DOI:
10.1039/C9FO02491C
(Paper)
Food Funct., 2020,
11, 1155-1164
Calcium relieves fluoride-induced bone damage through the PI3K/AKT pathway
Received
24th October 2019
, Accepted 8th December 2019
First published on 12th December 2019
Abstract
Bone is the main target of fluorosis, and it has been perfectly elaborated that a moderate dosage of calcium (Ca) can alleviate bone fluorosis. However, whether Ca can alleviate fluorosis through the phosphatidylinositol 3 kinase (PI3K)/protein kinase B (AKT) signaling pathway has not yet been reported. Hence, we evaluated the histopathological structure, the imbalance of the biochemical index of bone metabolism, and the expression levels of PI3K/AKT apoptosis signaling pathway-related genes in rats treated with sodium fluoride (NaF, F) and/or calcium carbonate (CaCO3) for 120 days. Our results suggest that 100 mg L−1 NaF induced histopathological injury as alkaline phosphatase (ALP) and tartrate-resistant acid phosphatase (StrACP) activity increased, with a decrease in the serum Ca levels (p < 0.05). Moreover, the results of qRT-PCR and western blotting showed that F increased the expression levels of transglutaminase 2 (TGM2), focal adhesion kinase (FAK), PI3K, AKT, forkhead box O1 (Foxo1), Bcl-2 interacting mediator of cell death (BIM), Bcl2-associated x protein (Bax) and Caspase 3 (p < 0.05, p < 0.01). It also decreased the expression of AnnexinA5 (Anxa5), 3′-phosphoinositide-dependent kinase 1 (PDK1) and B-cell lymphoma-2 (Bcl-2) (p < 0.05, p < 0.01), which finally activated the PI3K/AKT pathway. On the other hand, CaCO3 supplementation reversed the histopathological injury along with the levels of ALP, StrACP and serum Ca, alleviating the gene expression levels of PI3K/AKT pathway-related markers. Altogether, we can conclude that CaCO3 supplementation mitigated F-induced bone damage via the PI3K/AKT signaling pathway.
Introduction
Fluoride in inorganic or organic forms is the most active non-metallic element, and is widely distributed in natural resources, such as air, soil, water, food, and rock.1 Excessive intake of F can cause fluorosis, with skeletal fluorosis being the main feature.2,3 Many publications have reported that fluorosis of the tibiae collagen fibers present loose and crooked rows, along with non-uniform, dying and widened gaps in the bone lacuna, which finally lead to reduced bone plasticity.4,5 Recent reports have indicated that F may cause tissue damage through the mTOR autophagy pathway, phosphatidylinositol 3 kinase (PI3K)/protein kinase B (AKT) pathway,6 p38MAPK signaling pathway,7 Hedgehog signaling pathway,8etc. There are numerous literatures to prove that calcium (Ca) or Ca-containing compounds can have an appropriate preventive and therapeutic effect on fluorosis,9,10 which can significantly mitigate endoplasmic reticulum stress as well as mitochondrial dysfunction-induced bone damage.10,11 Also, recent studies have shown that the activation of the PI3K/AKT pathway induced by oxidative stress12 can regulate endoplasmic reticulum stress13 and mitochondrial dysfunction.14 It has been indicated that the PI3K-AKT pathway is involved in mitochondrial dysfunction induced by oxidative stress caused by momordin Ic. Furthermore, the mitochondrial disruption was blocked by PI3K activator pretreatment.15 Through inhibition of the PI3K-AKT signaling pathway, endoplasmic reticulum stress was induced by Wogonin in HL-60 leukemia cells.16 However, it is still unclear whether Ca can inhibit bone damage caused by fluorosis through the PI3K/AKT pathway.
The PI3K/AKT pathway is well-known to be anti-apoptotic, where PI3K activates AKT and 3′-phosphoinositide-dependent kinase 1 (PDK1), sequentially recruiting several downstream targets including glycogen synthase kinase-3β (GSK-3β), the proapoptotic forkhead box O1 (Foxo1) transcription factors, Bcl-2 interacting mediator of cell death (BIM), and several antiapoptotic effectors such as B-cell lymphoma-2 (Bcl-2) and some pro-apoptotic factors such as Caspase 3.17 Foxo1, a transcription factor in the FOXO family, plays role in cell cycle control, apoptosis, as well as metabolism, etc., as one of the downstream signals of AKT.18,19
In a previous study, we isolated and identified 17 skeletal differential proteins in rats exposed to NaF alone and/or combination with Ca by two-phase electrophoresis (2-DE) proteomics.20 In that study, two differential proteins were screened, including transglutaminase 2 (TGM2) and annexin A5 (Anxa5) related to the PI3K/AKT signaling pathway.20 TGM2 is present in the cytoplasm, nucleus, membrane and mitochondria of various tissues and organs.21 TGM2 expression can activate focal adhesion kinase (FAK) and further activate anti-apoptotic signaling pathways such as PI3K/AKT to promote cell proliferation.22,23 In addition, TGM2 secretes extracellular regulation factors and regulates the formation of cellular matrices, affecting cell–cell interactions.24 Anxa5 is a widely-expressed and highly-abundant intracellular protein in human that acts in numerous membrane-related events along exocytotic and endocytotic pathways.25 Furthermore, it has been reported that Anxa5 can regulate cell proliferation and apoptosis through the PI3K/AKT signaling pathway.26,27
To further demonstrate whether Ca alleviates F-induced skeletal toxicity through the PI3K/AKT signaling pathway or not, we aimed to explore the effect of NaF alone, as a fluorosis model, and/or in combination with 0.5% and 1% CaCO3 in this study. Quantitative real-time PCR (qRT-PCR) and western blotting (WB) were used to detect the expression changes in TGM2, Anxa5 and PI3K/AKT signaling pathway-related genes and proteins, respectively, to provide a theoretical basis for the effective treatment of skeletal fluorosis.
Materials and methods
Animals
Forty healthy 4-week-old male Sprague-Dawley (SD) rats (120 ± 20 g), were obtained from the Experimental Animal Center of Shanxi Medical University (Shanxi, China).
