DOI:
10.1039/C9FO02095K
(Paper)
Food Funct., 2020,
11, 730-740
Luteolin reduces fat storage in Caenorhabditis elegans by promoting the central serotonin pathway†
Received
9th September 2019
, Accepted 20th December 2019
First published on 23rd December 2019
Abstract
Serotonin plays a critical role in regulating energy homeostasis and fat metabolism. Various dietary flavonoids, e.g. luteolin and quercetin, possess potential anti-obesity properties. However, it is unclear whether the flavonoids exert their anti-obesity actions via central serotonin signaling. Here, employing a classic animal model C. elegans, we assessed the effects of six dietary flavonoids (flavones apigenin, chrysin, and luteolin and flavonols kaempferol, myricetin, and quercetin) on fat accumulation. The dose-dependent study revealed the substantial inhibitory actions of the six flavonoids on C. elegans fat accumulation and the strongest inhibitory activity of luteolin among the tested flavonoids. Meanwhile, flavonoid treatments did not have an obvious influence on worm growth, fecundity and feeding. Furthermore, the mutation of tph-1, which encodes the conserved rate-limiting enzyme of serotonin synthesis, fully abolished luteolin-induced fat loss but did not affect fat reduction by the other five flavonoids. In wild-type N2 worms, luteolin treatment not only elevated the expression of tph-1, but also enhanced the mRNA levels of mod-1 and ser-6, which are two serotonin-related receptors and play specific roles in serotonin-mediated fat reduction. The mutation of either mod-1 or ser-6 also fully abolished luteolin-induced fat loss. Finally, we found that luteolin treatment elevated serotonin synthesis in ADF neurons to promote lipolysis and fatty acid β-oxidation in C. elegans. Together, the results indicated that luteolin reduced C. elegans fat storage by promoting central serotonin signaling, suggesting new insights into elucidating the mechanism underlying fat regulation by luteolin.
Introduction
Obesity, characterized by excessive fat accumulation in the body, has become a worldwide epidemic disease. Excessive fat accumulation results in lipid metabolism dysfunction and chronic inflammation, and hence is strongly linked to many metabolic comorbidities, such as type 2 diabetes, fatty liver, cardiovascular disease, neurodegenerative disorder, and even certain cancers.1,2 Therefore, investigating critical modulators and associated signaling pathways on fat metabolism and energy homeostasis may suggest some viable approaches to combat obesity and metabolic diseases.
As a phylogenetically ancient signaling molecule, serotonin is found in all vertebrate and invertebrate species with nervous systems and orchestrates various behavioral and physiological responses mediating energy balance in the organisms.3–5 In mammals, multiple lines of evidence indicate that the 5-HT2C receptor is a crucial mediator of central serotonergic suppression of feeding. Pharmacological manipulations that enhance the central serotonin level6,7 or activate the 5-HT2C receptor8,9 result in animal hypophagia and body weight loss. By contrast, the genetic mutation of the 5-HT2C receptor leads to hyperphagia and obesity.10–12 Therefore, therapeutic targeting of this receptor for the development of agonists can offer a viable approach to combat obesity and metabolic diseases. In 2012, a specific agonist of the 5-HT2C receptor, lorcaserin, became the first FDA approved anti-obesity drug since 1999.13 However, it remains unknown whether the central serotoninergic pathway plays a direct role in fat metabolism or the aforesaid anti-obesity efforts are merely secondary to the anorectic action of central serotonin signaling. Given the complexity of the mammalian serotonin signaling and energy regulatory pathway,3,4 it is a challenging task to delineate the molecular mechanism through which the central serotonergic pathway impacts fat storage independently of feeding regulation.
Yet, the nematode Caenorhabditis elegans (C. elegans) offers an opportunity to identify modulators of fat metabolism and dissect complicated molecular circuits between tissues.5,14 In the tractable animal model, the core lipid metabolic pathways, such as insulin, TOR, serotonin, dopamine and glutamate pathways, are highly conserved with mammals.14 Unlike the ubiquitous nature of serotonergic influences in mammals,3,4C. elegans serotonin is synthesized by the conserved rate-limiting enzyme tryptophan hydroxylase (TPH) in only a few neurons, including head sensory ADF, pharyngeal NSM, and hermaphrodite-specific HSN neurons.5 In wild-type N2 worms, exogenous serotonin treatment elevates the feeding rate but lowers the body fat content.15 Conversely, serotonin-deficient tph-1 mutants exhibit increased body fat accumulation but decreased feeding rate.15,16 The inverse correlation between the feeding rate and fat content points out that a serotoninergic circuit can be independent of feeding regulation to control C. elegans fat storage.15 Recently, such central serotoninergic signaling, including serotonin from ADF neurons and serotonin-related receptors SER-6 in AWB neurons and MOD-1 in URX neurons as well as intestinal adipocyte triglyceride lipase ATGL-1, has been identified in C. elegans,17,18 suggesting that C. elegans serves as a suitable model to investigate central serotonin-mediated fat regulation.
Flavonoids, with a 15-carbon skeleton structure with two phenyl rings (i.e., A and B rings) and a heterocyclic C ring, are the secondary metabolites of plants and are widely distributed in edible plants. Based on the differences in the degree of oxidation and the substitution pattern of the C ring, flavonoids can be divided into several subclasses, including flavones and flavonols.19,20 Within the two subgroups of flavonoids, the flavones luteolin and apigenin and flavonols quercetin, kaempferol and myricetin are the commonest in foods and beverages of plant origin.19 Our previous studies showed that dietary supplementation of both luteolin21 and quercetin22 reduced body and adipose tissue weight gain and improved insulin resistance in mice fed with a high-fat diet (HFD). Dietary apigenin,23 kaempferol,24 and myricetin25 have also been reported to protect HFD-fed mice from obesity and associated metabolic alterations. The studies suggest the substantial activities of the compounds in counteracting fat accumulation. However, whether they exert the anti-obesity actions via central serotonin signaling remains unclear.
