DOI:
10.1039/C9FO02285F
(Paper)
Food Funct., 2020,
11, 297-304
Strawberry (Fragaria × ananassa cv. Romina) methanolic extract promotes browning in 3T3-L1 cells
Received
30th September 2019
, Accepted 23rd December 2019
First published on 26th December 2019
Abstract
In recent years, the conversion of white adipocytes to brown-like adipocytes by pharmacological and dietary compounds has gained attention as an effective strategy to fight obesity. Strawberry bioactive compounds present several biological activities including antioxidant, anti-inflammatory, anti-cancer, anti-atherosclerotic and antiadipogenic properties. However, to the best of our knowledge, the possible role of strawberry bioactive compounds in white adipose tissue (WAT) browning has never been explored. Our results demonstrated that a strawberry methanolic extract (SE) significantly reduced 3T3-L1 pre-adipocytes differentiation, and down-regulated the mRNA expression of the adipogenic transcription factors CCAAT/enhancer-binding protein (C/REB- α) and peroxisome proliferation-activated receptor (PPAR-γ). It also down-regulated the mRNA expression of resistin and angiotensinogen, two genes considered as markers of white adipocytes, while increased the mRNA expression of pyruvate dehydrogenase lipoamide kinase isozyme 4 (PDK4) and uncoupling protein 1 (UCP1) which, conversely, are brown adipocyte-specific markers. Likewise, SE stimulated AMP-activated protein kinase (AMPKα), sirtuin 1 (Sirt1) and the peroxisome proliferator activated receptor gamma coactivator 1-alpha (PGC-1α), suggesting a possible increase in mitochondrial biogenesis. It also stimulated oxygen consumption rate and uncoupled respiration. Taken together, all these results suggest that SE induces brown fat-like phenotype in 3T3-L1 cells and may have potential therapeutic implications for treatment and/or prevention of obesity.
Introduction
Obesity, which has been associated with other chronic disorders, including metabolic syndrome, cardiovascular disease, diabetes, and some cancers,1–3 is one of the major epidemiological problems of the 21st century worldwide. Approximately 30% of the world's adult population is obese (about 2.1 billion) and more than 3 million people die every year as result of this illness.4 It is well known that obesity is caused by a sustained imbalance between energy intake and expenditure that leads to an expansion of adipose tissue mass resulting from increased adipocyte number and/or size.1,5
In mammals, according to historic classification, there are two main types of adipose tissue: white adipose tissue (WAT) and brown adipose tissue (BAT). WAT is specialized to store energy in form of triglycerides,2 while BAT is responsible for dissipating chemical energy through uncoupled respiration and heat production (non-shivering thermogenesis).1,2,6 WAT has also been recognized as a major endocrine organ, as it produces certain hormones and cytokines.1,7,8
From a morphological point of view, white adipocytes contain large unilocular lipid droplets and fewer mitochondria. In fact, in obese individuals, mitochondrial content, thermogenic activity and oxygen consumption are decreased. On the contrary, brown adipocytes contain multilocular lipid droplets and numerous mitochondria. They also express uncoupling protein-1 (UCP-1), which is localized to the inner membrane of mitochondria and it is responsible for proton gradient diminution, generating heat instead of ATP.9 In addition to the direct regulation of energy homeostasis, brown adipocytes also modulate glucose and lipid metabolism, independently of their effects on weight loss.3
Although white adipocytes and brown adipocytes originate from different lineage precursor cells, they can be interconverted.10 Recently, it has been demonstrated that brown adipocytes can also emerge in WAT depots in response to specific stimuli, such as cold exposure, nutrient intake and β-adrenergic agonists.1,3,5,6,10–12 Depending on the stimulus, these inducible brown-like adipocytes (brite or beige adipocytes) display the molecular, morphological and metabolic characteristics of either white or native brown adipocytes.2,3,6 Usually, there is a consensus that beige adipocytes contain multilocular lipid droplets, high mitochondrial content and high levels of UCP1 similar to BAT.9,13 They also express some beige adipocyte markers including Cbp/p300-interacting transactivator 1 (CITED1); transmembrane protein 26 (Tmem26), T-box transcriptional factor (TBX1) and tumor necrosis factor receptor superfamily member 9 (Tnfrsf9/CD137).9,14
Formation of brown-like adipocytes can be modulated by several transcriptional regulators including C/EBPα (CCAAT/enhancer-binding protein), PPARγ (peroxisome proliferation-activated receptor), PRDM16 and PGC1α (peroxisome proliferator-activated receptor gamma coactivator 1-alpha), as well as various secreted mediators, such as atrial and brain natriuretic peptides, fibroblast growth factor 21 (FGF21), PGC1α-dependent myokineirisin, norepinephrine and bone morphogenetic protein 7 (BMP7).1,15
Induction of the brown adipocyte-like phenotype within WAT (referred to as browning) has been shown to enhance energy expenditure and thermogenesis protecting against obesity and related metabolic complications in different animal models.3,6,16 Traditionally, studies on the physiology of adipocytes have focused on adipogenesis. However, in the recent years the search for bioactive compounds capable of activating brown adipocytes and trans-differentiation of WAT into BAT has gained popularity and it has been proposed as a promising strategy to counteract obesity.1,2,5,17–20
In that sense, several bioactive compounds, including curcumin, resveratrol, berberine, cyanidin-3-glucoside, quercetin, capsaicin, honokiol, genistein, curcumin, proanthocyanidins as well as cocoa and green tea polyphenols, have demonstrated not only to inhibit adipogenesis in different in vitro and in vivo experimental models8,21–24 but also to ameliorate obesity by increasing thermogenesis and promoting WAT browning.1,5,9,11,12,15,18,25–28 However, the mechanisms driving these browning effects are still not well elucidated.
