e4281 CrossRef.
- J. S. Schmidt, M. B. Lauridsen, L. O. Dragsted, J. Nielsen and D. Staerk, Development of a bioassay-coupled HPLC-SPE-ttNMR platform for identification of α-glucosidase inhibitors in apple peel (Malus×domestica Borkh.), Food Chem., 2012, 135, 1692–1699 CrossRef CAS.
- P. C. Guo, H. D. Shen, J. J. Fang, T. M. Ding, X. P. Ding and J. F. Liu, On-line high-performance liquid chromatography coupled with biochemical detection method for screening of α-glucosidase inhibitors in green tea, Biomed. Chromatogr., 2018, 32, e4281 CrossRef.
- S. Zhang, D. Wu, H. Li, J. Zhu, W. Hu, M. Lu and X. Liu, Rapid identification of α-glucosidase inhibitors from Dioscorea opposita Thunb peel extract by enzyme functionalized Fe3O4 magnetic nanoparticles coupled with HPLC-MS/MS, Food Funct., 2017, 8, 3219–3227 RSC.
- T. Xiao, Z. Guo, B. Sun and Y. Zhao, Identification of Anthocyanins from Four Kinds of Berries and Their Inhibition Activity to α-Glycosidase and Protein Tyrosine Phosphatase 1B by HPLC–FT-ICR MS/MS, J. Agric. Food Chem., 2017, 65, 6211–6221 CrossRef CAS.
- N. Zaharudin, D. Staerk and L. O. Dragsted, Inhibition of α-glucosidase activity by selected edible seaweeds and fucoxanthin, Food Chem., 2019, 270, 481–486 CrossRef CAS.
- L. Wang, Y. Liu, Y. Luo, K. Huang and Z. Wu, Quickly Screening for Potential α-Glucosidase Inhibitors from Guava Leaves Tea by Bioaffinity Ultrafiltration Coupled with HPLC-ESI-TOF/MS Method, J. Agric. Food Chem., 2018, 66, 1576–1582 CrossRef CAS.
- L. Zhang, L. Xu, Y.-h. Ye, M.-f. Zhu, J. Li, Z.-c. Tu, S.-h. Yang and H. Liao, Phytochemical profiles and screening of α-glucosidase inhibitors of four Acer species leaves with ultra-filtration combined with UPLC-QTOF-MS/MS, Ind. Crops Prod., 2019, 129, 156–168 CrossRef CAS.
- K. T. Kongstad, C. Özdemir, A. Barzak, S. G. Wubshet and D. Staerk, Combined Use of High-Resolution α-Glucosidase Inhibition Profiling and High-Performance Liquid Chromatography-High-Resolution Mass Spectrometry–Solid-Phase Extraction–Nuclear Magnetic Resonance Spectroscopy for Investigation of Antidiabetic Principles in Crude Plant Extracts, J. Agric. Food Chem., 2015, 63, 2257–2263 CrossRef CAS.
- Y. Zhao, K. T. Kongstad, A. K. Jäger, J. Nielsen and D. Staerk, Quadruple high-resolution α-glucosidase/α-amylase/PTP1B/radical scavenging profiling combined with high-performance liquid chromatography–high-resolution mass spectrometry–solid-phase extraction–nuclear magnetic resonance spectroscopy for identification of antidiabetic constituents in crude root bark of Morus alba L, J. Chromatogr. A, 2018, 1556, 55–63 CrossRef CAS.
- S. G. Wubshet, I. M. C. Brighente, R. Moaddel and D. Staerk, Magnetic Ligand Fishing as a Targeting Tool for HPLC-HRMS-SPE-NMR: α-Glucosidase Inhibitory Ligands and Alkylresorcinol Glycosides from Eugenia catharinae, J. Nat. Prod., 2015, 78, 2657–2665 CrossRef CAS.
- T. Li, K. T. Kongstad and D. Staerk, Identification of α-Glucosidase Inhibitors in Machilus litseifolia by Combined Use of High-Resolution α-Glucosidase Inhibition Profiling and HPLC-PDA-HRMS-SPE-NMR, J. Nat. Prod., 2019, 82, 249–258 CrossRef CAS.
- X. Zhang, G. Li, D. Wu, X. Li, N. Hu, J. Chen, G. Chen and Y. Wu, Recent progress in the design fabrication of metal-organic frameworks-based nanozymes and their applications to sensing and cancer therapy, Biosens. Bioelectron., 2019, 137, 178–198 CrossRef CAS.