The biological activities of flavonoids depend on their chemical structure.26,27 As a group of polyphenolic compounds, both the degree and site of hydroxylation may affect the fat-regulatory activities of various flavonoids. The above-mentioned five common dietary flavonoids can be regarded as the derivatives of 5,7-dihydroxylflavone (i.e., chrysin), which is a natural flavone found in flowers, honeycombs, and mushrooms and can enhance lipid metabolism in 3T3-L1 adipocytes28 (Fig. 1). Based on the backbone of 5,7-dihydroxylflavone, the flavones apigenin and luteolin have the substitution of the C-4′ and C-3′,4′ hydroxyl group in the B ring, respectively. The flavonols kaempferol, quercetin, and myricetin possess 3-OH in the C ring and variable substitution of a hydroxyl group in the B ring (Fig. 1). The small structural differences based on the same nuclear skeleton are helpful for us to identify some critical substitutions of the hydroxyl group affecting the fat-regulatory activity of flavonoids. In this study, we utilized C. elegans as an in vivo model to assess the effects of the five dietary flavonoids and their skeleton molecule chrysin on fat accumulation. Meanwhile, at a 100 μM dose of flavonoids, the growth rate, brood size, and pumping rate were also determined in the worms. Furthermore, in serotonin-deficient tph-1 mutants, we examined the changes of worm fat contents after flavonoid treatment. Finally, the role of central serotonin signaling in the luteolin-induced fat loss was identified in C. elegans.
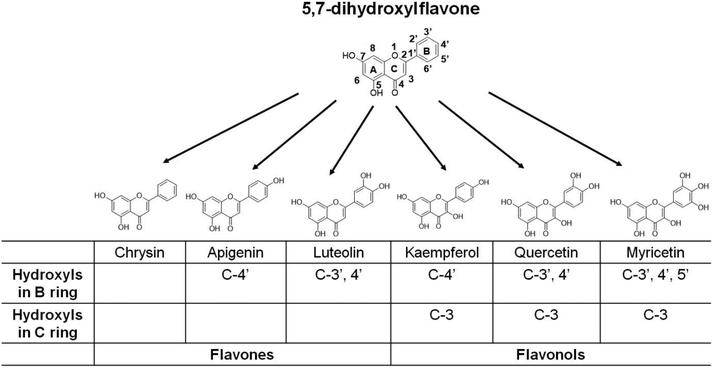 |
| Fig. 1 The chemical structures of flavonoids and the substitutional positions of hydroxyl groups in each flavonoid in this study, including the flavones chrysin, apigenin, and luteolin, and the flavonols kaempferol, quercetin, and myricetin. | |
Materials and methods
C. elegans
All worms were maintained at 20 °C according to the standard protocols in our experiment unless specifically noted.29 N2 Bristol was used as a wild-type reference strain. Four mutant strains, tph-1(mg280)II, mod-1(ok103)V, ser-6(tm2146)IV and mgls42[tph-1::GFP+pRF4(rol-6(su1006))], were obtained from the Caenorhabditis Genetics Center (CGC). The worm expressing lipid droplet marker DHS-3 fused green fluorescence protein (GFP) (LIU1 (ldrIs1 [dhs-3p::dhs-3::GFP+unc-76(+)])) was kindly provided by Professor Pingsheng Liu (State Key Laboratory of Biomacromolecules, Institute of Biophysics, Chinese Academy of Sciences). To investigate the effects of flavonoids on fat accumulation in C. elegans, the flavonoids were added into the sterile Nematode Growth Medium (NGM) after filtration sterilization at about 60 °C, respectively. The worms were fed on the plates with flavonoids from the L1 larva stage. After 44 h at 20 °C, the adult worms were harvested. To exclude the influence of bacterial metabolism, OP50 bacteria were killed by moist heat sterilization and dead OP50 bacteria were seeded on the NGM plates with different flavonoids.
Concentration and structure analysis of flavonoids in NGM plates
The concentration and structure of these flavonoids in NGM plates were measured by HPLC and UPLC-MS. The flavonoids were extracted using methanol. The concentration of these flavonoids was measured using a HPLC system equipped with a 150 × 4.6 mm C18-reversed phase column with a UV absorbance detector at 350 nm. The structure of these flavonoids was determined on a Waters Acquity UPLC LCT Premier XE. MS parameters were as follows: capillary voltage, 2500 V; cone voltage, 100 V; drying gas temperature, 350 °C; gas flow (N2) rate, 800 L h−1. The instrument was operated by positive/negative ion mode scanning.
Oil Red O staining and quantitation
Oil Red O staining was carried out as described with minor changes.30 0.5% Oil Red O solution was dissolved in 1,2-propanediol and placed on a bench for 1–2 days. Before staining, Oil Red O solution was filtered through a 0.22 μm syringe filter. Day 1 adult worms were washed from NGM plates with M9 buffer three times. After gravitational settling in tubes, 1000 μL M9 buffer and 50 μL 10% paraformaldehyde were added and immediately frozen at −80 °C. The tubes were thawed and refrozen for three cycles. Then the worms were washed in cold M9 buffer three times. The worms were dehydrated in 1,2-propanediol for 5 min and stained with 1 mL filtered Oil Red O solution for 5 h at room temperature. After staining, the worms were washed with 85% 1,2-propanediol and PBS and mounted on a 2% agarose slide for imaging. Oil Red O quantification was carried out as described with minor changes.30 The level of Oil Red O was quantified from the original images by determining the excess intensity in the red channel compared to the green and blue channels. Then the area of these regions was normalized by the area of the stained worm regions. 30 worms of each group were randomly selected and imaged as described above and quantified. Each experiment was repeated at least three times and 3000 worms were used in each experiment.
Phenotypic analysis of the developmental rate, brood size, and pumping rate
The phenotypic analysis of the developmental rate, brood size, and pumping rate was carried out as described15,31,32 with minor changes. The N2 worms were fed on standard NGM plates with E. coli strain OP50 and eggs were collected. Then, the synchronized L1 larvae were transferred to NGM plates with different flavonoids and incubated at 20 °C. After 50 h, the worms were washed from the NGM plates and mounted on agarose pads and examined under a compound microscope. The worms of L4 larva, young adult and gravid adult stages were distinguished by the development of the vulva. For each experiment at least 30 worms were scored. For brood sizes, the synchronized L1 larvae were transferred to NGM plates with different flavonoids and incubated at 20 °C. Then, the well-fed individual L4 larvae were transferred to NGM plates with the corresponding flavonoids. The daily laid eggs of each animal were counted until egg-laying stopped. The numbers of daily laid eggs were added up as the numbers of progeny. For each flavonoid treatment, at least 30 worms were counted. Pumping rates were measured in the day 1 adults raised at 20 °C on OP50 bacteria with or without six different flavonoids. The pumping rate for each worm was examined by counting the times of the rhythmic contractions of the pharyngeal bulb in 30 s. For each flavonoid treatment, 30 animals were counted. Each analysis was repeated at least three times.