The treatments were as follows: Control (C) (normal diet and distilled water), sodium fluoride (NaF, F) (normal diet and distilled water with 100 mg L−1 NaF), F + 0.5% Ca (distilled water with 100 mg L−1 NaF and diet with 0.5% CaCO3), F + 1% Ca (distilled water with 100 mg L−1 NaF and diet with 1% CaCO3), respectively and treated for 120 days. All rats were kept in a suitable environment (22–25 °C temperature, 55 ± 5% humidity, 12 h light/dark cycle). After orbital bleeding, the blood was centrifuged at 3000 rpm min−1 for 15 min, and the upper serum was collected. The thigh bones were stored at −80 °C for qRT-PCR and WB. Two samples of each group were fixed in 4% paraformaldehyde solution for bone histopathology. All animal procedures were performed in accordance with the Guidelines for Care and Use of Laboratory Animals of Shanxi Agricultural University and approved by the Animal Ethics Committee of Shanxi Agricultural University.
Bone histopathological analysis
Hematoxylin & eosin (HE) staining was used for histopathological examination. After being soaked in ethylene diamine tetraacetic acid (EDTA; Tianjin, China), the decalcified bone was cut into blocks in the longitudinal direction. After the bone was dehydrated in ethanol and transparent to xylene, it was embedded in paraffin for staining sections by conventional methods.
Bone metabolism indicators
The serum Ca levels, alkaline phosphatase (ALP) and tartrate-resistant acid phosphatase (StrACP) activity were measured with radioimmunoassay kits obtained from Nanjing Jiancheng Bioengineering Institute (Nanjing, China), in accordance with the instructions.
Quantitative real-time PCR (qRT-PCR)
After 0.1–0.3 g bone was ground in a mortar filled with liquid nitrogen, the bone fines were added to 1 ml TRizol for RNA extraction (Takara, Dalian, China). Total RNA was reversely transcribed at 37 °C for 15 min, 85 °C for 5 seconds and 4 °C for 10 min with the PrimeScript™ kit (Takara, Dalian, China). Quantitative real-time PCR (qRT-PCR) was performed by the QuantStudio 7 Flex qRT-PCR system (Life Technologies, USA) using the SYBR® Premix Ex Taq™ II Kit (Takara, Dalian, China).
The primer sequences were retrieved from the NCBI and were designed using Primer 3 plus, according to their mRNA CDS sequences of genes (Table 1). β-Actin was applied as a housekeeping gene, the 2−ΔΔCT method was used for calculating the relative expression levels of the target genes. The pre-denaturation reaction conditions were 95 °C for 30 s, followed by 40 polymerase chain reaction (PCR) cycles of 95 °C for 5 s denaturation, 60 °C for 30 s annealing and 72 °C for 30 s extension. At last, the dissociation protocol was 1 cycle of 95 °C for 15 s, 60 °C for 1 min, and 95 °C for 15 s.
Table 1 Primer sequences for qRT-PCR
Gene |
Primers sequences (5′ > 3′) |
Product (bP) |
Accession no. |
β-Actin
|
Forward: TGACAGGATGCAGAAGGAGA |
104 |
NM_031144.3 |
Reverse: TAGAGCCACCAATCCACACA |
TGM2
|
Forward: TCGCTCTTGCTCTCCAG |
94 |
NM-019386.2 |
Reverse: CAGGCTGGAAGGAGAAGATG |
Anxa5
|
Forward: CACCATCTTTGGGACACGC |
100 |
NM_013132.1 |
Reverse: ACTTCACGACAGCCAGGAGC |
FAK
|
Forward: AGCAGTAATGAGCCAACCAC |
92 |
NM-0130812 |
Reverse: GAGGCGAAATCCATAGCAG |
PI3K
|
Forward: GCTTAGCCTCCCTCTACTCACA |
100 |
NM_053481.2 |
Reverse: GCAGCAAAGAAGAATGGTCAC |
PDK1
|
Forward: TGGTCACAAGAACTCCGACC |
105 |
NM_031081.1 |
Reverse: CTTGTGAGCATTCCCGCTTG |
AKT
|
Forward: GAGACCGACACCAGGTATTTT |
92 |
NM_033230.2 |
Reverse: TGAGTAGGAGAACTGGGGAAA |
Foxo1
|
Forward: AACCAGTCCAACTCGACCAC |
132 |
NM_001191846.2 |
Reverse: TTCTAGCAGGCTCAGGTTGC |
BIM
|
Forward: AGGAACCTGAAGATCTGCGC |
95 |
NM_171988.2 |
Reverse: AAACGCCCTCCTCGTGTAAG |
Bax
|
Forward: GGACAACAACATGGAGCTGC |
99 |
NM_017059.2 |
Reverse: CCGTCTGCAAACATGTCAGC |
Bcl-2
|
Forward: ATGATAACCGGGAGATCGTG |
135 |
NM-016993.1 |
Reverse: CAGGCTGGAAGGAGAAGATG |
Caspase 3
|
Forward: ACTCTACAGCACCTGGTTACTATTC |
101 |
NM_009810.3 |
Reverse: TTCCGTTGCCACCTTCC |
Western blotting
Femoral tissue (100 mg) was taken near the proximal end of the rat femoral head with a bone clamp, ground to a powder with liquid nitrogen, and homogenized in RIPA lysis buffer mixed with PMSF as a volume ratio of 99
:
1 (Beyotime Biotechnology, Shanghai, China). After standing at 4 °C for 30 min, the solution was centrifuged at 12
000 rpm min−1 at 4 °C for 15 min and the resultant supernatants were collected and used for the next step. Protein concentrations of samples were determined at λ = 562 nm using a BCA kit (Beyotime Biotechnology, Shanghai, China).