- X. Zhang, D. Wu, X. Zhou, Y. Yu, J. Liu, N. Hu, H. Wang, G. Li and Y. Wu, Recent progress on the construction of nanozymes-based biosensors and their applications to food safety assay, TrAC, Trends Anal. Chem., 2019, 115668, DOI:10.1016/j.trac.2019.115668.
- J. Zhang, Y. Liu, J. Lv and G. Li, A colorimetric method for α-glucosidase activity assay and its inhibitor screening based on aggregation of gold nanoparticles induced by specific recognition between phenylenediboronic acid and 4-aminophenyl-α-d-glucopyranoside, Nano Res., 2015, 8, 920–930 CrossRef CAS.
- H. Chen, J. Zhang, H. Wu, K. Koh and Y. Yin, Sensitive colorimetric assays for α-glucosidase activity and inhibitor screening based on unmodified gold nanoparticles, Anal. Chim. Acta, 2015, 875, 92–98 CrossRef CAS.
- X. Cheng, Y. Huang, C. Yuan, K. Dai, H. Jiang and J. Ma, Colorimetric detection of α-glucosidase activity based on the etching of gold nanorods and its application to screen anti-diabetic drugs, Sens. Actuators, B, 2019, 282, 838–843 CrossRef CAS.
- T. Lin, M. Zhang, F. Xu, X. Wang, Z. Xu and L. Guo, Colorimetric detection of benzoyl peroxide based on the etching of silver nanoshells of Au@Ag nanorods, Sens. Actuators, B, 2018, 261, 379–384 CrossRef CAS.
- D. Wu, N. Hu, J. Liu, G. Fan, X. Li, J. Sun, C. Dai, Y. Suo, G. Li and Y. Wu, Ultrasensitive colorimetric sensing strategy based on ascorbic acid triggered remarkable photoactive-nanoperoxidase for signal amplification and its application to α-glucosidase activity detection, Talanta, 2018, 190, 103–109 CrossRef CAS.
- Y. Zhong, L. Yu, Q. He, Q. Zhu, C. Zhang, X. Cui, J. Zheng and S. Zhao, Bifunctional Hybrid Enzyme-Catalytic Metal Organic Framework Reactors for α-Glucosidase Inhibitor Screening, ACS Appl. Mater. Interfaces, 2019, 11, 32769–32777 CrossRef CAS.
- C. Tang, Z. Qian, Y. Qian, Y. Huang, M. Zhao, H. Ao and H. Feng, A fluorometric and real-time assay for α-glucosidase activity through supramolecular self-assembly and its application for inhibitor screening, Sens. Actuators, B, 2017, 245, 282–289 CrossRef CAS.
- W. Kong, D. Wu, L. Xia, X. Chen, G. Li, N. Qiu, G. Chen, Z. Sun, J. You and Y. Wu, Carbon dots for fluorescent detection of alpha-glucosidase activity using enzyme activated inner filter effect and its application to anti-diabetic drug discovery, Anal. Chim. Acta, 2017, 973, 91–99 CrossRef CAS PubMed.
- K. E. Sapsford, L. Berti and I. L. Medintz, Materials for fluorescence resonance energy transfer analysis: beyond traditional donor–acceptor combinations, Angew. Chem., Int. Ed., 2006, 45, 4562–4589 CrossRef CAS.
- X. Wu, Y. Song, X. Yan, C. Zhu, Y. Ma, D. Du and Y. Lin, Carbon quantum dots as fluorescence resonance energy transfer sensors for organophosphate pesticides determination, Biosens. Bioelectron., 2017, 94, 292–297 CrossRef CAS.
- F. Tian, J. Lyu, J. Shi and M. Yang, Graphene and graphene-like two-denominational materials based fluorescence resonance energy transfer (FRET) assays for biological applications, Biosens. Bioelectron., 2017, 89, 123–135 CrossRef CAS.
- Y. Liu, Q. Ouyang, H. Li, M. Chen, Z. Zhang and Q. Chen, Turn-On Fluoresence Sensor for Hg2+ in Food Based on FRET between Aptamers-Functionalized Upconversion Nanoparticles and Gold Nanoparticles, J. Agric. Food Chem., 2018, 66, 6188–6195 CrossRef CAS.