Quantitative real-time PCR
Total RNA of the worms was isolated using TRIzol reagent (TaKaRa), and cDNA was synthesized according to the manufacturer's protocol of PrimeScript™ RT Master Mix (TaKaRa). The cDNA was used as a template for real-time PCR (Bio-Rad MyiQ2 Real-time PCR System) in the presence of SYBR® Premix Ex Taq™ II (TaKaRa). All primer sequences for C. elegans are listed in ESI Table S1.† All data were processed using the ΔΔCT method and gpd-2 was used as the reference gene.
Statistical analysis
Data were presented as mean ± SEM. Student's t-test and one-way ANOVA were used to evaluate the difference among various C. elegans assay groups. GraphPad Prism 7.01 (GraphPad Software, Inc.) was used as the statistical software and p < 0.05 was considered as statistically significant.
Results
Treatments with dietary flavonoids reduced fat accumulation in C. elegans
Under laboratory conditions, C. elegans is usually grown on NGM plates with OP50 bacteria. To evaluate the effects of these six flavonoids on worm fat accumulation, we added flavonoids into the sterile NGM before solidifying at about 60 °C and developmentally synchronized L1 nematodes were cultured on the NGM plates with different concentrations of flavonoids (25, 50 and 100 μM), respectively. Using HPLC and UPLC-MS, we found that both the concentration and structure of these flavonoids were not affected by the supplemented procedures (ESI Fig. S1†). Utilizing Oil Red O staining, we found that only luteolin significantly reduced worm fat contents at a dose of 25 μM (Fig. 2A and B). At a dose of 50 μM, besides luteolin, quercetin also led to body fat loss (Fig. 2A and B). At a dose of 100 μM, all six tested flavonoids clearly induced a decline in C. elegans fat accumulation (Fig. 2A and B). Unlike mammals having specially appointed adipocytes, C. elegans store fat in lipid droplets in their intestinal and hypodermal cells.14 Thus, to further confirm the activities that the six flavonoids induced fat loss, we used a transgenic strain expressing lipid droplet marker protein DHS-3 (pDHS-3::DHS-3::GFP) to detect the fluorescence signals of lipid droplets.33 Consistent with the results of Oil Red O staining, at a dose of 100 μM, all the six flavonoids markedly lowered the amount of lipid droplets marked by DHS-3::GFP (Fig. 2C and D). Among the six flavonoids, luteolin most significantly reduced the fluorescence intensity of lipid droplets (Fig. 2C and D). We also analyzed the correlation between Oil Red O staining and DHS-3::GFP expression with Kappa analysis and found that both techniques presented a concordant result (Fig. 2E).
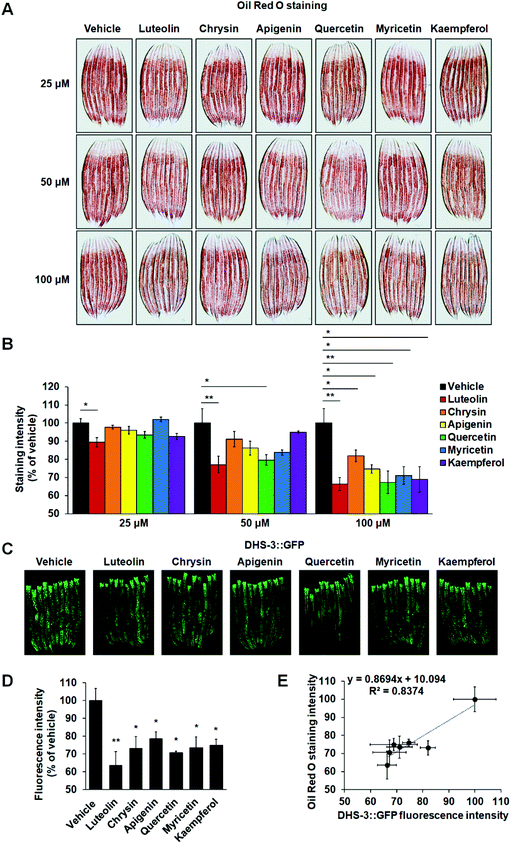 |
| Fig. 2 Flavonoid treatment reduces fat storage in C. elegans. (A and B) N2 wild-type worms were fed on NGM plates with or without different concentrations of flavonoids (25, 50, and 100 μM), respectively. Representative images (A) and quantification (B) of Oil Red O staining in N2 worms. (C and D) DHS-3::GFP worms were fed on NGM plates with or without 100 μM flavonoids. Representative images (C) and quantification (D) of green fluorescence in DHS-3::GFP worms. For each flavonoid treatment, at least 30 worms were quantified. In each flavonoid-treated condition, three independent experiments were performed. (E) The correlation analysis between Oil Red O staining intensity and DHS-3::GFP fluorescence intensity. The data in (B), (D) and (E) are presented as mean ± SEM. *p < 0.05 and **p < 0.01 by one-way ANOVA. | |
It is well known that flavonoids work as antibacterial agents against a wide range of pathogenic microorganisms.34 To exclude the influence of bacterial metabolism, developmentally synchronized L1 nematodes were cultured on the NGM plates seeded with dead OP50 bacteria with different flavonoids at 100 μM. Utilizing Oil Red O staining, we found that at a dose of 100 μM, all six tested flavonoids also induced a decline in fat accumulation in C. elegans fed with dead OP50 bacteria (ESI Fig. S2†). Together, the results suggest the substantial activities of the flavonoids in inhibiting C. elegans fat accumulation and luteolin possesses the highest inhibitory capability among the six flavonoids.
Treatments with dietary flavonoids did not affect the growth, fecundity, and feeding of C. elegans
In C. elegans, fat synthesis is a requirement for normal growth and fecundity, and food-related behaviors may potently regulate fat metabolism.5,35 To investigate whether flavonoid treatment affects these physiological events or flavonoid-induced fat loss is merely secondary to the changes of the events, we determined the developmental rate, brooding size, and pumping rate in the worms. At 100 μM, all six flavonoid treatments did not significantly affect worm growth (Fig. 3A and ESI Table S2†). In addition, the treatments also did not show an obvious impact on the brood size and pumping rate of C. elegans (Fig. 3B and C). Therefore, the flavonoid-induced fat loss did not correlate with the growth, fecundity, and feeding of C. elegans.