In brief, 60 μg bone protein was added to 10% SDS polyacrylamide electrophoresis gels (in the running buffer). Afterward, the injected proteins with different molecular masses and charges were separated according to isoelectric points. The electrophoretically separated components were then transferred from the gel to the NC membrane. The NC membrane was placed face-up in 5% skim milk powder for blocking and incubated at 4 °C overnight with a primary antibody diluted to the appropriate concentration, followed by incubation with secondary antibody for 2 h. Primary antibodies, including mouse anti-beta actin monoclonal antibody (1
:
1500), rabbit anti-TGM2 polyclonal antibody (1
:
500), rabbit anti-Anxa5 polyclonal antibody (1
:
1000), rabbit anti-PI3K polyclonal antibody (1
:
1000), rabbit anti-p-PI3K (p85) monoclonal antibody (1
:
800), rabbit anti-p-AKT (Phospho-T308) monoclonal antibody (1
:
1000), rabbit anti-Foxo1polyclonal antibody (1
:
800), rabbit anti-Bcl-2 polyclonal antibody (1
:
1000) and rabbit anti-Caspase 3 polyclonal antibody (1
:
1000), were purchased from Proteintech Group (Wuhan, China). Rabbit anti-AKT1 antibody (1
:
1500) was purchased from Bioss (Beijing, China). The electrochemiluminescence (ECL) reagent was evenly coated on the NC membrane for 2 min. Images were collected and the target band was analyzed via optical density using a Fluor Chem Q System (Alpha Innotech, CA, USA). All blots were repeated at least three times.
Immunofluorescence (IF) and immunohistochemistry (IHC)
For IF staining, bone sections were deparaffinized and put in a 95 °C water bath for 15 min in 0.01 mol L−1 sodium citrate buffer for antigen retrieval, then dipped in PBS and blocked with 5% BSA for 1 h at 37 °C. Afterward, the primary antibodies were added to the sections and left overnight at 4 °C, then incubated with FITC-conjugated secondary antibody (Proteintech Group, Wuhan, China) for 2 h in the dark. Finally, slices were sealed with anti-fluorescent quencher containing DAPI and images were taken by a fluorescence microscope (BioTek Cytation 5, USA).28
For IHC staining, bone sections were soaked in 3% H2O2 for 15 min to break down peroxidase. Then, the sections were boiled for antigen retrieval, blocked and incubated with primary antibodies (similar with IF staining). After being rinsed in PBS, the sections were incubated with secondary antibody for 20 min at RT. Subsequently, the sections were stained with 3,3′-diaminobenzidine (DAB) for 2 min and the nuclei were stained with hematoxylin for 10 s, and sealed with neutral resin (Beyotime Biotechnology, Shanghai, China). Images were observed using a biomicroscope (Olympus, Japan). Optical densities of positive cells were determined by Image-Pro® Plus (Media Cybernetics Inc., USA).
Statistical analysis
Statistical analysis was performed using GraphPad Prism-6. Data are expressed as the mean ± SEM of at least six independent experiments. One-way analysis of variance (ANOVA) was used to account for statistical differences, and when p < 0.05, the differences were determined to be statistically significant.
Results
Change in bone histopathology
The trabecular bone showed a uniform appearance with a cable-like structure in the C group. However, the trabeculae were broken unevenly in the F group. Compared with the F group, the trabecular bone in the F + Ca groups was more regular, showing a strip-like structure (Fig. 1).
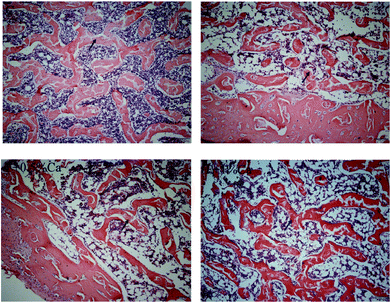 |
| Fig. 1 Effects of NaF treatment and Ca supplementation on rat bone histopathology. HE staining was used to observe the histopathology alterations and bone injuries. In the HE stain, arrows indicate bone trabeculae. Scale bars = 100 μm. | |
Effects of Ca on biochemical indicators related to bone metabolism
Results of serum Ca, ALP and StrACP are presented in Fig. 2. An increasing trend in the ALP and StrACP activity was recorded in the F group as compared to those in the C group (P > 0.05). Compared with the F group, ALP was reduced in the F + Ca groups (p < 0.05). Meanwhile, serum Ca was obviously enhanced in the supplementation with 1% Ca as compared with the F group (p < 0.05). Nevertheless, StrACP and serum Ca had no notable changes in the F + 0.5% Ca group as compared to the F group.
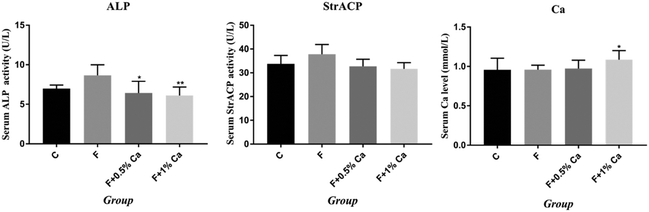 |
| Fig. 2 Effects of NaF treatment and Ca supplementation on rat bone metabolism-related biochemical indicator levels. Serum alkaline phosphatase (ALP) activity, serum tartrate-resistant acid phosphatase (StrACP) activity and serum Ca levels in rats of each group are depicted as mean ± SEM. *p < 0.05, **p < 0.01, as compared with the F group. | |
The mRNA expression levels in the TGM2, Anxa5 and PI3K/AKT pathway
The mRNA expression levels of TGM2, Anxa5, FAK, PI3K, PDK1, AKT, Foxo1, BIM, Bcl2-associated x protein (Bax), and Bcl-2, as well as Caspase 3 related to the PI3K/AKT pathway, are shown in Fig. 3. Compared to the C group, the mRNA expression levels of Anxa5, PDK1, and Bcl-2 were markedly reduced (p < 0.05) in F-treated rats. However, the relative mRNA expressions of Anxa5 and Bcl-2 were notably enhanced in the F + Ca groups as compared with the F group (p < 0.05). In addition, the mRNA expressions of TGM2, FAK, PI3K, AKT, Foxo1, Bax and Caspase 3 were obviously increased in the F group as compared with the C group (p < 0.05) but were obviously decreased in the F + 1% Ca group as compared to the F group (p < 0.05). However, TGM2, FAK, PI3K and AKT mRNA levels were markedly decreased in the F + 0.5% Ca group (p < 0.05) whereas, compared with the F group, the change in BIM mRNA expression was not significant in the other three groups.
