DOI:
10.1039/C9FO02234A
(Paper)
Food Funct., 2020,
11, 318-327
(−)-Epicatechin administration protects kidneys against modifications induced by short-term L-NAME treatment in rats
Received
24th September 2019
, Accepted 31st October 2019
First published on 5th November 2019
Abstract
The aim of this work was to evaluate the protective effects of (−)-epicatechin on the kidneys of NO-deprived rats. Male Sprague Dawley rats were divided into three groups: control (C), receiving water and standard diet; L-NAME (L), receiving a solution of N(ω)-nitro-L-arginine methyl ester (L-NAME) (360 mg l−1 in water) as a beverage and standard diet; and L-NAME-(−)-epicatechin (LE), receiving L-NAME solution as a beverage and standard diet supplemented with (−)-epicatechin (4 g kg−1 diet). The L-group showed altered kidney function parameters, evaluated based on plasma urea and creatinine. In parallel, kidney oxidative stress markers, i.e. superoxide anion production, malondialdehyde content, and 3-nitrotyrosine protein adducts, were significantly increased in the L group. In addition, L-NAME treatment induced modifications in kidney NO bioavailability determinants: increased expression of NOX subunits (p47phox, gp91phox, NOXO1, and NOX4) and lowered NOS activity. (−)-Epicatechin administration restored kidney function parameters, oxidative stress markers, expression of p47phox, gp91phox, and NOX4 and NOS activity to control values. These results indicate that (−)-epicatechin can mitigate NO-mediated impairment of kidney function, in part due to its capacity to modulate NOXs, NOSs, and consequently oxidative stress, and NO bioavailability.
Introduction
Nitric oxide (NO) deficiency in the kidney is associated with renal alterations, e.g. glomerular and tubulointerstitial injury and proteinuria, all of which lead to chronic kidney disease.1 NO bioavailability in cells depends on its enzymatic production by NO synthase (NOS) enzymes, and on NO degradation, mainly by reaction with superoxide anions.2 NADPH oxidase (NOX) enzymes are major superoxide anion sources,3 with the isoforms NOX1, NOX2 and NOX4 expressed in the kidney.4 An increase in kidney superoxide anion production is associated with oxidative stress which contributes in altering its hemodynamic and excretory functions, leading to sodium retention and the development of hypertension.5,6 Thus, the interplay between NO and superoxide anion bioavailabilities can be relevant to pathologies involving kidney oxidative stress and altered redox signaling.
The bioactivity of compounds present in edible fruits and vegetables appears to be related, to some extent, to their capacity to provide protection against oxidative stress and regulate redox signaling in organs and tissues. The protective effects of the flavanol (−)-epicatechin against several metabolic diseases have been attributed to its capacity to improve NO bioavailability.7–10 The decrease in NOX-derived superoxide anion production by (−)-epicatechin, which was observed to occur in different tissues, also contributes to the increase in NO bioavailability.8,9,11
In this study, we aimed to assess the protective effects of (−)-epicatechin administration on oxidative stress and the determinants of NO bioavailability in the renal cortex of N(ω)-nitro-L-arginine methyl ester (L-NAME)-treated rats. The obtained results indicate that (−)-epicatechin can mitigate NO-mediated impairment of kidney function, in part due to its capacity to modulate NOXs and NOSs, which results in the prevention of oxidative stress and improvement of NO bioavailability.
Materials & methods
Materials
Primary antibodies for inducible NOS (iNOS) (#7271), p47phox (#7660), endothelial NOS (eNOS) (#654), neuronal NOS (nNOS) (#648), gp91phox (#5826), manganese-SOD (Mn-SOD) (#133134), glutathione peroxidase (GPx) (#133160), 3-nitrotyrosine (#32731), NOX4 (21860), and β-actin (#47778) and secondary antibodies rabbit anti-goat IgG-HRP (#2768), mouse anti-rabbit IgG-HRP (#2357) and goat anti-mouse IgG-HRP (#2005) were from Santa Cruz Biotechnology (Santa Cruz, CA, USA). The primary antibody for copper/zinc-SOD (Cu/Zn-SOD) (AB1237) was from Chemicon International (Temecula, CA, USA), the primary antibody for p-eNOS Ser(1177) (#9570) was from Cell Signaling Technology (Boston, MA, USA), and the primary antibodies for catalase (ab52477), NOX1 (ab131088), NADPH oxidase organizer 1 (NOXO1) (ab34761) and β-tubulin (ab131205) were from Abcam Inc. (Cambridge, MA, USA). Bovine serum albumin, butylhydroxytoluene, (−)-epicatechin (purity ≥90%, HPLC), glucose-6-phosphate, glutathione reductase, heparin, L-NAME, 2-thiobarbituric acid (TBA), N,N′-dimethyl-9,9′-biacridinium dinitrate (lucigenin), NADPH, reduced glutathione, and superoxide dismutase (SOD) were from Sigma Chemical Co. (St Louis, MO, USA). The commercial rat chow was from Gepsa Feeds (Buenos Aires, Argentina). [14C]-Arginine was from PerkinElmer Life and Analytical Sciences (Boston, MA, USA).