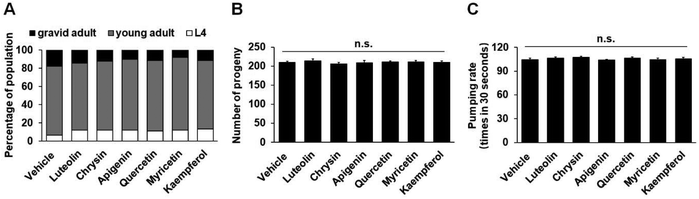 |
| Fig. 3 Flavonoid treatments do not affect the growth, fecundity and feeding of C. elegans. L1 larvae of N2 worm were placed onto the NGM plates with or without 100 μM flavonoids and maintained at 20 °C. (A) At 50 h after synchronization, the numbers of L4 larva, young adult and gravid adult worms were visually counted based on the development of the vulva. The animal percentage of each developmental stage is shown, black: gravid adult; gray: young adult; white: L4 larva; n = 30. (B) The number of progenies, n = 30. (C) The pharyngeal pumping rate, n = 30. All data are presented as mean ± SEM, n.s.: not significant vs. vehicle by one-way ANOVA. | |
The central serotonin signaling pathway was required for luteolin-induced body fat loss
In C. elegans, serotonin as a classic neurotransmitter regulates multiple physiological functions, such as feeding, egg-laying, and fat metabolism.5,14,35 A single TPH gene tph-1 is expressed in only a few central neurons and controls serotonin synthesis. tph-1 Mutants fail to synthesize serotonin and show increased body fat accumulation.15,16 To investigate the possible role of central serotonin signaling in flavonoid-induced fat loss, we fed N2 worms and tph-1(mg280) mutants on standard NGM plates or NGM plates with 100 μM flavonoids and utilized Oil Red O staining to examine their fat storage. Supporting the role of central serotonin signaling in worm fat regulation,15,16tph-1 mutants showed significantly more fat accumulation than the corresponding N2 worms on either standard or flavonoid-containing NGM plates (Fig. 4A and B). Meanwhile, in N2 worms, all six flavonoids induced fat loss again (Fig. 4A and B). Intriguingly, the mutation of tph-1 reversed luteolin-induced fat loss. Such reversion did not occur in those worms receiving the treatment of the other five flavonoids (Fig. 4A and B). Furthermore, in flavonoid-treated N2 worms, we used real-time PCR to detect the expressions of tph-1 and several serotonin-related receptor genes, including mod-1, ser-1, ser-4, and ser-6. Luteolin treatment enhanced the expression of tph-1 and the mRNA levels of mod-1 and ser-6 that play specific roles in serotonin-mediated fat loss,15,17,18 while it did not affect those of ser-1 and ser-4 that are required for serotonergic regulation of feeding and/or egg-laying15,31,36 (Fig. 4C). Although quercetin treatment also significantly elevated tph-1 expression, it did not affect the mRNA levels of any serotonin-related receptor genes. The other four flavonoids did not affect the expressions of all tested genes. Together, the results indicate that luteolin may induce fat loss by promoting central serotonin signaling.
 |
| Fig. 4 Serotonin signaling is required for luteolin-induced fat loss. (A and B) N2 worms and tph-1(mg280) mutants were fed on NGM plates with or without 100 μM flavonoids. The representative images (A) and quantification (B) of Oil Red O staining. (C) The real-time PCR analysis of genes involved in the serotonin signaling pathway in N2 worms. Three independent experiments were performed in each treatment condition. gpd-2 was used as the reference gene in real-time PCR analysis. The data in (B) and (C) are presented as mean ± SEM. *p < 0.05, **p < 0.01, and n.s.: not significant by one-way ANOVA. | |
Luteolin treatment elevated serotonin synthesis in ADF neurons and hence induced lipolysis and fatty acid β-oxidation
Given that luteolin treatment increased the expressions of mod-1 and ser-6 (Fig. 4C) and MOD-1 and SER-6 play a specific role in serotonergic regulation of fat in C. elegans,15,17,18 the critical serotonin-related receptors should also be required for luteolin-induced fat loss. To test the notion, we investigated the effect of 100 μM luteolin on the fat levels in mod-1(ok103) and ser-6(tm2146) mutants. As expected, luteolin treatment reduced fat accumulation in N2 worms (Fig. 5A–C). However, either mod-1 or ser-6 mutation fully reversed the fat-reducing effects of luteolin (Fig. 5A–C), suggesting the pivotal roles of MOD-1 and SER-6 in luteolin-induced fat loss.
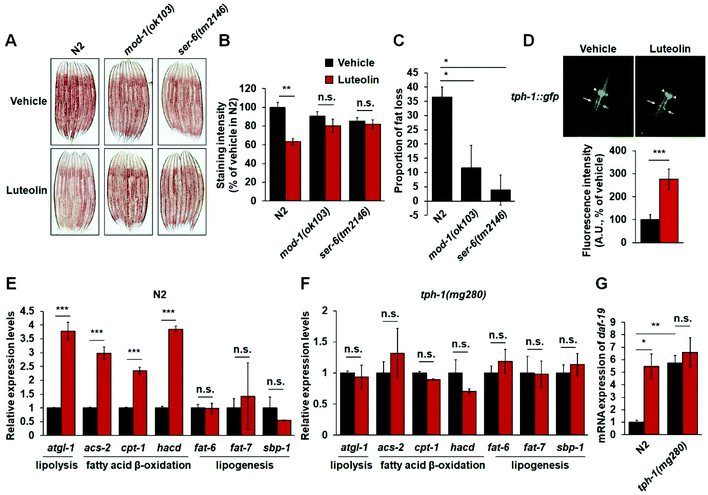 |
| Fig. 5 Luteolin treatment induces fat loss by promoting central serotonin signaling. (A and B) N2 worms and mod-1(ok103) and ser-6(tm2146) mutants were fed on NGM plates with or without 100 μM luteolin. Representative images (A) and quantification (B) of Oil Red O staining. (C) The quantification of fat loss in N2, mod-1(ok103) and ser-6(tm2146) worms fed with 100 μM luteolin. (D) The expression and quantification of C. elegans strain mgls42 expressing green fluorescence protein fused TPH-1 fed on NGM plates with or without 100 μM luteolin. tph-1::gfp was visible in NSM (arrowheads) and ADF (arrows). (E and F) The real-time PCR analysis of genes involved in lipolysis, fatty acid β-oxidation and lipogenesis in N2 worms (E) or tph-1(mg280) mutants (F) receiving 100 μM luteolin treatment. (G) The real-time PCR analysis of daf-19 gene expression in N2 and tph-1(mg280) worms receiving 100 μM luteolin treatment. gpd-2 was used as the reference gene in real-time PCR analysis. The data in (B), (C), (D), (E), (F) and (G) are presented as mean ± SEM. For (C), one-way ANOVA, and for (B), (D), (E), (F) and (G), two tailed Student's t-test were used for statistical analysis, *p < 0.05, **p < 0.01, ***p < 0.001 and n.s.: not significant. | |