- G. Li, W. Kong, M. Zhao, S. Lu, P. Gong, G. Chen, L. Xia, H. Wang, J. You and Y. Wu, A fluorescence resonance energy transfer (FRET) based “Turn-On” nanofluorescence sensor using a nitrogen-doped carbon dot-hexagonal cobalt oxyhydroxide nanosheet architecture and application to α-glucosidase inhibitor screening, Biosens. Bioelectron., 2016, 79, 728–735 CrossRef CAS.
- D. Bu, H. Zhuang, G. Yang and X. Ping, An immunosensor designed for polybrominated biphenyl detection based on fluorescence resonance energy transfer (FRET) between carbon dots and gold nanoparticles, Sens. Actuators, B, 2014, 195, 540–548 CrossRef CAS.
- S. Lu, G. Li, Z. Lv, N. Qiu, W. Kong, P. Gong, G. Chen, L. Xia, X. Guo, J. You and Y. Wu, Facile and ultrasensitive fluorescence sensor platform for tumor invasive biomaker β-glucuronidase detection and inhibitor evaluation with carbon quantum dots based on inner-filter effect, Biosens. Bioelectron., 2016, 85, 358–362 CrossRef CAS.
- M. Zheng, Z. Xie, D. Qu, D. Li, P. Du, X. Jing and Z. Sun, On–Off–On Fluorescent Carbon Dot Nanosensor for Recognition of Chromium(VI) and Ascorbic Acid Based on the Inner Filter Effect, ACS Appl. Mater. Interfaces, 2013, 5, 13242–13247 CrossRef CAS.
- S. Huang, E. Yang, J. Yao, Y. Liu and Q. Xiao, Carbon dots doped with nitrogen and boron as ultrasensitive fluorescent probes for determination of α-glucosidase activity and its inhibitors in water samples and living cells, Microchim. Acta, 2018, 185, 394 CrossRef.
- G. Li, H. Fu, X. Chen, P. Gong, G. Chen, L. Xia, H. Wang, J. You and Y. Wu, Facile and sensitive fluorescence sensing of alkaline phosphatase activity with photoluminescent carbon dots based on inner filter effect, Anal. Chem., 2016, 88, 2720–2726 CrossRef CAS.
- M. Shi, Y. Cen, G. Xu, F. Wei, X. Xu, X. Cheng, Y. Chai, M. Sohail and Q. Hu, Ratiometric fluorescence monitoring of α-glucosidase activity based on oxidase-like property of MnO2 nanosheet and its application for inhibitor screening, Anal. Chim. Acta, 2019, 1077, 225–231 CrossRef CAS PubMed.
- H. Ao, H. Feng, X. Huang, M. Zhao and Z. Qian, A reversible fluorescence nanoswitch based on dynamic covalent B-O bonds using functional carbon quantum dots and its application for alpha-glucosidase activity monitoring, J. Mater. Chem. C, 2017, 5, 2826–2832 RSC.
- A. Cao, Y. Tang and Y. Liu, Novel Fluorescent Biosensor for α-Glucosidase Inhibitor Screening Based on Cationic Conjugated Polymers, ACS Appl. Mater. Interfaces, 2012, 4, 3773–3778 CrossRef CAS PubMed.
- S.-Y. Liu, H. Wang, T. He, L. Qi and Z.-Q. Zhang, Sensitive fluorimetric assays for α-glucosidase activity and inhibitor screening based on β-cyclodextrin-coated quantum dots, Luminescence, 2016, 31, 96–101 CrossRef CAS PubMed.
- G. Ziyatdinova, E. Kozlova and H. Budnikov, Selective electrochemical sensor based on the electropolymerized p-coumaric acid for the direct determination of l-cysteine, Electrochim. Acta, 2018, 270, 369–377 CrossRef CAS.
- J. Li, G. He, B. Wang, L. Shi, T. Gao and G. Li, Fabrication of reusable electrochemical biosensor and its application for the assay of α-glucosidase activity, Anal. Chim. Acta, 2018, 1026, 140–146 CrossRef CAS PubMed.
- Y. Liu, H. Li, L. Lu, B. Sun, L. Huang, H. Chen, W. Qiu, J. Tao and P. Zhao, A ratiometric electrochemical sensor with integrated probe for the assay of α-glucosidase activity and screening of its inhibitors, J. Electrochem. Soc., 2019, 166, B133–B140 CrossRef CAS.
- J. Zhang, Y. Liu, X. Wang, Y. Chen and G. Li, Electrochemical assay of α-glucosidase activity and the inhibitor screening in cell medium, Biosens. Bioelectron., 2015, 74, 666–672 CrossRef CAS PubMed.