Animals, diets and experimental design
All procedures were in agreement with the standards for the care of laboratory animals as outlined in the National Institutes of Health Guide for the Care and Use of Laboratory Animals (NIH Pub. No. 85-23, Revised 1996) and were approved by the Committee for Animals Care and Use, School of Pharmacy and Biochemistry, University of Buenos Aires (EXP-FYB No. 0069322). Male Sprague Dawley rats were housed under conditions of controlled temperature (21–25 °C) and humidity, with a 12 h light/dark cycle. The rats were randomly divided into the following experimental groups: one group receiving ad libitum tap water and standard rat chow diet (control (C) group); a second group receiving ad libitum an L-NAME solution (360 mg l−1 in water) and standard rat chow diet (L-NAME (L) group); and a third group receiving ad libitum an L-NAME solution (360 mg l−1 in water) in water and standard rat chow diet supplemented with (−)-epicatechin (4 g kg−1 diet) (L-NAME-(−)-epicatechin (LE) group). The dose of (−)-epicatechin was selected based on the need to counteract the challenge imposed by the acute L-NAME administration. It was the same dose used in our previous studies11–13 and is in the range used in other experimental conditions in mice and rats.14–17
Food intake and beverage consumption were measured daily by the amount of food (g) and volume of beverage (ml) consumed. After 4 days on the respective treatments, the animals were weighed and euthanized with CO2. Blood was collected from the abdominal aorta into heparinized tubes; plasma was obtained after centrifugation (600g, 15 min, 4 °C), and frozen at −80 °C. The kidneys were excised and the renal cortex was immediately isolated and processed for further analysis.
Plasma and protein determinations
Plasma creatinine and urea levels were measured using commercially available kits from Wiener Lab (Rosario, Argentina). Protein content was measured according to Lowry et al.18
Estimation of superoxide anion production
A portion of the renal cortex was homogenized in buffer containing 7.6 mM KH2PO4, 42.4 mM K2HPO4, 150 mM NaCl, pH: 7.4, and centrifuged at 600g for 20 min at 4 °C. The supernatants were centrifuged at 10
000g for 20 min at 4 °C to obtain mitochondria-free supernatants that were stored at −80 °C until analysis. Lucigenin-enhanced chemiluminescence was measured according to Li et al.19
Western blot analysis
A portion of the renal cortex was homogenized in lysis buffer (150 mM NaCl, 50 mM Trizma-HCl, 1% (v/v) NP-40, pH: 8.0) in the presence of protease and phosphatase inhibitors, and centrifuged at 600g for 20 min. The supernatant was collected and used as total homogenate. Protein expression was determined by western blot as previously described.20 The protein bands were normalized to β-actin or β-tubulin content.
Determinations of SOD, GPx and catalase activities
Enzyme activities were measured in the supernatants of renal cortex homogenates prepared in buffer containing 7.6 mM KH2PO4, 42.4 mM K2HPO4, 150 mM NaCl, pH: 7.4, and centrifuged at 600g for 20 min at 4 °C. SOD activity was spectrophotometrically determined following the inhibition of the formation of adrenochrome.21 The determinations were carried out in the absence (total SOD) or in the presence of 4 μM KCN to inhibit Cu/Zn-SOD. This KCN-inhibitable SOD activity is considered an estimate of Mn-SOD activity. Cu/Zn-SOD activity was calculated as the difference between total and KCN-inhibitable SOD activities.21 GPx activity was determined spectrophotometrically following the enzymatic oxidation of NADPH according to Flohé and Günzler.22 Catalase activity was determined spectrophotometrically following the consumption of H2O2 at 240 nm.23,24
Determination of NOS activity
NOS activity was determined by incubating renal cortex slices in the presence of [14C] L-arginine.25 NOS activity was estimated by the amount of [14C] L-citrulline formed during 30 min, normalized to slice weight.
Determination of malondialdehyde (MDA) content
MDA content was measured by HPLC after derivatization with TBA in the supernatants of renal cortex homogenates prepared in buffer containing 7.6 mM KH2PO4, 42.4 mM K2HPO4, 150 mM NaCl, pH: 7.4, and centrifuged at 600g for 20 min at 4 °C, as previously described.12
Statistical analysis
The results are shown as mean ± SEM. Data were analyzed by ANOVA and Student's t test using StatView 5.0 (SAS Institute, Cary, NC, USA). A value of p < 0.05 was considered statistically significant.
Results
Effects of (−)-epicatechin on parameters of renal function in L-NAME treated rats
No differences were observed among the experimental groups in: (i) initial body weight (C = 235 ± 5 g; L = 235 ± 11 g, and LE = 254 ± 13 g); (ii) final body weight (C = 273 ± 7 g; L = 275 ± 7 g, and LE = 275 ± 9 g), and (iii) food and beverage intake (average for all groups: 16 ± 2 g d−1 and 28 ± 1 ml d−1, respectively). Similarly, there were no differences in kidney weight, and in the kidney/body weight ratio (Table 1). However, kidney function was altered as evidenced by the higher plasma concentration of urea (40%) and creatinine (42%) observed in the L group with respect to C. Plasma urea and creatinine concentrations were similar in LE and C groups (Table 1).