In C. elegans, it has been well defined that central serotonin signaling regulates fat loss. In central serotonin signaling, octopaminergic receptor SER-6 in AWB neurons is required for serotonin synthesis in ADF neurons. Serotonin from ADF neurons activates MOD-1 in URX body cavity neurons, and finally induces lipolysis and fatty acid β-oxidation in the intestine.15,17,18 Therefore, we utilized C. elegans strain mgls42, which are transgenic worms expressing TPH-1 fused GFP to assess the effect of luteolin on central serotonin synthesis. Notably, luteolin treatment induced an increased fluorescence intensity of tph-1::gfp in ADF neurons, rather than in NSM neurons (Fig. 5D). Furthermore, we examined the expressions of genes related to lipolysis, fatty acid β-oxidation and lipogenesis, including atgl-1, acs-2, cpt-1, hacd, fat-6, fat-7 and sbp-1. Luteolin treatment significantly increased the gene expression of lipolysis and fatty acid β-oxidation in N2 worms (Fig. 5E). However, in tph-1 mutants (Fig. 5F), the expressions of all tested genes were not affected by the treatment with luteolin. It is reported that RFX transcription factor DAF-19 could regulate the transcription of tph-1 in ADF neurons responding to the immune response.37 Thus, we detected mRNA expression of daf-19 in both N2 worms and tph-1(mg280) mutants. Luteolin treatment elevated daf-19 expression in N2 worms (Fig. 5G). However, tph-1 mutation abolished the influences of luteolin on daf-19 expression. The results show that luteolin treatment promotes serotonin synthesis in ADF neurons and activates the central serotonin-related receptors MOD-1 and SER-6 and finally induces intestinal lipolysis and fatty acid β-oxidation in C. elegans.
Discussion
The prevalence of obesity and metabolic diseases highlights the need for finding strategies to prevent fat accumulation and understanding the associated regulatory pathway. Luteolin, as a common dietary flavonoid, has been reported to possess potential anti-obesity properties. In HFD-fed mice, a diet supplement of luteolin ameliorates diet-induced obesity and insulin resistance.21 In mouse 3T3-L1 pre-adipocytes, luteolin treatment inhibits rosiglitazone- and insulin-induced adipogenesis.38,39 Moreover, its action on fat regulation is attributed to stabilizing mast cells,21 promoting UCP-1-mediated adipocyte browning and thermogenesis,40 and selectively modulating PPARγ activity.38 Given that C. elegans does not have specialized immune cells41 and adipocytes14 and the need for the maintenance of body temperature,42 some other conservative signaling pathways may play a critical role in luteolin-induced worm fat loss.
Serotonin is an ancient signaling molecule and is found in all vertebrate and invertebrate species with nervous systems.3–5 Recently, central serotonin signaling, which is integrated with octopamine signaling, has been found to control C. elegans body fat.17 Central serotonin signaling originates from octopaminergic receptor SER-6 in AWB neurons and is required for serotonin synthesis in ADF neurons. In turn, serotonin from ADF neurons activates serotoninergic receptor MOD-1 in URX neurons and finally stimulates intestinal lipolysis and fatty acid β-oxidation.17,18 Here, in the context of luteolin treatment, we recapitulated central serotonin signaling and demonstrated that luteolin promoted serotonin synthesis in ADF neurons and activated SER-6 and MOD-1 to induce lipolysis and fatty acid β-oxidation in C. elegans (Fig. 6). Consistent with the notion that serotonergic fat regulation is independent of its feeding regulation,15 luteolin-induced fat loss was not accompanied by the changes of feeding in C. elegans (Fig. 3C). Luteolin treatment merely elevated the expression of serotonin-related receptors ser-6 and mod-1 that play a specific role in serotonin-mediated fat regulation,15 but did not affect the mRNA level of ser-1, which is required for serotonergic feeding and egg-laying15,31 (Fig. 4C). Thus, luteolin can be regarded as an exogenous activator of serotonergic fat regulation in C. elegans and specifically induces worm fat loss.
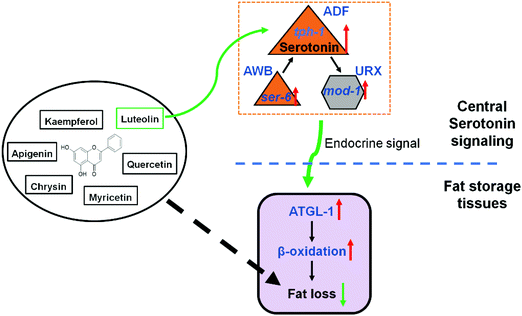 |
| Fig. 6 Schematic depiction of the role of central serotonin signaling in luteolin-induced fat loss. The six common diet flavonoids, including the flavones chrysin, apigenin, and luteolin, and the flavonols kaempferol, quercetin, and myricetin share a basic skeleton of 5,7-dihydroxylflavone. At a dose of 100 μM, all flavonoids can successfully induce fat loss in C. elegans. However, among them, luteolin is the only chemical that promotes central serotonin signaling to induce fat loss. As shown in this figure, luteolin treatment increases serotonin synthesis in ADF neurons and activates serotonin-related receptors MOD-1 and SER-6 and finally elevates lipolysis and fatty acid β-oxidation in C. elegans. | |
C. elegans fat accumulation is regulated by a complex molecular network, including hundreds of genes with functions in food sensation, neuroendocrine signaling, and metabolic pathways of fat.14 In this study, although only luteolin induced fat loss at a dose of 25 μM, the treatments with the other five tested flavonoids also significantly inhibited worm fat accumulation at their higher doses (50 or 100 μM) (Fig. 2). The mutation of tph-1 did not affect their action on worm fat contents (Fig. 3), suggesting that the flavonoids reduced worm fat storage through some pathways independent of central serotonin signaling. Thus, the possible mechanisms underlying fat reduction by flavonoids are yet to be further studied in C. elegans.