- P. Wu, X. Hou, J.-J. Xu and H.-Y. Chen, Ratiometric fluorescence, electrochemiluminescence, and photoelectrochemical chemo/biosensing based on semiconductor quantum dots, Nanoscale, 2016, 8, 8427–8442 RSC.
- H. H. Nguyen, J. Park, S. Kang and M. Kim, Surface Plasmon Resonance: A Versatile Technique for Biosensor Applications, Sensors, 2015, 15, 10481–10510 CrossRef CAS PubMed.
- J. Homola, S. S. Yee and G. Gauglitz, Surface plasmon resonance sensors: review, Sens. Actuators, B, 1999, 54, 3–15 CrossRef CAS.
- J. Homola, Surface Plasmon Resonance Sensors for Detection of Chemical and Biological Species, Chem. Rev., 2008, 108, 462–493 CrossRef CAS.
- H. Yang, A. L. Chan, V. LaVallo and Q. Cheng, Quantitation of Alpha-Glucosidase Activity Using Fluorinated Carbohydrate Array and MALDI-TOF-MS, ACS Appl. Mater. Interfaces, 2016, 8, 2872–2878 CrossRef CAS PubMed.
- Q. Shang, J.-F. Xiang and Y.-L. Tang, Screening alpha-glucosidase inhibitors from mulberry extracts via DOSY and relaxation-edited NNR, Talanta, 2012, 97, 362–367 CrossRef CAS PubMed.
- C.-C. Chen, P.-H. Chuang, Y.-S. Chen, C.-H. Yao and H.-M. Chen, Chip-based drug screening for inhibiting α-glucosidase, Fitoterapia, 2011, 82, 1249–1257 CrossRef CAS PubMed.
- X. Zhang, G. Li, D. Wu, B. Zhang, N. Hu, H. Wang, J. Liu and Y. Wu, Recent advances in the construction of functionalized covalent organic frameworks and their applications to sensing, Biosens. Bioelectron., 2019, 111699, DOI:10.1016/j.bios.2019.111699.
- S. Kandambeth, V. Venkatesh, D. B. Shinde, S. Kumari, A. Halder, S. Verma and R. Banerjee, Self-templated chemically stable hollow spherical covalent organic framework, Nat. Commun., 2015, 6, 6786 CrossRef CAS PubMed.
- Q. Sun, C.-W. Fu, B. Aguila, J. Perman, S. Wang, H.-Y. Huang, F.-S. Xiao and S. Ma, Pore Environment Control and Enhanced Performance of Enzymes Infiltrated in Covalent Organic Frameworks, J. Am. Chem. Soc., 2018, 140, 984–992 CrossRef CAS PubMed.
- J. Wu, S. Li and H. Wei, Integrated nanozymes: facile preparation and biomedical applications, Chem. Commun., 2018, 54, 6520–6530 RSC.
- S. Laing, L. E. Jamieson, K. Faulds and D. Graham, Surface-enhanced Raman spectroscopy for in vivo biosensing, Nat. Rev. Chem., 2017, 1, 0060 CrossRef CAS.
- Y. Su, D. Wu, J. Chen, G. Chen, N. Hu, H. Wang, P. Wang, H. Han, G. Li and Y. Wu, Ratiometric Surface Enhanced Raman Scattering Immunosorbent Assay of Allergenic Proteins via Covalent Organic Framework Composite Material Based Nanozyme Tag Triggered Raman Signal “Turn-on” and Amplification, Anal. Chem., 2019, 91, 11687–11695 CrossRef CAS PubMed.
- Z. Tang, H. Chen, H. He and C. Ma, Assays for alkaline phosphatase activity: Progress and prospects, TrAC, Trends Anal. Chem., 2019, 113, 32–43 CrossRef CAS.
- X. Liu, D. Huang, C. Lai, G. Zeng, L. Qin, C. Zhang, H. Yi, B. Li, R. Deng, S. Liu and Y. Zhang, Recent advances in sensors for tetracycline antibiotics and their applications, TrAC, Trends Anal. Chem., 2018, 109, 260–274 CrossRef CAS.
- Y. Li, Z. Wang, L. Sun, L. Liu, C. Xu and H. Kuang, Nanoparticle-based sensors for food contaminants, TrAC, Trends Anal. Chem., 2019, 113, 74–83 CrossRef CAS.
- D.-M. Liu, J. Chen and Y.-P. Shi, Screening of enzyme inhibitors from traditional Chinese medicine by magnetic immobilized α-glucosidase coupled with capillary electrophoresis, Talanta, 2017, 164, 548–555 CrossRef CAS PubMed.