Table 1 Effects of (−)-epicatechin on body and kidney weight and on the parameters of renal function in L-NAME treated rats
|
C |
L |
LE |
Results are shown as mean ± SEM (n = 6–8). *p < 0.05 with respect to C, #p < 0.05 with respect to L. |
Kidney weight (g) |
1.81 ± 0.02 |
1.69 ± 0.07 |
1.76 ± 0.05 |
Kidney/body weight (×10−4) |
70 ± 3 |
60 ± 2 |
60 ± 1 |
Plasma urea (mg dl−1) |
22 ± 1 |
31 ± 1* |
21 ± 2# |
Plasma creatinine (mg dl−1) |
0.33 ± 0.02 |
0.47 ± 0.03* |
0.34 ± 0.05# |
Effects of (−)-epicatechin on parameters of superoxide anion metabolism in L-NAME treated rats
A significantly higher (2.4 fold) NADPH-dependent superoxide anion production was observed in the L group with respect to both C and LE groups (Fig. 1A). Compared to controls, in the L group we observed a higher expression of NOX4, NOX1 regulatory subunit NOXO1, and p47phox and gp91phox, the regulatory and constitutive subunits of NOX2, respectively. The LE group showed NOXO1 protein levels similar to the L group, while those of NOX4, p47phox and gp91phox were significantly lower than that in the L group (Fig. 1B–F). No differences were found in NOX1 expression among the three experimental groups (Fig. 1D).
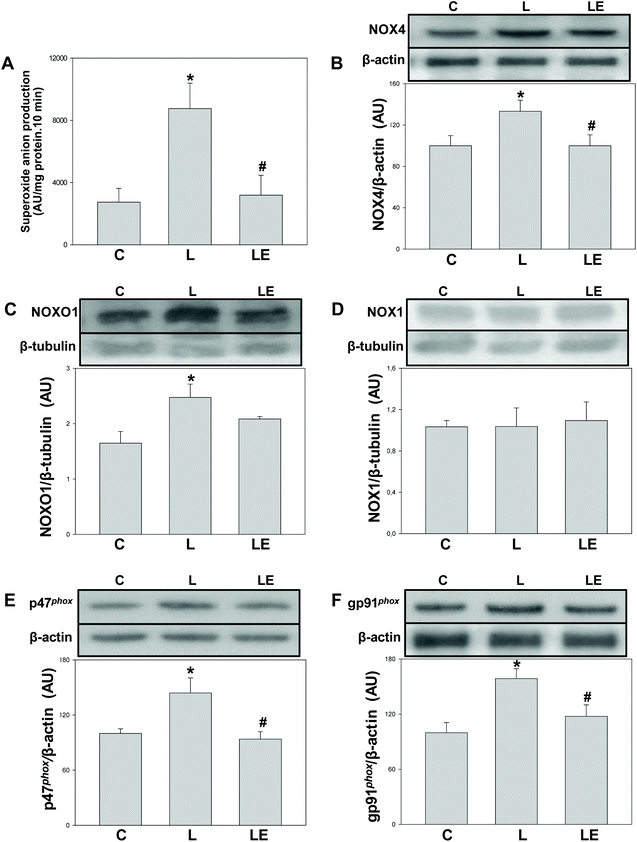 |
| Fig. 1 Effects of (−)-epicatechin supplementation on proteins involved in superoxide anion production in the renal cortex of L-NAME treated rats. SOD inhibitable-NADPH dependent lucigenin chemiluminescence (A); NOX4 (B), NOXO1 (C), NOX1 (D), p47phox (E) and gp91phox (F) protein levels were measured by western blot. In B–F, after quantification of the bands, values were corrected by β-tubulin or β-actin content and referred to control values. Results are shown as mean ± SEM (n = 6–8). *p < 0.05 with respect to C, #p < 0.05 with respect to L. | |
Effects of (−)-epicatechin on SOD, GPx and catalase expression and activity in L-NAME treated rats
Neither the expression nor the activity of Cu/Zn-SOD was affected by the treatments (Fig. 2A and B). Mn-SOD and GPx expression and activity were significantly higher in the L group with respect to C and LE groups (Fig. 2C–F). While catalase expression was similar among groups, its activity was higher in L than that in C and LE rats (Fig. 2G and H).