Flavonoids are believed to possess various potential activities, such as antioxidant, antimicrobial, anti-inflammatory, anti-allergic, anti-aging, cardioprotective, anti-diabetic, and anti-obesity activities.19,20,26,27,43 The relationships between their chemical structures and the activities have been extensively studied. It has been reported that quercetin and kaempferol could reduce fat accumulation in C. elegans.44 However, to our knowledge, this is the first study on the relationships between flavonoid chemical structures and the effects on fat regulation in C. elegans. In this study, six dietary flavonoids share a backbone of 5,7-dihydroxylflavone and possess the differences in 3-OH of the C ring and/or the varying substitution of the hydroxyl group in the B ring (Fig. 1). Numerous studies have attributed the possible health benefits of flavonoids to their antioxidant activities19,20,27 and the arrangement of hydroxyl groups correlates reasonably to the antioxidant activity of flavonoids.27 Although A ring substitution correlates little with antioxidant activity, the 5,7-dihydroxyl arrangement may contribute to antioxidant action.26,27 Consistent with the notion, we found that all six tested flavonoids significantly reduced worm fat storage at a dose of 100 μM (Fig. 2), suggesting the contributions of 5,7-dihydroxylation in the A ring to flavonoid-induced fat reduction. Furthermore, supporting the significant relationship of the 3′,4′-catechol group with antioxidant activity,26,27 only luteolin (possessing 3′,4′-OH in the B ring) promotes central serotonin signaling to induce worm fat loss (Fig. 3 and 4). Interestingly, similar to luteolin, quercetin (possessing 3′,4′-OH in the B ring and 3-OH in the C ring) also elevated tph-1 expression but failed to activate the downstream signaling of serotonin, indicating that the presence of 3-OH in the C ring may compromise the contribution of 3′,4′-OH in the B ring to serotonin-mediated fat loss. Indeed, with respect to the influence of 3-OH in the C ring on antioxidant activity, there are some contradictory observations.26,27 Therefore, to a certain extent, luteolin induced fat loss may correlate with the structure–activity relationship of its antioxidant activity, a hypothesis that merits further investigation.
In this study, only luteolin presented a dose-dependent fat regulatory capability among all six tested flavonoids, which may not be solely attributed to the structure–activity relationship of its antioxidant activity. On the one hand, it is well known that the biological properties of flavonoids depend on their bioavailability and many aspects affect their bioavailability.45 Chemical and biochemical factors, such as glycosylation and acylation, influence the gut absorption of dietary flavonoids.46 Therefore, based on the similar structure and function, the other five flavonoids may also inhibit worm fat accumulation in a dose-dependent manner when they are at higher concentration levels than those used in this study, although they do not promote central serotonin signaling in C. elegans. On the other hand, quercetin and luteolin have been reported as potential antidepressant compounds because of their suppressive effect on monoamine oxidase, which is the main enzyme in the metabolism of the neurotransmitter serotonin.47,48 The reports imply that luteolin may elevate the central serotonin level in C. elegans through inhibiting serotonin catabolism. Moreover, RFX transcription factor DAF-19 has been reported to regulate tph-1 expression in ADF neurons through acting downstream in the Toll-interleukin receptor domain adaptor protein (TIR-1) pathway.37 Here, we found that daf-19 expression was elevated in tph-1 mutants and luteolin treatment increased daf-19 expression in N2 worms. The results suggest daf-19 is likely to be involved in luteolin-induced fat loss by promoting tph-1 expression and serotonin synthesis in ADF neurons, a hypothesis that merits further investigation.
In conclusion, we here demonstrate that six dietary flavonoids can successfully reduce fat storage in C. elegans and only luteolin promotes central serotonin signaling to induce fat loss. As shown in Fig. 6, luteolin treatment increases serotonin synthesis in ADF neurons and activates serotonin-related receptors MOD-1 and SER-6 and finally elevates lipolysis and fatty acid β-oxidation in C. elegans, suggesting new insights into elucidating the mechanism underlying fat regulation by luteolin. Given that the serotonergic changes in feeding alone cannot account for serotonin-mediated alterations in the fat content in mammals,15 the mechanism merits direct testing in experimental mammals and humans.
Conclusions
In our research, the results indicated that luteolin reduced C. elegans fat storage by promoting central serotonin signaling, suggesting new insights into elucidating the mechanism underlying fat regulation by luteolin.
Author contributions
Y.L., B.B., and J.L. conceived and designed the experiments. Y.L. and N.Y. performed the experiments. Y.L., L.W., and J.C. analyzed the data. Y.L. and J.L. wrote the manuscript.
Abbreviations
C. elegans
|
Caenorhabditis elegans
|
HFD | High-fat diet |
TPH | Tryptophan hydroxylase |
NGM | Nematode growth medium |
GFP | Green fluorescence protein |
Conflicts of interest
There are no conflicts of interest to declare.
Acknowledgements
We thank Professor Pingsheng Liu (State Key Laboratory of Biomacromolecules, Institute of Biophysics, Chinese Academy of Sciences) for providing the LIU1 (ldrIs1 [dhs-3p::dhs-3::GFP+unc-76(+)]) strain. This work was supported by the National Natural Science Foundation of China (31401204, U1532269 to B. B.; 31671485 to J. L.) and the Natural Science Foundation of Anhui Province (1408085QC48 to B. B.). Some C. elegans strains were provided by the CGC, which is funded by the NIH Office of Research Infrastructure Programs (P40 OD010440).
References
- G. S. Hotamisligil, Inflammation and metabolic disorders, Nature, 2006, 444, 860–867 CrossRef CAS PubMed
.
- S. Gesta, Y. H. Tseng and C. R. Kahn, Developmental origin of fat: tracking obesity to its source, Cell, 2007, 131, 242–256 CrossRef CAS
.
- L. H. Tecott, Serotonin and the Orchestration of Energy Balance, Cell Metab., 2007, 6, 352–361 CrossRef CAS PubMed
.
- M. H. Donovan and L. H. Tecott, Serotonin and the regulation of mammalian energy balance, Front. Neurosci., 2013, 7, 36 Search PubMed
.
- G. A. Lemieux and K. Ashrafi, Neural Regulatory Pathways of Feeding and Fat in Caenorhabditis elegans, Annu. Rev. Genet., 2015, 49, 413–438 CrossRef CAS PubMed
.
- D. J. Heal, S. C. Cheetham, M. R. Prow, K. F. Martin and W. R. Buckett, A comparison of the effects on central 5-HT function of sibutramine hydrochloride and other weight-modifying agents, Br. J. Pharmacol., 1998, 125, 201–308 CrossRef PubMed
.