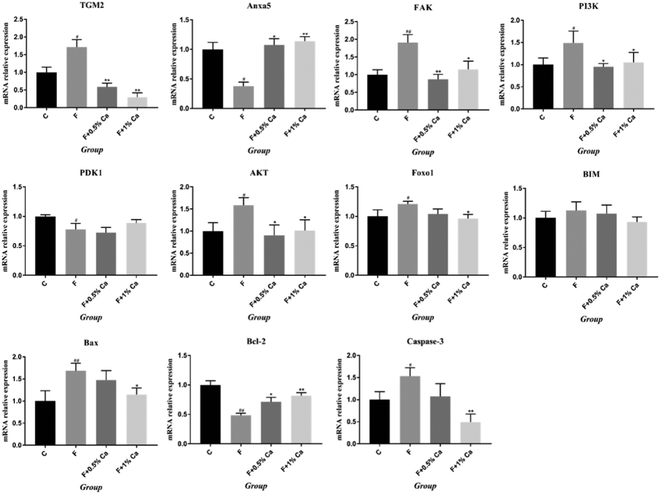 |
| Fig. 3 The expression levels of the apoptosis-related-gene, PI3K/AKT signaling pathway, in rat bone after treatment with NaF and Ca. #p < 0.05, ##p < 0.01, as compared with the control group; *p < 0.05, **p < 0.01, as compared with the F group. | |
The protein expressions of TGM2, Anxa5 and PI3K/AKT pathways
The protein expression levels of TGM2, Anxa5, PI3K, AKT, Foxo1, Bcl-2, Caspase 3, p-PI3K/β-actin, p-PI3K/PI3K, p-AKT/β-actin, and p-AKT/AKT were detected by WB (Fig. 4A and B). The protein expressions of TGM2, PI3K, AKT, Foxo1 and Caspase 3 were obviously enhanced in the F group as compared with the C group (p < 0.05), but were obviously reduced in the F + Ca groups, as compared with the fluoridated rats (p < 0.05). However, the protein expressions of Anxa5, Bcl-2, p-PI3K/β-actin, p-PI3K/PI3K, p-AKT/β-actin, and p-AKT/AKT were notably decreased in the F group, compared with the C group (p < 0.05). The protein expression levels of Anxa5, p-PI3K/β-actin, p-PI3K/PI3K and p-AKT/AKT, were markedly enhanced in the F + Ca groups when compared to the F group (p < 0.05).
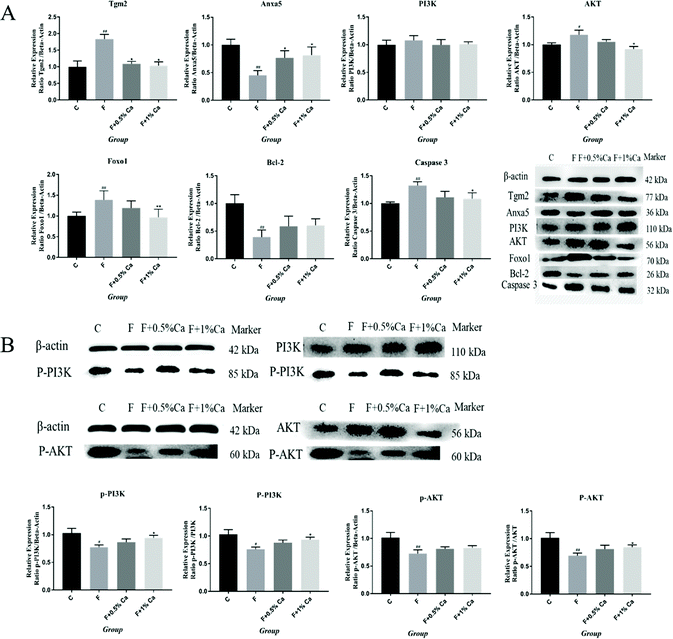 |
| Fig. 4 Western blotting was used to examine the apoptosis proteins related to the PI3K/AKT signaling pathway in rat bone after treatment with NaF and Ca supplementation. (A) The western blotting results of Tgm2, Anxa5, PI3K, AKT, Foxo1, Bcl-2 and Caspase 3. (B) The relative expression of p-PI3K and p-AKT was quantified by p-PI3K/β-actin, p-PI3K/PI3K, p-AKT/β-actin and p-AKT/AKT. #p < 0.05, ##p < 0.01, as compared with the control group; *P < 0.05, **P < 0.01, as compared with the F group. | |
The Anxa5 protein expression levels were determined with immunofluorescence (Fig. 5A). Anxa5 expression was notably reduced in the F group as compared with the C group (p < 0.05), while considerably increased in the F + 1% Ca group (p < 0.05), as compared with the F group. Foxo1 and Caspase 3 protein expression (Fig. 5B and C) were significantly increased in the F group as compared to the C group (p < 0.01). Compared to the F group, the Foxo1 protein level was considerably decreased in the F + 1% Ca group (p < 0.05). Anxa5, Foxo1 as well as Caspase 3 expression levels were consistent with that of WB (Fig. 4A).