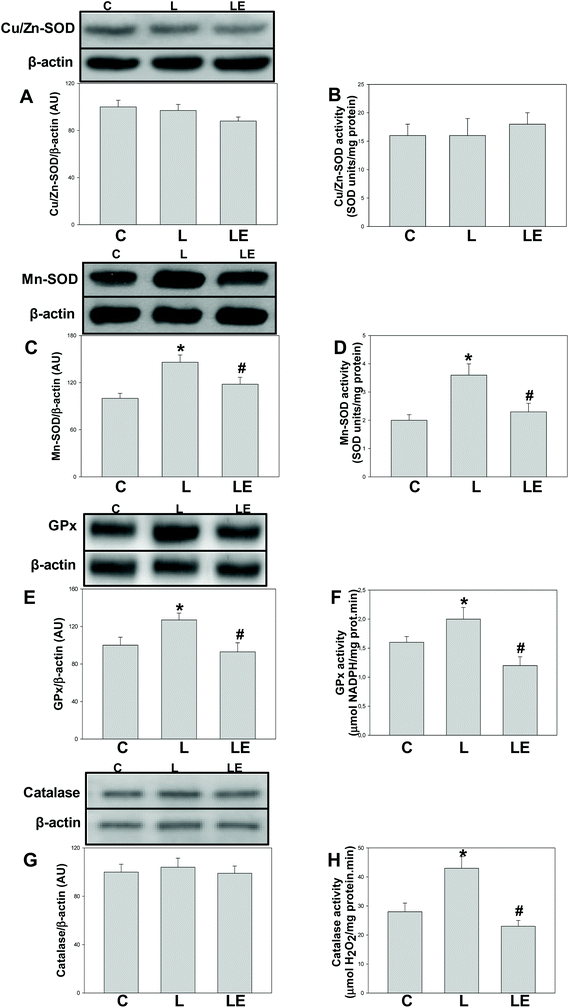 |
| Fig. 2 Effects of (−)-epicatechin supplementation on the expression and activity of enzymes involved in the degradation of superoxide anions/hydrogen peroxide in the renal cortex of L-NAME treated rats. Cu/Zn-SOD expression (A) and activity (B); Mn-SOD expression (C) and activity (D); GPx expression (E) and activity (F); and catalase expression (G) and activity (H). In A, C, E and G, after quantification of the bands, values were corrected by β-actin content and referred to control values. Results are shown as mean ± SEM (n = 6–8). *p < 0.05 with respect to C, #p < 0.05 with respect to L. | |
Effects of (−)-epicatechin on NO production-related parameters in L-NAME treated rats
A significant decrease in NOS activity was observed in the L group compared to C and LE groups (Fig. 3A). No differences in the expressions of iNOS and eNOS were observed among the three experimental groups (Fig. 3B and C). In the L group, nNOS expression was higher compared to C and LE groups (Fig. 3D). Similar levels of the activating Ser(1177) phosphorylation of eNOS were found in all the experimental groups (Fig. 3E).
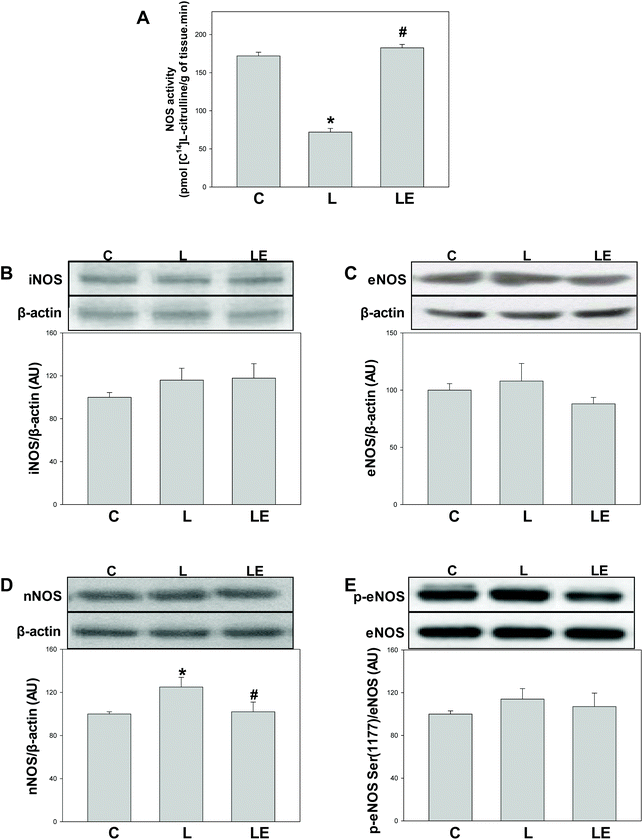 |
| Fig. 3 Effect of (−)-epicatechin supplementation on NOS activity and expression and on eNOS phosphorylation in the renal cortex of L-NAME treated rats. NOS activity (A) and iNOS (B), eNOS (C), nNOS (D) p (Ser 1177)-eNOS and eNOS (E) protein levels were measured by western blot. After quantification of the band values for iNOS, eNOS and nNOS, values were corrected by β-actin content, and p(Ser 1177)-eNOS by total eNOS content. Data were referred to control values. Results are shown as mean ± SEM (n = 4–6). *p < 0.05 with respect to C, #p < 0.05 with respect to L. | |
Effects of (−)-epicatechin on MDA content and levels of 3-nitrotyrosine protein adducts in L-NAME-treated rats
MDA content and the levels of 3-nitrotyrosine protein adducts were significantly higher in the L group with respect to C (24% and 68%, respectively) and LE groups (21% and 41%, respectively) (Fig. 4A and B).
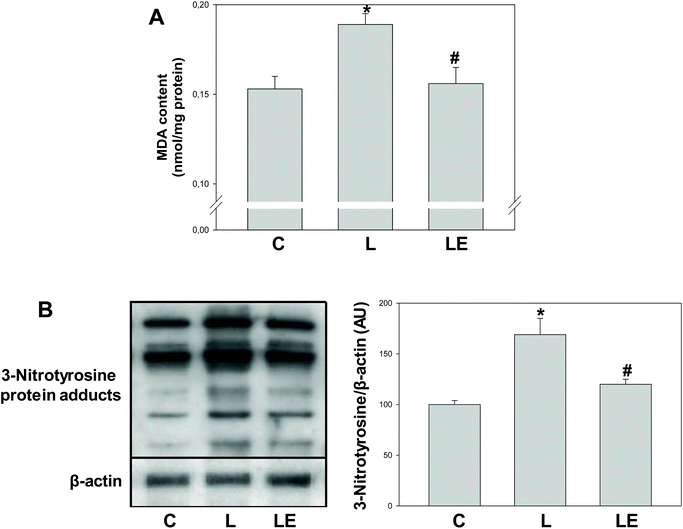 |
| Fig. 4 Effects of (−)-epicatechin supplementation on markers of oxidative and nitrosative stress in the renal cortex of L-NAME treated rats. MDA content was measured by HPLC (A) and levels of 3-nitrotyrosine protein adducts were measured by western blot and corrected by β-actin content and referred to control values (B). Results are shown as mean ± SEM (n = 4–6). *p < 0.05 with respect to C, #p < 0.05 with respect to L. | |
Discussion
Kidney function is supported in part by NO bioavailability, and the loss of the tissue capacity to maintain an adequate NO steady-state concentration leads to biochemical alterations that can ultimately compromise kidney function.1,26 Nutritional strategies can be designed to protect cells from an impaired capacity to synthesize NO, and/or against NO accelerated decomposition. Beyond the limitations imposed by the use of an animal model of renal damage, we show that the flavonoid (−)-epicatechin can mitigate L-NAME-mediated kidney dysfunction. This protection was associated with both the maintenance of adequate NO levels and a reduction of oxidant production.