Strawberry (Fragaria × ananassa) and in particular the Romina variety, is considered an important source of phytochemicals, especially flavonoids, hydrolysable tannins and phenolic acids.29 To the best of our knowledge, the possible role of strawberry bioactive compounds in WAT browning has never been explored. The aim of this study was to evaluate the effect of a SE on the induction of brown fat-like phenotype in 3T3-L1cells.
Materials & methods
Strawberry extract (SE) preparation
Strawberry fruits Fragaria × ananassa (cv. Romina) were collected at the ripening time in the experimental fields of the Agricultural Faculty of the Università Politecnica delle Marche, Italy. For the extract preparation, 10 g of fruits were added to 100 mL of the extraction solution consisting of methanol/MilliQ water/formic acid (80
:
20
:
0.1 v/v) and homogenized using an Ultraturrax T25 homogenizer (Janke & Kunkel, IKA Labortechnik). Then, the mixture was centrifuged at 2400g for 15 min in two sequential times and supernatants were filtered through a 0.45 μm Minisart filter (PBI International). For cellular treatment, the extract was further concentrated through a rotary evaporator and stored in aliquots at −80 °C.
Cells culture and differentiation
3T3-L1 mouse embryo fibroblasts cells were purchased from the American Type Culture Collection (ATCC® CL-173™). This cell line was chosen as experimental model because it is widely described, used, easy to culture and allows to obtain scientifically reproducible and reliable results. Cells were grown in pre-adipocytes expansion medium (DMEM, 10% bovine calf serum, 100 IU mL−1 penicillin and 100 μg mL−1 streptomycin) into 6-well plates (for Oil Red O Staining, western blotting or RNA extraction) or in Seahorse V7 cell plates (for mitochondrial function measurement). Two days after 100% confluence (Day 0), cells were induced to differentiate by using the differentiation medium (DMEM, 10% fetal bovine serum, 100 IU mL−1 penicillin and 100 μg mL−1 streptomycin, 0.5 mM IBMX, 1 μM dexamethasone and 1 μg mL−1 insulin). After further 48 h, this medium was replaced by the maintenance medium (DMEM, 10% fetal bovine serum, 100 IU mL−1 penicillin, 100 μg mL−1 streptomycin and 1 μg mL−1 insulin), which was changed every 48 h until Day 10. SE was added on Day 0 and maintained throughout the differentiation period.
Oil Red O staining
Cell differentiation was evaluated by the presence of lipid droplets as previously described.30 Briefly, cells were fixed with 4% formalin for 1 h at room temperature and stained with an Oil Red O solution (0.4% Oil Red O dye in isopropanol) for 20 min. Images were captured with a Leitz Fluovert FU (Leica Microsystems) microscope.
RNA extraction and quantitative real-time PCR analysis
Total RNA was extracted using a PureLink® RNA Mini Kit (Invitrogen, Carlsbad, CA, USA) according to the manufacturer's protocol, while the reverse transcription was carried out using a 5× All-In-One RT MasterMix kit (Applied Biological Materials) following the manufacturer's instructions. PCR reactions were performed in 20 μL volume using Eva Green 2X qPCR MasterMix kit (Applied Biological Materials). The sequences of primers used are shown in Table 1. Relative gene expression was calculated using the 2−ΔΔCT method and GADPH as reference gene. Values were expressed as relative mRNA level compared to control (fold change over control).
Table 1 Sequences of primers used for quantitative RT-PCR
Gene |
Sequences |
UCP1: Uncoupling protein 1; PDK4: pyruvate dehydrogenase kinase 4; GAPDH: glyceraldehyde-3-phosphate dehydrogenase. |
Resistin |
Forward: |
5′-CTCCCTGTTTCCAAATGC-3′ |
Reverse: |
5′-TGTCCAGTCTATCCTTGC-3′ |
Angiotensino-gen |
Forward: |
5′-GCAGGAGAGGAGGAACAG-3′ |
Reverse: |
5′-AGATGGCGAACAGGAAGG-3′ |
UCP1 |
Forward: |
5′-GATGGTGAACCCGACAACTT-3′ |
Reverse: |
5′-CTGAAACTCCGGCTGAGAAG-3′ |
PDK4 |
Forward: |
5′-TGTGATGTGGTAGCAGTAGTC-3′ |
Reverse: |
5′-ATGTGGTGAAGGTGTGAAGG-3′ |
GAPDH |
Forward: |
5′-AAGAAGGTGGTGAAGCAGGCATC-3′ |
Reverse: |
5′-GAAGGTGGAAGAGTGGGAGTTG-3′ |
Western blotting analysis
Cells were lysed in a buffer containing 20 mM Tris-HCl (pH 7.5), 0.9% NaCl, 0.2% Triton X-100 and 1% of the protease inhibitor cocktail (Sigma-Aldrich) and protein content was determined by Bradford assay. Equal amount of each sample were loaded and separated in a 10% acrylamide SDS/PAGE. After electrophoresis, samples were transferred to nitrocellulose membranes (Bio-Rad), using a Trans-Blot Turbo Transfer System (Bio-Rad Laboratories, Inc., Hercules, CA), then washed with PBS, blocked with 5% non-fat dry blocker (Bio-Rad) and incubated with the primary antibody at 4 °C overnight. All the polyclonal antibodies for the protein investigated [PGC1α: sc-13067; sirtuin 1 (Sirt1): sc-15404; AMP-activated protein kinase (AMPKα1/2): sc-74461; phosphorylated AMP-activated protein kinase (p-AMPKα1/2): sc-101630 and GAPDH: sc-25778] were purchased from Santa Cruz Biotechnology Inc. Next, membranes were washed with 5% Tween 20 in Tris-HCl buffered saline and incubated with the secondary anti-rabbit IgG-Peroxidase antibody (Sigma-Aldrich). Protein signals were detected by a Lycor C-Digit Blot Scanner and quantification of protein expression was made using the software Image Studio 3.1.