- M. G. Silverstein-Metzler, C. A. Shively, T. B. Clarkson, S. E. Appt, J. J. Carr, S. B. Kritchevsky, S. R. Jones and T. C. Register, Sertraline inhibits increases in body fat and carbohydrate dysregulation in adult female cynomolgus monkeys, Psychoneuroendocrinology, 2016, 68, 29–38 CrossRef CAS PubMed
.
- P. A. Sargent, A. L. Sharpley, C. Williams, E. M. Goodall and P. J. Cowen, 5-HT2C receptor activation decreases appetite and body weight in obese subjects, Psychopharmacology, 1997, 133, 309–312 CrossRef CAS PubMed
.
- C. Yan, Y. Yang, K. Saito, P. Xu, C. Wang, A. O. Hinton Jr., X. Yan, Q. Wu, Q. Tong, J. K. Elmquist, M. Fukuda and Y. Xu, Meta-chlorophenylpiperazine enhances leptin sensitivity in diet-induced obese mice, Br. J. Pharmacol., 2015, 172, 3510–3521 CrossRef CAS PubMed
.
- L. H. Tecott, L. M. Sun, S. F. Akana, A. M. Strack, D. H. Lowenstein, M. F. Dallman and D. Julius, Eating disorder and epilepsy in mice lacking 5-HT2c serotonin receptors, Nature, 1995, 374, 542–546 CrossRef CAS PubMed
.
- K. Nonogaki, A. M. Strack, M. F. Dallman and L. H. Tecott, Leptin-independent hyperphagia and type 2 diabetes in mice with a mutated serotonin 5-HT2C receptor gene, Nat. Med., 1998, 4, 1152–1156 CrossRef CAS PubMed
.
- K. Nonogaki, L. Abdallah, E. H. Goulding, S. J. Bonasera and L. H. Tecott, Hyperactivity and reduced energy cost of physical activity in serotonin 5-HT(2C) receptor mutant mice, Diabetes, 2003, 52, 315–320 CrossRef CAS PubMed
.
- E. Colman, J. Golden, M. Roberts, A. Egan, J. Weaver and C. Rosebraugh, The FDA's assessment of two drugs for chronic weight management, N. Engl. J. Med., 2012, 367, 1577–1579 CrossRef CAS PubMed
.
-
K. Ashrafi, Obesity and the regulation of fat metabolism, WormBook, 2007, pp. 1–20, DOI:10.1895/wormbook.1.130.1
.
- S. Srinivasan, L. Sadegh, I. C. Elle, A. G. L. Christensen, N. J. Faergeman and K. Ashrafi, Serotonin Regulates C. elegans Fat and Feeding through Independent Molecular Mechanisms, Cell Metab., 2008, 7, 533–544 CrossRef CAS PubMed
.
- J. Y. Sze, M. Victor, C. Loer, Y. Shi and G. Ruvkun, Food and metabolic signalling defects in a Caenorhabditis elegans serotonin-synthesis mutant, Nature, 2000, 403, 560–564 CrossRef CAS PubMed
.
- T. Noble, J. Stieglitz and S. Srinivasan, An Integrated Serotonin and Octopamine Neuronal Circuit Directs the Release of an Endocrine Signal to Control C. elegans Body Fat, Cell Metab., 2013, 18, 672–684 CrossRef CAS PubMed
.
- L. Palamiuc, T. Noble, E. Witham, H. Ratanpal, M. Vaughan and S. Srinivasan, A tachykinin-like neuroendocrine signalling axis couples central serotonin action and
nutrient sensing with peripheral lipid metabolism, Nat. Commun., 2017, 8, 14237 CrossRef CAS PubMed
.
- J. A. Ross and C. M. Kasum, Dietary flavonoids: bioavailability, metabolic effects, and safety, Annu. Rev. Nutr., 2002, 22, 19–34 CrossRef CAS PubMed
.
- D. Gentile, M. Fornai, C. Pellegrini, R. Colucci, C. Blandizzi and L. Antonioli, Dietary flavonoids as a potential intervention to improve redox balance in obesity and related co-morbidities: a review, Nutr. Res. Rev., 2018, 31, 239–247 CrossRef CAS PubMed
.
- N. Xu, L. Zhang, J. Dong, X. Zhang, Y. G. Chen, B. Bao and J. Liu, Low-dose diet supplement of a natural flavonoid, luteolin, ameliorates diet-induced obesity and insulin resistance in mice, Mol. Nutr. Food Res., 2014, 58, 1258–1268 CrossRef CAS PubMed
.
- J. Dong, X. Zhang, L. Zhang, H. X. Bian, N. Xu, B. Bao and J. Liu, Quercetin reduces obesity-associated ATM infiltration and inflammation in mice: a mechanism including AMPKalpha1/SIRT1, J. Lipid Res., 2014, 55, 363–374 CrossRef CAS PubMed
.
- D. Gentile, M. Fornai, R. Colucci, C. Pellegrini, E. Tirotta, L. Benvenuti, C. Segnani, C. Ippolito, E. Duranti, A. Virdis, S. Carpi, P. Nieri, Z. H. Nemeth, L. Pistelli, N. Bernardini, C. Blandizzi and L. Antonioli, The flavonoid compound apigenin prevents colonic inflammation and motor dysfunctions associated with high fat diet-induced obesity, PLoS One, 2018, 13, e0195502 CrossRef
.
- Y. Zang, L. Zhang, K. Igarashi and C. Yu, The anti-obesity and anti-diabetic effects of kaempferol glycosides from unripe soybean leaves in high-fat-diet mice, Food Funct., 2015, 6, 834–841 RSC
.
- H. M. Su, L. N. Feng, X. D. Zheng and W. Chen, Myricetin protects against diet-induced obesity and ameliorates oxidative stress in C57BL/6 mice, J. Zhejiang Univ., Sci., B, 2016, 17, 437–446 CrossRef CAS PubMed
.
- K. E. Heim, A. R. Tagliaferro and D. J. Bobilya, Flavonoid antioxidants: chemistry, metabolism and structure-activity relationships, J. Nutr. Biochem., 2002, 13, 572–584 CrossRef CAS PubMed
.
- T.-y. Wang, Q. Li and K.-s. Bi, Bioactive flavonoids in medicinal plants: Structure, activity and biological fate, Asian J. Pharm. Sci., 2018, 13, 12–23 CrossRef
.