The extension of the L-NAME treatment (4 days) was intended to address the changes due to early responses to NO deficiency. Longer periods of treatment generate a more complex scenario mixing short- and long-term effects. For example, 7–10 days of L-NAME administration led to renal fibrosis.27 Furthermore, a 4-week exposure to L-NAME was associated with proteinuria,28 chronic kidney inflammation and sustained activation of the sympathetic nervous and renin–angiotensin systems.29 Treatment of rats with L-NAME for 4 days led to incipient kidney dysfunction as indicated by the higher urea and creatinine levels in plasma. However, when (−)-epicatechin was present in the diet, those increases were not observed, suggesting a protective action of (−)-epicatechin on L-NAME-induced loss of kidney function. Such protection was similar to that observed in other organs/systems, i.e. normalization of blood pressure12 and cardiac functional parameters,11 in rats subjected to the same treatment. In addition, (−)-epicatechin provided protective actions in other models of kidney injury that also involved increased oxidant production, e.g. cisplatin nephropathy,30 and lipopolysaccharide administration31 or diminished NO bioavailability, e.g. long-term L-NAME administration,32 aging,33 and high-fructose diet.8
From a biochemical point of view, the capacity of kidney cells to generate oxidants via NOXs was increased by L-NAME, and returned to normal levels by (−)-epicatechin. The coordinated response of the different NOXs has been reported in other models of kidney dysfunction.20,31,34 Concerning the expression of NOX subunits, it was observed that (−)-epicatechin supplementation led to decreases only in the overexpression of the NOX2 organizer subunit and NOX4 catalytic subunit, stressing the specific activation mechanisms for each NOX isoform.35 These effects of (−)-epicatechin on select NOX subunits agree with those observed in other experimental models.8,20,31,36–38
The modifications in the ability of kidneys to generate oxidants could be dictating NO bioavailability, which in turn, would lead to alterations of kidney function. In addition, and confirmatory of the modulation of oxidant levels, we observed that when (−)-epicatechin was present in the diet, the condition of oxidative stress induced by L-NAME was not detected. In this context, the observed increases in Mn-SOD, GPx, and catalase activities, and GPx and catalase expressions could be the result of adaptive responses to a higher oxidant production triggered by L-NAME. The prevention of L-NAME-induced oxidative stress by (−)-epicatechin was also reflected in kidney lipid oxidation and protein nitrosylation. NO and superoxide anions are necessary for peroxynitrite production that leads to the formation of the 3-nitrotyrosine protein adduct as the hallmark product. Interestingly, in the present work, 3-nitrotyrosine protein adduct levels were increased even under NO deficit conditions, supporting the superoxide anion-driven nature of the process under in vivo conditions.39
As expected, L-NAME decreased the capacity of kidneys to synthesize NO which remained unaltered in rats treated with (−)-epicatechin. A similar trend was observed for NO plasma concentration, and for the activity of NOS in aorta and heart from rats under the same experimental conditions.11,12 Modifications in NOS activity cannot be ascribed to modifications in NOS expression, considering that both eNOS and iNOS expressions were not affected by the treatments. On the other hand, the expression of nNOS was high in kidneys from the L-NAME-treated rats, probably as an adaptation to the NO deficit. Indeed, the action of (−)-epicatechin seems to be upstream of nNOS regulation and driven by the reduced bioavailability of NO. In other experimental models, the effect of (−)-epicatechin increasing NO production by eNOS was associated with higher levels of activating phosphorylation of eNOS and lower levels of inhibiting phosphorylation.11,32,40 In the present study, the activating phosphorylation of eNOS was not modified by the treatments, indicating a higher relevance of protein synthesis over enzyme activation.