Measurement of the mitochondrial function
Oxygen consumption rate (OCR) and extracellular acidification rate (ECAR) were measured in real-time by using the Seahorse XF24 Analyzer (Seahorse Bioscience). Cells were seeded at a density of 3 × 104 cells per well and were induced to differentiate as described above. Prior to the analysis start, cells were incubated for 1 h in the assay medium containing 25 mM glucose, 2 mM glutamine, 1 mM sodium pyruvate, and without serum, (pH 7.4) in a non-CO2 incubator at 37 °C.
Mitochondrial respiration was evaluated by sequential injection of oligomycin (2.5 μg mL−1), carbonyl cyanide-4-(trifluoromethoxy) phenylhydrazone (FCCP, 2.5 μM) and rotenone/antimycin A (1 μM/10 μM). The maximal respiration capacity was calculated as the difference between OCR value after FCCP addition and OCR value after rotenone/antimycin A injection. Likewise, the proton leak was calculated as the difference between the OCR value after oligomycin addition and rotenone/antimycin A injection. ECAR was evaluated by sequential injection of rotenone (1 μM), glucose (30 mM) and 2-deoxy-D-glucose (2-DG) (100 mM). Glycolitic capacity was calculated as the difference between ECAR value after glucose addition and ECAR value after 2-DG injection. OCR was reported in picomoles per minute (pMoles per min) per 10 × 104 cells while ECAR was reported as milli-pH per minute (mpH min−1) per 10 × 104 cells. The best concentration for each inhibitor and uncoupler, as well as the optimal cells seeding density were determined in preliminary analyses. A minimum of five wells per treatment were utilized in any given experiment.
Statistical analysis
Statistical analyses were performed using STATISTICA software (Statsoft Inc., Tulsa, OK, USA). Data were subjected to one-way analysis of variance for mean comparison, and significant differences among different treatments were calculated according to HSD Tukey's multiple range test. Data are reported as mean ± standard deviation (SD). All the analyses were performed in triplicate.
Results and discussion
The SE used in the present work was previously characterized by our group.29 The main bioactive compounds identified were Pelargonidin 3-O-glucoside (29.30 ± 0.59 mg g−1) followed by Pelargonidin 3-malonylglucoside (5.20 ± 0.10 mg g−1), gallic acid derivatives (1.74 ± 0.12 mg g−1) and flavonols (0.26 ± 0.01 mg g−1). Its total antioxidant capacity was 168.25 ± 3.95 μmol Trolox equivalent per g and 35.51 ± 0.06 μmol Trolox equivalent per g, according to the methods Ferric-reducing antioxidant power (FRAP) and Trolox equivalent antioxidant capacity (TEAC), respectively.
In general, the Romina strawberry variety has values of total polyphenols, flavonoids, anthocyanins and total antioxidant capacity higher than those of other commercial varieties such as Alba, Adria or Sveva.31
SE inhibits 3T3-L1 pre-adipocytes differentiation and induces BAT specific genes in mature adipocytes
In order to evaluate the effects of SE on adipogenesis and phenotype reprogramming in mature adipocytes we used the well-established 3T3-L1 cells model. Differentiation of 3T3-L1 mouse embryo fibroblasts into mature adipocytes is one of the most common in vitro models used for understanding adipocyte biology and adipogenesis.32
Cells were treated with of 10, 50 and 100 μg mL−1 of SE during the entire differentiation period (from day 0 to Day 10). These concentrations were selected according to proliferation and viability tests previously achieved by our group.33 As shown in Fig. 1A, SE inhibited 3T3-L1 pre-adipocytes differentiation in a dose-dependent manner as demonstrated by the diminution of lipid content determined by the Oil Red O Staining. Likewise, SE supplementation decreased the mRNA expression of the main adipogenic transcription factors C/EBP-α and PPAR-γ when applied at 50 and 100 μg mL−1. At the lowest concentration (10 μg mL−1) no significant inhibition on the mRNA expression was observed compared to untreated cells (Fig. 1B). Additionally, SE treatment induced morphological changes in the adipocytes, making them smaller and filled with numerous lipid droplets (Fig. 1A).