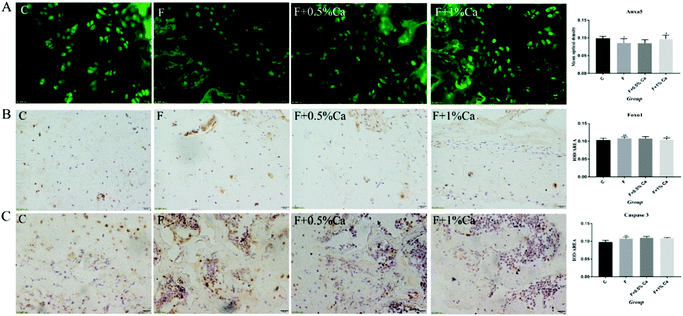 |
| Fig. 5 Protein expressions of three selected markers Anxa5, Foxo1 and Caspase 3 on rat bone challenged with NaF and Ca. (A) Representative images of Anxa5 immunofluorescent staining of the bones of rats treated with NaF and Ca. (B and C) Representative images of Foxo1 and Caspase 3 immunohistochemistry (IHC) staining of bone; brownish-yellow granules are shown as positive cells. Scale bars are 20 μm. Quantitative results of protein expression in bone; the bar graph shows the mean optical density and the values are presented as means ± SEM. #p < 0.05, ##p < 0.01, as compared with the control group; *p < 0.05, **p < 0.01, as compared with the F group. | |
Discussion
Fluoride is an environmental pollutant with cumulative toxicity and its harmful effects are mainly characterized as dental fluorosis and skeletal fluorosis.29 In this study, it has been shown that 120 days of 100 mg L−1 F exposure might increase the trabecular area in rats, while 1% CaCO3 supplementation could ameliorate this bone injury, which is a result similar to that obtained in previous reports.10,30
When the body takes in excess fluoride, F− will replace the phosphate in the bone salt and precipitate in the form of calcium fluoride (CaF2) on bones, teeth and other tissues.31 In the early stages of skeletal fluorosis, a high dose of F stimulates the osteoblast, thus reducing the Ca2+ concentration in the blood, resulting in hypocalcemia.31,32 By supplementing Ca nutrition, the toxicity of fluorine can be antagonized. Similar to our observations, other reports have also shown that CaCO3 supplementation might decrease the deposition of F in the bone by maintaining normal Ca level in the blood.33 In addition, relative studies revealed that the serum ALP and StrACP activities can indicate the changes in bone metabolism induced by F exposure.34 In this study, F considerably increased the activity of ALP and StrACP; however, previous studies have demonstrated that Ca supplementation can reduce ALP and StrACP activity,35 which is consistent with our results. It can be concluded that supplementation with a suitable Ca dosage effectively alleviated the fluorosis, namely 1% CaCO3.
Recent studies indicated that F causes bone damage through various pathways such as anti-apoptotic-related PI3K/AKT pathways.6 Studies have also reported that Anxa5 regulates cell proliferation and apoptosis via the PI3K/AKT signaling pathway.26 As a major intracellular Ca2+-binding protein, Anxa5 has been known to regulate the cell cycle and cell proliferation, as well as invasion in a number of tumor types.36,37 Ravassa S reported that the degree of heart failure in patients with Anxa5 and myocardial synchronized therapy was negatively correlated with Anxa5 in plasma; on the other hand, Anxa5 was found to promote cardiomyocyte apoptosis by promoting AKT and p38 phosphorylation, activation of Caspase 3 and inhibition of the anti-apoptotic effects of Bcl in vitro studies.38 In the present study, F exposure significantly decreased mRNA and protein expressions of Anxa5 in bone, while Ca supplementation notably reversed the adverse effects of F on Anxa5. Therefore, it is suggested that Anxa5 promotes osteoblast survival against apoptotic stress in a PI3K-dependent manner.
In addition, TGM2 also activates anti-apoptotic signaling pathways such as PI3K/AKT to promote cell proliferation.22,23 TGM2 is ubiquitously expressed and exerts a variety of normal physiological functions, such as wound healing, apoptosis, and cell adhesion, by targeting specific substrates.39 The relationship between TGM2 and apoptosis has been well established. Overexpression of TGM2 leads to spontaneous apoptosis or sensitivity of cells to apoptosis-inducing agents.40 Many studies have shown that TGM2 expression in PDAC cells led to the activation of FAK and its downstream PI3K/AKT pathway.41 Increased expression of TGM2 is correlated with increased cell motility and adhesion,42 and cell adhesion induces the activation of FAK-dependent signaling.43 It has been shown that microRNA inhibited cell growth and induced apoptosis in human cervical cancer via the PI3K/AKT pathway by increasing the expression levels of FAK.44 Tatsukawa et al. found that TGM2 enhances apoptosis with alcoholic steatohepatitis in vivo.45 In this study, F exposure significantly increased mRNA and protein expressions of TGM2 and FAK in bone, while the effect of F on TGM2 and FAK was significantly reversed by Ca supplementation. These observations are in accordance with the previous studies that reported that the PI3K/AKT pathway plays a critical role against apoptosis. Hence, we can conclude that Ca mitigates the adverse effects of NaF through the alleviation of the activation of PI3K, AKT, TGM2 and FAK.
As a survival and anti-apoptotic signaling pathway, the PI3K/AKT pathway plays a crucial role.17 Composed of the regulatory subunit (p85) and the catalytic subunit (P110), PI3K is a heterodimer.17 AKT is a key factor and important anti-apoptotic regulator in the downstream signaling pathways of PI3K, which produces an extensive biological effect mainly through phosphorylation.46 PI3K recruits and activates AKT, PDK1 and monomeric G-proteins, leading to the activation of several downstream targets including GSK-3β, mTOR, Foxo1, and several antiapoptotic effectors. It is reported that the mRNA and proteins expression levels of PI3K, as well as AKT1, were obviously enhanced in fluorosis hepatocytes, while the phosphorylated PI3K (p-PI3K) and phosphorylated AKT (p-AKT) was lower.46 Additionally, research has shown that the C2C12 cells’ PDK1 expression was notably decreased after F treatment.6 The current studies were more reliable, with the F-induced increase in the expressions of PI3K, AKT and decreased expressions of p-PI3K, p-AKT, PDK1, while Ca supplementation inhibited the harmful effects of F on them.
Foxo1, one of the downstream signals of AKT, regulated animal growth, cell differentiation, metabolism as well as apoptosis signal transduction as a transcription factor.47 Similar to our observations, it was found that upon exposure to NaF, Foxo1 mRNA and protein expression levels were considerably enhanced in the LS8 cells.47 These results suggest that Ca regulates the expression of the PI3K/AKT signaling pathway-related genes and proteins to attenuate skeletal cell apoptosis in fluorosis in rats. All together, these findings signify that Ca attenuates the F-induced bone damage through regulating the PI3K/AKT signaling pathway.