We can only speculate on the reason(s) for the (−)-epicatechin-mediated recovery of NO availability in tissues of LE rats. Under our experimental conditions, the displacement of N(ω)-nitro-L-arginine (L-NNA, the active metabolite of L-NAME) from the active site by (−)-epicatechin and/or any of its metabolites is not expected. In a previous work using the same experimental conditions,12 the L-NNA plasma levels were significantly higher than the IC50 for the binding to eNOS;41 meanwhile, the reached (−)-epicatechin plasma levels were markedly lower than the IC50 reported for eNOS inhibition by (−)-epicatechin.12,42 Therefore, alternative mechanisms through which (−)-epicatechin and/or its metabolites could directly or indirectly interfere in L-NNA interaction with NOS active sites should be considered. In addition, the observed effects of (−)-epicatechin on NO production cannot be ascribed to changes in the expression of the three types of NOS present in the kidney. This stresses the fact that both, the effects of L-NAME and the protection by (−)-epicatechin, are associated with alterations in the capacity of the enzymes to generate NO by affecting NOS active sites, promoting posttranslational modifications, and/or regulating substrate/cofactor levels.43–45
The present results support the capacity of (−)-epicatechin to protect kidney function when NO production is compromised and oxidant levels increased. The association between NOSs and NOXs, and (−)-epicatechin consumption could afford an explanation for the renal health benefits provided by the consumption of flavonoid-rich diets.
Abbreviations
eNOS | Endothelial nitric oxide synthase |
GPx | Glutathione peroxidase |
iNOS | Inducible nitric oxide synthase |
L-NAME |
L-NNA N(ω)-nitro-L-arginine methyl ester |
L-NNA |
N(ω)-Nitro-L-arginine |
MDA | Malondialdehyde |
NO | Nitric oxide |
NOS | Nitric oxide synthase |
nNOS | Neuronal nitric oxide synthase |
NOX | NADPH oxidase |
NOXO1 | NOX1 organizer |
SOD | Superoxide anion dismutase |
TBA | 2-Thiobarbituric acid |
Conflicts of interest
The authors declare no conflict of interest.
Acknowledgements
The authors would like to thank Dr Rosana Elesgaray from Cátedra de Fisiología, Departamento de Ciencias Biológicas, Facultad de Farmacia y Bioquímica, Universidad de Buenos Aires, for assistance with the measurement of NOS activity.
This work was supported by grants from University of Buenos Aires 20020170100586BA and 20020160100132BA and PIP-CONICET 11220170100585CO.
References
- C. Baylis, Nitric oxide synthase derangements and hypertension in kidney disease, Curr. Opin. Nephrol. Hypertens., 2012, 21, 1–6 CrossRef CAS.
- U. Forstermann and W. C. Sessa, Nitric oxide synthases: regulation and function, Eur. Heart J., 2012, 33, 829–837 CrossRef.
- B. Lassègue, A. San Martín and K. K. Griendling, Biochemistry, physiology, and pathophysiology of NADPH oxidases in the cardiovascular system, Circ. Res., 2012, 110, 1364–1390 CrossRef.
- M. Sedeek, R. Nasrallah, R. M. Touyz and R. L. Hébert, NADPH oxidases, reactive oxygen species, and the kidney: friend and foe, J. Am. Soc. Nephrol., 2013, 24, 1512–1518 CrossRef CAS PubMed.
- D. S. A. Majid and L. Kopkan, Nitric oxide and superoxide interactions in the kidney and their implication in the development of salt-sensitive hypertension, Clin. Exp. Pharmacol. Physiol., 2007, 34, 946–952 CrossRef CAS.
- L. Kopkan and L. Cervenka, Renal interactions of renin-angiotensin system, nitric oxide and superoxide anion: implications in the pathophysiology of salt-sensitivity and hypertension, Physiol. Res., 2009, 58, S55–S67 CAS.
- M. C. Litterio, M. A. Vazquez Prieto, A. M. Adamo, R. Elesgaray, P. I. Oteiza, M. Galleano and C. G. Fraga, (-)-Epicatechin reduces blood pressure increase in high-fructose-fed rats: effects on the determinants of nitric oxide bioavailability, J. Nutr. Biochem., 2015, 26, 745–751 CrossRef CAS PubMed.
- P. D. Prince, C. R. Lanzi, J. E. Toblli, R. Elesgaray, P. I. Oteiza, C. G. Fraga and M. Galleano, Dietary (-)-epicatechin mitigates oxidative stress, NO metabolism alterations, and inflammation in renal cortex from fructose-fed rats, Free Radicals Biol. Med., 2016, 90, 35–46 CrossRef CAS.
- V. Calabró, B. Piotrkowski, L. Fischerman, M. A. Vazquez Prieto, M. Galleano and C. G. Fraga, Modifications in nitric oxide and superoxide anion metabolism induced by fructose overload in rat heart are prevented by (-)-epicatechin, Food Funct., 2016, 7, 1876–1883 RSC.
- H. Schroeter, C. Heiss, J. Balzer, P. Kleinbongard, C. L. Keen, N. K. Hollenberg, H. Sies, C. Kwik-Uribe, H. H. Schmitz and M. Kelm, (-)-Epicatechin mediates beneficial effects of flavanol-rich cocoa on vascular function in humans, Proc. Natl. Acad. Sci. U. S. A., 2006, 103, 1024–1029 CrossRef CAS.
- B. Piotrkowski, V. Calabró, M. Galleano and C. G. Fraga, (-)-Epicatechin prevents alterations in the metabolism of superoxide anion and nitric oxide in the hearts of L-NAME-treated rats, Food Funct., 2015, 6, 155–161 RSC.
- M. C. Litterio, G. Jaggers, G. Sagdicoglu Celep, A. M. Adamo, M. A. Costa, P. I. Oteiza, C. G. Fraga and M. Galleano, Blood pressure-lowering effect of dietary (-)-epicatechin administration in L-NAME-treated rats is associated with restored nitric oxide levels, Free Radicals Biol. Med., 2012, 53, 1894–1902 CrossRef CAS.