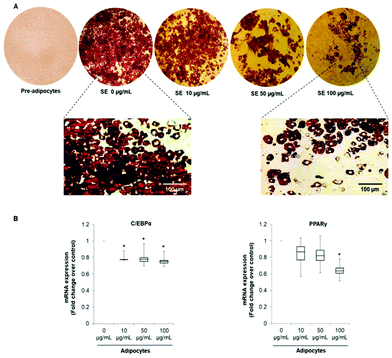 |
| Fig. 1 (A) Strawberry extract (SE) inhibits 3T3-L1 pre-adipocytes differentiation and change the distribution of intracellular lipid droplets. Cells were induced to differentiated in the presence of SE at the indicated concentrations. Representative images of three independent experiments are shown. (B) SE decreases mRNA expression of C/EBP-α and PPAR-γ. GADPH was amplified under the same PCR conditions for normalized quantitative data. Results are expressed as median, first quartiles, third quartiles, minimum and maximum values. Values are expressed as mean ± SD of three independent experiments (n = 3). *indicates statistical significance (p < 0.05) compared to control. The concentration 0 μg mL−1 corresponds to the control (fully differentiated adipocytes without SE treatment). C/EBPα: CCAAT/enhancer-binding protein, PPARγ: peroxisome proliferation-activated receptor. | |
The pattern of lipid accumulation observed in the treated adipocytes is distinctive of beige/brown adipocytes. The difference between WAT and BAT lipid droplets morphology derives from the different function of these tissues. White adipocytes usually contain a single large lipid droplet occupying most of the cytoplasm (unilocular) and a peripherally-located nucleus, while brown adipocytes are filled with multiples mall lipid droplets (multilocular) and a central nucleous.6,14 Hence, our observations suggest that SE induced brown fat-like phenotype in 3T3-L1 cells. Next, we determined if SE caused a shift in gene expression profile consistent with white adipocytes browning. For that purpose we evaluated the mRNA expression of white (Resistin and Angiotensinogen) and brown specific genes (PDK4 and UCP1) in fully differentiated 3T3-L1 adipocytes. Results of the qPCR assays showed that SE treatment significantly (p < 0.05) down-regulated the mRNA expression of resistin, but not of angiotensinogen, in a dose-dependent manner compared to control. At the same time, it stimulated mRNA expression of PDK4 and UCP1 (Fig. 2).
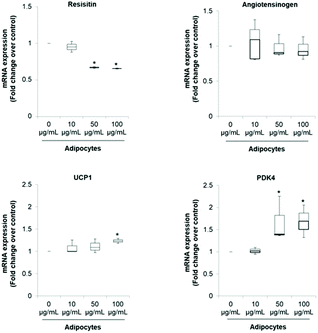 |
| Fig. 2 SE decreases mRNA expression of resistin and angiotensinogen while stimulates mRNA expression of UCP1 and PDK4. GADPH was amplified under the same PCR conditions for normalized quantitative data. Results are expressed as median, first quartiles, third quartiles, minimum and maximum values. Values are expressed as mean ± SD of three independent experiments (n = 3). *indicates statistical significance (p < 0.05) compared to control. The concentration 0 μg mL−1 corresponds to the control (fully differentiated adipocytes without SE treatment). UCP1: uncoupling protein 1; PDK4: pyruvate dehydrogenase kinase 4. | |
The significant up-regulation of brown-fat markers in treated adipocytes, suggests a possible conversion of white adipocytes into brown adipocytes. Similar results were observed in 3T3-L1 cells treated with berberine,5 chrysin,34 magnolol,10 cyaniding-3-glucoside,9 resveratrol15 and curcumin.27 Resistin is an adipokine that has been associated with obesity, metabolic syndrome and systemic inflammation.35 Hence, inhibition of resistin mRNA expression by SE may contribute to attenuate the pathogenesis of obesity-associated metabolic alterations. However, its precise role in insulin resistance and diabetes is still controversial.35,36 On the other hand, it has been demonstrated that PDK4 up-regulation in adipocytes contributes to the hypolipidemic effect of thiazolidinediones by modulating glyceroneogenesis.37 It has been recognized as a crucial mediator in redirecting glucose from oxidation towards triglyceride synthesis, favoring in this way fatty acid utilization as energy substrate for uncoupling mitochondria. In fact, PDK4 siRNA mediated knockdown prevents induction of fatty acid oxidation in beige adipocytes.38
Regarding UCP1 function, it is well established that it constitutes the main factor regulating non-shivering thermogenesis in BAT. It promotes heat generation by dissipating the proton gradient generated by electron transport which lead to uncoupling oxidative phosphorylation rather than ATP synthesis. This futile cycle stimulates substrate oxidation of fatty acids, resulting in increased energy expenditure.2,6,11 Hence, up-regulation of UCP1 mRNA is very robust evidence of WAT browning since respiratory uncoupling is essential to the distinctive phenotype of beige or brown adipose tissue.28 In that sense, food-derived thermogenic activators capable of inducing UCP1 and WAT manipulation to acquire BAT-like characteristics may be promising approaches to manage and/or prevent obesity and metabolic complications.