Bcl-2, Bax and Caspase 3 are well-known downstream apoptotic regulators of AKT that are of vital importance in the regulation of apoptosis.48,49 Studies have suggested that with an increase in the NaF dose, the Bcl-2 expression was inhibited while the Bax expression was considerably increased, which induced the apoptosis of MC3T3-E1 osteoblasts.49 Furthermore, it has been shown that the Caspase 3 mRNA expression was notably up-regulated in the thymus of F-treated female rats.50 In our previous research, the expression of Bcl-2 was inhibited with the increased expression of Bax as well as Caspase 3 in the bones of rats subjected to F, while Ca dietary supplementations changed the expression of these pivotal genes.10 These published results are consistent with this study, and it was further demonstrated that Ca attenuates skeletal cell apoptosis in fluorosis in rats by activating PI3K/AKT signaling.
In conclusion, excessive F exposure affects the synthesis of collagen fibers, destroys osteoblasts, and thus affects skeletal and biochemical markers related to bone metabolism. TGM2 and Anxa5 induced activation of the downstream PI3K/AKT survival pathway. However, a 1% CaCO3 supplementation diet decreased the damage to bone, and further relieved bone metabolism and apoptosis via the PI3K-AKT signaling pathway (Fig. 6).
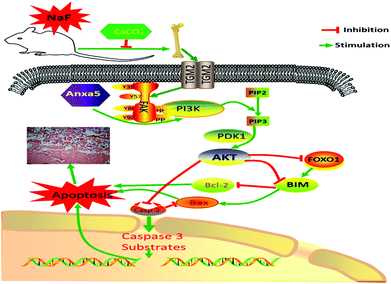 |
| Fig. 6 Effects of PI3K/AKT pathways in F-induced apoptosis and bone injury. TGM2 and Anxa5 induced activation of the downstream PI3K/AKT survival pathway. The pathway, including downstream targets and transcription factors such as Foxo1, BIM, Bcl-2 and Caspase 3, promote or inhibit the apoptosis of cells. Arrows indicate up-regulated genes or proteins, “T” symbols indicated down-regulated genes or proteins. | |
Conflicts of interest
The authors declare that there are no conflicts of interest in this research project.
Acknowledgements
This research was supported by the China National Natural Science Foundation (Grant No. 31972751), Shanxi Province Excellent Doctoral Work Reward Fund (Grant No. SXYBKY201731), Shanxi Province innovation project for graduate students (Grant No. 2019SY219).
References
- S. K. Jha, V. K. Mishra, D. K. Sharma and T. Damodaran, Fluoride in the Environment and Its Metabolism in Humans, Rev. Environ. Contam. Toxicol., 2011, 211(211), 121–142 CrossRef CAS.
- S. T. Sebastian, R. R. Soman and S. Sunitha, Prevalence of dental fluorosis among primary school children in association with different water fluoride levels in Mysore district, Karnataka, Indian J. Dent. Res., 2016, 27(2), 151–154 CrossRef.
- A. H. Mahvi, M. Yousefi and A. A. Mohammadi, Fluoride concentration level in rural area in Poldasht city and daily fluoride intake based on drinking water consumption with temperature, Data Brief, 2017, 13(C), 312–315 Search PubMed.
- X. Yan, X. Hao, Q. Nie, C. Feng, H. Wang, Z. Sun, R. Niu and J. Wang, Effects of fluoride on the ultrastructure and expression of Type I collagen in rat hard tissue, Chemosphere, 2015, 128, 36–41 CrossRef CAS.
- M. I. Jimenez-Cordova, M. Cardenas-Gonzalez, G. Aguilar-Madrid, L. C. Sanchez-Pena, A. Barrera-Hernandez, I. A. Dominguez-Guerrero, C. Gonzalez-Horta, O. C. Barbier and L. M. Del Razo, Evaluation of kidney injury biomarkers in an adult Mexican population environmentally exposed to fluoride and low arsenic levels, Toxicol. Appl. Pharmacol., 2018, 352, 97–106 CrossRef CAS.
- B. H. Zhou, P. P. Tan, L. S. Jia, W. P. Zhao, J. C. Wang and H. W. Wang, PI3K/AKT signaling pathway involvement in fluoride-induced apoptosis in C2C12 cells, Chemosphere, 2018, 199, 297–302 CrossRef CAS.
- Z. Shi, Y. Zhan, J. Zhao, J. Wang and H. Ma, Effects of Fluoride on the Expression of p38MAPK Signaling Pathway-Related Genes and Proteins in Spleen Lymphocytes of Mice, Biol. Trace Elem. Res., 2016, 173(2), 333–338 CrossRef CAS.
- Z. J. Zhu, Y. U. Yan-Ni, X. Tao, D. O. Pathology and G. M. University, Role of Hh signaling pathway in fluoride-induced primary chondrocyte damage in rats, Chin. J. Public Health, 2015, 31(5), 574–578 CAS (in Chinese).
- J. Wang, J. Yang, X. Cheng, F. Yin, Y. Zhao, Y. Zhu, Z. Yan, F. Khodaei, M. M. Ommati, R. K. Manthari and J. Wang, Influence of Calcium Supplementation against Fluoride-Mediated Osteoblast Impairment in Vitro: Involvement of the Canonical Wnt/beta-Catenin Signaling Pathway, Agric. Food Chem., 2019, 67(37), 10285–10295 CrossRef CAS.
- J. Wang, J. Yang, X. Cheng, R. Xiao, Y. Zhao, H. Xu, Y. Zhu, Z. Yan, M. M. Ommati, R. K. Manthari and J. Wang, Calcium Alleviates Fluoride-Induced Bone Damage by Inhibiting Endoplasmic Reticulum Stress and Mitochondrial Dysfunction, Agric Food Chem., 2019, 67(39), 10832–10843 CrossRef CAS.
- Y. Zhang, K. Zhang, L. Ma, H. Gu, J. Li and S. Lei, Fluoride induced endoplasmic reticulum stress and calcium overload in ameloblasts, Arch. Oral Biol., 2016, 69(Complete), 95–101 CrossRef CAS.