- M. Galleano, I. Bernatova, A. Puzserova, P. Balis, N. Sestakova, O. Pechanova and C. G. Fraga, (-)-Epicatechin reduces blood pressure and improves vasorelaxation in spontaneously hypertensive rats by NO-mediated mechanism, IUBMB Life, 2013, 65, 710–715 CrossRef CAS.
- E. E. Mulvihill, J. M. Assini, B. G. Sutherland, A. S. DiMattia, M. Khami, J. B. Koppes, C. G. Sawyez, S. C. Whitman and M. W. Huff, Naringenin decreases progression of atherosclerosis by improving dyslipidemia in high-fat-fed low-density lipoprotein receptor-null mice, Arterioscler. Thromb. Vasc. Biol., 2010, 30, 742–748 CrossRef CAS.
- C. L. Chen, J. T. Chen, C. M. Liang, M. C. Tai, D. W. Lu and Y. H. Chen, Silibinin treatment prevents endotoxin-induced uveitis in rats in vivo and in vitro, PLoS One, 2017, 12, e0174971 CrossRef.
- J. Luo, L. Han, L. Liu, L. Gao, B. Xue, Y. Wang, S. Ou, M. Miller and X. Peng, Catechin supplemented in a FOS diet induces weight loss by altering cecal microbiota and gene expression of colonic epithelial cells, Food Funct., 2018, 9, 2962–2969 RSC.
- R. L. Castillo, E. A. Herrera, A. Gonzalez-Candia, M. Reyes-Farias, N. de la Jara, J. P. Peña and C. Carrasco-Pozo, Quercetin prevents diastolic dysfunction induced by a high-cholesterol diet: role of oxidative stress and bioenergetics in hyperglycemic rats, Oxid. Med. Cell. Longevity, 2018, 2018, 7239123 Search PubMed.
- O. H. Lowry, N. J. Rosebrough, A. L. Farr and R. J. Randall, Protein measurement with the Folin phenol reagent, J. Biol. Chem., 1951, 193, 265–275 CAS.
- J.-M. Li, S. Wheatcroft, L. M. Fan, M. T. Kearney and A. M. Shah, Opposing roles of p47phox in basal versus angiotensin II-stimulated alterations in vascular O2- production, vascular tone, and mitogen-activated protein kinase activation, Circulation, 2004, 109, 1307–1313 CrossRef CAS.
- P. D. Prince, C. Rodríguez Lanzi, C. G. Fraga and M. Galleano, Dietary (−)-epicatechin affects NF-κB activation and NADPH oxidases in the kidney cortex of high-fructose-fed rats, Food Funct., 2019, 10, 26–32 RSC.
- A. Boveris, C. G. Fraga, A. I. Varsavsky and O. R. Koch, Increased chemiluminescence and superoxide production in the liver of chronically ethanol-treated rats, Arch. Biochem. Biophys., 1983, 227, 534–541 CrossRef CAS.
- L. Flohé and W. A. Günzler, Assays of glutathione peroxidase, Methods Enzymol., 1984, 105, 114–121 Search PubMed.
- H. Aebi, Catalase in vitro, Methods Enzymol., 1984, 105, 121–126 CAS.
- B. Chance, H. Sies and A. Boveris, Hydroperoxide metabolism in mammalian organs, Physiol. Rev., 1979, 59, 527–605 CrossRef CAS.
- M. Romero, C. Caniffi, G. Bouchet, M. A. Costa, R. Elesgaray, C. Arranz and A. L. Tomat, Chronic treatment with atrial natriuretic peptide in spontaneously hypertensive rats: beneficial renal effects and sex differences, PLoS One, 2015, 10, e0120362 CrossRef.
- P. S. Modlinger, C. S. Wilcox and S. Aslam, Nitric oxide, oxidative stress, and progression of chronic renal failure, Semin. Nephrol., 2004, 24, 354–365 CrossRef CAS.
- J. Rincón, D. Correia, J. L. Arcaya, E. Finol, A. Fernández, M. Pérez, K. Yaguas, E. Talavera, M. Chávez, R. Summer and F. Romero, Role of angiotensin II type 1 receptor on renal NAD(P)H oxidase, oxidative stress and inflammation in nitric oxide inhibition induced-hypertension, Life Sci., 2015, 124, 81–90 CrossRef.
- M. D. Paredes, P. Romecín, N. M. Atucha, F. O'Valle, J. Castillo, M. C. Ortiz and J. García-Estañ, Moderate effect of flavonoids on vascular and renal function in spontaneously hypertensive rats, Nutrients, 2018, 10, 1107 CrossRef.
- M. Kashiwagi, M. Shinozaki, H. Hirakata, K. Tamaki, T. Hirano, M. Tokumoto, H. Goto, S. Okuda and M. Fujishima, Locally activated renin-angiotensin system associated with TGF-beta1 as a major factor for renal injury induced by chronic inhibition of nitric oxide synthase in rats, J. Am. Soc. Nephrol., 2000, 11, 616–624 CAS.
- K. Tanabe, Y. Tamura, M. A. Lanaspa, M. Miyazaki, N. Suzuki, W. Sato, Y. Maeshima, G. F. Schreiner, F. J. Villarreal, R. J. Johnson and T. Nakagawa, Epicatechin limits renal injury by mitochondrial protection in cisplatin nephropathy, Am. J. Physiol., 2012, 303, F1264–F1274 CAS.