Enhanced mRNA levels of brown-fat markers was accompanied by stimulated protein expression of PGC1α, Sirt 1 and p-AMPKα (Fig. 3A–C). Overexpression of PGC1α in white adipocytes stimulates UCP1 expression and essential mitochondrial enzymes of the respiratory chain.2,6 PGC1α is considered as a master regulator of mitochondrial biogenesis, which is one of the main characteristics of brown adipocytes.17 On the contrary, it has been demonstrated that PGC1α deletion results in a thermogenic response diminution and decreased core body temperature after cold exposure in mice and increased susceptibility to insulin resistance in humans.6
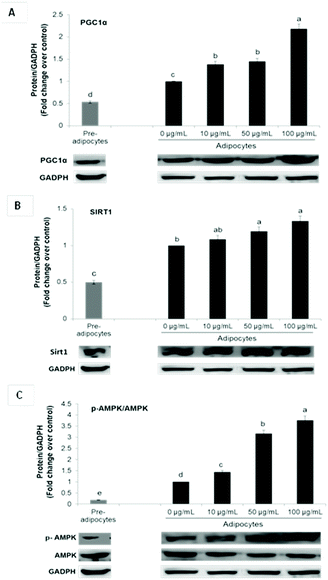 |
| Fig. 3 SE increases PGC1α (A), Sirt1 (B) and p-AMPK (C) expression in 3T3-L1 cells. The protein signals were detected by a Lycor C-Digit Blot Scanner and quantification was made using the software Image Studio 3. Values are expressed as mean ± SD of three independent experiments (n = 3) and normalized to the GADPH signal. Different superscript letters for each parameter in the chart bar indicate statistical significance (p < 0.05). PGC1α: Proliferator activated receptor gamma coactivator 1-alpha; Sirt1: sirtuin 1, p-AMPK: phosphorylated AMP-activated; GAPDH: glyceraldehyde-3-phosphate dehydrogenase. | |
Although PGC1α is not required for native BAT development, it is indispensable for WAT browning.6,11 Furthermore, it is fundamental for lipid and glucose metabolism under obese conditions.6
In conjunction with PGC1α, Sirt1 and AMPK signaling have been shown to play a critical role in energy homeostasis and obesity. Phosphorylation and consequent activation of AMPK induces ATP generating pathways and stimulates the expression of mitochondrial biogenesis signals via PGC1α up-regulation.11,31 On the other hand, Sirt1 cross-regulates AMPK and is capable of directly deacetylating PGC-1α, leading to its transcriptional activation. In adipose tissue, it is related not only with energy homeostasis but also with inflammation suppression. Activators of this histone/protein deacetylase improve insulin sensitivity and can repress inflammatory gene expression by suppression of JNK and NF-kB signaling.39
In addition to energy expenditure, AMPK activation during 3T3-L1 differentiation results in inhibition of the main adipogenic factors such as PPARγ and C/EBPα, leading to adipogenesis diminution.40 Although the role of AMPK in adipose tissue metabolism has been well characterized, its function in white adipocytes browning is very controversial.27 Nevertheless, it has been demonstrated that induction of in vitro WAT browning by some bioactive compounds including curcumin, ginsenoside Rb2, quercetin and resveratrol depends upon AMPK activation.3,11,12,27
In this study, UCP1 stimulation by SE may be attributed to its ability to upregulate PGC1α and p-AMPKα.
SE increases energetic metabolism in 3T3-L1 cells
To assess whether morphological transformations and genes profile variations in 3T3-L1 cells were accompanied by changes in energy metabolism, we evaluated OCR and ECAR as indicators of mitochondrial respiration and glycolysis, respectively. We also calculated the maximal respiratory capacity, the proton leak and the glycolytic capacity as reported in Fig. 4A and 5A, respectively.
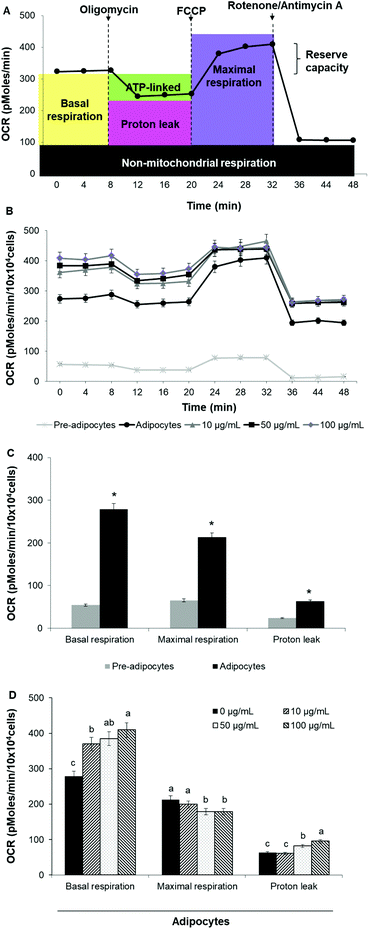 |
| Fig. 4 SE increases oxygen consumption rate and uncoupled respiration in 3T3-L1 cells. (A) Schematic representation of XF cell Mito stress test profile and the parameters that can be calculated, (B) XF cell Mito stress test profile in 3T3-L1 cells, (C) mitochondrial function in 3T3-L1 fibroblast vs. mature adipocytes, (D) mitochondrial function in adipocytes treated with different concentrations of SE. Values are expressed as mean ± SD of three independent experiments (n = 3). Different superscript letters for each parameter in the chart bar indicate statistical significance (p < 0.05). *indicates p < 0.05 compared to pre-adipocytes. OCR: Oxygen consumption rate; FCCP: carbonylcyanide-4-(trifluoromethoxy) phenylhydrazone. | |
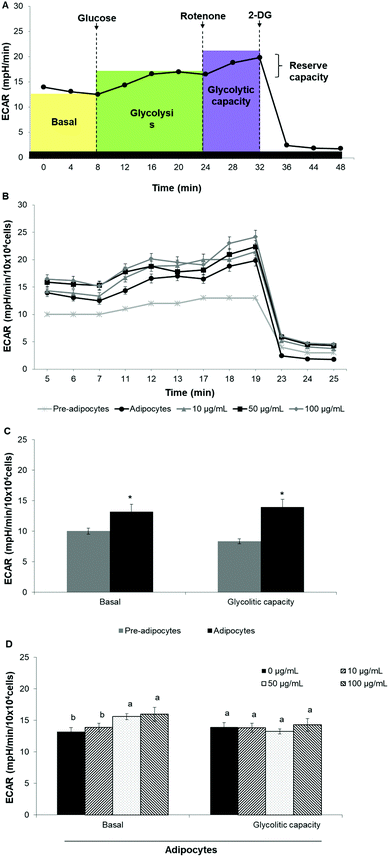 |
| Fig. 5 SE increases extracellular acidification rate in 3T3-L1 cells. (A) Schematic representation of XF cell Glycolysis stress test profile and the parameters that can be calculated, (B) XF cell Glycolysis stress test profile in 3T3-L1 cells, (C) basal glycolysis and glycolysis capacity in 3T3-L1 fibroblast vs. mature adipocytes, (D) basal glycolysis and glycolysis capacity in adipocytes treated with different concentrations of SE. Values are expressed as mean ± SD of three independent experiments (n = 3). Different superscript letters for each parameter in the chart bar indicate statistical significance (p < 0.05). *indicates p < 0.05 compared to pre-adipocytes. ECAR: Extracellular acidification rate; 2-DG: 2-deoxy-D-glucose. | |
Differentiation of 3T3-L1 pre-adipocytes into mature adipocytes led to an augmentation of all parameters related to mitochondrial respiration including basal respiration, maximum respiratory capacity and proton leak (Fig. 4B and C). Likewise, SE treatment significantly (p < 0.05) increased these indicators in a dose-dependent manner compared to un-treated cells (Fig. 4D). Contribution of proton leak to basal and maximal respiratory revealed greater mitochondrial uncoupling respiration in cells incubated with SE, which correspond to brown adipocytes characteristics.