- X. Yang, X. Zhang, J. Zhang, Q. Ji, W. Huang, X. Zhang and Y. Li, Spermatogenesis disorder caused by T-2 toxin is associated with germ cell apoptosis mediated by oxidative stress, Environ. Pollut., 2019, 251, 372–379 CrossRef CAS.
- L. J. Li, C. Ye, X. J. Guo, S. L. Chu and L. S. Zhang, Effects of endoplasmic reticulum stress on autophagy and apoptosis of human leukemia cells via inhibition of the PI3K/AKT/mTOR signaling pathway, Mol. Med. Rep., 2018, 17(6), 7886–7892 CAS.
- D. Li, D. Li, X. Wei, X. Wei, M. Ma, M. Ma, H. Jia, H. Jia, Y. Zhang and Y. Zhang, FFJ-3 inhibits PKM2 protein expression via the PI3K/Akt signaling pathway and activates the mitochondrial apoptosis signaling pathway in human cancer cells, Oncol. Lett., 2017, 13(4), 2607–2614 CrossRef CAS.
- J. Wang, L. Yuan, H. Xiao, C. Xiao, Y. Wang and X. Liu, Momordin Ic induces HepG2 cell apoptosis through MAPK and PI3K/Akt-mediated mitochondrial pathways, Apoptosis, 2013, 18(6), 751–765 CrossRef CAS.
- C. Hu, M. Xu, R. Qin, W. Chen and X. Xu, Wogonin induces apoptosis and endoplasmic reticulum stress in HL-60 leukemia cells through inhibition of the PI3K-AKT signaling pathway, Oncol. Rep., 2015, 33(6), 3146–3154 CrossRef CAS.
- Z. Wu, A. Huang, J. Yan, B. Liu, Q. Liu, J. Zhang, X. Zhang, C. Ou and M. Chen, Resveratrol ameliorates cardiac dysfunction by inhibiting apoptosis via the PI3K/Akt/FoxO3a pathway in a rat model of diabetic cardiomyopathy, J. Cardiovasc. Pharmacol., 2017, 70(3), 184–193 CrossRef CAS.
- P. K. Battiprolu, B. Hojayev, N. Jiang, Z. V. Wang, X. Luo, M. Iglewski, J. M. Shelton, R. D. Gerard, B. A. Rothermel and T. G. Gillette, Metabolic stress–induced activation of FoxO1 triggers diabetic cardiomyopathy in mice, J. Clin. Invest., 2012, 122(3), 1109–1118 CrossRef CAS.
- J. Nakae, W. H. Biggs, T. Kitamura, W. K. Cavenee, C. V. E. Wright, K. C. Arden and D. Accili, Regulation of insulin action and pancreatic β-cell function by mutated alleles of the gene encoding forkhead transcription factor Foxo1, Nat. Genet., 2002, 32(2), 245–253 CrossRef CAS.
- J. Wang, H. Xu, Z. Yan, J. Yang, Y. Zhu, X. Cheng and J. Wang, Separation and identification of differential protein in rat's bone with fluorosis and calcium supplementation intervention, Chin. J. Biotechnol., 2019, 35(06), 1097–1108 Search PubMed (in Chinese).
- R. L. Eckert, M. T. Kaartinen, M. Nurminskaya, A. M. Belkin, G. Colak, G. V. W. Johnson and K. Mehta, Transglutaminase Regulation of Cell Function, Physiol. Rev., 2014, 94(2), 383–417 CrossRef CAS.
- S. Mishra and L. J. Murphy, The p53 oncoprotein is a substrate for tissue transglutaminase kinase activity, Biochem. Biophys. Res. Commun., 2006, 339(2), 726–730 CrossRef CAS.
- K. Tanaka, Y. Yokosaki, F. Higashikawa, Y. Saito, A. Eboshida and M. Ochi, The integrin α5β1 regulates chondrocyte hypertrophic differentiation induced by GTP-bound transglutaminase 2, Matrix Biol., 2007, 26(6), 409–418 CrossRef CAS.
- S. Mishra, G. Melino and L. J. Murphy, Transglutaminase 2 Kinase Activity Facilitates Protein Kinase A-induced Phosphorylation of Retinoblastoma Protein, J. Biol. Chem., 2007, 282(25), 18108–18115 CrossRef CAS.
- V. Gerke and S. E. Moss, Annexins: From Structure to Function, Physiol. Rev., 2002, 82(2), 331–371 CrossRef CAS.
- C. Ji, H. Guo, P. Zhang, W. Kuang, Y. Fan and L. Wu, AnnexinA5 promote glioma cell invasion and migration via the PI3K/Akt/NF-κB signaling pathway, J. Neuro-Oncol., 2018, 138(3), 469–478 CrossRef CAS.
- J. Tang, Z. Qin, P. Han, W. Wang, C. Yang, Z. Xu, R. Li, B. Liu, C. Qin and Z. Wang, High Annexin A5 expression promotes tumor progression and poor prognosis in renal cell carcinoma, Internet J. Oncol., 2017, 50(5), 1839–1847 CrossRef CAS PubMed.
- J. Zhang, Y. Zhu, C. Liang, M. Qie, R. Niu, Z. Sun, J. Wang and J. Wang, Effects of Fluoride on Expression of P450,
CREM and ACT Proteins in Rat Testes, Biol. Trace Elem. Res., 2017, 175(1), 156–160 CrossRef CAS.
- Y. Liu, C. Liang, Y. Gao, S. Jiang, Y. He, Y. Han, A. Olfati, R. K. Manthari, J. Wang and J. Zhang, Fluoride Interferes with the Sperm Fertilizing Ability via Downregulated SPAM1, ACR, and PRSS21 Expression in Rat Epididymis, Agric. Food Chem., 2019, 67(18), 5240–5249 CrossRef CAS.
- Y. Wei, B. Zeng, H. Zhang, C. Chen, Y. Wu, N. Wang, Y. Wu, D. Zhao, Y. Zhao and J. Iqbal, Comparative proteomic analysis of fluoride treated rat bone provides new insights into the molecular mechanisms of fluoride toxicity, Toxicol. Lett., 2018, 291, 39–50 CrossRef CAS.