- P. D. Prince, L. Fischerman, J. E. Toblli, C. G. Fraga and M. Galleano, LPS-induced renal inflammation is prevented by (−)-epicatechin in rats, Redox Biol., 2017, 11, 342–349 CrossRef CAS.
- M. Gómez-Guzmán, R. Jiménez, M. Sánchez, M. Romero, F. O'Valle, R. Lopez-Sepulveda, A. M. Quintela, P. Galindo, M. J. Zarzuelo, E. Bailón, E. Delpón, F. Perez-Vizcaino and J. Duarte, Chronic (-)-epicatechin improves vascular oxidative and inflammatory status but not hypertension in chronic nitric oxide-deficient rats, Br. J. Nutr., 2011, 106, 1337–1348 CrossRef.
- A. Moreno-Ulloa, L. Nogueira, A. Rodriguez, J. Barboza, M. C. Hogan, G. Ceballos, F. Villarreal and I. Ramirez-Sanchez, Recovery of indicators of mitochondrial biogenesis, oxidative stress, and aging with (-)-epicatechin in senile mice, J. Gerontol., Ser. A, 2015, 70, 1370–1378 CrossRef CAS.
- A. Manea, L. I. Tanase, M. Raicu and M. Simionescu, Transcriptional regulation of NADPH oxidase isoforms, Nox1 and Nox4, by nuclear factor-kappaB in human aortic smooth muscle cells, Biochem. Biophys. Res. Commun., 2010, 396, 901–907 CrossRef CAS.
- R. P. Brandes, N. Weissmann and K. Schröder, Nox family NADPH oxidases in mechano-transduction: mechanisms and consequences, Antioxid. Redox Signal., 2014, 20, 887–898 CrossRef CAS.
- A. Bettaieb, M. A. Vazquez Prieto, C. Rodriguez Lanzi, R. M. Miatello, F. G. Haj, C. G. Fraga and P. I. Oteiza, (-)-Epicatechin mitigates high-fructose-associated insulin resistance by modulating redox signaling and endoplasmic reticulum stress, Free Radicals Biol. Med., 2014, 72, 247–256 CrossRef CAS.
- A. Bettaieb, E. Cremonini, H. Kang, J. Kang, F. G. Haj and P. I. Oteiza, Anti-inflammatory actions of (-)-epicatechin in the adipose tissue of obese mice, Int. J. Biochem. Cell Biol., 2016, 81, 383–392 CrossRef CAS.
- M. Gómez-Guzmán, R. Jiménez, M. Sánchez, M. J. Zarzuelo, P. Galindo, A. M. Quintela, R. López-Sepúlveda, M. Romero, J. Tamargo, F. Vargas, F. Pérez-Vizcaíno and J. Duarte, Epicatechin lowers blood pressure, restores endothelial function, and decreases oxidative stress and endothelin-1 and NADPH oxidase activity in DOCA-salt hypertension, Free Radicals Biol. Med., 2012, 52, 70–79 CrossRef.
- J. Zielonka, M. Zielonka, L. VerPlank, G. Cheng, M. Hardy, O. Ouari, M. M. Ayhan, R. Podsiadły, A. Sikora, J. D. Lambeth and B. Kalyanaraman, Mitigation of NADPH oxidase 2 activity as a strategy to inhibit peroxynitrite formation, J. Biol. Chem., 2016, 291, 7029–7044 CrossRef CAS PubMed.
- I. Ramírez-Sánchez, A. Rodríguez, A. Moreno-Ulloa, G. Ceballos and F. Villarreal, (-)-Epicatechin-induced recovery of mitochondria from simulated diabetes: Potential role of endothelial nitric oxide synthase, Diabetes Vasc. Dis. Res., 2016, 13, 201–210 CrossRef.
- B. Mayer, M. Schmid, P. Klatt and K. Schmidt, Reversible inactivation of endothelial nitric oxide synthase by NG-nitro-L-arginine, FEBS Lett., 1993, 333, 203–206 CrossRef CAS.
- M. Chiesi and R. Schwaller, Inhibition of constitutive endothelial NO-synthase activity by tannin and quercetin, Biochem. Pharmacol., 1995, 49, 495–501 CrossRef CAS.
- I. Ramirez-Sanchez, L. Maya, G. Ceballos and F. Villarreal, (-)-Epicatechin activation of endothelial cell endothelial nitric oxide synthase, nitric oxide, and related signaling pathways, Hypertension, 2010, 55, 1398–1405 CrossRef CAS.
- L. Nogueira, I. Ramirez-Sanchez, G. A. Perkins, A. Murphy, P. R. Taub, G. Ceballos, F. J. Villarreal, M. C. Hogan and M. H. Malek, (-)-Epicatechin enhances fatigue resistance and oxidative capacity in mouse muscle, J. Physiol., 2011, 589, 4615–4631 CrossRef CAS.
- C. A. Schmitt and V. M. Dirsch, Modulation of endothelial nitric oxide by plant-derived products, Nitric Oxide, 2009, 21, 77–91 CrossRef CAS PubMed.
|
This journal is © The Royal Society of Chemistry 2020 |
Click here to see how this site uses Cookies. View our privacy policy here.