Stimulation of energetic metabolism by certain natural compounds in 3T3-L1 cells has also been reported by Kang et al.,19 Aziz et al.28 and Lin et al.5 In addition, SE treatment significantly (p < 0.05) stimulated basal ECAR and glycolytic capacity although these effects were less pronounced than those in the OCR (Fig. 5B–D). Differentiation of 3T3-L1 pre-adipocytes into mature adipocytes as well as SE treatment increased both aerobic and anaerobic flux, as shown in Fig. 6.
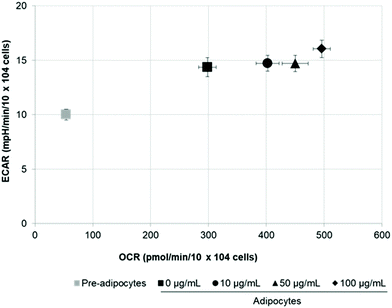 |
| Fig. 6 SE increases bioenergetic metabolism of 3T3-L1 cells. Values are expressed as mean ± SD of three independent experiments (n = 3). Different superscript letters for each parameter in the chart bar indicate statistical significance (p < 0.05). ECAR: Extracellular acidification rate; OCR: oxygen consumption rate. | |
Since mitochondrial number and activity are decreased in obese and diabetic patients and mitochondria play a significant role in energy generation via ATP production and thermogenesis, increasing energetic metabolism has been proposed as an effective strategy for obesity management.9
Conclusions
In this study, we established for the first time that SE is able to induce a brown-fat like phenotype in cultured white adipocytes by stimulating: (i) morphological transformation from large lipid droplets to multilocular smaller droplets; (ii) brown fat-specific genes and proteins such as Resistin, PDK4, UCP1 and PCG1α and (iii) bioenergetic metabolism. In addition, SE stimulated Sirt1 and p-AMPKα expression suggesting that this pathway can be implicated in the SE-mediated effects. Our results encourage further in vitro (on primary cell cultures) and in vivo (on animals) studies to determine the underlying mechanism of SE effects.
Conflicts of interest
There are no conflicts to declare.
Acknowledgements
Tamara Y. Forbes-Hernández is supported by a “Juan de la Cierva-Formación” post-doctoral contract.
References
- L. Wu, L. Zhang, B. Li, H. Jiang, Y. Duan, Z. Xie, L. Shuai, J. Li and J. Li, AMP-Activated Protein Kinase (AMPK) regulates energy metabolism through modulating thermogenesis in adipose tissue, Front. Physiol., 2018, 9, 122 CrossRef PubMed.
- H. E. Lee, G. Yang, S. Han, J. Lee, T. An, J. Jang and J. Y. Lee, Anti-obesity potential of Glycyrrhizauralensis and licochalcone A through induction of adipocyte browning, Biochem. Biophys. Res. Commun., 2018, 503, 2117–2123 CrossRef CAS PubMed.
- Y. Hong, Y. Lin, Q. Si, L. Yang, W. Dong and X. Gu, Ginsenoside Rb2 alleviates obesity by activation of brown fat and induction of browning of white fat, Front. Endocrinol., 2019, 10, 153 CrossRef PubMed.
- GBD 2017 Diet Collaborators, Health effects of dietary risks in 195 countries, 1990–2017: a systematic analysis for the Global Burden of Disease Study 2017, Lancet, 2019, 393, 1958–1972 CrossRef.
- Y. Lin, Y. Lee, Y. Lin and J. Lin, Berberine promotes beige adipogenic signatures of 3T3-L1 cells by regulating posttranscriptional events, Cells, 2019, 8, 632 CrossRef PubMed.
- A. Fenzl and F. W. Kiefer, Brown adipose tissue and thermogenesis, Horm. Mol. Biol. Clin. Invest., 2014, 19, 25–37 CAS.
- M. Lee, H. H. Lee, J. K. Lee, S. K. Ye, S. H. Kim and S. H. Sung, Antiadipogenic activity of compounds isolated from Idesiapolycarpa on 3T3-L1 cells, Bioorg. Med. Chem. Lett., 2013, 23, 3170–3174 CrossRef CAS PubMed.