- J. M. T. Cate, Review on fluoride, with special emphasis on calcium fluoride mechanisms in caries prevention, Eur. J. Oral Sci., 1997, 105(5), 461–465 CrossRef CAS.
- M. Jyotsana, J. P. Puri and J. K. Singh, Growth, feed efficiency and blood profile of buffalo calves consuming high levels of fluoride: growth and blood metabolites in buffaloes fed high fluoride, Trop. Anim. Health Prod., 2009, 41(3), 295–298 CrossRef.
- F. Zhu, Z. Liu and Y. Ren, Mechanism of melatonin combined with calcium carbonate on improving osteoporosis in aged rats, Exp. Ther. Med., 2018, 16(1), 192–196 Search PubMed.
- Y. E. Song, H. Tan, K.-j. Liu, Y.-z. Zhang, Y. Liu, C.-r. Lu, D.-l. Yu, J. Tu and C.-y. Cuie, Effect of fluoride exposure on bone metabolism indicators ALP, BALP, and BGP, Environ. Health Prev. Med., 2011, 16(3), 158–163 CrossRef CAS.
- C. S. Haworth, A. M. Jones, J. E. Adams, P. L. Selby and A. K. Webb, Randomised double blind placebo controlled trial investigating the effect of calcium and vitamin D supplementation on bone mineral density and bone metabolism in adult patients with cystic fibrosis, J. Cystic Fibrosis, 2004, 3(4), 233–236 CrossRef CAS.
- D. W. Shin, Y. J. Kwon, D. J. Ye, H. S. Baek, J. E. Lee and Y. J. Chun, Auranofin Suppresses Plasminogen Activator Inhibitor-2 Expression through Annexin A5 Induction in Human Prostate Cancer Cells, Biomol. Ther., 2017, 25(2), 177–185 CrossRef CAS.
- N. Park and Y.-J. Chun, Auranofin Promotes Mitochondrial Apoptosis by Inducing Annexin A5 Expression and Translocation in Human Prostate Cancer Cells, J. Toxicol. Environ. Health, Part A, 2014, 77(22–24), 1467–1476 CrossRef CAS.
- R. Susana, G. B. Ignacio, Z. Amaia, M. Alfonso, J. J. Gavira, B. Javier, A. Teresa, H. Ana and D. Javier, Cardiac resynchronization therapy-induced left ventricular reverse remodelling is associated with reduced plasma annexin A5, Cardiovasc. Res., 2010, 88(2), 304–313 CrossRef.
- S. S. Akimov and A. M. Belkin, Cell surface tissue transglutaminase is involved in adhesion and migration of monocytic cells on fibronectin, Blood, 2001, 98(5), 1567–1576 CrossRef CAS.
- L. Fesus and M. Piacentini, Transglutaminase 2: an enigmatic enzyme with diverse functions, Trends Biochem. Sci., 2002, 27(10), 534–539 CrossRef CAS.
- V. Amit, W. Huamin, M. Bramanandam, J. Y. Fok, A. P. Mann, K. Rakesh and M. Kapil, Increased expression of tissue transglutaminase in pancreatic ductal adenocarcinoma and its implications in drug resistance and metastasis, Cancer Res., 2006, 66(21), 10525–10533 CrossRef.
- J. F. Herman, L. S. Mangala and K. Mehta, Implications of increased tissue transglutaminase (TG2) expression in drug-resistant breast cancer (MCF-7) cells, Oncogene, 2006, 25(21), 3049–3068 CrossRef CAS.
- B. Zita, V. Elisabetta, C. Russell, G. Stephane, A. Julian and G. Martin, Analysis of tissue transglutaminase function in the migration of Swiss 3 T3 fibroblasts: the active-state conformation of the enzyme does not affect cell motility but is important for its secretion, J. Biol. Chem., 2002, 277(19), 16567–16575 CrossRef PubMed.
- J. Xu, W. Zhu, L. Chen and L. Liu, MicroRNA-433 inhibits cell growth and induces apoptosis in human cervical cancer through PI3K/AKT signaling by targeting FAK, Oncol. Rep., 2018, 40(6), 3469–3481 CAS.
- H. Tatsukawa, Y. Fukaya, G. Frampton, A. Martinez-Fuentes, K. Suzuki, T. F. Kuo, K. Nagatsuma, K. Shimokado, M. Okuno and J. Wu, Role of Transglutaminase 2 in Liver Injury via Cross-linking and Silencing of Transcription Factor Sp1, Gastroenterology, 2009, 136(5), 1783–1795 CrossRef CAS.
- F. Bin, Y. Yanni and Z. Ying, PI3K-Akt1 expression and its significance in liver tissues with chronic fluorosis, Int. J. Clin. Exp. Pathol., 2015, 8(2), 1226–1236 Search PubMed.
- J. Li, L. Zhao, X. Zhao, P. Wang, Y. Liu and J. Ruan, Foxo1 Attenuates NaF-Induced Apoptosis of LS8 Cells through the JNK and Mitochondrial Pathways, Biol. Trace Elem. Res., 2018, 181(1), 104–111 CrossRef CAS.
- D. D. Lou, Z. Z. Guan and J. J. Pei, Alterations of apoptosis and expressions of Bax and Bcl-2 in the cerebral cortices of rats with chronic fluorosis, Fluoride, 2014, 47(3), 199–207 CrossRef CAS PubMed.
- S. Yang, Z. Wang, C. Farquharson, R. Alkasir, M. Zahra, G. Ren and B. Han, Sodium fluoride induces apoptosis and alters bcl-2 family protein expression in MC3T3-E1 osteoblastic cells, Biochem. Biophys. Res. Commun., 2011, 410(4), 910–915 CrossRef CAS PubMed.
- H. W. Wang, B. H. Zhou, J. L. Cao, X. L. Gu, C. F. Cao and J. D. Wang, Effects of dietary protein and calcium on thymus apoptosis induced by fluoride in female rats (Wistar rats), Environ. Toxicol., 2010, 24(3), 218–224 CrossRef PubMed.
|
This journal is © The Royal Society of Chemistry 2020 |
Click here to see how this site uses Cookies. View our privacy policy here.