- R. L. C. Voeten, I. K. Ventouri, R. Haselberg and G. W. Somsen, Capillary Electrophoresis: Trends and Recent Advances, Anal. Chem., 2018, 90, 1464–1481 CrossRef CAS PubMed.
- D.-Q. Li, Z.-M. Qian and S.-P. Li, Inhibition of Three Selected Beverage Extracts on α-Glucosidase and Rapid Identification of Their Active Compounds Using HPLC-DAD-MS/MS and Biochemical Detection, J. Agric. Food Chem., 2010, 58, 6608–6613 CrossRef CAS PubMed.
- D. Q. Li, J. Zhao, J. Xie and S. P. Li, A novel sample preparation and on-line HPLC–DAD–MS/MS–BCD analysis for rapid screening and characterization of specific enzyme inhibitors in herbal extracts: Case study of α-glucosidase, J. Pharm. Biomed. Anal., 2014, 88, 130–135 CrossRef CAS PubMed.
- S. G. Wubshet, H. H. Moresco, Y. Tahtah, I. M. C. Brighente and D. Staerk, High-resolution bioactivity profiling combined with HPLC–HRMS–SPE–NMR: α-Glucosidase inhibitors and acetylated ellagic acid rhamnosides from Myrcia palustris DC. (Myrtaceae), Phytochemistry, 2015, 116, 246–252 CrossRef CAS PubMed.
- G. Chen and M. Guo, Rapid Screening for α-Glucosidase Inhibitors from Gymnema sylvestre by Affinity Ultrafiltration–HPLC-MS, Front. Pharmacol., 2017, 8, 228 CrossRef PubMed.
- S. Deng, L. Xia and H. Xiao, Screening of α-glucosidase inhibitors from green tea extracts using immobilized enzymes affinity capture combined with UHPLC-QTOF MS analysis, Chem. Commun., 2014, 50, 2582–2584 RSC.
- A.-h. Ge, Y. Bai, J. Li, J. Liu, J. He, E.-w. Liu, P. Zhang, B.-l. Zhang, X.-m. Gao and Y.-x. Chang, An activity-integrated strategy involving ultra-high-performance liquid chromatography/quadrupole-time-of-flight mass spectrometry and fraction collector for rapid screening and characterization of the α-glucosidase inhibitors in Coptis chinensis Franch. (Huanglian), J. Pharm. Biomed. Anal., 2014, 100, 79–87 CrossRef CAS PubMed.
- L. Wang, Q. Cui, Y. Hou, F. Bai, J. Sun, X. Cao, P. Liu, M. Jiang and G. Bai, An integrated strategy of ultra-high-performance liquid chromatography/quadrupole-time-of-flight mass spectrometry and virtual screening for the identification of α-glucosidase inhibitors in acarviostatin-containing complex, J. Chromatogr. A, 2013, 1319, 88–96 CrossRef CAS PubMed.
- Y. Zhao, M. X. Chen, K. T. Kongstad, A. K. Jäger and D. Staerk, Potential of Polygonum cuspidatum Root as an Antidiabetic Food: Dual High-Resolution α-Glucosidase and PTP1B Inhibition Profiling Combined with HPLC-HRMS and NMR for Identification of Antidiabetic Constituents, J. Agric. Food Chem., 2017, 65, 4421–4427 CrossRef CAS PubMed.
- E. L. Silva, R. C. Almeida-Lafetá, R. M. Borges and D. Staerk, Dual high-resolution inhibition profiling and HPLC-HRMS-SPE-NMR analysis for identification of α-glucosidase and radical scavenging inhibitors in Solanum americanum Mill, Fitoterapia, 2017, 118, 42–48 CrossRef CAS PubMed.
- S. G. Wubshet, Y. Tahtah, A. M. Heskes, K. T. Kongstad, I. Pateraki, B. Hamberger, B. L. Møller and D. Staerk, Identification of PTP1B and α-Glucosidase Inhibitory Serrulatanes from Eremophila spp. by Combined use of Dual High-Resolution PTP1B and α-Glucosidase Inhibition Profiling and HPLC-HRMS-SPE-NMR, J. Nat. Prod., 2016, 79, 1063–1072 CrossRef CAS PubMed.
|
This journal is © The Royal Society of Chemistry 2020 |
Click here to see how this site uses Cookies. View our privacy policy here.