- K. K. Li, C. L. Liu, H. T. Shiu, H. L. Wong, W. S. Siu, C. Zhang, X.Q. Han, C. X. Ye, P. C. Leung and C. H. Ko, Cocoa tea (Camellia ptilophylla) water extract inhibits adipocyte differentiation in mouse 3T3-L1 preadipocytes, Sci. Rep., 2016, 6, 20172 CrossRef CAS PubMed.
- T. Matsukawaa, M. O. Villareal, H. Motojimac and H. Isoda, Increasing cAMP levels of preadipocytes by cyanidin-3- glucoside treatment induces the formation of beige phenotypes in 3T3-L1 adipocytes, J. Nutr. Biochem., 2017, 40, 77–85 CrossRef PubMed.
- H. A. Parray, J. Lone, J. P. Park, J. W. Choi, J. W. Yun and J. P. Park, Magnolol promotes thermogenesis and attenuates oxidative stress in 3T3-L1 adipocytes, Nutrition, 2018, 50, 82–90 CrossRef CAS PubMed.
- J. H. Choi, C. S. Kim and R. Yu, Quercetin upregulates uncoupling protein 1 in white/brown adipose tissues through sympathetic stimulation, J. Obes. Metab. Syndr., 2018, 27, 102–109 CrossRef PubMed.
- J. S. Samuels, R. Shashidharamurthy and S. Rayalam, Novel anti-obesity effects of beer hops compound xanthohumol: role of AMPK signaling pathway, Nutr. Metab., 2018, 15, 42 CrossRef PubMed.
- J. H. Choi, N. J. Song, A. R. Lee, D. H. Lee, M. J. Seo, S. Kim, S. H. Chang, D. K. Yang, Y. J. Hwang, K. A. Hwang, T. S. Ha, U. J. Yun and K. W. Park, Oxyresveratrol increases energy expenditure through Foxo3a-mediated Ucp1 induction in high-fat-diet-induced obese mice, Int. J. Mol. Sci., 2019, 20, 26 CrossRef PubMed.
- Y. Unno, H. Yamamoto, S. Takatsuki, Y. Sato, T. Kuranaga, K. Yazawac, Y. Onoa and T. Wakimoto, Palmitoyl lactic acid induces adipogenesis and a brown fat-like phenotype in 3T3- L1 preadipocytes, Biochim. Biophys. Acta, Mol. Cell Biol. Lipids, 2018, 1863, 772–782 CrossRef CAS PubMed.
- S. Wang, X. Liang, Q. Yang, X. Fu, C. J. Rogers, M. Zhu, B. D. Rodgers, Q. Jiang, M. V. Dodson and M. Du, Resveratrol induces brown-like adipocyte formation in white fat through activation of AMP-activated protein kinase (AMPK), Int. J. Obes., 2015, 39, 967–976 CrossRef CAS PubMed.
- H. Lim, J. Park, H. L. Kim, J. W. Kang, M. Y. Jeong, D. H. Youn, Y. Jung, Y. Kim, H. J. Kim, K. S. Ahn, S. J. Kim, S. K. Choe, S. H. Hong and J. Y. Um, Chrysophanic acid suppresses adipogenesis and induces thermogenesis by activating AMP Activated Protein Kinase Alpha In vivo and In vitro, Front. Pharmacol., 2016, 7, 476 Search PubMed.
- M. Choi, S. Mukherjee, N. H. Kang, J. L. Barkat, H. A. Parray and J. W. Yun, L-rhamnose induces browning in 3T3-L1 white adipocytes and activates HIB1B brown adipocytes, IUBMB Life, 2018, 70, 563–573 CrossRef CAS PubMed.
- J. Lone and J. Yun, Honokiol exerts dual effects on browning and apoptosis of adipocytes, Pharmacol. Rep., 2017, 69, 1357–1365 CrossRef CAS PubMed.
- N. H. Kang, S. Mukherjee, T. Min, S. C. Kang and J. W. Yun, Trans-anethole ameliorates obesity via induction of browning in white adipocytes and activation of brown adipocytes, Biochimie, 2018, 151, 1–13 CrossRef CAS PubMed.
- T. Kusudo, M. Hashimoto, N. Kataoka, Y. Li, A. Nozaki and H. Yamashita, CREG1 promotes uncoupling protein 1 expression and brown adipogenesis in vitro, J. Biochem., 2019, 165, 47–55 CrossRef CAS PubMed.
- M. S. Lee, C. T. Kim, I. H. Kim and Y. Kim, Inhibitory effects of green tea catechin on the lipid accumulation in 3T3-L1 adipocytes, Phytother. Res., 2009, 23, 1088–1091 CrossRef CAS PubMed.
- A. Ejaz, D. Wu, P. Kwan and M. Meydani, Curcumin inhibits adipogenesis in 3T3-L1 adipocytes and angiogenesis and obesity in C57/BL mice, J. Nutr., 2009, 139, 919–925 CrossRef CAS PubMed.
- L. Tian, Z. Song, W. Shao, W. Du, L. Zhao, K. Zeng, B. Yang and T. Jin, Curcumin represses mouse 3T3-L1 cell adipogenic differentiation via inhibiting miR-17-5p and stimulating the Wnt signalling pathway effector Tcf7l2, Cell Death Dis., 2017, 8, 2559 CrossRef PubMed.