- J. H. Choi and J. W. Yun, Chrysin induces brown fat-like phenotype and enhances lipid metabolism in 3 T3-L1 adipocytes, Nutrition, 2016, 32, 1002–1010 CrossRef CAS PubMed
.
- S. Brenner, The genetics of Caenorhabditis elegans, Genetics, 1974, 77, 71–94 CAS
.
- E. J. O'Rourke, A. A. Soukas, C. E. Carr and G. Ruvkun, C. elegans Major Fats Are Stored in Vesicles Distinct from Lysosome-Related Organelles, Cell Metab., 2009, 10, 430–435 CrossRef
.
- H. Xiao, V. M. Hapiak, K. A. Smith, L. Lin, R. J. Hobson, J. Plenefisch and R. Komuniecki, SER-1, a Caenorhabditis elegans 5-HT2-like receptor, and a multi-PDZ domain containing protein (MPZ-1) interact in vulval muscle to facilitate serotonin-stimulated egg-laying, Dev. Biol., 2006, 298, 379–391 CrossRef CAS
.
- L. T. MacNeil, E. Watson, H. E. Arda, L. J. Zhu and A. J. Walhout, Diet-induced developmental acceleration independent of TOR and insulin in C. elegans, Cell, 2013, 153, 240–252 CrossRef CAS
.
- P. Zhang, H. Na, Z. Liu, S. Zhang, P. Xue, Y. Chen, J. Pu, G. Peng, X. Huang, F. Yang, Z. Xie, T. Xu, P. Xu, G. Ou, S. O. Zhang and P. Liu, Proteomic study and marker protein identification of Caenorhabditis elegans lipid droplets, Mol. Cell. Proteomics, 2012, 11, 317–328 CrossRef
.
- T. P. Cushnie and A. J. Lamb, Antimicrobial activity of flavonoids, Int. J. Antimicrob. Agents, 2005, 26, 343–356 CrossRef CAS PubMed
.
- S. Srinivasan, Regulation of Body Fat in C. elegans, Annu. Rev. Physiol., 2015, 77, 161–178 CrossRef CAS PubMed
.
- C. M. Dempsey, S. M. Mackenzie, A. Gargus, G. Blanco and J. Y. Sze, Serotonin (5HT), fluoxetine, imipramine and dopamine target distinct 5HT receptor signaling to modulate Caenorhabditis elegans egg-laying behavior, Genetics, 2005, 169, 1425–1436 CrossRef CAS
.
- Y. Xie, M. Moussaif, S. Choi, L. Xu and J. Y. Sze, RFX transcription factor DAF-19 regulates 5-HT and innate immune responses to pathogenic bacteria in Caenorhabditis elegans, PLoS Genet., 2013, 9, e1003324 CrossRef CAS PubMed
.
- A. C. Puhl, A. Bernardes, R. L. Silveira, J. Yuan, J. L. Campos, D. M. Saidemberg, M. S. Palma, A. Cvoro, S. D. Ayers, P. Webb, P. S. Reinach, M. S. Skaf and I. Polikarpov, Mode of peroxisome proliferator-activated receptor gamma activation by luteolin, Mol. Pharmacol., 2012, 81, 788–799 CrossRef CAS PubMed
.
- A. Mosqueda-Solis, A. Lasa, S. Gomez-Zorita, I. Eseberri, C. Pico and M. P. Portillo, Screening of potential anti-adipogenic effects of phenolic compounds showing different chemical structure in 3 T3-L1 preadipocytes, Food Funct., 2017, 8, 3576–3586 RSC
.
- X. Zhang, Q. X. Zhang, X. Wang, L. Zhang, W. Qu, B. Bao, C. A. Liu and J. Liu, Dietary luteolin activates browning and thermogenesis in mice through an AMPK/PGC1[alpha] pathway-mediated mechanism, Int. J. Obes., 2016, 40, 1841–1849 CrossRef CAS PubMed
.
- C. J. Kuo, M. Hansen and E. Troemel, Autophagy and innate immunity: Insights from invertebrate model organisms, Autophagy, 2018, 14, 233–242 CrossRef CAS PubMed
.
- M. J. Angilletta Jr., J. P. Youngblood, L. K. Neel and J. M. VandenBrooks, The neuroscience of adaptive thermoregulation, Neurosci. Lett., 2019, 692, 127–136 CrossRef PubMed
.
- M. Kawai, T. Hirano, S. Higa, J. Arimitsu, M. Maruta, Y. Kuwahara, T. Ohkawara, K. Hagihara, T. Yamadori, Y. Shima, A. Ogata, I. Kawase and T. Tanaka, Flavonoids and related compounds as anti-allergic substances, Allergol. Int., 2007, 56, 113–123 CrossRef CAS
.
- S. Bhattacharya, K. B. Christensen, L. C. Olsen, L. P. Christensen, K. Grevsen, N. J. Faergeman, K. Kristiansen, J. F. Young and N. Oksbjerg, Bioactive components from flowers of Sambucus nigra L. increase glucose uptake in primary porcine myotube cultures and reduce fat accumulation in Caenorhabditis elegans, J. Agric. Food Chem., 2013, 61, 11033–11040 CrossRef CAS
.
- A. Scalbert and G. Williamson, Dietary intake and bioavailability of polyphenols, J. Nutr., 2000, 130, 2073s–2085s CrossRef CAS PubMed
.
- C. Manach, A. Scalbert, C. Morand, C. Remesy and L. Jimenez, Polyphenols: food sources and bioavailability, Am. J. Clin. Nutr., 2004, 79, 727–747 CrossRef CAS PubMed
.
- Y. Bandaruk, R. Mukai and J. Terao, Cellular uptake of quercetin and luteolin and their effects on monoamine oxidase-A in human neuroblastoma SH-SY5Y cells, Toxicol. Rep., 2014, 1, 639–649 CrossRef CAS PubMed
.
- B. K. Vazhayil, S. S. Rajagopal, T. Thangavelu, G. Swaminathan and E. Rajagounder, Neuroprotective effect of Clerodendrum serratum Linn. leaves extract against acute restraint stress-induced depressive-like behavioral symptoms in adult mice, Indian J. Pharmacol., 2017, 49, 34–41 CAS
.
Footnote |
† Electronic supplementary information (ESI) available. See DOI: 10.1039/c9fo02095k |
|
This journal is © The Royal Society of Chemistry 2020 |
Click here to see how this site uses Cookies. View our privacy policy here.