- S. Y. Leu, Y. C. Chen, Y. C. Tsai, Y. W. Hung, C. H. Hsu, Y. M. Lee and P. Y. Cheng, Raspberry ketone reduced lipid accumulation in 3T3-L1 cells and ovariectomy-induced obesity in wistar rats by regulating autophagy mechanisms, J. Agric. Food Chem., 2017, 65, 10907–10914 CrossRef CAS PubMed.
- R. K. Baboota, D. P. Singh, S. M. Sarma, J. Kaur, R. Sandhir, R. K. Boparai, K. K. Kondepudi and M. Bishnoi, Capsaicin induces “brite” phenotype in differentiating 3T3-L1 preadipocytes, PLoS One, 2014, 9, 103093 CrossRef PubMed.
- Z. Zhang, H. Zhang, B. Li, X. Meng, J. Wang, Y. Zhang, S. Yao, Q. Ma, L. Jin, J. Yang, W. Wang and G. Ning, Berberine activates thermogenesis in white and brown adipose tissue, Nat. Commun., 2014, 5, 5493 CrossRef CAS PubMed.
- J. Lone, J. H. Choi, S. W. Kim and J. W. Yun, Curcumin induces brown fat-like phenotype in 3T3-L1 and primary white adipocytes, J. Nutr. Biochem., 2016, 27, 193–202 CrossRef CAS PubMed.
- S. A. Aziz, L. A. Wakeling, S. Miwa, G. Alberdi, J. E. Hesketh and D. Ford, Metabolic programming of a beige adipocyte phenotype by genistein, Mol. Nutr. Food Res., 2017, 61, 1600574 CrossRef PubMed.
- T. Y. Forbes-Hernández, M. Gasparrini, S. Afrin, D. Cianciosi, A. M. González-Paramás, C. Santos-Buelga, B. Mezzetti, J. L. Quiles, M. Battino, F. Giampieri and S. Bompadre, Strawberry (cv. Romina) methanolic extract and anthocyanin-enriched fraction improve lipid profile and antioxidant status in HepG2 cells, Int. J. Mol. Sci., 2017, 18, 1149 CrossRef PubMed.
- J. F. Liu, Y. Ma, Y. Wang, Z. Y. Du, J. K. Shen and H. L. Peng, Reduction of lipid accumulation in HepG2 Cells by luteolin is associated with activation of AMPK and mitigation of oxidative stress, Phytother. Res., 2010, 25, 588–596 CrossRef PubMed.
- T. Y. Forbes-Hernández, F. Giampieri, M. Gasparrini, S. Afrin, L. Mazzoni, M. D. Cordero, B. Mezzetti, J. L. Quiles and M. Battino, Lipid accumulation in HepG2 cells is attenuated by strawberry extract through AMPK activation, Nutrients, 2017, 9, 621 CrossRef PubMed.
- S. Morrison and S. L. McGee, 3T3-L1 adipocytes display phenotypic characteristics of multiple adipocyte lineages, Adipocyte, 2015, 4, 295–302 CrossRef CAS PubMed.
- T. Y. Forbes-Hernández, S. Afrin, D. Cianciosi, P. P. Manna, J. Zhang, M. Gasparrini and P. Reboredo-Rodríguez, Strawberry extract attenuates oxidative stress in 3T3-L1 cells, J. Berry Res., 2018, 8, 193–203 Search PubMed.
- J. H. Choi and J. W. Yun, Chrysin induces brown fat–like phenotype and enhances lipid metabolism in 3T3-L1 adipocytes, Nutrition, 2016, 32, 1002–1010 CrossRef CAS PubMed.
- R. V. Cherneva, O. B. Georgiev, D. S. Petrova, T. L. Mondeshki, S. R. Ruseva, A. D. Cakova and V. I. Mitev, Resistin - the link between adipose tissue dysfunction and insulin resistance in patients with obstructive sleep apnea, J. Diabetes Metab. Disord., 2013, 12, 5 CrossRef PubMed.
- J. Beltowski, Adiponectin and resistin–new hormones of white adipose tissue, Med. Sci. Monit, 2003, 9, 55–61 Search PubMed.
- T. Cadoudal, E. Distel, S. Durant, F. Fouque, J. M. Blouin, M. Collinet, S. Bortoli, C. Forest and C. Benelli, Pyruvate dehydrogenase kinase 4: regulation by thiazolidinediones and implication in glyceroneogenesis in adipose tissue, Diabetes, 2008, 57, 2272–2279 CrossRef CAS PubMed.
- V. Barquissau, D. Beuzelin, D. F. Pisani, G. E. Beranger, A. Mairal, A. Montagner, B. Roussel, G. Tavernier, M. A. Marques, C. Moro, H. Guillou, E. Z. Amri and D. Langin, White-to-brite conversion in human adipocytes promotes metabolic reprogramming towards fatty acid anabolic and catabolic pathways, Mol. Metab., 2016, 5, 352–365 CrossRef CAS PubMed.
- K. W. Cho and C. N. Lumeng, SirT1: A guardian at the gates of adipose tissue inflammation, Diabetes, 2011, 60(12), 3100–3102 CrossRef CAS PubMed.
- H. L. Kim, J. Park, Y. Jung, K. S. Ahn and J. Y. Um, Platycodin D, A novel activator of AMP-activated protein kinase, attenuates obesity in db/db mice via regulation of adipogenesis and thermogenesis, Phytomedicine, 2019, 52, 254–263 CrossRef CAS PubMed.
Footnote |
† These authors have contributed equally. |
|
This journal is © The Royal Society of Chemistry 2020 |
Click here to see how this site uses Cookies. View our privacy policy here.